Abstract
Free full text

IL-12 Family Cytokines: Immunological Playmakers
Abstract
The interleukin-12 (IL-12) family is unique in comprising the only heterodimeric cytokines, which includes IL-12, IL-23, IL-27 and IL-35. This endows these cytokines with a unique set of connections and functional interactions not shared within other cytokine families. Despite sharing many structural features and molecular partners, they mediate surprisingly diverse functional effects. Here we discuss the unique and unusual structural and functional characteristics of this cytokine family. We outline how cells might interpret seemingly similar cytokine signals to give rise to the diverse functional outcomes which characterize this cytokine family. We will also discuss the therapeutic implications of this complexity.
Within the vast array of bioactive cytokines, the interleukin-12 (IL-12) family is unique. They are the only heterodimeric cytokine family and this endows them with several unique and distinctive features. It also presents a number of molecular and functional realities that provide unprecedented opportunities for positive and negative feedback control, some of which may remain unrealized. Chain pairing promiscuity is a common feature of this heterodimeric cytokine family which currently includes IL-12, IL-23, IL-27 and IL-35 (Box 1)1,2. Despite many structural similarities in the cytokines, their receptors and downstream signaling components, they possess vastly contrasting biological activities which belie there common features. IL-12 and IL-23 are predominantly proinflammatory/prostimulatory cytokines which contribute key roles in the development of Th1 and Th17 cells, respectively3–5. Although IL-27 was initially thought to be a proinflammatory cytokine with some recent observations supporting this view6, the majority view is that IL-27 is an immunoregulatory cytokine7–9. IL-35, the newest member of this family, is a potent inhibitory cytokine produced by natural, thymus-derived regulatory T cell (nTreg) populations1,10. This establishes a functionally balanced dichotomy within this family with IL-12 and IL-23 being positive regulatory while IL-27 and IL-35 are negative regulators (Fig. 1).
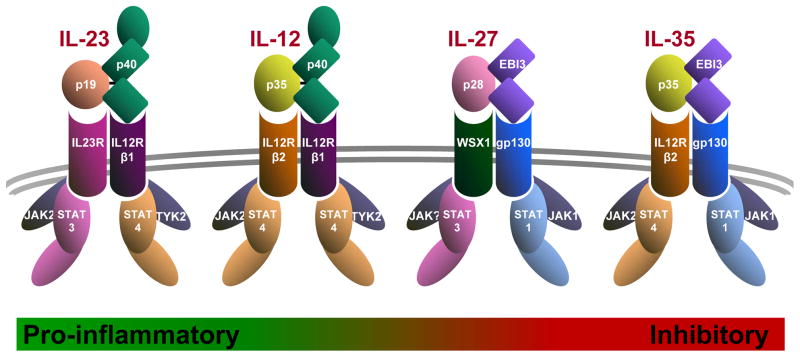
Diagram shows a schematic of the IL12 family cytokines, their receptors and JAK-STAT signaling partners. The Greed-Red bar along the bottom depicts their functional spectrum from most pro-inflammatory (IL-23) to most inhibitory (IL-35).
Here we discuss the implications of the unique structural and functional features of the IL-12 cytokine family and how this leads to a greater level of control and crosstalk than is typically observed in other cytokine families. We will also discuss what remains to be determined and speculate on additional layers of complexity that may exist, and assess how these features may complicate the therapeutic targeting and utilization of this fascinating cytokine family. We will primarily focus on the molecular aspects of these cytokines and their effect on T cell differentiation, acknowledging that they also affect other cell populations and impact a wide variety of disease conditions which have been extensively covered in other reviews (Box 2)1,4,11–16.
