Abstract
Free full text

Vaccine-Induced Env V1–V2 IgG3 Correlates with Lower HIV-1 Infection Risk and Declines Soon After Vaccination
Abstract
HIV-1–specific immunoglobulin G (IgG) subclass antibodies bind to distinct cellular Fc receptors. Antibodies of the same epitope specificity but of a different subclass therefore can have different antibody effector functions. The study of IgG subclass profiles between different vaccine regimens used in clinical trials with divergent efficacy outcomes can provide information on the quality of the vaccine-induced B cell response. We show that HIV-1–specific IgG3 distinguished two HIV-1 vaccine efficacy studies (RV144 and VAX003 clinical trials) and correlated with decreased risk of HIV-1 infection in a blinded follow-up case-control study with the RV144 vaccine. HIV-1–specific IgG3 responses were not long-lived, which was consistent with the waning efficacy of the RV144 vaccine. These data suggest that specific vaccine-induced HIV-1 IgG3 should be tested in future studies of immune correlates in HIV-1 vaccine efficacy trials.
INTRODUCTION
The Thai phase 3 (RV144) vaccine regimen, ALVAC prime/bivalent clade B/E recombinant gp120 boost, provided an estimated 31.2% efficacy against the acquisition of HIV-1 infection at 42 months after vaccination (1). V1–V2 immunoglobulin G (IgG) antibody responses correlated with decreased risk of HIV-1 infection (2, 3), and a virus sieve analysis demonstrated immune pressure at position 169 in the V2 loop of gp120 (169K) (4). Notably, there was 60.5% estimated efficacy at 6 months after vaccination (5), suggestive of an early vaccine effect that wanes over time. This highlights the need for a better understanding of the quality of the antibody responses that are induced but not durable, so that further vaccine studies can be designed to specifically boost particular immune responses. The VAX003 clinical trial, in a high-risk injection drug use cohort, containing the same bivalent clade B/E gp120 protein immunogen as RV144, without the ALVAC prime did not show protection (6) despite higher vaccine-elicited neutralizing antibodies (nAbs) compared to RV144 (7). Identifying potential markers of infection risk, as well as determining the differences in the quality of the antibody responses among different vaccine regimens, is critical for designing further immunogens to test specific hypotheses in future efficacy studies.
Antibody subclasses (IgG1 to IgG4) have distinct affinities for Fc receptors (8). Thus, antibodies with the same epitope specificity, but of different subclasses, can have different functional attributes. In particular, IgG3 antibodies can fix complement, have high affinity for FcγRI, FcγRII, FcγRIIa, and FcγRIII, and also have the longest and most flexible hinge region of the IgG subclasses. There is precedence for the role of IgG3 antibodies in immune-mediated pathogen control. Antigen-specific IgG3 antibodies were associated with long-term control of malaria caused by the Plasmodium falciparum parasite (9), as well as clearance and long-term clinical protection from Chikungunya virus (CHIKV) (10). IgG3 antibodies were responsible for monocyte-mediated cellular inhibition of P. falciparum (11) and were associated with CHIKV neutralization (10). Little is known about the potentially protective role of vaccine-elicited IgG3 antibodies for HIV-1, other than that some HIV-1 broadly nAbs [for example, 2F5 and 4E10 monoclonal antibodies (mAbs)] are of IgG3 origin (12) and are associated with different effector functions [for example, antibody-dependent cellular cytotoxicity (ADCC), neutralization (13), and complement fixation (14)].
Here, we demonstrate that Env IgG3 responses mark a qualitative difference in immune response between two HIV-1 vaccine regimens with divergent efficacy outcomes in human volunteers. We show that Env IgG3 responses correlate with decreased infection risk in a correlates analysis and are part of an immediate vaccine-induced humoral response that quickly wanes.
RESULTS
IgG subclass profiles between RV144 and VAX003 vaccine regimens are distinct
It was previously reported that nAbs (7) were higher in a protein boost vaccine strategy (VAX003) compared to vector prime/protein boost (RV144). Thus, we examined whether there was a form of antibody response that might be higher in RV144 that was not apparent when measuring total IgG. We examined each of the IgG subclass responses (IgG1 to IgG4) to HIV-1 envelope proteins (vaccine strain and consensus envelope proteins) for both RV144 [ALVAC prime and two protein boosts, visit 8 (V8)] and VAX003 [four protein boosts, visit 9 (V9)] and also after two protein boosts for VAX003 [visit 5 (V5)]. In addition to examining vaccine strain responses, we also included group M and clade AE consensus envelopes. ConS is a consensus of the consensus sequences of each subtype, central to all circulating clades, and reacts well with sera from all subtypes including AE (15–17). Moreover, the group M consensus envelope was similar to autologous envelopes in detection of the initial antibody response in acute infections and is sensitive for determining vaccine immunogenicity in vaccine trials (2, 18). Env IgG1 response rates were generally higher in VAX003 (V9) compared to RV144 (V8) (Fig. 1). We examined vaccine strain antigens (A244 gp120, MN gp120, and 92TH023 gp120), a clade B envelope (GNE8 gp120), and consensus envelope antigens [AE Con gp140 and ConS gp140 (group M)] to determine the quality of the vaccine-induced antibody response. At V9, the VAX003 vaccine induced significantly higher IgG2 and IgG4 response rates (Fig. 1). In contrast, the frequency of some envelope IgG3 responses was higher in RV144 compared to VAX003, most predominantly when compared at V9. Although Env IgG3 responses were induced by VAX003 and the frequency of responders was elevated at V5, the Env IgG3 responses declined by V9 and were neither maintained nor elevated, after two additional protein boosts. This decline in the IgG3 response rate was in stark contrast to those for IgG1, IgG2, and IgG4 that were generally boosted to higher response rates and magnitudes by V9 in VAX003.
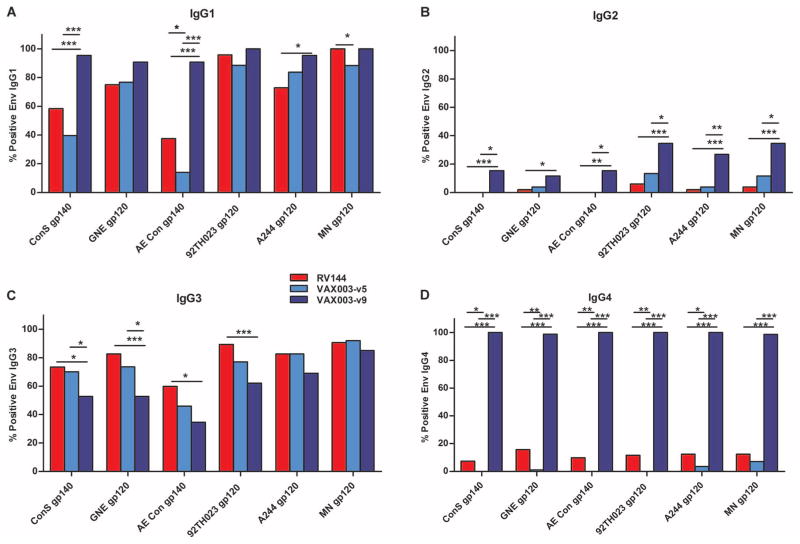
(A to D) HIV-1 Envelope antibody response rates for proteins from the vaccine strain immunogens in the prime (92Th023) and boost (A244, MN) as well as against group M and clade AE consensus proteins (ConS gp140 and AE Con gp140, respectively) (percent responders are shown for RV144 V8 and VAX003 V5 and V9) for IgG1 (A), IgG2 (B), IgG3 (C), and IgG4 (D). ***P < 0.0005; **P < 0.005; *P < 0.05. P values were calculated with the two-tailed Fisher’s exact test and adjusted with the Benjamini-Hochberg correction.
