Abstract
Free full text

Hepatic Inflammation and Fibrosis: Functional Links and Key Pathways
Abstract
Inflammation is one of the most characteristic features of chronic liver disease of viral, alcoholic, fatty and autoimmune origin. Inflammation is typically present in all disease stages, and associated with the development of fibrosis, cirrhosis and hepatocellular carcinoma. In the past decade, numerous studies have contributed to improved understanding of the links between hepatic inflammation and fibrosis. Here, we review mechanisms that link inflammation with the development of liver fibrosis, focusing on the role of inflammatory mediators in hepatic stellate cell (HSC) activation and HSC survival during fibrogenesis and fibrosis regression. We will summarize the contributions of different inflammatory cells, including hepatic macrophages, T- and B-lymphocytes, NK cells and platelets, as well as key effectors such as cytokines, chemokines, and damage-associated molecular patterns. Furthermore, we will discuss the relevance of inflammatory signaling pathways for clinical liver disease and for the development of anti-fibrogenic strategies.
Introduction
Fibrosis is a highly conserved response to hepatic injury, occurring in virtually all types of diseases with hepatocellular death. Accordingly, development of liver fibrosis is observed in patients with chronic viral hepatitis, non-alcoholic fatty liver disease (NAFLD), alcoholic liver disease, cholestatic and autoimmune liver disease. Fibrogenesis is a multi-cellular response with hepatic stellate cells (HSCs) constituting the main effectors - contributing to about 90% of extracellular matrix (ECM)-producing myofibroblasts (1) - and inflammatory signaling pathways (see Table 1) regulating many of the crucial cell-cell interactions as well as the recruitment of bone marrow-derived cells. During this multi-cellular wound healing response, HSCs interact closely with liver-resident cells such as hepatocytes, Kupffer cells, cholangiocytes, endothelial cells and infiltrating immune cells (2-8) (see Fig. 1 and Table 2). These dynamic interactions are characteristic for both the development as well as the regression of liver fibrosis, and control the activation status of HSCs and hepatic ECM content.
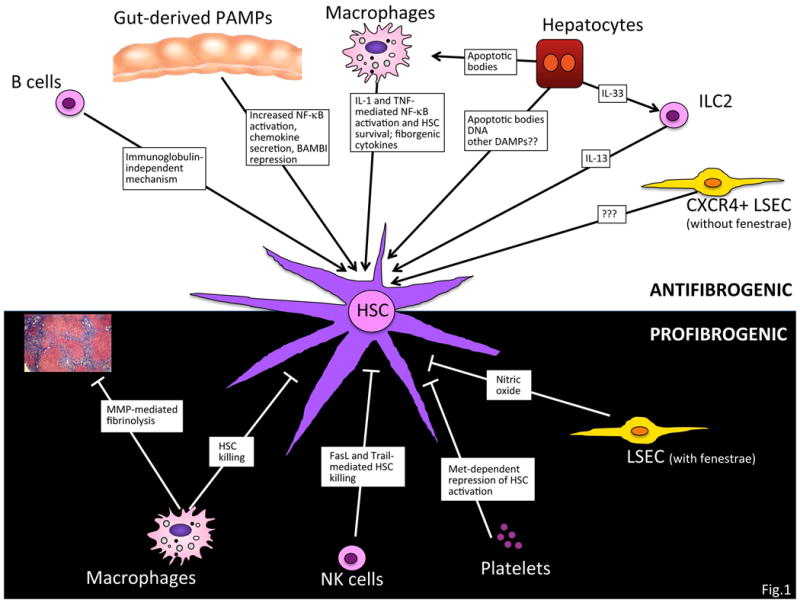
Numerous cell-cell interactions control both fibrogenesis and fibrosis regression. The activation of HSCs is promoted by interactions with hepatocytes, macrophages, innate lymphoid cells, B cells, NKT cells and CXCR4-positive endothelial cells. At the same time, HSC interaction with other cell types including NK cells, macrophages, platelets and endothelial cells results in decreased fibrogenesis and/or enhanced fibrosis regression.
Table 1
Origin | Cell Type | Target Cell/Mechanisms of Action | Liver Disease Models | References |
---|---|---|---|---|
Bone marrow | Macrophages* | - promote HSC survival via cytokines - upregulate TIMP1 via cytokines - promote liver fibrosis as demonstrated by genetic or pharmacologic ablation - promote fibrosis regression through MMP12 and MMP13-mediated ECM degradation and through killing of HSC via TRAIL | Fibrosis induced by CCl4 or
BDL Experimental NASH model induced by MCD or CDAA diet | (2,6,14) |
NK cells | - active killing of HSC via FasL and
Trail - reduction of liver fibrosis in mice treated with poly I:C activated NK cells - increased fibrosis in SCID-BEIGE mice lacking NK cells | Fibrosis induced by CCl4, DDC diet, or CCl4 plus EtOH | (7,28,31) | |
NKT cells | - promote liver fibrosis through
CXCL16-CXCR6 - αGalCer treatment enhances NKT cell-mediated liver fibrosis | Fibrosis induced by CCl4, or MCD diet | (21,32) | |
B lymphocytes | - promotion of collagen but not αSMA
expression in HSC - effects independent of Ig | Fibrosis induced by CCl4 or ANIT | (34) | |
T lymphocytes | - no decrease in fibrosis observed in mice lacking CD4+ T cells, CD8+ T cells, and γδT cells | Fibrosis induced by CCl4 | (34) | |
Neutrophils | - recruited in a TLR2-CXCL2-S100A9-dependent
manner - play minor roles for liver fibrosis | Fibrosis induced by CCl4, | (35) | |
Innate lymphoid cells (ILC2) | - in response to IL-33, ILC2 expand and promote HSC activation and liver fibrosis through IL-13 | Fibrosis induced by CCl4, BDL, Schistosoma mansonii infection or IL-33 overexpression | (19) | |
Platelets | - Increased fibrosis in thrombocytopenic
mice - Decreased fibrosis in SCID mice receiving human platelets - repression of HSC activation by platelets through Met | Fibrosis induced by BDL or CCl4 | (38,39) | |
Liver resident | Hepatocytes | - promotion of HSC activation and liver
fibrosis through heptocyte-secreted IL-33 - apoptotic hepatocytes promote HSC activation through phagocytosis - apoptotic hepatocytes increase inflammatory and profibrogenic cytokines in macrophages | Fibrosis induced by CCl4, BDL, Schistosoma mansonii infection | (5,17-19,38,39) |
Cholangiocytes | - promotion of portal fibroblast activation by
cholangiocyte-secreted CCL2 - promotion of cholangiocyte proliferation via hyaluronan from myofibroblasts | Fibrosis induced by BDL and in vitro models | (24,25) | |
Liver Sinusosoidal Endothelial Cells (LSEC) | - LSEC from normal liver suppress HSC
activation - LSEC from fibrotic liver lose the ability to suppress HSC activation - LSEC from fibrotic liver promote HSC activation depending on CXCR4 and FGFR-1 expression on LSEC | Fibrosis induced by CCl4, TAA or BDL | (3,4,27) |
Table 2
Class | Mediator | Target Cell and Mechanisms of Action | Liver Disease/Liver Disease model | References |
---|---|---|---|---|
Inflammatory Cytokines | IL-1β | - upregulates TIMP1 and downregulates BAMBI in
