Abstract
Purpose of review
Noroviruses (NoVs) are the most common cause of epidemic and sporadic cases of acute gastroenteritis worldwide. This review summarizes recent NoV disease burden estimates, epidemiology findings and provides an update on virus-like particle (VLP) vaccine studies.Recent findings
NoVs are the leading cause of food-borne gastroenteritis and are replacing rotavirus as the predominant gastrointestinal pathogen in pediatric populations. Genogroup II, genotype 4 NoVs (GII.4) remain the dominant genotype worldwide. Increased NoV activity reported in late 2012 was associated with the emergence of a new GII.4 variant called Sydney 2012. New studies suggest that human NoVs can bind a larger range of histoblood group antigens, a susceptibility factor for NoV illness, thus expanding the susceptible population pool for infection. Intranasal immunization with a monovalent GI NoV VLP vaccine showed proof-of-concept efficacy. Studies using intramuscular immunization with a bivalent formulation including GII.4 VLPs are now underway.Summary
The importance of NoVs as a major gastrointestinal pathogen underscores the need for well tolerated and effective vaccines. Results of VLP vaccine trials appear promising. However, the rapid evolution of NoV genotypes through antigenic drift and changing glycan specificities provide new challenges to epidemiology studies and vaccine trials.Free full text

Epidemiology of human noroviruses and updates on vaccine development
Abstract
Purpose of review
Noroviruses (NoVs) are the most common cause of epidemic and sporadic cases of acute gastroenteritis worldwide. This review summarizes recent NoV disease burden estimates, epidemiology findings and provides an update on virus-like particle (VLP) vaccine studies.
Recent findings
NoVs are the leading cause of food-borne gastroenteritis and are replacing rotavirus as the predominant gastrointestinal pathogen in pediatric populations. Genogroup II, genotype 4 NoVs (GII.4) remain the dominant genotype worldwide. Increased NoV activity reported in late 2012 was associated with the emergence of a new GII.4 variant called Sydney 2012. New studies suggest that human NoVs can bind a larger range of histoblood group antigens, a susceptibility factor for NoV illness, thus expanding the susceptible population pool for infection. Intranasal immunization with a monovalent GI NoV VLP vaccine showed proof-of-concept efficacy. Studies using intramuscular immunization with a bivalent formulation including GII.4 VLPs are now underway.
Summary
The importance of NoVs as a major gastrointestinal pathogen underscores the need for well tolerated and effective vaccines. Results of VLP vaccine trials appear promising. However, the rapid evolution of NoV genotypes through antigenic drift and changing glycan specificities provide new challenges to epidemiology studies and vaccine trials.
INTRODUCTION
Recent epidemiological studies demonstrate the immense burden of noroviruses (NoVs) as a leading cause of gastroenteritis across all settings and age groups [1,2]. NoV epidemiology is complex and influenced by many factors, including population immunity, virus evolution, the environment and seasonality. The highly infectious nature of NoVs and their ability to remain stable in the environment pose multiple challenges to infection control practices. The development of NoV vaccines may provide an approach to reduce disease burden [3,4]. This article summarizes recent estimates on NoV disease burden, reviews new developments in human NoV epidemiology, and progress and challenges in the development of virus-like particle (VLP) vaccines.
STRUCTURE AND CLASSIFICATION
NoVs are positive sense, single-stranded RNA viruses belonging to the family Caliciviridae. The genus Norovirus is divided into six genogroups (GI–GVI), and viruses in genogroups I, II and IV are known to infect humans (Fig. 1a) [5,6]. Seroepidemiology studies detect antibodies to GIII bovine NoVs in human populations; however, this may be because of cross reactive epitopes between bovine and human NoVs as bovine NoVs bind to a glycan not present in human tissues [7,8].