Variations on a Theme: Promiscuity in Cytokine and Receptor Pairing
Chain sharing is a key feature of the IL-12 cytokine and cytokine receptor family (Box 1; Fig. 1)1,2. Both cytokine β chains are components of two cytokines, while at least one α chain (p35) is also a constituent of two cytokines (IL-12 / IL-35). The rationale for this propensity is unclear. It could just be expedient to create multiple biologically diverse moieties from the same building blocks. Alternatively, it could help maintain immune balance such that deletion or disruption of a single protein or gene removes positive and negative components from the immune repertoire. While this may seem a sound argument in the case of p35 or IL12Rβ2, components of IL-12, IL-35 and their receptors, this argument breaks down when one considers the consequences of losing p40, Ebi3 or IL12Rβ1, which would preferentially impact one side of this immune equation (Fig. 1).
Given this propensity for chain sharing, one also wonders if other combinations, such as p40/p28 and Ebi3/p19, can pair and perform physiologically relevant functions or whether the IL-12 family α/β chains can pair with other relatives within the IL-6 cytokine superfamily. Indeed, a recent study has suggested that p28 can pair with cytokine-like factor 1 (CLF) to generate a cytokine that regulates NK and T cell activity via IL6Rα 17. A complete picture of all the physiologically relevant interactions could provide the important insight required to complete this immunological jigsaw.
The existence of a heterodimeric cytokine family that shares components may also provide a unique mechanism to control cytokine secretion and stability. Interestingly, the pairing efficiency and the capacity of the individual components to be secreted alone or in combination with other cytokines are vastly different. For instance, IL-12 and IL-23 are disulphide-linked heterodimers and are secreted efficiently, while IL-27 and IL-35 lack this disulphide, pair poorly and thus are secreted in vastly reduced amounts (especially IL-35)2,18–21. This raises the possibility that in cells that express multiple IL-12 cytokine family chains, such as activated DC, macrophages or B cells, they may compete for pairing and secretion. Given the apparent stability of IL-12 and IL-23, they may dominate over IL-27 or IL-35. Thus, more studies are warranted to determine if this competition dynamic does occur and if so whether it has a physiologic impact. It is also curious that the two positive regulatory cytokines (IL-12/IL-23) are disulphide linked while the two negative regulatory cytokines (IL-27/IL-35) are not. Whether this is coincidental or establishes a valid structure-function link remains to be determined.
In additional to their canonical function as heterodimers, some of the IL-12 family components can function autonomously as monomers or homodimers. For instance p28 alone (also referred to as IL-30), in the absence of its normal partner Ebi3, has been shown to bind to but not signal via gp130, thereby antagonizing IL-6 and IL-27 receptor signaling, limiting IL-17 and IL-10 production, respectively, and inhibiting germinal center formation and antibody production22. In contrast, there is no clear evidence suggesting that p19 or p35 can be secreted without a partner. Homodimers of p40 have been shown to antagonize IL-12-driven responses by competing for its binding to IL12Rβ1 while retaining the capacity to activate DCs23–26. While Ebi3 can be secreted, the extent to which this occurs and its physiological impact are unknown. Taken together, there are hints that IL-12 family monomers or homodimers could impact the function of their heterodimeric siblings, and/or immune function in general, although more analysis is clearly required.
The chain sharing theme also extends to receptor usage with several cytokines utilizing the same receptor chains (Box 1; Fig. 1)1,2,27. For instance, IL12Rβ1 is a component of the IL-12 and IL-23 receptors, IL12Rβ2 is used by IL-12 and IL-35, and gp130 is bound by IL-27 and IL-35. As these receptors chains are expressed as monomers and dimerize following cytokine ligation, it remains unclear whether this receptor sharing creates competition for binding when cells are exposed to multiple IL-12 family cytokines. Given that competition of receptor binding is the paradigm under which p28 operates22, it is possible that this may also occur with the heterodimers thereby providing an additional level of immune regulation. For instance, could IL-27 and IL-35 compete for gp130, could IL-12 and IL-23 compete for IL12Rβ1, or could IL-12 and IL-35 compete for IL12Rβ2? Indeed, could p28/IL-30 inhibit IL-35 binding given that it also utilizes gp130?22,28
An additional layer of complexity has recently been revealed by the observation that IL-35 can also ligate and signal via gp130-gp130 and IL12Rβ2-IL12Rβ2 homodimers28. While this appears to be a unique feature of IL-35 not shared by other members of the IL-12 cytokines family, it is not without precedent as gp130 can also signal as a homodimer following ligation with IL-6:sIL6Rα complexes or viral IL-629,30. How this impacts the function of IL-35 compared with its other IL-12 family siblings remains to be determined.