Concentration ranges for HIV-1 antigen–specific IgG subclasses were 0.1 to 55.3 μg/ml (IgG1), 0.6 to 10.5 μg/ml (IgG2), 6.0 × 10−4 to 0.15 μg/ml (IgG3), and 3.0 × 10−3 to 6.5 μg/ml (IgG4) for RV144. In both studies, the concentrations of IgG1 were the highest among the IgG subclasses for both RV144 and VAX003, consistent with relative total concentrations in the blood and HIV-1–specific concentrations found in infected individuals (14, 19) (Fig. 2A). The concentrations of IgG1 specific for the vaccine strain immunogens and to HIV-1 AE and group M consensus antigens were significantly lower in RV144 compared to VAX003 (Fig. 2A). Among the Env-specific IgG subclass responses in RV144, IgG2 and IgG4 were less frequently elicited, with IgG2 being the least frequently detected subclass. The concentrations of Env IgG2 were, overall, lower in RV144 than in VAX003 (Fig. 2B). Similarly, Env IgG4 response rates and concentrations were profoundly lower in RV144 than in VAX003 (Figs. 1D and and2D2D).
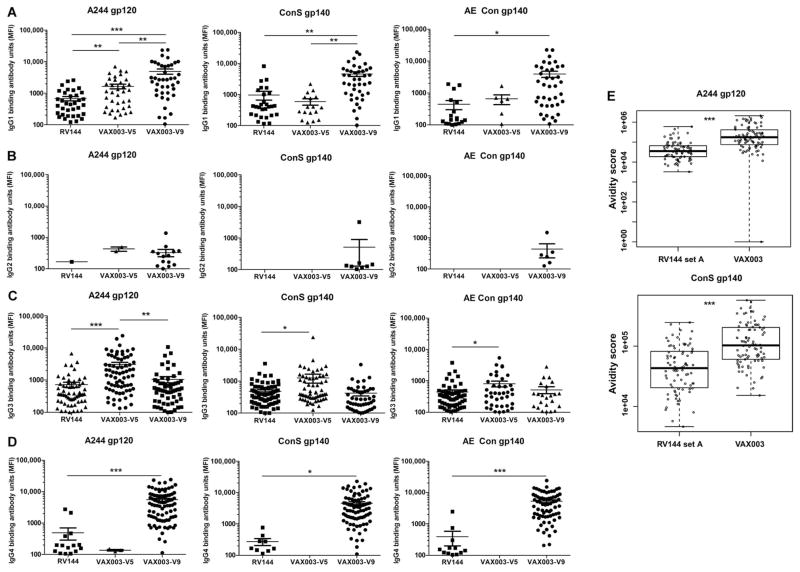
(A) HIV-1 Env IgG1 antibody concentrations were lower in RV144 compared to VAX003. Antibody response magnitude in mean fluorescence intensity (MFI) is shown for positive responders only. (B) HIV-1 Env IgG2 antibody responses were higher in VAX003 than in RV144. (C) HIV-1 Env IgG3 antibody responses were elevated after two protein boosts (V5) in VAX003 and then declined after four protein boosts (V9). (D) HIV-1 Env IgG4 responses were lower in RV144 compared to VAX003. (E) IgG avidity to the vaccine strain antigen, A244, and also to the group M consensus gp140 was measured by surface plasmon resonance (SPR). ***P < 0.0005; **P < 0.005; *P < 0.05. P values for (A) to (D) were calculated with two-tailed t tests. P values for (E) and (F) were calculated with the Wilcoxon rank-based test.
IgG3 response rates were significantly higher (P = 0.0002 to 0.0084, two-tailed Fisher’s exact test) in RV144 compared to VAX003 (for ConS gp140, GNE8 gp120, AE Con gp140, and 92TH023 gp120) at the peak immunogenicity time point based on maximum overall humoral responses (V8 for RV144 and V9 for VAX003) for each trial (Fig. 1C and Table 1A). Among the vaccinees with HIV-1 Env IgG3 responses, the concentrations of Env-specific IgG3 were similar at the peak immunogenicity time point for RV144 and VAX003 (Fig. 2C). Response rates to A244 gp120 and MN gp120, the two proteins used in the RV144 and VAX003 boost, were more similar between the two regimens. We next examined the IgG subclass distribution after two protein boosts to compare to RV144 (ALVAC prime + two protein boosts). The magnitude of the Env IgG3 response was generally elevated after two protein boosts in VAX003 and then declined after four protein boosts (Fig. 2C). This suggests that continued protein/alum boosting may help drive the subclass response toward lower IgG3 and higher IgG4. The IgG antibody avidity score to the vaccine strain immunogens was overall lower in RV144 compared to VAX003 (Fig. 2E). Although each time point was after four immunizations for both VAX003 and RV144, the difference in avidity is likely due to four doses of protein in VAX003 compared to two doses in RV144. Both the resonance units and dissociation rates (Kd) are lower in RV144 compared to VAX003. Therefore, the difference in avidity is not solely due to higher binding responses. Because these measurements were performed on purified polyclonal samples, certain specificities of higher avidity may predominate.
Table 1
IgG3 HIV-1 Env binding antibody differences between RV144 and VAX003. P values <0.05 are in bold.