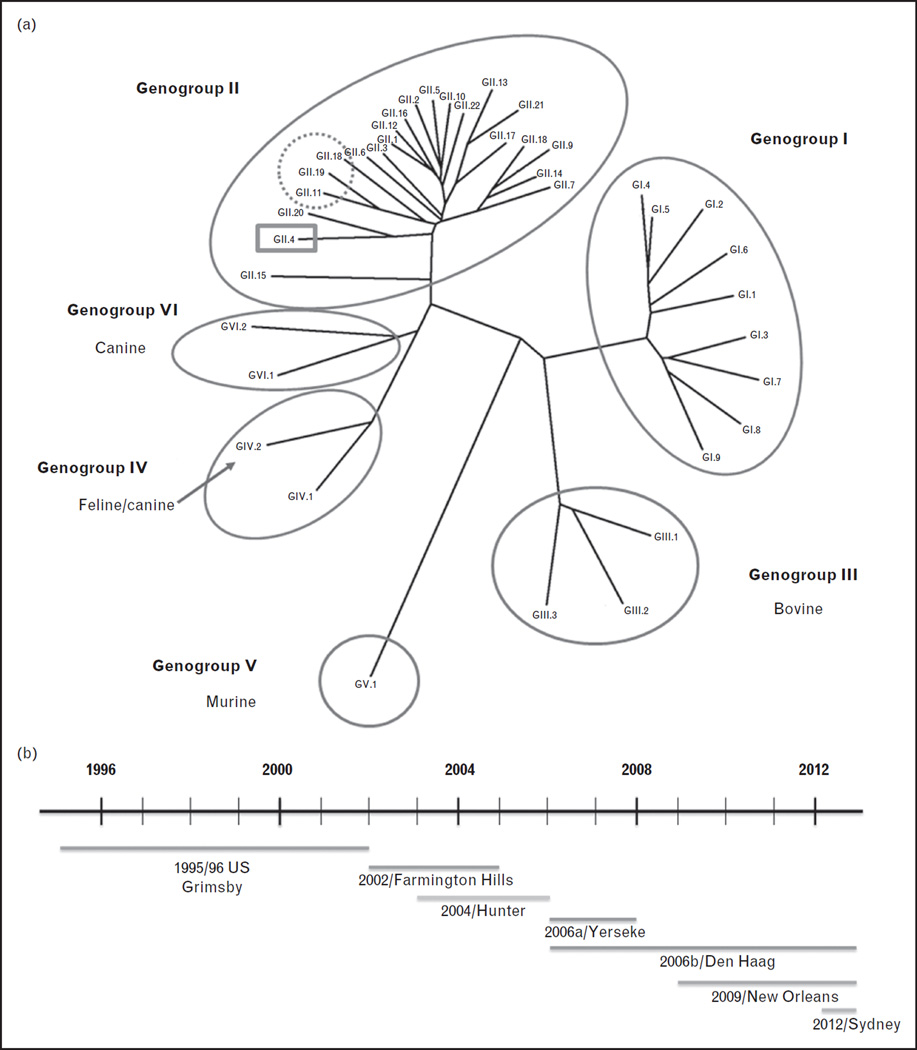
Phylogenetic analysis of noroviruses (NoVs). (a) NoVs are classified into genogroups and genotypes based on the amino acid sequence of the capsid protein VP1. Human NoVs belong to genogroups I, II and IV and some porcine viruses are also detected within GII (dotted circle). The predominant human NoV genotype described worldwide is GII.4 (highlighted in the grey box). New variants emerge every 2–3 years and replace the previously dominant GII.4 variant. (b) From the 1990s to 2013, seven different GII.4 variants have been associated with global epidemics of gastroenteritis. These include the US 1996/96 variant in 1996, the Farmington Hills variant in 2002, the Hunter variant in 2004, the 2006a, 2006b variants in 2007–08, the New Orleans variant from 2009 to 2012 and Sydney 2012 in late 2012. The timeline of emergence and circulation of predominant GII.4 variants is shown. The line above the variant name indicates when the variant emerged to when it was last detected.