In summation, an unprecedented level of internal regulation may occur within the IL-12 family due to the extensive known, and potential, cytokine and receptor chain sharing and interaction. It is possible that the full complexity of this network remains to be fully realized.
Immunological Playmakers: IL-12 Family Cytokines Shape Immune Responses
In many respects, the IL-12 cytokine family epitomizes the central tenet of immunoregulation. IL-12 and IL-23 are pro-inflammatory/pro-stimulatory cytokines, while IL-27 and IL-35 are inhibitory cytokines (Box 2, Fig. 1)1,3–5,7–10. This raises the possibility that there is an unappreciated spectrum of immune balance that is established by, and within, the IL-12 cytokine family. This is impacted by multiple positive and negative feedback loops and interactions that modulate many aspects of immune function (Fig. 2)1,3–5,7–10. In addition to modulating the behavior of multiple T cell populations, the IL-12 cytokine family has a substantial impact on the direction of immune responses and a wide variety of diseases by also controlling the development of many contrasting T cell populations. Many of the immunoregulatory properties of this cytokine family are due to their effects on T cell differentiation and function. Whereas IL-12 and IL-23 can induce or promote generation of proinflammatory Th1 and Th17 cells, IL-27 and IL-35 can induce regulatory Tr1 cells and iTr35 cells, respectively. In addition, these cytokines can cross-regulate the development of other T cell subsets directly or indirectly through the induction of regulatory T cell populations (Tr1 and iTr35). The most profound example is that IL-27 suppresses development of proinflammatory Th17 cells by inhibiting key transcription factors in Th17 differentiation and at the same time induces the development of Tr1 cells that suppress immune responses by multiple, distinct mechanisms (Fig. 2). In this regard one might argue that the IL-12 cytokine family has a greater impact in shaping immune responses than any other cytokine family.
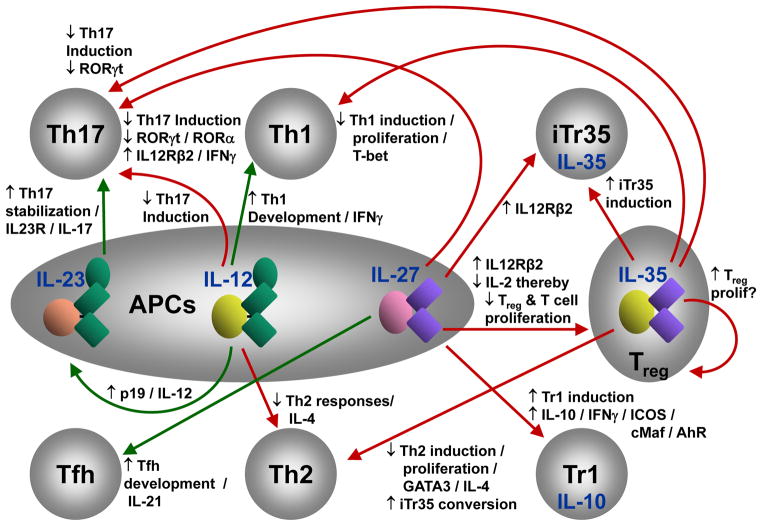
Depicts the myriad of functional connections and effects mediated by members of the IL-12 cytokine family. Green arrows depict actions that result in pro-inflammatory/pro-stimulatory effects. Red arrows depict actions that result in inhibitory effects. Black arrows depict whether a particular event is up- (↓) or down- (↑) regulated.