HSC - promotes HSC survival - promotes lipid accumulation and cell death in hepatocytes during NASH and ALD | Experimental fibrosis induced by BDL or
TAA Experimental NASH induced by CDAA diet Experimental ALD model induced by Lieber-DeCarli and EtOH binge injection | (6,51-53) |
IL-33 | - secreted from damaged hepatocytes, stimulating ILC2 to produce IL-13 that in turnactivates HSC | Human Liver Cirrhosis; experimental fibrosis induced by CCl4, TAA, Schistosoma mansonii infection | (19) | |
TNFα | - induces hepatocyte apoptosis - upregulates TIMP1 and downregulates BAMBI in HSC - promotes HSC survival and proliferation - activates liver macrophages | Experimental fibrosis induced by
BDL Experimental NASH model induced by MCD diet | (41,55-57) | |
IL-17 | - stimulates Kupffer cells and HSC to produce
IL-6, TNFα and TGF-β - activates NF-κB and STAT3 in Kupffer cells and HSC - HSC activation through STAT3 | Hepatitis B Experimental fibrosis induced by CCl4 or BDL | (58,59) | |
IL-20 | - promotes activation, proliferation and
migration of HSC - prevents hepatocyte injury | HBV- and HCV-induced liver
cirrhosis Experimental fibrosis induced by CCl4 | (60) | |
IL-22 | - induces HSC senescence through
STAT3-p53 - HSC senescence inhibits liver fibrosis | HBV- and HCV- and alchol-induced liver
cirrhosis Experimental fibrosis induced by CCl4 | (61,62) | |
IFNγ | - suppresses HSC proliferation and
activation - activate NK cells to promote HSC killing | Experimental fibrosis induced by CCl4 | (29) | |
Chemokines | CCL2 (MCP-1) | - macrophage and HSC recruitment, and HSC activation | Experimental fibrosis induced by
CCl4 or BDL Experimental NASH model induced by MCD or CDAA diet | (65-67) |
CCL5 | - macrophage and HSC recruitment; HSC activation | Experimental fibrosis induced by CCl4 or BDL | (68,69) | |
CXCL9 | - suppresses HSC activation - inhibits angiogenesis that inhibits liver fibrosis | Experimental fibrosis induced by CCl4 | (20) | |
CXCL10 | - promotes hepatocyte death and HSC
activation - inhibits NK cell-mediated HSC inactivation | Experimental fibrosis induced by CCl4 | (20) | |
CX3CL1 | - prolongs Kupffer cell survival - promotes anti-inflammatory property in Kupffer cells | Experimental fibrosis induced by CCl4 or BDL | (72,73) | |
Gut Microbiota Axis/Toll like receptor pathway | TLR4 | - directly stimulate HSC to downregulate BAMBI
and produce chemokines in BDL and CCl4–induced liver
fibrosis - stimulates Kupffer cells to produce pro-inflammatory and fibrogenic cytokines that activate HSC in ALD and NASH - stimulates LSEC to induce angiogenesis that promote HSC activation and fibrosis | Experimental fibrosis induced by
CCl4 or BDL Experimental NASH model induced by MCD or CDAA diet Experimental ALD model induced by Lieber-DeCarli or Tsukamoto-French model | (14,40,42,44-46) |
TLR2 | - stimulates Kupffer cells to produce
cytokines that activate HSC in NASH - stimulates macrophages in intestine, which promote bacterial translocation | Experimental fibrosis induced by
CCl4 or BDL Experimental NASH model induced by CDAA diet | (47,100) | |
TLR9 | - stimulates Kupffer cells to produce
cytokines that activate HSC in NASH - stimulates HSC by host DNA released from apoptotic hepatocytes | Experimental NASH model induced by CDAA
diet Experimental fibrosis induced by CCl4 or BDL | (48,51) | |
TLR3 | - stimulates NK cells to produce IFN-γ that induces anti-fibrotic effect by killing HSC | Experimental fibrosis induced by CCl4 or Lieber-DeCarli plus CCl4 | (7,29,31) | |
TLR7 | - stimulates dendritic cells to produce type I interferon that inhibits liver fibrosis | Experimental fibrosis induced by CCl4 or BDL | (49) |
Cell death and inflammation constitute two characteristic and intricately linked features of chronic liver disease that promote the development of fibrosis. Accordingly, cell death, inflammation and fibrosis are key parameters of histological classification scores such as the Scheuer and Knodell classifications (9,10). Cell death is typically the precipitating event, which subsequently triggers activation of inflammatory and fibrogenic signaling cascades (Fig. 1). Biomarkers of cell death correlate well with risk of fibrosis development (11). Wound healing responses to acute injury are beneficial in several ways: (i) Fibrosis provides mechanical stability; (ii) inflammatory cells contribute to the removal of cellular debris; and (iii) inflammatory signals also exert important functions in the promotion of liver regeneration (12). Together, these events ensure restoration of hepatic architecture and function following acute liver injury. However, these wound healing responses become maladaptive when the underlying disease cannot be eliminated and hepatocyte death becomes chronic, thus triggering chronic inflammation and the development of progressive liver fibrosis. Functional links between cell death and inflammation exist at several levels and in both directions (Fig.2). For example, infiltrating immune cells are important mediators of cell death in the setting of viral hepatitis, where they kill infected hepatocytes (13). Likewise, chronic inflammation and hepatic infiltration of leukocytes increase the presence of cell death-inducing cytokines and ligands from the TNF receptor family, thereby amplifying liver injury (11). At the same time, cell death constitutes the most important trigger for leukocyte infiltration and inflammation (11). As cell death and inflammation in the liver are virtually inseparable, it remains controversial to what extent cell death promotes fibrosis independently of inflammation and vice versa.