Subdivision of genogroups into genotypes was based on greater than 15% pairwise difference in the amino acid sequence of the capsid protein VP1 [9]. To account for within-genotype variation, classification of VP1 sequences is now proposed to rely on phylogeny-based clustering analyses [5]. Genetic drift of the VP1 gene can occur within a genotype, leading to the emergence of new variants; this phenomenon is best recognized among genogroup II, genotype 4 (GII.4) strains but may also occur in other genotypes such as GII.2 and GI.3 [10,11]. Assignment of new genotypes and variants is to be coordinated by an international working group of NoV experts. With increasing evidence for recombination among NoV genotypes, the working group has proposed a dual nomenclature system using both VP1 and open reading frame 1 sequences to include accurate genotyping of recombinant viruses [5].
BURDEN OF DISEASE
NoV studies include four distinct epidemiological categories: outbreaks of food-borne and water-borne gastroenteritis, infections in the immunocompromised and the elderly, sporadic cases of acute gastroenteritis in children below 5 years of age, and sporadic cases of acute gastroenteritis among adults [12]. Historically, the best recognized of these are the gastroenteritis outbreaks. NoVs are the most common cause of food-borne gastroenteritis in the United States, identified in over 58% of reported outbreaks [13]. NoV epidemiology of nonfood-borne outbreaks was clarified after a National Outbreak Reporting System Network was established. Of 4455 outbreaks reported from 2009 to 2010, NoV was identified in 1908 of 2819 (68%) single-etiology outbreaks, and thus was the leading cause of single-etiology outbreaks [14]. NoVs were associated with 69 145 illnesses, 1093 hospitalizations and 125 deaths in these outbreaks, thus accounting for 78% of all illnesses, 46% of hospitalizations and 86% of deaths. The majority of NoV outbreaks (48.8%) occurred in healthcare facilities. Severe outcomes of NoV infections are more likely to occur in these settings and indeed, most NoV-associated deaths are reported in the elderly in healthcare facilities [15]. Although NoV illness is usually self-limiting, prolonged infection and virus shedding has been documented in immunocompromised persons (reviewed in [16]). In contrast with the winter peaks of NoV illness seen in immunocompetent persons, infections may occur year round in immunosuppressed hosts. The possibility of severe outcomes and the effect on quality of life in these vulnerable populations make it essential to use rigorous precautionary measures and reduce risk to this population.
Sporadic cases of gastroenteritis caused by NoVs are predominantly reported in children below 5 years of age. NoVs account for approximately 12% of diarrheal hospitalizations in developed and developing countries, and are the second most common viral cause of gastroenteritis after rotavirus in this age group in many countries [17,18]. In pediatric populations in industrialized countries in which effective rotavirus vaccines are used, NoVs are rapidly replacing rotavirus as the most common cause of viral gastroenteritis. An 8.5-year-long study on enteric viruses at a large pediatric hospital before and after rotavirus vaccine introduction documented a 64% decrease in cases of rotavirus gastroenteritis after vaccine introduction, with NoV becoming the most common viral enteric pathogen [19]. Similarly, an overall decline in gastroenteritis calls after rotavirus vaccine introduction was documented in a telephone triage program and the peak call proportions then correlated with regional NoV outbreaks [20]. In a prospective surveillance study carried out in three counties in the United States in 2009 and 2010, NoV was detected in 21% of children with acute gastroenteritis presenting to hospitals, emergency departments and outpatient clinics, far exceeding cases of rotavirus gastroenteritis (12%) [21
]. These new data indicate that the burden of NoV gastroenteritis in children is higher than previously estimated. Changed shedding dynamics of these viruses is also documented by lower rotavirus prevalence and higher NoV prevalence in wastewater samples from hospital and community settings following rotavirus vaccine introduction in Nicaragua [22]. Contrasting with the epidemiology data published from many developing countries [17,18], NoVs were not identified as a major gastrointestinal pathogen in children in five of the seven countries in a Global Enteric and Malnutrition Study (GEMS) [23]. Whether NoVs were associated with less severe diarrhea and did not meet the inclusion criteria for the GEMS or whether the multiplex PCR used for NoV detection was less sensitive than real-time reverse transcriptase-PCR used elsewhere remains unclear.