Type of Env protein | HIV-1 Env protein | RV144-VAX003 V5
| RV144-VAX003 V9
| ||
---|---|---|---|---|---|
P* | % Difference† | P* | % Difference† | ||
Group M consensus envelope protein | ConS gp140 | 0.687 | 3.4 | 0.0084 | 20.6 |
Clade B‡ | GNE8 gp120 | 0.235 | 9.1 | 0.0002 | 29.8 |
Circulating strain in Thailand (AE) consensus | AE Con gp140 | 0.112 | 13.8 | 0.0019 | 25.3 |
RV144 vaccine prime | 92TH023 gp120 | 0.087 | 12.3 | 0.0002 | 27.2 |
RV144 and VAX003 vaccine boost | A244 gp120 | 1.000 | 0.1 | 0.075 | 13.7 |
RV144 and VAX003 vaccine boost | MN gp120 | 0.845 | 1.3 | 0.394 | 5.6 |
HIV-1 IgG3 antibodies correlate with antiviral function
To determine the functional capacity of the vaccine-induced IgG3 response, we examined whether there were correlations between the vaccine-induced IgG3 response and ADCC. We hypothesized that Env IgG3 may have improved FcR-mediated effector function, so we examined the association of high/medium versus low ADCC in association with Env IgG3. There was a significant association of Env IgG3 and V1–V2 antibodies with ADCC levels in RV144, but not VAX003 (Table 2 and fig. S1). The RV144 data are representative of tests with nine antigens (three different envelopes and six V1–V2 antigens) that all showed significant correlations (P = 0.000024 to 0.0172, Wilcoxon rank-based test) with ADCC. The VAX003 data are representative of tests with 11 antigens (7 different envelopes and 4 V1–V2 antigens). All tests for the VAX003 comparison were not significant for any antigen. Only one showed a trend (gp70 B.CaseA2 V1–V2: P = 0.055, Wilcoxon rank-based test). All P values for the RV144 comparison were significant (P = 0.03 to <0.00001, Wilcoxon rank-based test). The correlation with ADCC was measured by two different assays, also strongly correlated with Env and V1–V2 IgG3 responses. These data, along with the study by Chung et al. (20), indicate an antiviral functional role for these vaccine-elicited antibodies. To examine the ability of vaccine-induced IgG3 antibodies to recognize HIV-1 virions, we also examined correlations of the Env IgG3 responses with neutralization of two isolates: MN (clade B RV144 vaccine strain) and C1080 (clade AE, the predominant subtype circulating in Thailand). C1080 is representative of circulating strains in Thailand because it is a tier 2 virus in the TZM-bl assay but exhibits a tier 1 phenotype in A3R5 similar to three of seven circulating Thai AE viruses (7). Neutralization of tier 1 viruses provides some information on the ability of antibodies to recognize virus particles and may be a marker of another functional antiviral response mediated by envelope-specific antibodies. Neutralization significantly correlated with IgG3 antibodies to AE Con gp140 and group M consensus (ConS gp140) (both had an R = 0.52, P < 0.05, Spearman correlation).
Table 2
ADCC responses correlate with IgG3 responses in RV144. The P values are from rank-based two-sample test (Wilcoxon), where the two samples are based on ADCC categorization. Est: The Hodge-Lehmann estimator of the difference in location center, close to difference in median. P values <0.05 are in bold. AUC, area under the curve.
Type of Env protein/sequence information | IgG3 antibody | ADCC high (infected cell assay)
| ADCC AUC high (gp120-coated)
| ||
---|---|---|---|---|---|
Est | P | Est | P | ||
RV144 and VAX003 vaccine boost protein (monomeric) | A244 gp120 | 0.5652 | 0.0013 | 0.5090 | 0.0012 |
V1–V2 from clade C | C.1086 V1–V2 tags | 0.7699 | 0.0003 | 0.4991 | 0.0002 |
V1–V2 from Haynes et al. (2) | gp70 B.CaseA2 V1–V2 | 0.6146 | 0.0172 | 0.9232 | 0.0001 |
V1–V2 with mutation (169K) | gp70 B.CaseA2 V1–V2 169K | 0.4598 | 0.0108 | 0.4921 | 0.0030 |
V1–V2 IgG3 responses are higher in vector prime/protein boost compared to protein-only immunization
Given that V1–V2 IgG antibodies correlated with decreased risk of infection in the RV144 correlates analysis (2), we next tested whether V1–V2 IgG3 binding antibody responses were greater in RV144 vaccine recipients compared to VAX003 vaccinees. RV144 elicited significantly higher V1–V2 IgG3 response rates than VAX003 at both time points [P = 0.039 (V5) and P < 0.0001(V9), two-tailed Fisher’s exact test] (Fig. 3A). However, among those that were positive, the magnitude of the response was higher in VAX003 at V5 (Fig. 3, B and C) (P = 0.0116 to <0.0001, two-tailed t test).
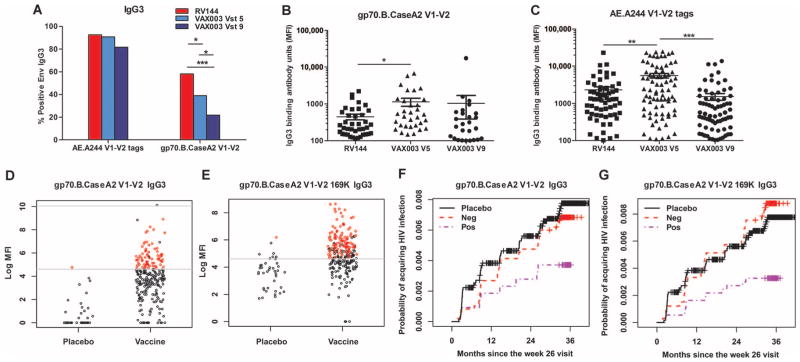
(A) Responders to V1–V2 antigens in RV144 and VAX003. (B and C) Magnitude of V1–V2 responses to RV144 at V8 and VAX003 at V5 and V9. (D and E) Magnitude of V1–V2 IgG3 responses in the RV144 case-control study. (F and G) Cumulative incidence plots for V1–V2 IgG3 responses. ***P < 0.0005; **P < 0.005; *P < 0.05. P values in (A) were calculated with the two-tailed Fisher’s exact test. P values in (B) and (C) were calculated with two-tailed t tests.
Overall, vaccine-induced IgG3 and IgG responses for either the HIV-1 envelope or V1–V2 were not strongly predictive of one another (figs. S2 and S3), indicating that the measurement of the IgG3 response is a unique measurement that cannot be inferred from measuring total IgG. Thus, we determined whether V1–V2–specific IgG3 antibodies correlated with decreased risk of HIV-1 transmission in a blinded follow-up RV144 case-control study. Rolland et al. (4) reported that position 169 in the V2 sequence was a site of immune pressure, and vaccine-elicited V2-directed antibodies were sensitive and/or dependent on this residue (21, 22). We tested whether IgG3 antibodies specific to the V1–V2 sequence that matched the major circulating strains in Thailand, at a known site of immune pressure (gp70 B.CaseA2 V1–V2 169K), would correlate with decreased risk of infection. We modified the V1–V2 fusion protein used in the primary RV144 correlates analysis (2), with a mutation at site 169 (V169K). In a blinded case-control study, we tested plasma drawn 2 weeks after the last vaccination for 205 vaccinees, 41 cases (infected vaccinees), and 40 HIV-1 uninfected participants enrolled in the placebo arm of the trial by a validated binding multiplex assay (2, 18) for binding to different V1–V2 antigens (Fig. 3, D to G, and fig. S4). Both the response rate (Table 3A) and magnitude (Table 3B) to the indicated V1–V2 antigens resulted in a lowered odds ratio (OR), trending toward decreased risk of infection (table S1). The analysis was performed with both univariate models (analysis of one immune variable at a time) and IgA-adjusted models, which include the IgA score variable (an important independent predictor of infection risk), in addition to the immune variable of interest. In the IgA-adjusted models, the magnitude of the IgG3 response to three different V1–V2 antigens significantly correlated with decreased risk of infection (P < 0.05, logistic regression), suggesting that some of the response could target multiple V1–V2 sequences. Remarkably, IgG3 antibodies to gp70 B.CaseA2 V1–V2 169K significantly correlated with decreased risk of infection in both the univariate and IgA-adjusted models (P = 0.006 and 0.003, respectively, logistic regression) (Table 3A and Fig. 3, D to G).