In deciphering the multiple layers of interaction and influence, it is helpful to examine the impact of the IL-12 cytokine family on differentiation of specific T cell subsets. Several IL-12 family cytokines impact Th1 and Th17 development. The capacity of IL-12 to drive Th1 development is well known, while Th17 development is facilitated by IL-23. As one would expect, this is governed to a large extent by regulated receptor expression. Naïve T cells express IL12Rβ1 and gp130 but not IL12Rβ2, IL23R and WSX131–33. While TCR signaling with co-stimulation via CD28 is sufficient to induce IL12Rβ2, expression is enhanced by IL-27, as well as IL-2, tumor-necrosis factor α (TNFα) and IFNγ. In contrast, TCR signaling is not sufficient to induce IL23R expression. Cytokines that induce STAT3 activation are the most potent drivers of IL23R expression. STAT3 plus RORγt cooperate to transactivate IL-23 and IL23R thereby facilitating a positive feed-forward loop which enhances IL23R, IL-17 and IL-22 expression, and stabilizes the Th17 phenotype. In addition, IL-12 and IL-27 impact Th17 development by inhibiting key transcription factors (RORγt, RORα) that induce Th17 differentiation. In addition, IL-12 and IL-27 can act on differentiating Th17 cells by regulating expression of receptors for other IL-12 family members and making them responsive to inhibitory cytokines and thus inducing IFNγ from developing Th17 cells. Similarly, IL-12 can also enhance APC production of p19, and component of IL-23, thereby limiting Th1 and favoring Th17 development34.
IL-27 can limit Th development in general by blocking IL-2 production which is required for early T cell proliferation35,36. However, once IL-2 production is initiated, the influence of IL-27 can be limited by the IL-2-driven downregulation of WSX137,38. IL-27 was initially thought to be a prostimulatory cytokine by virtue of its capacity to enhance the production of IFNγ in the presence of low concentrations of IL- 1239. However the function of IFNγ, or any cytokine for that matter, can be greatly influenced by co-secreted cytokines. IL-27 is also a potent inducer of IL-10 which in combination with IFNγ represses Th17 cells and promotes Tr1 cells40–43. This is also facilitated by the IL-27-mediated induction of STAT1 which blocks RORγt expression44 and induction of transcription factors cMaf and AhR, growth factor IL-21 and costimulatory molecule ICOS, which coordinately act to induce Tr1 differentiation43,45. IL-27 induces both IFNγ and IL-10 in developing Tr1 cells, and both IFNγ and IL-10 are utilized in the regulation of other effector T cell subsets. Whereas IL-10 was crucial for suppressing immune responses at multiple levels, IFNγ produced by Tr1 cells was shown to suppress the development of Th17 cells46, therefore both of these cytokines produced by Tr1 cells are utilized for immunoregulation. IL-27 has been shown to suppress Th17 cells and induce Tr1 cells in human T cells as well, by a AhR/cMaf dependent mechanism47.
IL-35 can block the development of Th1 and Th17 cells by limiting early T cell proliferation and thus expression of key differentiating transcription factors and cytokines required for their differentiation (Chaturvedi & D.A.A.V., unpublished observations). IL-35 is unusual in having three receptors (IL12Rβ2:gp130; IL12Rβ2:IL12Rβ2; gp130:gp130), which is likely to substantially expand its sphere of influence28. While IL12Rβ2 is expressed mainly by activated T cells and NK cells, gp130 is fairly ubiquitously expressed48. Consequently, naïve T cells can be suppressed by IL-3549–51. IL-2 or IL-27 pretreatment upregulates IL12Rβ2 and thus increases T cell sensitivity to IL-35-mediated suppression28. Given the suppressive phenotype of IL-27 one wonders whether some of this activity is mediated by increased sensitivity to IL-35.
Although IL-35 can inhibit Th1 proliferation, it has no effect on Th17 proliferation perhaps due to limited receptor expression. This may provide further insight into the resistance of Th17 cells to nTreg- mediated suppression10. Interestingly, it has been suggested that IL-23 may also contribute to Th17 resistance to nTreg-mediated suppression via γδ T cells, although the mechanism remains unclear52. Although IL-35 can inhibit Th1 proliferation, they are resistant to iTr35 conversion due to the potent inhibition of Ebi3 and Il12a transcription by IFNγ (Th1-produced or added exogenously; V.C. & D.A.A.V., unpublished observations). Given the IL-12 can induce IFNγ, it is possible IL-12 may also limit iTr35 conversion indirectly.