Less is documented about sporadic cases of NoV gastroenteritis among adults. Large studies on the incidence and cause of infectious intestinal disease in the United Kingdom between 1993–1996 and 2008–2009 [24] found an increased incidence of NoV gastroenteritis among individuals in a community cohort and those presenting to a general practitioner. Incidence rates rose from approximately 7% in both settings to 16.4% in the community and 12.4% in cases presenting to general practitioners, similar to the rates of 16% in the community and 12% among outpatient cases reported in the United States [1].
Overall disease incidence estimates suggest NoV gastroenteritis results in 570–800 deaths, 56 000–71 000 hospitalizations, 400 000 emergency department visits, 1.7–1.9 million outpatient visits and 19–21 million total illnesses annually in the United States [2]. The economic impact is high with food-borne NoV illness costing $2 billion for healthcare and loss of productivity whereas NoV-associated hospitalizations cost about $500 million in the United States [25
]. These studies reiterate the growing recognition of NoVs as important public health pathogens across all settings, with the increased recognition of disease burden attributed to increased awareness, better surveillance and use of more sensitive PCR-based diagnostic techniques.
EPIDEMIOLOGY OF NOROVIRUS GENOTYPES
Most human NoV infections are caused by GII strains. Water-borne outbreaks are more likely to be caused by GI NoVs whereas GII strains are involved in food-borne outbreaks or person-to-person transmission [26]. A correlation between water-borne outbreaks and GI strains may reflect GI viruses being more stable in water for prolonged time periods [27,28].
A striking feature of the epidemiology of NoV genotypes is the predominance of GII.4 strains. GII.4 variants are responsible for approximately 55–85% of the gastroenteritis cases worldwide. Severe outcomes of hospitalizations and deaths are more likely to be caused by GII.4 NoVs [29]. From the 1990s to early 2013, seven different GII.4 variants were associated with global epidemics of gastroenteritis (Fig. 1b). New variants emerged every 2–3 years and largely replaced the previously dominant GII.4 variant but not other endemic strains. Heightened NoV activity is often associated with the emergence of new variants [30]. In late 2012, emergence of a new GII.4 variant, called Sydney 2012, caused increased NoV activity in several parts of the world [31]. Outbreaks of gastroenteritis due to Sydney 2012 virus received widespread, global media coverage. Continued surveillance for NoV outbreaks through the Noro-Net and CaliciNet systems enables assessment of the public health impact of this new GII.4 variant [32].
The worldwide predominance and persistence of GII.4 variants and GII.4 strain emergence and replacement is a fascinating observation that remains to be fully understood but has been attributed to several factors, including antigenic drift, greater person-to-person transmission and escape from herd immunity [33,34]. The evolution of new GII.4 variants may be primarily because of immune pressure that drives antigenic variation in the P2 domain of VP1, which binds histoblood group antigens (HBGAs) [35]. HBGAs serve as cell attachment factors for NoVs and the association between HBGA expression on epithelial surfaces and susceptibility to certain human NoVs is well documented. Reduced infection and illness is observed in persons with serum antibodies that block strain-specific NoV VLP binding to HBGAs [36–38]. HBGA expression on cell surfaces is affected by the ABO, Secretor and Lewis genotypes of an individual. In general, GII.4 viruses can bind a larger range of HBGAs in comparison to other genotypes. Thus, GII.4 NoVs have a larger susceptible population pool for infection. Nonsecretor individuals are largely resistant to infection with many human NoV genotypes including GII.4 NoV variants, although one nonsecretor was reported to be infected in an outbreak in Spain in 2004 [39]. Recent biochemical, structural and epidemiological studies indicate additional glycan specificities for many NoV genotypes [40–42]. Some GII.4 variants post 2002 could bind to nonsecretor HBGAs including Lewis fucose [40,43,44]. This indicates the potential for GII.4 NoVs to infect Lewis positive nonsecretors. Indeed, in an outbreak of GII.4 gastroenteritis in China, 7% of nonsecretors were infected by a GII.4 virus that was highly similar to one variant shown to bind nonsecretor HBGAs in vitro [45]. More recently, a secretor negative and Lewis negative child was infected with a GII.4 variant in Burkina Faso, Africa, indicating that binding to the Lewis fucose may also not be mandatory [46]. Although currently limited, these reports are important in the context of increased potential for infection with the newer GII.4 variants and defining who constitutes the susceptible population pool for these strains.