Table 3
Plasma HIV-1–specific IgG3 responses from 205 vaccinees, 41 infected vaccinees (cases), and 40 placebos at 2 weeks after the last vaccination were analyzed with clades A, B, and C V1–V2 antigens for risk of infection. (A) Case-control results from the categorical analysis (responder/nonresponder) for V1–V2 antigens. (B) Case-control results from the quantitative analysis of the magnitude of the response for V1–V2 antigens. P values <0.05 are in bold.
A. Correlation of IgG3 response rate with risk of infection in the RV144 case-control study
| |||||
---|---|---|---|---|---|
Sequence description | HIV-1 Env scaffold | Univariate
| IgA-adjusted
| ||
OR | P | OR | P | ||
V1–V2 from RV144 vaccine boost (A244 gp120) | AE.A244 V1–V2 tags | 0.494 | 0.162 | 0.391 | 0.075 |
V1–V2 from clade C | C.1086 V1–V2 tags | 0.564 | 0.142 | 0.511 | 0.093 |
V1–V2 from Haynes et al. (2) | gp70 B.CaseA2 V1–V2 | 0.522 | 0.131 | 0.419 | 0.054 |
V1–V2 with mutation (169K) | gp70 B.CaseA2 V1–V2 169K | 0.354 | 0.006 | 0.317 | 0.003 |
B. Correlation of IgG3 magnitude with risk of infection in the RV144 case-control study
| |||||
---|---|---|---|---|---|
Type of Env protein/sequence information | HIV-1 Env scaffold | Univariate
| IgA-adjusted
| ||
OR | P | OR | P | ||
V1–V2 from RV144 vaccine boost (A244 gp120) | AE.A244 V1–V2 tags | 0.791 | 0.185 | 0.682 | 0.045 |
V1–V2 from Haynes et al. (2) | gp70 V1–V2 CaseA2 | 0.749 | 0.088 | 0.689 | 0.034 |
V1–V2 from clade C gp120 | gp70.C. V1–V2 | 0.753 | 0.104 | 0.703 | 0.049 |
We measured the durability of Env and V1–V2 IgG and IgG3 antibody responses in a longitudinal follow-up study for RV144 from week 26 to week 182 after vaccination (Table 4). A previous report showed that V2 peptide-specific antibodies declined after RV144 vaccination (23). We found that the IgG response rate to the vaccine strain A244 gp120 monomer declined from 100 to 29% between weeks 26 and 182, whereas IgG responses to the V1–V2 antigens declined from 97 to 0 to 11% (depending on the antigen) (Table 4A). There was a trend toward a greater decline in total IgG antibody responses to the V1–V2 antigens compared to the envelope protein gp120. This may reflect the higher overall responses to the gp120 protein compared to the V1–V2 region, allowing these responses to remain above the limit of detection longer than the V1–V2 responses. The IgG3 envelope response rate declined from 79 to 0% (weeks 26 to 182) (Table 4B). Notably, IgG3 responses showed a larger decline than the total IgG responses to the vaccine strain gp120 (45% IgG versus 0% IgG3 gp120 at week 52), which is also consistent with the shorter apparent half-life of each IgG3 response. The RV144-induced Env V1–V2 IgG3 apparent response half-life is 1.6- to 3.7-fold shorter than IgG (Table 4C). Moreover, envelope IgG4 responses either remained the same or were elevated at the later visits (table S2), in contrast to the decline in the IgG3 responses. IgG3 responses to V1–V2 antigens also declined from 50 to 84% to 3% between weeks 26 and 52. This is in contrast to IgG responses to V1–V2 that declined from 97 to 11% to 50% between weeks 26 and 52. Thus, in both vaccine studies, we identified differential kinetics in the IgG subclass responses to vaccination over time, with a vaccine-induced IgG3 response that waned early after vaccination.
Table 4
RV144-induced Env V1–V2 IgG3 apparent half-life is 1.6- to 3.7-fold shorter than IgG.
Longitudinal HIV-1 Env and V1–V2 IgG (A) and IgG3 (B) binding antibody response were determined longitudinally from the peak antibody response at weeks 26 to 182 (seven time points). The percent responders for each antigen at each time point after vaccination are shown. (C) Apparent half-life was calculated with an exponential decay model from the binding (MFI) of IgG and IgG3 to specific antigens at a series of sequential time points after vaccination.