IL-12 family cytokines can also impact Th2 cells. Whereas IL-12 will inhibit IL-4 production and antagonize Th2 responses11, IL-35 blocks Th2 development by repressing GATA3 and IL-4 expression and limiting Th2 proliferation (V.C. & D.A.A.V., unpublished observations). IL-35 can also mediate iTr35 conversion from Th2 cells, although this can be blocked by IFNγ.
Synonymous with the effects of these cytokines on effector populations, IL-12 family cytokines can also impact regulatory T cell development, homeostasis and function. Tregs are highly dependent on IL- 2 for effective proliferation and homeostasis53,54. IL-12 and IL-27 can limit IL-2 production and consequently have been shown to negatively impact nTregs36, 55–57. Conversely, IL-35 has been suggested to enhance nTreg proliferation58,59. As IL-27 or IL-2 pretreatment upregulates IL12Rβ2, this may also affect their sensitivity to IL-3528. Although IL-23 is not known to affect nTregs, it can limit TGFβ-driven iTreg development52. In contrast but IL-27 and IL-35 can mediate the development of distinct Foxp3− iTreg populations. While IL-27 can induce the development of an IL-10-producing Tr1-like regulatory population40–42,60, IL-35 can induce the development of IL-35-producing iTr3551,61.
Taken together, these observations clearly show that the IL-12 family cytokines act as the immunological playmaker, shaping immune responses by directly inducing the development of T cell subpopulations and altering the function and fate of many cell populations that dictate disease outcome.
Appearances Can Be Deceiving: Similar Signals but Different Outcomes
These structural and functional observations present an important question; how do cells mediate divergent outcomes in response to seemingly similar signals generated by common signaling components? The importance of this question is emphasized by the fact that there are ~40 cytokines that signal through receptors that utilize a selection of only 4 JAKs and 7 STATs27. There is considerable overlap in JAK/STAT usage within the IL-12 cytokine family with all the receptors signaling via JAK2 and three of the four receptors using STAT4 (Box 1; Fig. 1). These cytokines clearly mediate different downstream consequences and thus these signals must be interpreted differently.
Insight into one possible mechanism was recently provided by analysis of IL-35 receptor signaling. IL-35 signals via STAT1 and STAT4 to drive an inhibitory program, while interferon-γ (IFN-γ) and IL-12 in combination induce activation of the same STATs yet drive a prostimulatory program. The key distinguishing feature is the generation of STAT1:STAT4 heterodimers following IL-35 receptor ligation that does not occur following combinatorial treatment with IFN-γ and IL-1228. Furthermore, STAT1:STAT4 heterodimers, but not homodimers, were found to bind to STAT consensus sites within the Ebi3 and Il12a promoters, and mediate IL-35 production. Why STAT1:STAT4 heterodimers are only generated following IL-35 ligation remains to be determined, but could be due to the proximity of STAT1 and STAT4 phosphorylation favoring heterodimer formation. Indeed, the distribution of the IFNGR and the IL12R may not be uniform and is altered following T cell activation, suggesting that these receptors might even be actively segregated as a mechanism to actively prevent STAT1:STAT4 heterodimer formation62–64. Alternatively, IL-35 quantitatively promotes the generation of almost equal levels of pSTAT1 and pSTAT4 promoting heterodimerization, thereby leaving little free pSTATs for homodimerization. Given that the STAT DNA consensus binding motif (TTC-N2-5-GAA) is not considered to vary considerably between the different STAT family members65, it is also not clear why STAT1:STAT4 heterodimers uniquely bind to and transcribe Ebi3 and Il12a promoters while STAT1 and STAT4 homodimers do not. This infers that additional distinguishing differences must exist. This could include unique modifications and/or recruitment of co-activators via the distinctive STAT1:STAT4 carboxy-terminal transactivation domain combination that ultimately modifies binding and/or transcription66,67.