New data on glycan specificities are not restricted to the widely circulating GII.4 NoVs. Several GI viruses (GI.2, GI.3, GI.4 and GI.8 VLPs) bind to Lewis a (Lea) antigen that is expressed by nonsecretors [47]. X-ray crystallographic studies support the in-vitro binding assays with a GI.2 strain showing binding to Lea and other HBGAs. The interaction is predicted to be mediated by a longer P-loop and a glutamine in amino acid position 389 that is lacking in GI.1 NoV that does not bind Lea [48]. These findings raise questions on how sequence variability and structural changes alter glycan specificities for different NoVs and whether strains with similar structural changes will be able to bind nonsecretor HBGAs. In a recent outbreak of GI.3 NoV infection in Sweden, individuals were infected irrespective of their ABO, secretor or Lewis status [49]. Biochemical studies with a similar GI.3 NoV demonstrated binding to multiple glycans [47]. These developments provide new avenues to explore the reasons behind any unusual NoV activity. For example, increases in the number of outbreaks due to GI.6 NoVs have been reported in the United States [50]. A dramatic increase in GI NoV activity from 7.8% in the last decade to 37% since June 2012 was reported in Alberta, Canada [51]. These outbreaks were predominantly caused by GI.6 and GI.7 NoVs. It remains to be seen if such changes in epidemiology correlate with changes in glycan specificities of these viruses. Overall, understanding the molecular basis for this wider range of HBGA–host interactions is important to evaluate the basis of evolution of these viruses.
Data from GII.4, GII.2 and GI.2 indicate that immune pressure may drive amino acid changes that can play a significant role in affecting glycan binding [11,40,48]. Interestingly, immune suppression also can result in the production of multiple genetic variants [52]. Using next generation sequencing of samples from immunocompetent and immunocompromised hosts, a single variant was predominant in the former whereas multiple variants were detected in the immunocompromised host. Intergenotype and intragenotype recombination events also may contribute to NoV evolution [10]. In addition, infections with human GII.4 strains have been described in livestock [53]. In attempts to establish animal models of human NoVs, GII.4 infections have been described in gnotobiotic pigs, calves and mice [54–56]. These studies raise further questions about the ease of transmissibility of GII.4 viruses.
UPDATE ON VACCINES TO NOROVIRUS
The high and increasing burden of NoV disease supports the need for well tolerated, effective methods to prevent infection and illness. Expression of recombinant NoV capsid proteins results in the assembly of VLPs that are morphologically and antigenically identical to the infectious virion [57]. Since their first description, VLPs have been proposed as NoV vaccine candidates. Preclinical studies in mice showed VLPs are immunogenic when delivered through intranasal, oral or parenteral routes, inducing serum and mucosal immunity and with better mucosal immune responses achieved when coadministered with a mucosal adjuvant [58]. Initial phase I clinical studies assessed the safety and immunogenicity of oral immunization with increasing dosages of GI.1 Norwalk virus VLPs in adults [59,60]. Following these studies, an intranasal route for VLP administration was evaluated. Two doses of a dry powder formulation adjuvanted with monophosphoryl lipid A (MPL) and the mucoadherent chitosan were tested for safety and immunogenicity in adults [61]. The vaccine was well tolerated with no severe adverse events and was immunogenic, showing a dose-dependent increase in serum antibody titers. Functional antibodies were induced and IgA and IgG antibody secreting cells showed homing potential to the gut mucosa and to peripheral lymphoid tissue. This study demonstrated, for the first time, the production of a B-cell memory response to an enteric pathogen in response to intranasal immunization, and the frequency of antigen-specific memory B cells correlated with serum antibody responses [62].