A
| ||||||||
---|---|---|---|---|---|---|---|---|
IgG response rates
| Weeks after vaccination
| |||||||
Type of Env protein | HIV-1 Env protein | 26 | 52 | 78 | 104 | 130 | 156 | 182 |
RV144 Env boost (monomeric form) | A244 gp120 | 100 | 79 | 45 | 34 | 34 | 34 | 29 |
V1–V2 from clade C | C.1086 V1–V2 tags | 97 | 50 | 24 | 21 | 13 | 8 | 11 |
V1–V2 from Haynes et al. (2) | gp70 B.CaseA2 V1–V2 | 97 | 11 | 3 | 3 | 3 | 0 | 0 |
V1–V2 with mutation (169K) | gp70 B.CaseA2 V1–V2 169K | 97 | 13 | 3 | 3 | 3 | 3 | 3 |
B
| ||||||||
---|---|---|---|---|---|---|---|---|
IgG3 response rates
| Weeks after vaccination
| |||||||
Type of Env protein | HIV-1 Env protein | 26 | 52 | 78 | 104 | 130 | 156 | 182 |
RV144 Env boost (monomeric form) | A244 gp120 | 79 | 0 | 0 | 0 | 0 | 0 | 0 |
V1–V2 from clade C | C.1086 V1–V2 tags | 84 | 3 | 5 | 3 | 3 | 3 | 3 |
V1–V2 from Haynes et al. (2) | gp70 B.CaseA2 V1–V2 | 37 | 3 | 3 | 3 | 3 | 3 | 3 |
V1–V2 with mutation (169K) | gp70 B.CaseA2 V1–V2 169K | 50 | 3 | 3 | 3 | 3 | 3 | 3 |
C. Apparent half-life of Env-specific IgG and IgG3 in RV144
| ||||
---|---|---|---|---|
Type of Env protein/sequence information | HIV-1 Env protein | Apparent half-life (weeks)
| Fold difference | |
IgG | IgG3 | |||
RV144 vaccine boost (monomer) | A244 gp120 | 18.0 | 8.4 | 2.1 |
V1–V2 from clade C | C.1086 V1–V2 tags | 23.7 | 10.2 | 2.3 |
V1–V2 from Haynes et al. (2) | gp70 B.CaseA2 V1–V2 | 11.7 | 7.5 | 1.6 |
V1–V2 with mutation (169K) | gp70 B.CaseA2 V1–V2 169K | 17.6 | 4.7 | 3.7 |
DISCUSSION
Multiple HIV-1 vaccine efficacy studies have been completed, yet only one trial in Thailand resulted in partial efficacy (RV144) (1). Further understanding of the differences in the quality of the immune response induced by different vaccine regimens with divergent outcomes will allow for specific hypotheses to be tested in further vaccine trials. Another trial in Thailand (VAX003) that used a protein component of the RV144 vaccine showed no efficacy (6). We identified that the Env IgG subclass profile was markedly different between the two Thailand efficacy studies, and we further identified that V1–V2 IgG3 correlated with decreased infection risk. Moreover, this IgG3 response waned quickly, corresponding with the observed waning vaccine efficacy in RV144. Thus, these data provide a rationale for further testing in HIV-1 vaccine trials for induction and maintenance of specific Env IgG3 responses, as well as testing in other studies whether the IgG3 response is a correlate of infection risk. Here, we found higher IgG3 Env antibody response rates to the gp70 V1–V2 clade B–CaseA2 Env fusion protein (gp70 B.CaseA2 V1–V2), as well as to ALVAC 92TH023 Env protein, and consensus AE and group M (ConS gp140) Env proteins in RV144 compared to VAX003 vaccinees. These IgG3 response rates at peak immunogenicity were the only humoral responses that were more frequently detected in RV144 than in VAX003; all other antibody responses tested, as well as the previously reported nAb responses, were lower in RV144 as compared to VAX003 (7). In our analyses of antibody binding, we used the envelope proteins that matched the RV144 vaccine immunogen. We previously reported that these antigens contain an N-terminal Env sequence deletion that altered the antigenicity of these proteins compared to other proteins (24). Likely, the differences in the MN gp120 results obtained in the Chung et al. (20) study and our study are due to differences in epitope exposure on the different types of MN proteins (with and without the N-terminal deletion sequence).
In addition to the lower number of protein boosts, the ALVAC prime component of the RV144 vaccine regimen may have stimulated different antibody subclasses and specificities compared to those stimulated in the protein-only vaccine (VAX003), presumably through differential antigen presentation and B cell maturation pathways. The hypothesis that the ALVAC prime drove the preferential induction of particular IgG3 specificities is consistent with the finding that the most significant difference in the IgG3 responses between the two vaccines was identified with 92TH023 envelope (sequence present in the ALVAC prime) but not with MN and A244 envelopes (present in the protein boost of both vaccine regimens). Alternatively, differences in immune status, including activation state, of the two populations participating in the clinical trials (injection drug users for VAX003 versus community-based for RV144) could have also contributed to the observed differences in vaccine-elicited IgG subclass responses. The finding of higher Env IgG4 responses in the protein-only vaccine regimen compared to the vector prime/protein boost regimen is consistent with previous reports that found that MN gp160/gp120 prime boost protein vaccination elicited high levels of Env IgG4 (25), whereas the ALVAC (vcp152) prime with an MN gp120 boost elicited lower Env IgG4 relative to IgG1 and IgG3 responses (26). Repeated protein boosting may induce higher levels of nAbs; however, our study also indicates that the IgG subclass distribution can be skewed away from IgG3 with additional protein boosts. Moreover, there may be a delayed kinetics of induction of IgG4 after vaccination such that the time after initial vaccination may also contribute to the differences in antibody subclasses.
We previously tested IgG3 antibody responses to different HIV-1 envelopes as part of the secondary immune-correlates analyses in RV144 and found that only one IgG3 Env response rate, but not magnitude, to A244 gp120 weakly correlated with decreased risk of infection (P = 0.03) (2). Additional envelope antigens tested here (table S1) also showed lower OR but did not reach statistical significance. Here, we tested the IgG3 response to the V1–V2 sequence, matching the major circulating strains in Thailand and a site of immune pressure, and report that this IgG3 response significantly correlated with a decreased risk of infection. We also show that RV144 induced a breadth of IgG3 V1–V2 responses such that clades AE and C V1–V2 (represented by Envs C.1086 and C.97ZA012 in the analysis) also correlated with reduced transmission risk. Thus, our study also enables a direct comparison of vaccine-induced antibody responses in efficacy trials including HVTN 505 (27) and planned South African trials. The clade C.1086 V1–V2 tags (Env subunit protein) (21) contain the sequences from the subtype C.1086 envelope that has been chosen as the envelope immunogen for planned HIV-1 efficacy studies in South Africa on the basis of the antigenicity of the envelope protein as well as originating from a clade C transmitted founder virus (28). Thus, responses to these specific V1–V2 antigens in this study may be used as a minimum benchmark for antibody response rates and magnitudes in further HIV-1 efficacy trials. The blinded follow-up case-control study, together with the RV144 and VAX003 comparison study, demonstrates that the Env IgG3 responses may distinguish the quality of vaccine-induced humoral responses.
Together, these findings suggest that the recombinant canary pox–based priming immunogen ALVAC-HIV (vCP1521) may have affected the adaptive immune response elicited by AIDSVAX B/E rgp120 subunit formulation through innate signaling or differential stimulation of the CD4+ T cell response, and thereby generated a qualitatively different Env-specific IgG subclass profile. The prime-boost RV144 vaccine efficacy trial regimen could have elicited a biased class switch recombination to IgG3 by affecting T cell cytokine profiles or activated a different population of responder B cells (and marginal zone B cells). Further studies will examine whether CD27−IgG+ B cells that show a preference for IgG3 expression (and that have low somatic mutation) (29) were preferentially elicited in the RV144 prime/boost regimen compared to protein-only regimens. These B cell populations could represent a first wave of memory B cells in response to vaccination that need to be stimulated or redirected to a long-lived memory B cell population (30). The role of effector cells (with different FcR expression) in mediating antiviral activity together with vaccine-elicited HIV-1 Env IgG3 responses is an important area to explore in future HIV-1 vaccine trials. Peripheral blood mononuclear cells (PBMCs) were not stored for VAX003; however, a follow-up study with the VAX003 regimen (RV328) will collect PBMCs, bone marrow aspirates, and mucosal biopsies. Similarly, follow-up studies to RV144 (that is, RV305 and RV306) will collect similar samples, allowing further comparisons between RV144 and VAX003 regimens to understand the induction and ontogeny of HIV-1 envelope–specific IgG3 responses. Vaccine-induced IgG3 responses correlated with ADCC, indicating that this antibody subclass may have effector function. Notably, the association of Env IgG3 with medium/high ADCC was significant in RV144, but not in VAX003, suggesting that the IgG3 responses are, overall, of a different quality in RV144. ADCC-mediating antibodies in RV144 targeted multiple epitopes (V2, V3, conformation C1) that could all contribute to the ADCC responses measured in RV144 plasma (31). It will be important to investigate the relative contribution of different epitope-specific ADCC responses induced by further protective versus nonprotective vaccine regimens (like VAX003).