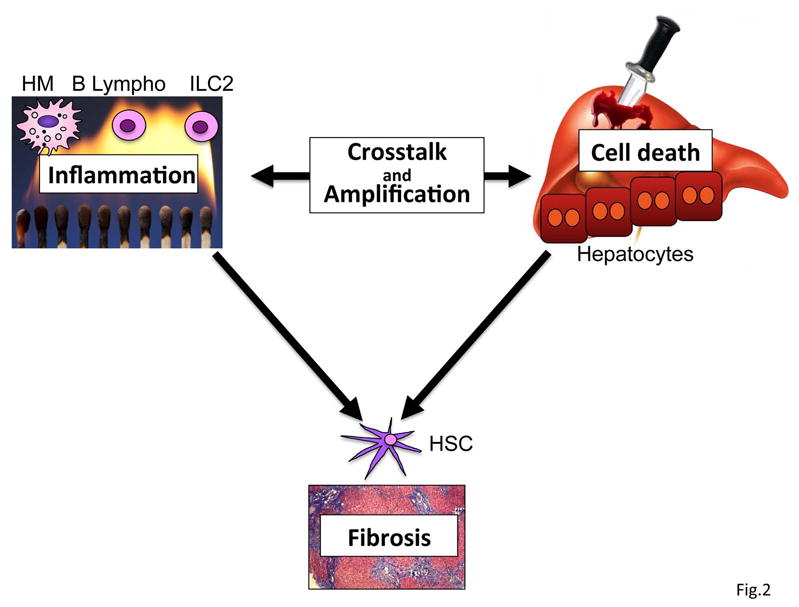
In many disease settings, cell death and inflammation are intimately linked. Cell death triggers the infiltration of bone marrow-derived leukocytes and inflammation in the liver. Vice versa, inflammatory cells can amplify cell death through the release of cytotoxic cytokines or directly kill hepatocytes. Together, cell death and inflammation promote the development of fibrosis. HM, hepatic macrophages.
1. Cell Types that Link Inflammation and Fibrosis
Hepatic stellate cells
As main executors of fibrogenesis, HSCs receive a wide range of signals from injured hepatocytes and the perturbed hepatic microenvironment, most of these mediated by cytokines. As such, HSCs interact with hepatocytes, hepatic macrophages, lymphocytes and endothelial cells resulting in the promotion of fibrogenesis (2-6,14). Additionally, activated HSCs interact with natural killer cells resulting in HSC death and termination of the fibrogenic response (7). HSCs of murine or human origin are highly responsive to pro-inflammatory cytokines and LPS, resulting in the activation of pro-inflammatory signaling pathways such as nuclear factor κB (NF-κB) and AP-1, and subsequent production of chemokines and cytokines (14,15). However, compared to the key role of macrophages and other leukocyte populations HSCs probably make only minor contributions to overall hepatic inflammation in vivo. Rather than promoting the overall inflammatory status of the liver, it is likely that HSCs mainly function as recipients of inflammatory signals, and that inflammatory pathways regulate their activation and ensure the survival of activated HSCs, e.g. through NF-κB. Along this line, clinical evidence suggests that cirrhotic livers, especially in advanced stages, display a lower degree of inflammation (“burned-out cirrhosis”), suggesting that cells other than myofibroblasts are the primary sources of hepatic inflammation. Putting these findings into context, it is likely that HSCs exert specific function in the regulation of inflammation within the fibrotic liver: (i) Following their initial activation, HSCs require “perpetuation” signals that maintain HSC in their activated state and contribute to their survival (16). It is likely that many survival signals are mediated by cytokines, either coming from HSCs themselves in an autocrine manner or from inflammatory cells. (ii) HSCs may also provide specific chemotactic signals that regulate their interaction with inflammatory cell types, both during fibrogenesis (promoting the recruitment of cells that promote HSC activation) and during fibrosis regression (promoting recruitment of cells that degrade ECM and that kill HSCs). (iii) HSCs provide a link between gut and liver through their high expression of Toll-like receptors (TLRs), promoting HSC activation and fibrosis (14). (iv) Specific, yet unidentified chemotactic signals or gradients promote the migration of HSCs towards site of injury.
Hepatocytes
Hepatocyte death, triggered by viral infection, steatosis or alcohol toxicity, is the main driver of chronic liver inflammation and fibrosis. As such, apoptotic hepatocyte bodies have been demonstrated to promote the secretion of pro-inflammatory and profibrogenic cytokines from macrophages, and to directly promote HSC activation (5,17,18). Moreover, it has been suggested that damage-associated molecular patterns (DAMPs) released from dying hepatocytes may also directly or indirectly promote fibrogenesis (11). Recent studies also implicated inflammatory cytokine secretion from stressed hepatocytes, e.g. IL-33, in the promotion of fibrogenesis (19). Pathways by which hepatocyte death promotes fibrosis have recently been reviewed in detail elsewhere (11).
Macrophages and dendritic cells
Liver injury promotes the recruitment of LY6Chi macrophages from the bone marrow, adding macrophages to the already-large number of liver-resident macrophages (“Kupffer cells”). Macrophages constitute an essential component of the fibrogenic response as pharmacologic or genetic macrophage depletion blunt fibrosis (2,6,14). The mechanisms and mediators through which macrophages promote HSC activation are not fully understood. Besides releasing profibrogenic cytokines, macrophages also promote the survival of activated HSCs, thereby keeping their numbers elevated (6). In addition, macrophages may promote HSC migration through secretion of CCL2, CCL3, CCL4, CCL5, CCL7, and CCL8 (20,21). Accordingly, hepatic macrophages are often found in close proximity to fibrotic scars (21). Of note, macrophages have divergent functions in fibrogenesis and specific populations also promote fibrosis resolution through enhanced ECM degradation via increased MMP12 and MMP13 expression (2,22). In addition, “pro-resolution” macrophages may additionally contribute to the killing of HSCs, e.g. through the expression of TRAIL (21). Dendritic cells are a myeloid lineage closely related to macrophages. Although dendritic cells do not contribute to fibrosis development in mice (6), they have been reported to promote the resolution of liver fibrosis via MMP9-dependent mechanisms (23).