The intranasal vaccine with Norwalk virus VLPs was tested in a proof-of-concept efficacy trial in healthy, secretor-positive adults who received two doses of vaccine or placebo 3 weeks apart, followed by challenge with homotypic live virus given at approximately 10 times the dose required to infect 50% of the participants [36]. Vaccinated individuals were less likely to develop gastroenteritis compared with placebo recipients (37 vs. 69%, respectively, P = 0.006) and infections were also lower in the vaccine arm (61 vs. 82% respectively, P = 0.05). Among vaccinees that developed gastroenteritis, delayed onset of illness and overall reduction in disease severity were observed.
These proof-of-concept studies are important for showing that a NoV VLP vaccine can induce protective immunity. However, they tested the GI.1 genotype, and the most common genotypes worldwide are GII.4 viruses. Cross-challenge studies using GI and GII viruses carried out in the 1970s failed to demonstrate heterotypic protection, suggesting an effective vaccine might need to include VLPs from both GI and GII genogroups. To address this, a new GII.4 VLP was designed by aligning the VP1 sequence from three human GII.4 viruses [63]. Preclinical studies of the new VLPs, designated as consensus VLPs, showed that intramuscular immunization of a bivalent formulation including GI.1 and consensus VLPs induced higher antibody levels than the intranasal route of immunization.
The first studies on intramuscular immunization with the bivalent vaccines in adult volunteers are completed; no severe adverse events were reported in a dose escalation study [64]. The vaccine was immunogenic, with IgA and IgG responses to both VLPs seen 7 days after the first dose of vaccine. The second dose did not further boost the antibody response. IgA and IgG ASC responses were observed, with a bias toward IgA. Like the previous intranasal study, mucosal homing and chemokine receptor phenotype were observed. Immunogenicity of a single dose of this bivalent formulation evaluated in adults from different age groups – 18–49, 50–64 and 65–85 years – showed lower fold change in antibody response in the higher age groups [65]. A new GII.4 NoV challenge pool will test the efficacy of this bivalent formulation [66]. Immunogenicity data from the human clinical trials carried out with the baculovirus-expressed VLPs are summarized in Table 1.
Table 1
Summary of immunogenicity data from human clinical trials carried out with baculovirus expressed NoV VLPs
Study (Ref.) | Vaccine | Route of immunization | Assessment end points | VLP concentration (µg) | Serum IgA GMFR (95% CI) | Serum IgG GMFR (95% CI) | HAI/HBGA blocking antibody GMFR (95% CI) |
---|---|---|---|---|---|---|---|
Ball et al. [59] | GI.1 (Norwalk) | Oral | Safety, immunogenicity | 100 | 3.0 (1.4–6.5) | 5.3 (1.3–22.3) | Not tested |
250 | 4.4 (2.1–9.2) | 8.8 (5.4–14.2) | Not tested | ||||
Placebo | 1.4 (0.7–2.7) | 1.4 (0.7–2.7) | Not tested | ||||
Tacket et al. [60] | GI.1 (Norwalk) | Oral | Safety, immunogenicity | 250, 500, 2000 | Data not shown | Data not shown | Not tested |
El-Kamary et al. [61] | GI.1 (Norwalk), MPL, Chitosan | Intranasal | Safety, immunogenicity | 5 | 1.2 | 0.9 | Not tested |
15 | 2.5 | 1.9 | Not tested | ||||
50 | 4.5 | 4.7 | Not tested | ||||
MPL+Chitosan control | 1.0 | 1.0 | Not tested | ||||
GI.1 (Norwalk), MPL, Chitosan | Intranasal | Safety, immunogenicity | 50 | 7.6 (4.2–13.8) | 4.6 (2.5–8.6) | HAI/4.0 (2.0–7.9) | |
100 | 9.1 (4.7–17.6) | 4.8 (3.2–7.1) | HAI/9.1 (4.0–20.7) | ||||
MPL+Chitosan control | 1.0 (0.8–1.3) | 1.1 (0.9–1.4) | HAI/1.4 (1.0–1.8) | ||||
Atmar et al. [36] | GI.1 (Norwalk), MPL, Chitosan; Challenge with GI.1 live virus | Intranasal | Safety, immunogenicity and efficacy | 100 | 7.5 (4.6–12.2) | 4.5 (3.1–6.5) | HBGA blocking/3.6 (2.8–4.7) |