Relatively few studies have suggested that STAT heterodimers can be generated following cytokine signaling. IFNγ can shift from a pro-inflammatory to an anti-inflammatory response by changing the relative expression and activation of STAT3. A dominant STAT3 response favors STAT1:STAT3 heterodimerization, thereby inhibiting homodimerization of STAT1 and the biological activities that are induced in response to STAT1 homodimers68.
These observations raise the interesting hypothesis that STAT homodimers generate predominantly pro-inflammatory responses, while STAT heterodimers drive predominantly anti-inflammatory or inhibitory responses27. Consistent with this hypothesis is the observation that IL-27 induces both pSTAT3 and pSTAT1 mediating T cell inhibition, and loss of STAT1 abrogates the suppressive effects of IL-27 by limiting the development of pSTAT-1:pSTAT-3 heterodimers (Fowler, Peters and Kuchroo, unpublished observations). Clearly, further analysis will be required to test this notion with signaling via the IL27R a particularly interesting candidate. Of course, the IL23R induces the activation of STAT3 and STAT4 so if heterodimers are generated this might undermine this hypothesis give the pro-inflammatory effects of IL-23, but availability of excess free pSTAT3 induced by IL-23 might be responsible for activating effects of IL-23. Alternatively, differential modifications and/or co-activator recruitment may be critical. Regardless, further examination of signaling via all the IL-12 cytokine receptors is likely to be extremely informative, not only for deciphering how these signals shape diverse functions but perhaps also established paradigms that might guide our understanding of cytokine signaling in general.
Future Questions and Challenges
Although substantial advances have been made in our understanding of this fascinating family of cytokines, much remains to be clarified and discovered. Its molecular complexities have also presented significant obstacles which will need to be overcome. For instance, many of the knockout mice lack multiple cytokines greatly complicating data interpretation1. The generation of mutant lines that lack a single, functional cytokine would be invaluable for detailed in vivo analysis with disease models. Likewise, the development of more specific neutralizing mAbs, more sensitive ELISA assay, and better reagents against receptor chains are sorely needed. Lastly, IL-35 has proven extremely difficult to produce limiting detailed functional analysis. In additional to these reagent issues, important questions remain.
First, are there any more members of this family? Given that IL-35 was only discovered five years ago it is possible that other combinations exist that either utilize known chains within this cytokine family or novel members.
Second, what is purpose and benefits (or limitations) of chain sharing? This could be a mechanism to create many cytokines with diverse functions from relatively few building blocks. Alternatively, by incorporating a protein into a positive and negative regulatory cytokine, genetic insults that alter the expression of these proteins may limit their physiological impact and disease consequences. Of course, the true importance for this unique feature may remain elusive.
Third, what is the extent and physiological impact of competition for cytokine or cytokine receptor chain pairing and binding? For instance, if p40 pairs with p35, does this limit IL-23 secretion? If IL-35 binds to IL12Rβ2:gp130, does this limit the availability of the IL-12 and IL-27 receptors?
Fourth, how do seemingly similar signals generate diverse outcomes? The recent observation that part of the IL-35 inhibitory program is mediated by STAT1:STAT4 heterodimers in contrast to the STAT4 homodimers generated by IL-12 receptor signaling28, presents one possible answer but also raises many questions. For instance, do other cytokines with inhibitory properties, such as IL-27, also generate heterodimers, while cytokines with pro-inflammatory properties, such as IL-23, do not? How do STAT heterodimers versus homodimers mediate such diverse functional programs?
Fifth, IL-27 has been shown to have both pro-inflammatory and anti-inflammatory effects. How the same cytokine induces pro-inflammatory and anti-inflammatory effects and under which context or combination needs to be resolved if IL-27 neutralization is going to be tested in a therapeutic setting.