Cholangiocytes
Cholestatic liver disease is often associated with profound inflammation and fibrosis. Cholangiocytes are a rich source of inflammatory cytokines and chemokines and highly responsive to TLR agonists (8). Moreover, cholangiocytes interact with multiple inflammatory cell types in cholangiopathies, in particular T lymphocytes (8). Cholangiopathies and the associated bile duct proliferation often result in the activation and expansion of myofibroblasts, surrounding ducts. Recent fate tracing studies in multiple models of murine cholestatic liver fibrosis demonstrated that HSCs – and not portal fibroblasts - are the main ECM-producing cell type (1). However, the interactions between cholangiocytes and HSCs are not well characterized, and it is not known whether chemokines or other pro-inflammatory mediators are involved. On the other hand, several studies have shown CCL2-mediated crosstalk between cholangiocytes and portal fibroblasts (24). Vice versa, hyaluronan from fibrogenic cells promotes the proliferation of cholangiocytes via the hyaluronan receptor CD44 (25). In addition to fibrosis in cholestatic liver disease, there is also a significant desmoplastic response to cholangiocarcinoma, providing additional evidence for specific signals from cholangiocyte compartment that promote the recruitment of myofibroblasts (26). Together, these data suggest that the interaction of cholangiocytes with multiple cell types – including lymphocytes, HSC and portal fibroblasts – contributes to chronic inflammation and fibrosis in cholestatic liver disease.
Endothelial cells
Like in most other organs, endothelial cells not only closely interact with leukocytes during their transmigration from blood vessels into tissue, but also form functional units with pericytes. Accordingly, HSCs as liver-specific pericytes interact closely with endothelial cells in the normal and injured liver. In the normal liver, fenestrated liver sinusoidal endothelial cells (LSECs) actively repress the activation of HSC (3,27). Capillarization of endothelia following injury leads to the loss of the ability of LSECs to suppress HSC activity, hence providing a permissive state for HSC activation (3,27). Recent studies suggest that LSECs in fact hold the key between the decision to regenerate (via CXCR7 expressed on LSECs) or to promote HSC activation and fibrosis (via CXCR4 and FGFR-1 expressed on LSECs) (4). How precisely CXCR4 and FGFR-1 on LSECs promote HSC activation remains to be determined. LSECs themselves are also highly responsive to TLR ligands and a source of multiple inflammatory mediators. Together, these data suggest that LSECs and HSCs – as a functional unit – interact with multiple other cell types in chronic inflammation, thereby driving fibrogenesis.
Natural killer cells and natural killer T cells
Liver lymphocytes are enriched in natural killer (NK) and NKT cells. NK cells exert key roles in innate immune responses against infection and tumors. In hepatic fibrosis, NK cells exert anti-fibrotic functions (7,28) through IFNγ and by killing activated HSCs via TRAIL and FasL (29). Data from patients demonstrate an inverse correlation of fibrosis stage with the ability of NK cells to kill HSCs (30). The anti-fibrotic function of NK cells may be suppressed in the setting of liver fibrosis through elevated levels of transforming growth factor β (TGFβ) (29) and also through alcohol consumption (31). NKT cells express both NK and T cell markers that recognize lipid antigens presented by CD1d molecule and produce both Th1 and Th2 cytokines (IFN-γ and IL-4, respectively) to regulate innate and adaptive immunity. Although invariant NKT cells induce killing of HSCs, activation of invariant NKT cells by α-GalCer enhances CCl4-induced liver fibrosis. In addition, the CXCL16-CXCR6 axis plays a role in migration of hepatic NKT cells to promote CCl4-induced liver fibrosis. (21,32).
T and B lymphocytes
In the chronically injured liver, lymphocytes and HSC are commonly localized in very close proximity, suggesting functional interactions (33). Different lymphocyte subsets exert specific roles in HSC activation and liver fibrosis. B cells represent as much as half of the intrahepatic lymphocyte population. JH−/− mice, which lack B cells, displayed significantly decreased CCl4-induced liver fibrosis induced as did RAG2-/- mice which lack both B and T cells (34). The effect of B cells on liver fibrosis is not mediated by secreted or surface immunoglobulin as shown by genetic approaches (34). Surprisingly, the effect of B cells was selectively on ECM production without affecting αSMA-positive myofibroblast numbers. In contrast to the suggested role of B cells, T cells do not exert a major impact on toxin-induced liver fibrosis, highlighted by the finding that mice lacking CD4+ T cells, CD8+ T cells, and γδT cells have unaltered fibrosis induced by CCl4 (34). However, in human diseases with a significant role of T cell-mediated immunity such as viral hepatitis, the role of T cells may be different than in mouse models of toxic liver fibrosis.
Neutrophils
Neutrophils are crucial for the elimination of invading organisms, but overwhelming activation of neutrophils may amplify liver damage. In the CCl4 model, TLR2, but not TLR4, plays a role in recruiting neutrophils (35). Neutrophils are recruited into the liver in a CXCL2-dependent or S100A9-dependent manner. However, fibrogenic responses were unchanged in mice with depletion of neutrophils, suggesting no direct role of neutrophils in liver fibrogenesis (35).
Innate lymphoid cells
Innate lymphoid cells (ILC) are a recently discovered cell population, which express many TH cell-associated cytokines, but no cell-surface markers of known lymphoid lineage. Among these, ILC2 are producers of IL-5, IL-9 and IL-13. In liver fibrosis, hepatic ILC2 expand in response to IL-33, secreted from hepatocytes, and contribute to HSC activation through an IL-13- and STAT6-dependent but TGFβ-independent pathway (19). The relevance of this pathway is further emphasized by increased IL-33 levels in cirrhotic patients (19). A profibrotic role of ILC2 has also been observed in the lung (36). The contribution of other ILC subset to hepatic fibrosis remains currently unknown.
Platelets
In parallel to the decreased number and function of platelets in chronic liver disease, there is commonly also hypercoagulability, caused by decreased levels of protein C, protein S, antithrombin, α2-macroglobulin and heparin cofactor II and increased levels of factor VIII and von Willebrand factor, resulting in the formation of micro- and macrothrombi in the liver (37). In this setting platelets may be a source of growth factors such as PDGF and contribute to the activation of profibrotic coagulation cascades (37). However, several studies have described anti-fibrotic effects of platelets (38,39). As such, thrombocytopenic mice develop exacerbated liver fibrosis and platelets suppress collagen expression of co-cultured HSCs in a Met-dependent manner (38).