Control | 1.0 (0.9–1.0) | 1.0 (0.9–1.0) | HBGA blocking/1.2 (1.0–1.5) | ||||
Frey et al. [64] | GI.1 (Norwalk)+GII.4 (Consensus) MPL, Alum | Intramuscular | Safety, immunogenicity | 5+5, 15+15, 50+50, 150+150 | Analysis on-going | ||
Treanor et al. [65] | GI.1 (Norwalk)+GII.4 (Consensus) MPL, Alum | Intramuscular | Safety, immunogenicity (in three different age groups) | 50+50 | Analysis on-going |
CI, confidence interval; GI, genogroup I; GMFR, geometric mean fold rise; HAI, hemagglutination inhibition; HBGA, histoblood group antigens; VLP, virus-like particle.
The advances in NoV vaccine development are promising. The induction of ASCs showing mucosal homing and production of memory B cells are important findings. However, many questions remain. What is the duration of protection following infection and vaccination? Natural infection may not confer long-term protection as seen in early volunteer studies, although a recent modeling study suggests immunity following infection lasts 4–9 years [67]. Will increasing vaccine immunogenicity increase their effectiveness? Are T-cells and cytokine responses important in the context of infection or vaccination? Will these vaccines be effective in pediatric populations wherein NoVs are a significant burden? Specific for this population, some studies on the development of combined vaccines to rotavirus and NoV are being conducted [68]. The highest mortality rates are seen in the immunocompromised elderly. Will NoV vaccine be effective in this high risk age group? How will the NoV evolution impact the effectiveness of vaccines against newly emerging genotypes or variants? Although the production of broadly cross reactive monoclonal antibodies to NoVs has been described, heterotypic protection was not described in early cross-challenge studies, and it remains unknown if this was caused by use of high dosages of challenge virus. In this context, field efficacy studies will provide an accurate estimate of protection from vaccines in the natural environment in which multiple genotypes of NoVs are present.
CONCLUSION
The public health impact of human NoVs is being increasingly recognized worldwide. As highlighted in the past year, new NoV variants continue to emerge and cause significant illness worldwide. Rapid evolution through antigenic variation and recombination, and new glycan specificities pose new challenges to our understanding of this disease. A striking feature in this context seen with both the recent GII.4 variants as well as GII.2 and GI strains is how amino acid modifications can impact the glycan specificity of NoVs. Such changes can alter the perspective of who are currently considered susceptible to most human NoVs and will have implications for vaccine trials. Many fundamental questions about the biology of these viruses remain unanswered. When will a fully permissive cell culture system or small animal model of human NoVs be developed? Despite these many challenges, several advances have been made in NoV epidemiology and vaccine development in recent years. Significant efforts have been made to obtain accurate measures of disease incidence through the establishment of better surveillance and reporting systems in all settings. NoV VLPs appear to be attractive vaccine candidates and the advances in the area of these vaccines are highly promising.
Acknowledgements
The authors are supported for their work on noroviruses in part from the NIH Public Health Service grants (P01 AI 57788, P30 DK56336), the Agriculture and Food Research Initiative Competitive grant from the United States Department of Agriculture (USDA 2011–68003–30395) and the John S. Dunn Research Foundation.