A limitation of our study on RV144 correlates of risk is that we did not define mechanistic correlates of protection (32). Thus, additional studies are needed to determine whether the antibody responses identified here are a marker of HIV-1 risk or whether they play a mechanistic role in decreased HIV-1 risk. Hence, the role of IgG3 compared to other IgG subclasses in other functional assays such as assays that measure antiviral functions at mucosal sites or virion capture (21, 33) will be important to investigate. Another limitation of our study is that our analyses did not include interrogating the different antibody binding epitopes comprised within the V1–V2 antigens or protein envelopes for the IgG subclass response. Differences in epitope recognition on HIV-1 virions or infected cells may also play a role in the different quality of the IgG3 response between RV144 and VAX003, as well as affecting the functional profile of the antibodies. Finally, whether there is a relationship between the early vaccine effect in RV144 (60% early at 12 months waning to 31.2% later at 42 months) and the initial peak and decline in the vaccine-induced envelope IgG3 response is unknown. The rapid decline in the vaccine-induced IgG3 response in both the RV144 and VAX003 regimens may indicate that this response will be difficult to maintain. Thus, it will be important to test different adjuvants and immunogens to determine whether vaccine-induced Env IgG3 durability can be improved. Together, these data provide a rationale to investigate IgG3 responses as correlates of transmission risk in future HIV-1 vaccine trials and to explore whether this response could be tested as a correlate of protection (32).
MATERIALS AND METHODS
Study design
We tested the hypothesis that the antibody subclass responses would be significantly different between the two HIV-1 vaccine efficacy trials testing different vaccine regimens in different risk populations with different efficacy outcomes (RV144 and VAX003 clinical trials). Antibody subclass responses were evaluated at baseline and peak immunogenicity for both trials and also at 2 weeks after the second protein immunization for VAX003. Statistical analyses of the study results were performed at both the Duke Human Vaccine Institute (DHVI) and Statistical Center for HIV/AIDS Research and Prevention (SCHARP). Given the results from this study, we next tested the hypothesis that V1–V2 IgG3 would correlate with decreased HIV-1 infection risk. We examined IgG3 binding responses in a placebo-controlled, case-control study for RV144. HIV-1 IgG3 binding antibodies at weeks 0 and 26 (2 weeks after the last vaccination) were tested, blinded for infection and vaccination status, on 205 vaccinees, 41 infected vaccinees (cases), and 40 participants enrolled in the placebo arm of the study. Data were sent to SCHARP for statistical analysis and unblinding of placebo and infection status and analyzed for risk of infection as previously described (2). We next hypothesized that Env IgG3 responses waned more rapidly than the overall Env IgG response. To test this hypothesis, we examined longitudinal follow-up samples from the RV144 vaccine study (38 vaccinees and 19 placebos) to measure antibody subclass responses (IgG, IgG3, and IgG4) at weeks 26, 52, 78, 104, 130, 156, and 182. Data were sent to SCHARP for statistical analysis and unblinding of placebo and vaccine status and to the DHVI Biostatistics and Bio-informatics Center for the analysis of apparent half-life.
Clinical trials
Participants in RV144 (1) received vCP1521 (containing HIV-1 gag, env, and protease genes) and gp120 92TH023 (CRF01_AE) linked to transmembrane portion of subtype B gp41 without the gp41 ectodomain (Sanofi Pasteur) at months 0, 1, 3, and 6 with coadministration of AIDSVAX B/E gp120 [MN gp120 and CRF01_AE CM244 (A244)] [Global Solutions for Infectious Diseases (GSID)] (34) at months 3 and 6. Participants in VAX003 solely received intramuscular injections of AIDSVAX B/E at months 0, 1, 6, 12, 18, 24, and 30 (6). All clinical trials were conducted in accordance with the Declaration of Helsinki and local institutional review board requirements. Written informed consent was obtained from all subjects.
Plasma/serum specimens
Antibody subclasses were measured at baseline and at 2 weeks after the fourth immunization for RV144 (up to 88 vaccinees and 30 placebos) in a pilot RV144 set and in the RV144 immune correlates set (205 vaccinees, 41 infected cases, and 40 placebos) as previously described (2) (fig. S5A), and at baseline and at 2 weeks after the second and fourth immunization for VAX003 (up to 121 vaccinees and 30 placebos) (fig. S5B). All placebo recipients were negative for HIV-1–specific IgG subclass responses.
HIV-1–specific binding antibody assay
Plasma HIV-1–specific antibodies to HIV-1 gp120/gp140 proteins and V1–V2 scaffolds were measured by a custom HIV-1 binding antibody multiplex assay as previously described (2, 18, 35). All assays were run under Good Clinical Laboratory Practice–compliant conditions, including tracking of positive controls by Levy-Jennings charts with 21CFR Part 11 compliant software. Positive controls included a HIVIG titration, CH58 mAb IgG titration, and IgG1 to IgG4 standard curve titrations. Negative controls included in every assay were blank beads, HIV-1–negative sera, and baseline (prevaccination) samples. To control for antigen performance, we used the preset criteria that the positive control titer (HIVIG) included on each assay (and for assays with V1–V2 antigens, CH58 mAb) had to be within ±3 SDs of the mean for each antigen (tracked with a Levy-Jennings plot with preset acceptance of titer) (calculated with a four-parameter logistic equation, SigmaPlot, Systat Software). HIV-specific antibody iso-types were detected with mouse anti-human IgG1 (BD Pharmingen), mouse anti-human IgG2 (Southern Biotech), mouse anti-human IgG3 (Calbiochem), or mouse anti-human IgG4 (BD Pharmingen) conjugated to biotin, at 4 μg/ml, followed by washing and incubation with streptavidin-phycoerythrin (PE) (BD Pharmingen). Antibody measurements were acquired on a Bio-Plex instrument (Bio-Rad) with 21CFR Part 11 compliant software, and the readout is in MFI or μg/ml equivalents based on titrating purified IgG1 to IgG4 human myeloma proteins (Sigma) on beads coupled to purified anti-human IgG1 to IgG4 antibodies and detecting with goat anti-human κ (Southern Biotech) conjugated to biotin followed by washing and incubation with streptavidin-PE (BD Pharmingen). Binding antibody μg/ml equivalents were calculated with a 4PL curve analysis. Both μg/ml concentrations and MFI levels were measured and compared for each antibody subclass between VAX003 and RV144 with similar results. The preset assay criteria for sample reporting were as follows: coefficients of variation per duplicate values for each sample were 15% and >100 beads counted per sample. Additionally, responders for each IgG subclass were calculated as MFI ≥100 and threefold over baseline. For comparison across assay runs, MFI values are shown as the most robust comparison. To confirm the findings on the comparison study of IgG3 binding responses between RV144 and VAX003, samples were repeated on different days by different operators, and samples from both studies were examined side by side in the same assay run.