2. Key Pathways Modulating Hepatic Inflammation
2A. Triggers of Inflammatory Signaling
The gut microbiota -TLR pathway
Increased bacterial translocation is a hallmark of chronic liver disease which not only contributes to characteristic infectious complications but also generates a chronic inflammatory state in the liver. These inflammatory responses are mediated by bacterial molecules, termed pathogen-associated molecular patterns (PAMPs), through the activation of TLRs. The relevance of the gut microbiota-TLR4 for liver fibrosis has been demonstrated by the ability of non-absorbable antibiotics and genetic TLR4 inactivation to suppress liver fibrosis (14,40). The TLR4 ligand lipopolysaccharide (LPS) activates inflammatory signals in multiple hepatic cell types including HSCs, which express high levels of TLR4 even in the quiescent state. In HSCs, TLR4 signaling induces expression of chemokines and adhesion molecules suggesting a contribution of TLR4-mediated signals in HSCs for the recruitment of macrophages to sites of fibrogenesis (14). On HSCs, TLR4 signaling also downregulates the TGFβ decoy receptor BMP and activin membrane bound inhibitor (BAMBI), thereby enhancing TGF-β-mediated HSC activation (14,41). Moreover, TLR4 signaling can promote portal hypertension and angiogenesis in liver fibrosis by stimulating LSECs directly or indirectly through fibronectin produced from HSCs (40,42). The clinical relevance of TLR4 in human liver fibrosis has been demonstrated by the SNP analysis for liver fibrosis in HCV patients where inhibitory TLR4 SNPs are associated with decreased fibrosis (43). In alcohol- and NASH- induced fibrosis, TLR4 acts mainly through Kupffer cells via the production of pro-inflammatory cytokines, such as TNFα, IL-1β, CCL2 and CCL20 (44-46). In contrast to the contribution of TLR4 via liver-resident cells, TLR2 plays a role in macrophages in the intestine where it regulates expression of intestinal tight junction proteins protecting from bacterial translocation and subsequent HSC activation (47). TLR9, the receptor for CpG-motif containing DNA, is expressed on HSCs. TLR9 on HSCs can also be activated by damaged hepatocyte-derived DNA, which contributes to liver fibrosis progression (48).
In contrast, TLR3 and TLR7 signaling protects mice from liver fibrosis. TLR3 activation induces IFN-γ production in NK cells, which causes cell cycle arrest and apoptosis of HSC, and suppressed liver fibrosis (7,29). In alcoholic liver disease, TLR3 signaling in NK cells is inhibited, thereby eliminating their inhibitory effect on HSC activation and liver fibrosis (31). Activation of TLR7 on dendritic cells induces type I IFN production that stimulates Kupffer cells to produce anti-fibrotic IL-1 receptor antagonist, thereby inhibiting liver fibrosis (49).
Inflammatory Cytokines
IL-1β is a potent inflammatory cytokine mainly produced by macrophages. IL-1β production requires stimulation with TLR ligands as well as a second signal such as MDP-mediated NLR stimulation or ATP-mediated P2X7 stimulation to induce both upregulation of Ilb transcription and subsequent processing of IL-1β protein by the inflammasome multiprotein complex (50). IL-1β participates in toxic, ethanol and NASH-induced fibrosis (51-53). In HSCs, IL-1β mediates upregulation of fibrogenic TIMP-1 and downregulation of BAMBI (51). Moreover, IL-1β can prolong the survival of HSCs (6). Knock-in mice with constitutive activation of NLRP3 and hyperproduction of IL-1β develop spontaneous liver injury and fibrosis. (54).
TNFα is another highly pro-inflammatory cytokine. Effects of TNFα are diverse, contributing to hepatocyte apoptosis, immune cell activation and HSC activation. TNFα- and TNFR type I deficient mice display reduced cholestatic liver fibrosis (55). TNFα stimulation does not increase collagen α1(I) production, but may contribute to fibrosis by upregulating TIMP-1, downregulating BAMBI and by preventing HSC apoptosis (41,55-57).
IL-17 is mainly produced from CD4+ Th17 T cells, and its upregulation is observed in viral hepatitis, alcoholic liver disease and autoimmune hepatitis. In experimental liver fibrosis, IL-17A stimulates both Kupffer cells and HSCs to produce IL-6, TNFα and TGFβ through activation of NF-κB and STAT3 (58,59). In addition to these pro-inflammatory activities, IL-17 also directly induces STAT3-dependent HSC activation. Both IL-17A- and IL-17 RA-deficient mice display decreased liver fibrosis (58,59).
Recently, IL-20 was identified as a profibogenic cytokine that is upregulated in human and murine liver fibrosis (60). IL-20 promotes the activation, proliferation and migration of HSCs (60). Inhibition of IL-20 or its receptor by genetic or pharmacologic approaches decreased not only fibrosis but also liver injury (60), suggesting that IL-20 may not only act on HSCs but also hepatocytes.
IL-22 has been implicated in the defense against bacterial infections by inducing anti-microbial proteins including β-defensin, as well as in cell proliferation, tissue repair and wound healing. In the liver, IL-22 suppresses fibrosis by inducing HSC senescence in a STAT3-p53-p21-dependent manner (61). In human liver cirrhosis, IL-22 levels are elevated and associated with the development of ascites, hepatorenal syndrome, spontaneous bacterial peritonitis and reduced survival (62). While IL-22 has the capacity to inhibit liver fibrosis, its upregulation can be used as biomarker to predict the prognosis of liver cirrhosis.
IL-33 is an IL-1 family member and binds to the IL-33 receptor ST2 and IL-1R associated protein (IL-1R3) heterodimer. IL-33 and ST2 expression are significantly upregulated in murine and human liver fibrosis (19). Liver injury induces hepatocellular IL-33 secretion which, in turn, stimulates ILC2 to produce IL-13. IL-13 then promotes HSC activation through IL-4Rα and STAT6 activation (19). IL-33-deficient mice, mice treated with soluble ST2 receptor, or ILC2-depleted mice exhibit reduced liver fibrosis, indicating that IL-33 and hepatic innate lymphoid cells link hepatocellular injury to fibrogenesis (19).