In additional to these and other questions, there are considerable therapeutic implications and challenges. Cytokine neutralization or receptor blockade is inherently more challenging given the chain sharing propensity of this cytokine family. However, encouraging proof-of-principle does exist. For instance, mAbs specific for IL-12 have been generated (anti-p70), raising the possibility that mAbs could be raised to epitopes that are uniquely formed by a particular chain combination or neo epitopes induced allosteric changes induced upon pairing. Interestingly, recent insights into the dimer interface and mode of chain pairing have revealed some clear differences between the members of this cytokine family18,21. This is most evident with IL-35, thereby validating the identification of mAbs that will only neutralize IL-3551,61. Lastly, as discussed above, members of this cytokine family remain very challenging to generate in high quantities limiting they therapeutic potential, inferring that some form of mutagenesis, without loss of biological activity, will probably be required. Despite the challenges that remain, it is likely that many surprising and exciting developments lie ahead.
Acknowledgments
Supported by the National Institutes of Health (AI091977 to D.A.A.V.; NS30843 to V.K.K.), an NCI Comprehensive Cancer Center Support CORE grant (CA21765; D.A.A.V.), and the American Lebanese Syrian Associated Charities (ALSAC; D.A.A.V.).
References
Full text links
Read article at publisher's site: https://doi.org/10.1038/ni.2366
Read article for free, from open access legal sources, via Unpaywall:
https://europepmc.org/articles/pmc4158817?pdf=render
Citations & impact
Impact metrics
Citations of article over time
Alternative metrics
Smart citations by scite.ai
Explore citation contexts and check if this article has been
supported or disputed.
https://scite.ai/reports/10.1038/ni.2366
Article citations
Monocyte-regulated interleukin 12 production drives clearance of Staphylococcus aureus.
PLoS Pathog, 20(10):e1012648, 17 Oct 2024
Cited by: 0 articles | PMID: 39418302 | PMCID: PMC11521269
Ebi3 Binding to IFN-γ and IL-10 Limits Their Function.
J Immunol, 213(8):1115-1124, 01 Oct 2024
Cited by: 0 articles | PMID: 39240167
Cellular immunity to nucleoproteins (NP) of Crimean-Congo hemorrhagic fever virus (CCHFV) and Hazara Virus (HAZV).
Med Microbiol Immunol, 213(1):20, 25 Sep 2024
Cited by: 1 article | PMID: 39320473
The chemical composition of secondary organic aerosols regulates transcriptomic and metabolomic signaling in an epithelial-endothelial in vitro coculture.
Part Fibre Toxicol, 21(1):38, 19 Sep 2024
Cited by: 0 articles | PMID: 39300536 | PMCID: PMC11411994
Local administration of mRNA encoding cytokine cocktail confers potent anti-tumor immunity.
Front Immunol, 15:1455019, 03 Sep 2024
Cited by: 0 articles | PMID: 39290693 | PMCID: PMC11406011
Go to all (701) article citations
Other citations
Data
Similar Articles
To arrive at the top five similar articles we use a word-weighted algorithm to compare words from the Title and Abstract of each citation.
The IL-12 family of cytokines in infection, inflammation and autoimmune disorders.
Inflamm Allergy Drug Targets, 8(1):40-52, 01 Mar 2009
Cited by: 199 articles | PMID: 19275692
Review
Influence of glycosylation on IL-12 family cytokine biogenesis and function.
Mol Immunol, 126:120-128, 18 Aug 2020
Cited by: 10 articles | PMID: 32823236
Revisiting the combinatorial potential of cytokine subunits in the IL-12 family.
Biochem Pharmacol, 165:240-248, 15 Mar 2019
Cited by: 13 articles | PMID: 30885765
Possible roles of IL-12-family cytokines in rheumatoid arthritis.
Nat Rev Rheumatol, 9(4):252-256, 23 Oct 2012
Cited by: 49 articles | PMID: 23090510 | PMCID: PMC10091854
Review Free full text in Europe PMC
Funding
Funders who supported this work.
NCI NIH HHS (3)
Grant ID: CA21765
Grant ID: P30 CA021765
Grant ID: R01 CA203689
NIAID NIH HHS (2)
Grant ID: R01 AI091977
Grant ID: AI091977
NINDS NIH HHS (4)
Grant ID: R01 NS030843
Grant ID: R37 NS030843
Grant ID: R29 NS030843
Grant ID: NS30843