Production of recombinant HIV-1 proteins
RV144 vaccine immunogen proteins A244 gp120, GNE8 gp120, and B.MN gp120 (MN gp120) were produced, purified, and supplied by GSID. Recombinant HIV-1 gp120 proteins including the subtype AE A244gDneg, A244 gp120 delta11 293F (referred to as A244 gp120 monomer in the text), 92TH023 gp120, and B.MN gp120 were expressed in 293F cells by transfection and purified with lectin-affinity columns (16) followed by size exclusion chromatography on a Superdex 200 FPLC (GE Healthcare) to homogeneity for monomeric gp120. Recombinant Envs including consensus Envs (group M CON-S gp140CFI and AE. con gp140CF) were produced in 293T cells by recombinant vaccinia viruses (16). gp70 B.CaseA2 V1–V2, gp70 A.92RW020V1–V2 (gp70 V1–V2 A), gp70 C.97ZA012 V1–V2 (gp70.C. V1–V2), and gp70 B.CaseA2 V1–V2 169K were made as fusion proteins with murine leukemia virus (MLV) gp70 and produced as described (21, 36). MLV gp70 carrier protein without V1–V2 sequence was produced in 293F cells, purified by nickel columns, and used as negative control for gp70 scaffold proteins. Sequences of HIV-1 Envs, AE.703357 and AE.427299, were derived from RV144 subjects in the placebo arm and represent infection Envs with wild-type V2 sequences that do not match the AE vaccine strains A244 and 92TH023. AE.A244 V1–V2 tags and C.1086 V1–V2 tags were designed with Ig leader (METDTLLLWVLLLWVPGSTGD) serving as a mature protein cleavage and secretion signal at the N terminus and with the C-terminal avi-tag followed by His6-tag for purification, produced in 293F cells by transfection, and purified by nickel columns (37).
Plasma IgG purification
IgG was isolated from plasma with the above Protein G HP MultiTrap 96-well plates (GE Healthcare Inc.) as previously described (2, 38).
Surface plasmon resonance
SPR binding assays were performed on a Biacore 3000 (Biacore Inc.) instrument at 25°C, and data analyses were performed with the BIAevaluation 4.1 software (Biacore) as previously described (2, 39).
nAbs and ADCC
Neutralizing assays and ADCC were conducted as previously described (7, 31, 40, 41). ADCC assays were performed with both an infected cell assay (T cell lines derived from NKR.CEM-CCR5 cells were infected with HIV-1 AE.92TH023 as the target) and a gp120-coated assay (CM243 gp120-coated target cells with granzyme B as the readout, as previously described).
Statistical analyses
The levels of IgG subclasses were compared between the RV144 and VAX003 trials with two-tailed t tests (GraphPad Prism). For IgG subclasses, response rates for each antigen were compared with two-tailed Fisher’s exact test. To correct for family-wise α inflation, P values were adjusted with the Benjamini-Hochberg correction (42). IgG antibody avidity was compared between the RV144 and VAX003 trials with the Wilcoxon rank-based test. The case-control analyses were implemented with the osDesign package in R, which performed maximum likelihood estimation in a logistic regression model while accommodating the two-phase sampling design. Gender and baseline behavioral risk were included in every regression model. The IgG3 continuous readouts were blank-subtracted, log-transformed, not thresholded by the positivity call criterion, and not baseline-adjusted. The IgG3 discrete readouts were dichotomized according to the positivity call. The IgG3 positivity call criterion was MFI >100, threefold above baseline for both blank-subtracted and blank-unsubtracted readouts, and blank MFI <5000. The univariate models included only one immune variable at a time. Besides the univariate model, we also use IgA-adjusted models, which include the primary IgA score variable assessed previously (1) in addition to the immune variable of interest. We previously demonstrated that the IgA score is an important predictor of the case status (1). Adjusting for such variables tends to increase the statistical power for the inference of interest, and gives the OR for IgG3 an interpretation that controls for the level of the primary IgA score. We report the P values for the individual hypothesis tests in the case-control analyses without multiplicity adjustment, because the different variants of V1–V2 antigens are all closely related to the original V1–V2 antigen previously studied (2). Details of the case-control sampling designs can be found in (2). Apparent antibody half-life measurements were determined by an exponential decay model as previously described (2, 18, 35, 43).
Supplementary Material
Supp Materials
Fig. S1. Significant correlation of Env IgG3 and V1–V2 with ADCC in RV144, but not VAX003.
Fig. S2. Lack of direct correlations for all HIV-1 Env IgG1 and IgG3 antibody responses.
Fig. S3. Weak association between V1–V2 IgG and V1–V2 IgG3 measurements.
Fig. S4. Cumulative incidence plots of IgG3 to V1–V2 antigens in an RV144 case-control analysis.
Fig. S5. Vaccination scheme for RV144 (top) and VAX003 (bottom) vaccine regimens.
Table S1. ORs for HIV-1 infection risk in univariate analyses of IgG3 measurements.
Table S2. HIV-1 Env IgG4 response rates in RV144 vaccinees do not decline between peak immunogenicity (week 26) and week 52.
Acknowledgments
The views expressed in this article are those of the authors and should not be construed as official or as representing the views of the Department of Health and Human Services, the National Institute of Allergy and Infectious Diseases (NIAID), the Department of Defense, or the Department of the Army. Trade names are used for identification purposes only and do not imply endorsement. The funders had no role in study design, data collection and analysis, decision to publish, or preparation of the manuscript. We thank the participants and clinical staff of the VAX003 and RV144 vaccine trials. We thank M. Robb, C. Andrews, K. A. Soderberg, K. Greene, and H. Gao for clinical and project management oversight. We thank M. Donathan, J. T. Lucas, V. Ashley, R. Howington, A. Deal, R. G. Overman, A. Lan, M. Archibald, S. Stewart, and F. Jaeger for technical assistance and M. Sarzotti-Kelsoe for quality assurance oversight.