TGF-β is a pleiotropic cytokine with key roles in development, immunity, carcinogenesis and wound healing (63). TGF-β represents an important link between immune cells and fibrogenic cells across organs: The majority of TGF-β is produced by immune cells, including hepatic macrophages (63), and directly promotes fibrogenesis in myofibroblasts. In HSCs, TGF-β induces the transcription of type I and III collagen through Smad-dependent pathways, but also represses HSC proliferation (63). HSCs also produce TGF-β, but to a lesser degree. TGF-β requires processing to become bioactive, which can be mediated by MMPs, pH, thrombospondin-1, ROS or αv integrins (63,64). TGF-β and pro-inflammatory signaling pathways interact at multiple levels, as demonstrated by the downregulation of TGF-β pseudoreceptor BAMBI by LPS or TNFα (41). TGF-β also represses the activity of NK cells, thereby preventing NK cell-induced HSC apoptosis (29), suggesting that some contribution of TGF-β in fibrosis occur also in bone marrow-derived inflammatory cells, where TGF-β exerts multiple immunregulatory functions.
Chemokines
The major function of chemokines and their receptors is the recruitment of immune and non-immune cells into the inflamed sites. In the liver, chemokines have a major role in the coordination of the multi-cellular wound healing response, including the recruitment of inflammatory cells from the circulation and bone marrow, as well as the migration of resident liver cells to sites of injury. Among the many chemokines involved in hepatic injury and wound healing processes, CCL2 (MCP-1) and CCL5 (RANTES) are the best characterized. CCL2 – mainly produced by Kupffer cells and HSCs - contributes to the recruitment of macrophages and monocytes into the liver. Moreover, CCL2 promotes HSC activation (65-67). Genetic or pharmacologic inhibition of CCL2 or its receptor CCR2 result in reduced hepatic fibrosis in various experimental fibrosis models (65,67). CCL5 and its receptors CCR1 and CCR5 have also been implicated in promoting liver fibrogenesis. Both liver macrophages and HSCs express CCR1 and CCR5, with CCR1 predominantly promoting fibrogenesis indirectly through macrophages and CCR5 exerting effects on HSC. Inhibition of the ligands for CCR1 and CCR5 by using viral-derived chemokine inhibitor 35k and CCL5 antagonist Met-CCL5 both inhibit the progression of liver fibrosis (68,69). Moreover, pharmacologic inhibition of CCL2 and CCL5 also promote fibrosis regression (65,69). The CCL1-CCR8 and CCL25-CCR9 axis also play crucial roles in recruiting macrophages to promote liver fibrosis (70,71). In contrast to pathogenic effect of chemokines in liver fibrosis, the CX3CL1-CX3CR1 interaction on liver macrophages negatively regulates liver inflammation by enhancing macrophage survival and partly inducing an anti-inflammatory phenotype (72,73). CX3CL1 is mainly expressed on HSCs, which suggests an anti-inflammatory property of HSCs. Additional chemokines implicated in the regulation of hepatic fibrosis, including CXCL16 and its receptor CXCR6, CCL20 and its receptor CCR6, and CXCL9 and CXCL10 and their receptor CXCR3 have been reviewed in detail elsewhere (20).
Interferons
Type I interferons (IFNα and IFNβ) and type II interferon (IFNγ) are increased in chronic liver disease. They are secreted by a wide range of immune cells, often in response to activation of TLR as well as cytosolic nucleic acid receptors (e.g. RIG-I, MDA-5 and cGAS) (74) and increased in chronic liver disease. IFNγ exerts direct anti-fibrogenic effects in HSCs by suppressing proliferation and αSMA expression, and indirectly through NK cell activation (29,75). IFNα downregulates basal and TGFβ-induced collagen gene transcription in HSCs. Accordingly, treatment with type I or type II interferons suppresses experimental liver fibrosis (7,76,77). In addition to direct anti-fibrogenic effects on HSCs, IFNγ also promotes the activation of NK cells that in turn kill activated HSCs, thereby ameliorating liver fibrosis. Accordingly, interferon-based antiviral therapies reduced fibrosis in some studies even in virological non-responders (78) Interferons have also been tested as pure anti-fibrotic agents in patients. Anti-fibrotic effects were observed in small trial in patients with less advanced fibrosis (79), but not in patients with advanced fibrosis (80). Recent studies have suggested that in vivo application of IFNγ only inefficiently target HSCs, and subsequently demonstrated that targeted delivery to HSCs vastly improves its anti-fibrotic effects (81).
Inflammatory and fibrogenic signals from dead cells: DAMPs and apoptotic bodies
Cell death represents the key and most upstream trigger of fibrogenesis as demonstrated by genetic models in which hepatocyte-specific deletion of cytoprotective factors not only results in cell death, but also in the development of liver fibrosis (11). However, mechanisms linking cell death to inflammation and fibrogenesis are not fully understood. Although DAMPs released from dead cells may provide such a link, no fibrogenic DAMP has yet been identified. It is possible that DAMPs do not directly act on HSCs but through other cell types that in turn activate HSCs. As such, multiple DAMPs including HMGB1, formyl peptides and ATP (via its receptor P2X7) may promote hepatic inflammation (82), and indirectly – through increased inflammation –promote fibrogenesis. Apoptosis is a well-established form of cell death in many human diseases. Although apoptosis is considered a non-reactive form of cell death, there is overwhelming clinical evidence that it is linked to fibrosis development (11). Whereas secondary necrosis or necroptosis may occur in this setting, there is also evidence suggesting that apoptotic bodies may directly act on inflammatory cells, in particular macrophages, as well as HSCs to promote fibrogenesis through mechanisms that involve phagocytosis of apoptotic bodies (11).
2B. Downstream Signaling Pathways Linking Inflammation and Fibrosis
NF-κB
NF-κB is a transcription factor that acts as a key regulator of inflammation and cell death, thus exerting a major role in chronic liver diseases. NF-κB is activated by various stimuli and cellular stress, such as TLRs, IL-1β and TNFα. Suppression of NF-κB activation in hepatocytes is required for efficient induction of cell death (reviewed in Ref (11)), and subsequent responses such as inflammation and fibrogenesis. As such, hepatocyte-specific deletion of key regulators of NF-κB activation including NEMO or TAK1 causes spontaneous cell death and subsequent hepatic inflammation, fibrosis and carcinogenesis (83). While physiological NF-κB activation prevents hepatocyte apoptosis and is therefore protective, pathological overactivation of NF-κB in hepatocytes or inflammatory cells promotes liver inflammation by increased production of pro-inflammatory, NF-κB-regulated cytokines such as TNFα, IL-1β and IL-6. Selective inhibition of NF-κB in Kupffer cells by using the NF-κB decoy nucleotides attenuates CCl4-induced liver fibrosis (84). Moreover, NF-κB activation in HSCs results in the promotion of fibrogenesis due to increased HSC survival. Mediators that increase NF-κB and survival in HSC appear to be largely derived from Kupffer cells, such as IL-1β and TNFα (6), but also include LPS and its receptor TLR4 (14). In addition, NF-κB activation triggers the secretion of chemokines by HSCs (promoting the recruitment and interaction with inflammatory cells) and is responsible for TLR4- and TNFα-mediated downregulation of BAMBI (sensitizing HSCs to TGFβ, thereby enhancing fibrogenesis) (14,83). c-Rel, another subunit of NF-κB, can promote liver fibrosis through HSC activation (85). On the other hand, deficiency of p50 – a subunit of NF-κB that may suppress its transcriptional activity - results in aggravated CCl4-induced liver inflammation and fibrosis by increasing neutrophil infiltration and elevating TNFα expression (86).