Footnotes
Conflicts of interest
R.L.A. has received grant support and acted as unpaid consultant for Takeda Pharmaceuticals USA (formerly LigoCyte Pharmaceuticals, Inc.). M.K.E. has a patent on the use of norovirus virus-like particles as a vaccine and has served as a consultant to Ligocyte Pharmaceuticals, Inc. The authors have no other relevant affiliations or financial involvement with any organization or entity with a financial interest in or financial conflict with the subject matter or materials discussed apart from those disclosed.
REFERENCES AND RECOMMENDED READING
Papers of particular interest, published within the annual period of review, have been highlighted as:
of special interest
of outstanding interest
Full text links
Read article at publisher's site: https://doi.org/10.1097/mog.0000000000000022
Read article for free, from open access legal sources, via Unpaywall:
https://europepmc.org/articles/pmc3955997?pdf=render
Citations & impact
Impact metrics
Citations of article over time
Alternative metrics
Smart citations by scite.ai
Explore citation contexts and check if this article has been
supported or disputed.
https://scite.ai/reports/10.1097/mog.0000000000000022
Article citations
Norovirus acute gastroenteritis amongst US and European travellers to areas of moderate to high risk of travellers' diarrhoea: a prospective cohort study.
J Travel Med, 31(7):taad051, 01 Oct 2024
Cited by: 1 article | PMID: 37074164 | PMCID: PMC11500662
Genetic Diversity and Phylogenetic Relationship of Human Norovirus Sequences Derived from Municipalities within the Sverdlovsk Region of Russia.
Viruses, 16(7):1001, 21 Jun 2024
Cited by: 0 articles | PMID: 39066164 | PMCID: PMC11281373
Navigating Viral Gastroenteritis: Epidemiological Trends, Pathogen Analysis, and Histopathological Findings.
Cureus, 16(5):e61197, 27 May 2024
Cited by: 0 articles | PMID: 38939260
Screening for viral pathogens in the gastrointestinal tract from cases of sudden unexpected death in infancy at the Tygerberg Medico-legal Mortuary.
Virol J, 20(1):281, 29 Nov 2023
Cited by: 0 articles | PMID: 38031160 | PMCID: PMC10688011
Standardization of an antiviral pipeline for human norovirus in human intestinal enteroids demonstrates nitazoxanide has no to weak antiviral activity.
Antimicrob Agents Chemother, 67(10):e0063623, 03 Oct 2023
Cited by: 4 articles | PMID: 37787556 | PMCID: PMC10583671
Go to all (113) article citations
Other citations
Similar Articles
To arrive at the top five similar articles we use a word-weighted algorithm to compare words from the Title and Abstract of each citation.
Genetic diversity and epidemiology of Genogroup II noroviruses in children with acute sporadic gastroenteritis in Shanghai, China, 2012-2017.
BMC Infect Dis, 19(1):736, 22 Aug 2019
Cited by: 17 articles | PMID: 31438883 | PMCID: PMC6704660
Molecular epidemiology of genogroup II norovirus infection among hospitalized children with acute gastroenteritis in Suzhou (Jiangsu, China) from 2010 to 2013.
J Med Virol, 88(6):954-960, 10 Dec 2015
Cited by: 11 articles | PMID: 26547266
Molecular epidemiology of noroviruses associated with sporadic gastroenteritis in children in Novosibirsk, Russia, 2003-2012.
J Med Virol, 87(5):740-753, 18 Feb 2015
Cited by: 27 articles | PMID: 25693507
Molecular epidemiology of noroviruses associated with acute sporadic gastroenteritis in children: global distribution of genogroups, genotypes and GII.4 variants.
J Clin Virol, 56(3):185-193, 05 Dec 2012
Cited by: 174 articles | PMID: 23218993
Review
Funding
Funders who supported this work.
NIAID NIH HHS (2)
Grant ID: P01 AI057788
Grant ID: P01 AI57788
NIDDK NIH HHS (3)
Grant ID: P30 DK056338
Grant ID: P30 DK056336
Grant ID: P30 DK56336