Funding: This work was supported by the Bill and Melinda Gates Foundation grants, Collaboration for AIDS Vaccine Discovery (CAVIMC OPP1032144, 38619) (N.L.Y., W.T.W., S.M.A., G.F., D.C.M., and G.D.T.), OPP 1033098 (N.L.Y., W.T.W., G.F., B.F.H., and G.D.T.), and NIH/NIAID/Division of Acquired Immunodeficiency Syndrome: Center for HIV/AIDS Vaccine Immunology Grant (U01 AI067854) (N.L.Y., H.-X.L., N.A.V., B.F.H., and G.D.T.). In addition, funding was provided by HIV-1 Vaccine Trials Network (HVTN) (5U01 AI46725-05) (N.L.Y., W.T.W., K.E.S., and G.D.T.) and the Duke University Center for AIDS Research (CFAR) Grant (P30 AI 64518) (G.D.T.). The work was also supported in part by the intramural program of NIH (Vaccine Research Center, NIAID) (G.J.N. and Z.-y.Y.) and an Interagency Agreement (Y1-AI-2642-12 and Y1-AI-2642-16) between the U.S. Army Medical Research and Material Command and the NIAID and by a cooperative agreement (W81XWH-11-2-0174 and W81XWH-07-2-0067) between the Henry M. Jackson Foundation for the Advancement of Military Medicine Inc. and the U.S. Department of Defense (M.D.A., D.T.E., R.J.O., N.L.M., J.H.K., B.F.H., and G.D.T.) and including contract #694251 (Y.F., A.d.C., and P.B.G.).
Footnotes
Author contributions: N.L.Y., P.B.G., S.Z.-P., D.C.M., B.F.H., and G.D.T. designed the study. N.L.Y., Y.F., A.d.C., N.A.V., W.T.W., S.M.A., G.F., K.E.S., M.D.A., B.F.H., D.T.E., P.B.G., and G.D.T. designed the experiments and analyzed the data. Y.F., A.d.C., N.A.V., and P.B.G. were responsible for the statistical analyses. N.L.Y. and W.T.W. performed binding assays. S.M.A. designed avidity assays. M.D.A., D.T.E., and G.F. designed and performed ADCC experiments. H.-X.L., A.P., Z.y.-Y., G.J.N., and B.F.H. designed and produced specialized reagents (HIV-1 envelope and V1–V2 antigens). P.W.B., D.F., F.S., C.L., and J.T. designed and carried out the VAX003 clinical trial and provided specimens. R.J.O., S.N., S.R.-N., J.K., P.P., N.L.M., and J.H.K. designed and/or carried out the RV144 clinical trial and provided specimens. N.L.Y., Y.F., G.F., R.J.O., J.T., S.Z.-P., P.B.G., N.L.M., J.H.K., D.C.M., B.F.H., and G.D.T. wrote the paper.
Competing interests: J.T. was employed by Sanofi when these studies were performed. G.J.N. and Z.-y.Y. have subsequently taken positions at Sanofi. M.D.A. is the founder of Immunathon Inc. A.P. consults for Avatar Biomedical. A.P. is listed as inventor on U.S. Patent 6,815,201 “HIV-1 gp120 V1/V2 domain epitopes capable of generating neutralizing antibodies.” A provisional patent application Ser. No. 61/884,038 “Correlates of protection against HIV-1 infection” was filed on 28 September 2013. The inventors listed on this patent application are B.F.H., G.D.T., H.-X.L., and S.Z.-P.
References
Full text links
Read article at publisher's site: https://doi.org/10.1126/scitranslmed.3007730
Read article for free, from open access legal sources, via Unpaywall:
https://europepmc.org/articles/pmc4116665?pdf=render
Citations & impact
Impact metrics
Article citations
Potent broadly neutralizing antibodies mediate efficient antibody-dependent phagocytosis of HIV-infected cells.
PLoS Pathog, 20(10):e1012665, 28 Oct 2024
Cited by: 0 articles | PMID: 39466835 | PMCID: PMC11542898
Non-HIV Vaccine-Induced Immune Responses as Potential Baseline Immunogenicity Predictors of ALVAC-HIV and AIDSVAX B/E-Induced Immune Responses.
Viruses, 16(9):1365, 27 Aug 2024
Cited by: 0 articles | PMID: 39339842 | PMCID: PMC11437453
Safety and immunogenicity after a 30-month boost of a subtype C ALVAC-HIV (vCP2438) vaccine prime plus bivalent subtype C gp120/MF59 vaccine boost (HVTN 100): A phase 1-2 randomized double-blind placebo-controlled trial.
PLOS Glob Public Health, 4(9):e0003319, 20 Sep 2024
Cited by: 0 articles | PMID: 39302924 | PMCID: PMC11414935
Use of 3M-052-AF with Alum adjuvant in HIV trimer vaccine induces human autologous neutralizing antibodies.
J Exp Med, 221(10):e20240604, 05 Sep 2024
Cited by: 1 article | PMID: 39235529
Immune correlates analysis of the Imbokodo (HVTN 705/HPX2008) efficacy trial of a mosaic HIV-1 vaccine regimen evaluated in Southern African people assigned female sex at birth: a two-phase case-control study.
EBioMedicine, 108:105320, 04 Sep 2024
Cited by: 0 articles | PMID: 39236556 | PMCID: PMC11404224
Go to all (307) article citations
Data
Data behind the article
This data has been text mined from the article, or deposited into data resources.
BioStudies: supplemental material and supporting data
Similar Articles
To arrive at the top five similar articles we use a word-weighted algorithm to compare words from the Title and Abstract of each citation.
Comparison of Antibody Responses Induced by RV144, VAX003, and VAX004 Vaccination Regimens.
AIDS Res Hum Retroviruses, 33(5):410-423, 30 Jan 2017
Cited by: 31 articles | PMID: 28006952 | PMCID: PMC5439458
Polyfunctional Fc-effector profiles mediated by IgG subclass selection distinguish RV144 and VAX003 vaccines.
Sci Transl Med, 6(228):228ra38, 01 Mar 2014
Cited by: 254 articles | PMID: 24648341
RV144 HIV-1 vaccination impacts post-infection antibody responses.
PLoS Pathog, 16(12):e1009101, 08 Dec 2020
Cited by: 16 articles | PMID: 33290394 | PMCID: PMC7748270
Prospects for a globally effective HIV-1 vaccine.
Vaccine, 33 Suppl 4:D4-12, 20 Jun 2015
Cited by: 24 articles | PMID: 26100921
Review
Funding
Funders who supported this work.
Intramural NIH HHS
NIAID NIH HHS (13)
Grant ID: P30 AI064518
Grant ID: Y1-AI-2642-12
Grant ID: Y1-AI-2642-16
Grant ID: U01 AI067854
Grant ID: P30 AI 64518
Grant ID: R01 AI098485
Grant ID: U01 AI046725
Grant ID: 5U01 AI46725-05
Grant ID: UM1 AI068614
Grant ID: P01 AI100151
Grant ID: UM1 AI068635
Grant ID: R01 AI102718
Grant ID: U19 AI067854