c-Jun-N-terminal Kinases (JNK)
JNKs are MAPKs activated by various stimuli including TLRs, IL-1β, TNFα, ROS, and saturated free fatty acids. The liver expresses JNK1 and JNK2 but not JNK3 (87). JNK substrates include transcription factors, such as c-Jun, Jun B, Jun D and Fos, which are the components of transcription factor AP-1. JNK is involved in multiple signaling cascades with relevance to hepatocellular injury, metabolism, inflammation and fibrosis (reviewed in (87)). In HSCs, JNK exerts a direct profibrogenic role by promoting PDGF, TGF-β and angiotensin II-induced proliferation, αSMA expression and/or collagen production (88). Interestingly, JNK plays important roles in TGF-β and PDGF-mediated Smad2 and Smad3 phosphorylation (89). Together, these data implicate JNK as a link between inflammatory and fibrogenic signals. In addition to regulating fibrogenic events, JNK also regulates hepatic steatosis, cell death in hepatocytes and inflammatory gene expression in immune cells, all of which modulate liver fibrosis (87). Importantly, JNK isoforms often exert distinct functions in chronic liver disease. As such, JNK1 promotes, but JNK2 protects from cholestasis- and CCl4-induced liver fibrosis (88).
3. Links between inflammation and fibrosis in specific liver diseases
Viral hepatitis
HCV-induced liver disease typically presents with simultaneous presence of periportal inflammation and fibrosis, suggesting a relevant link between these two events. In patients with chronic viral hepatitis, biomarkers of cell death, including serum ALT and cytokeratin 18, provide the strongest determinants of fibrosis development (83). This supports the hypothesis that immune cell-mediated killing of hepatocytes is the primary disease driver in this setting, and that inflammation and fibrosis are subsequent events triggered by cell death. Accordingly, a number of inflammatory chemokines and their receptors, including CCL2, CCL21, IL-8, IL-17, IL-22, CXCL9, CXCL10, CXCL11 and CXCR1 have been positively associated with fibrogenesis in HBV- and HCV-infected patients (90-94), suggesting that inflammation represents an important driver or modifier of fibrogenesis in these patients. Moreover, HCV-induced IL-1β production via TLR7, MyD88 and NLRP3 provides an additional cell-death independent pathway by which HCV promotes hepatic inflammation (95). It has also been shown that HCV core and non-structural proteins can directly induce inflammatory and profibrogenic events in HSCs (96). There also appears to be an important interplay between immune cells and HSCs in HCV infection as CCL21 - derived from T cells and dendritic cells in HCV-infected patients - promotes migration and activation of extracellular signal-regulated kinase, Akt, and NF-κB via CCR7, resulting in the induction of pro-inflammatory genes in HSCs (92).
Alcoholic Liver Disease
In the United States, 48% of cirrhosis-related death is associated with heavy alcohol drinking. Alcohol-mediated hepatocyte injury, via generation of acetaldehyde and ROS, increases the production of ROS, TNFα, IL-1β, TGF-β, and CCL2 in the liver, predominantly through macrophages (53,97). In part, increased inflammation in alcoholic liver disease (ALD), is mediated by increased translocation of intestinal bacterial products, such as LPS (97), promoting TLR4 activation on liver macrophages and HSCs, cytokine and chemokine production, leading to liver inflammation and fibrosis.
In addition to increased overall inflammation and fibrosis-promoting activation of the TLR4, the enhanced fibrogenesis in ALD is also due to inhibition of NK cell activity by chronic ethanol exposure (31). Ethanol consumption downregulates expression of TRAIL and IFNγ in NK cells and inhibits NK cell killing of HSCs, thereby enhancing HSC survival and liver fibrosis.
With the exception of alcoholic hepatitis, an often lethal, acute-on-chronic form of ALD that is commonly treated with corticosteroids, there are no approaches that target inflammatory signaling pathways in ALD and abstinence remains the key therapeutic principle. Based on strong evidence in animal models, it is conceivable that targeting the gut-microbiota axis in patients, e.g. by using non-absorbable antibiotics such as Rifaximin, may improve both inflammation and fibrosis.
Non-Alcoholic Steatohepatitis
Non-alcoholic steatohepatitis (NASH) is distinguished from simple steatosis by the presence of hepatocyte death, inflammation and a varying degree of fibrosis. Hepatocyte death and inflammation are considered important triggers of disease progression, mediated by HSC activation and fibrosis. Multiple mechanisms have been suggested to promote the progression from simple steatosis to NASH and NASH-associated fibrosis: (i) Inflammatory cytokines released from “inflamed adipose tissues” in obese patients (98); (ii) ROS production by dysregulated hepatic lipid and cholesterol metabolism (98); and (iii) translocated gut-derived LPS from “leaky-gut” promoting hepatic inflammation lipid accumulation, hepatocyte damage and HSC activation (99). As the ligands for TLRs, intestine-derived bacterial products, saturated free fatty acids and HMGB1 stimulate TLR2, TLR4 and TLR9 on Kupffer cells and HSCs to produce CCL2 (51,67,100). CCL2 induces the recruitment of circulating CCR2+Ly6C+ monocytes into the liver (67). Recruited monocytes are more pro-inflammatory than resident Kupffer cells and produce pro-inflammatory cytokines, such as TNFα and IL-1β that contribute to the development of NASH and fibrosis. Inhibiting the recruitment of CCR2+Ly6C+ monocytes by using CCL2 or CCR2 inhibitors prevents the progression of NASH and fibrosis, and accelerates the regression of NASH-mediated fibrosis (67,101). The addition of fructose to high fat diets, which on their own only induce little hepatic inflammation and no fibrosis, promotes the development of fibrosis (102). Fructose has been implicated in the promotion of bacterial translocation, again pointing towards the relevance of the gut-liver axis in the interplay of inflammation and fibrosis (103). There is accumulating evidence that high fructose consumption in patients is associated with the development of insulin resistance, visceral adiposity and development of liver fibrosis (103).
Adipokines are also involved in NASH progression. Leptin promotes HSC activation through STAT3 and NADPH oxidase p47 phox, whereas adiponectin suppresses HSC activation via AMPK activation in NASH (104,105).
Besides weight loss and improved diets, there are no well-established treatments that directly target inflammation and fibrosis in NAFLD. Weight loss by dietary interventions or bariatric surgery leads to amelioration of steatosis but hepatic inflammation and fibrosis persisted in some studies (106). Vitamin E, the current first-line medical treatment, may decrease inflammation but not fibrosis after 96 weeks (107), but longer-term effects on inflammation and fibrosis still need to be confirmed.
Conclusion
Although inflammation is a key component and contributor to hepatic wound healing and fibrogenic responses, inflammatory signaling pathways have received relatively little attention as targets for chronic liver disease. This may in part be due to the complex regulatory mechanisms in which multiple mediators and receptors on various cell types affect multiple cellular responses – such as inflammation and cell survival – in an often cell-type specific manner. Future studies on inflammation and fibrosis should focus on defining “core pathways” (108). This may not only improve our understanding of this complex network, but also allow to focus therapeutic efforts on specific candidate molecules, such as key upstream mediators or transcriptional master regulators. In this context, the intimate link between gut and liver may represent a key and upstream mechanism in chronic liver disease and provide opportunities to target disease-promoting inflammation, e.g. using probiotic, non-absorbable antibiotics such as Rifaximin or gut microbiota transplantation. As alternative strategy, efforts could also focus on well-established profibrogenic pathways for which inhibitors already exist such as CCR5 and its ligand CCL5 (Maraviroc®). Targeting chronic inflammation in the context of fibrogenesis may also be beneficial for other inflammation-driven outcomes such as the development of HCC.
Acknowledgments
Financial support: ES was supported by NIH grants 5R01AA02017204, 5R01DK085252 and 5P42ES010337. RFS was supported by NIH grants 5R01AA020211, 1U01AA021912 and 5R01DK076920.
List of Abbreviations
α-SMA | α smooth muscle actin |
BAMBI | BMP and activin membrane bound inhibitor |
CCl4 | carbon tetrachloride |
DAMP | damage-associated molecular pattern |
ECM | extracellular matrix |
HSC | hepatic stellate cell |
ILC | innate lymphoid cells |
JNK | c-Jun-N-terminal kinase |
LSEC | liver sinusoidal endothelial cell |
LPS | lipopolysaccharide |
NAFLD | non-alcoholic fatty liver disease |
NF-κB | nuclear factor κB |
NK cells | natural killer cells |
PAMP | pathogen-associated molecular pattern |
TLR | toll-like receptor |
TGFβ | transforming growth factor β |
References
Full text links
Read article at publisher's site: https://doi.org/10.1002/hep.27332
Read article for free, from open access legal sources, via Unpaywall:
https://europepmc.org/articles/pmc4306641?pdf=render
Citations & impact
Impact metrics
Article citations
Evaluating the antifibrotic potential of naringenin, asiatic acid, and icariin using murine and human precision-cut liver slices.
Physiol Rep, 12(21):e16136, 01 Nov 2024
Cited by: 1 article | PMID: 39501714 | PMCID: PMC11538472
Purinergic signaling in liver disease: calcium signaling and induction of inflammation.
Purinergic Signal, 25 Sep 2024
Cited by: 0 articles | PMID: 39320433
Review
Highly Selective Artificial K<sup>+</sup> Transporters Reverse Liver Fibrosis In Vivo.
JACS Au, 4(10):3869-3883, 27 Aug 2024
Cited by: 0 articles | PMID: 39483224 | PMCID: PMC11522913
Dietary total antioxidant capacity in relation to disease severity and risk of mortality in cirrhosis; results from a cohort study.
Heliyon, 10(18):e37733, 12 Sep 2024
Cited by: 0 articles | PMID: 39315216 | PMCID: PMC11417536
The involvement of the Stat1/Nrf2 pathway in exacerbating Crizotinib-induced liver injury: implications for ferroptosis.
Cell Death Dis, 15(8):600, 19 Aug 2024
Cited by: 0 articles | PMID: 39160159 | PMCID: PMC11333746
Go to all (479) article citations
Similar Articles
To arrive at the top five similar articles we use a word-weighted algorithm to compare words from the Title and Abstract of each citation.
Cooperation of liver cells in health and disease.
Adv Anat Embryol Cell Biol, 161:III-XIII, 1-151, 01 Jan 2001
Cited by: 210 articles | PMID: 11729749
Review
Molecular mechanisms of hepatic fibrosis in non-alcoholic steatohepatitis.
Dig Dis, 28(1):229-235, 07 May 2010
Cited by: 36 articles | PMID: 20460917
Review
Hepatic inflammatory responses in liver fibrosis.
Nat Rev Gastroenterol Hepatol, 20(10):633-646, 03 Jul 2023
Cited by: 69 articles | PMID: 37400694
Review
Exosome-Mediated Intercellular Communication between Hepatitis C Virus-Infected Hepatocytes and Hepatic Stellate Cells.
J Virol, 91(6):e02225-16, 28 Feb 2017
Cited by: 111 articles | PMID: 28077652 | PMCID: PMC5331806
Funding
Funders who supported this work.
NIAAA NIH HHS (6)
Grant ID: 5R01AA02017204
Grant ID: 5R01AA020211
Grant ID: R01 AA020211
Grant ID: U01 AA021912
Grant ID: 1U01AA021912
Grant ID: R01 AA020172
NIDDK NIH HHS (4)
Grant ID: R01 DK076920
Grant ID: 5R01DK076920
Grant ID: 5R01DK085252
Grant ID: R01 DK085252
NIEHS NIH HHS (2)
Grant ID: P42 ES010337
Grant ID: 5P42ES010337
National Institutes of Health (3)
Grant ID: 1U01AA021912
Grant ID: 5R01DK076920
Grant ID: 5R01AA020211