Abstract
Free full text

Anticancer immunotherapy by CTLA-4 blockade relies on the gut microbiota
Abstract
Antibodies targeting CTLA-4 have been successfully used as cancer immunotherapy. We find that the antitumor effects of CTLA-4 blockade depend on distinct Bacteroides species. In mice and patients, T cell responses specific for B. thetaiotaomicron or B. fragilis were associated with the efficacy of CTLA-4 blockade. Tumors in antibiotic-treated or germ-free mice did not respond to CTLA blockade. This defect was overcome by gavage with B. fragilis, by immunization with B. fragilis polysaccharides, or by adoptive transfer of B. fragilis–specific T cells. Fecal microbial transplantation from humans to mice confirmed that treatment of melanoma patients with antibodies against CTLA-4 favored the outgrowth of B. fragilis with anticancer properties. This study reveals a key role for Bacteroidales in the immunostimulatory effects of CTLA-4 blockade.
Ipilimumab is a fully human monoclonal antibody (Ab) directed against CTLA-4, a major negative regulator of T cell activation (1), approved in 2011 for improving the overall survival of patients with metastatic melanoma (MM) (2). However, blockade of CTLA-4 by ipili-mumab often results in immune-related adverse events at sites that are exposed to commensal microorganisms, mostly the gut (3). Patients treated with ipilimumab develop Abs to components of the enteric flora (4). Therefore, given our previous findings for other cancer therapies (5), addressing the role of gut microbiota in the immunomodulatory effects of CTLA-4 blockade is crucial for the future development of immune checkpoint blockers in oncology.
We compared the relative therapeutic efficacy of the CTLA-4–specific 9D9 Ab against established MCA205 sarcomas in mice housed in specific pathogen–free (SPF) versus germ-free (GF) conditions. Tumor progression was controlled by Ab against CTLA-4 in SPF but not in GF mice (Fig. 1, A and B). Moreover, a combination of broad-spectrum antibiotics [ampicillin + colistin + streptomycin (ACS)] (Fig. 1C), as well as imipenem alone (but not colistin) (Fig. 1C), compromised the antitumor effects of CTLA-4–specific Ab. These results, which suggest that the gut microbiota is required for the anticancer effects of CTLA-4 blockade, were confirmed in the Ret melanoma and the MC38 colon cancer models (fig. S1, A and B). In addition, in GF or ACS-treated mice, activation of splenic effector CD4+ T cells and tumor-infiltrating lymphocytes (TILs) induced by Ab against CTLA-4 was significantly decreased (Fig. 1, D and E, and fig. S1, C to E).
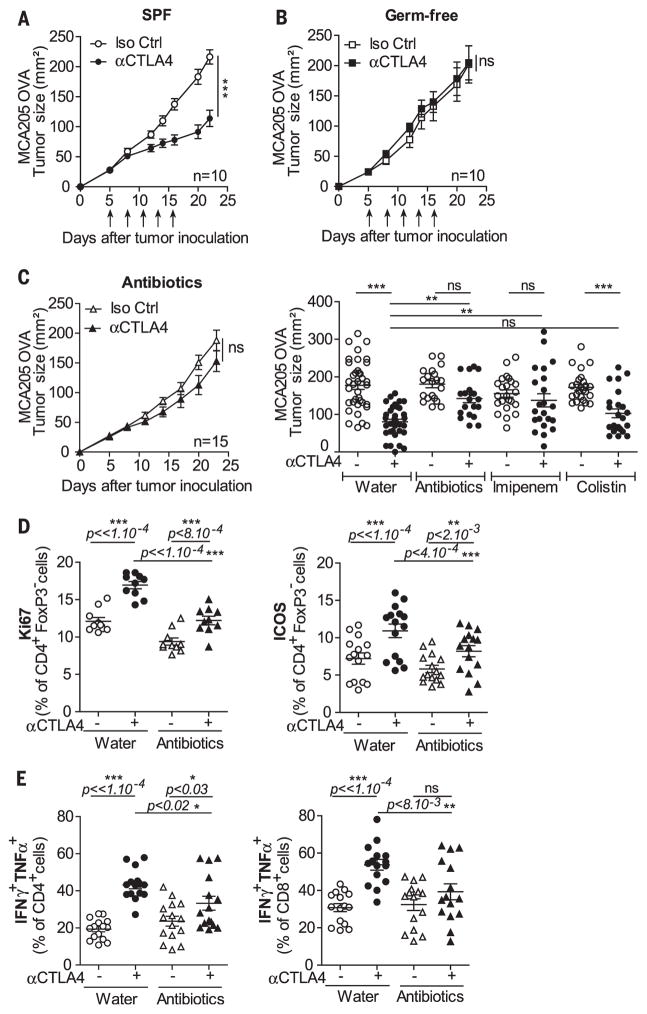
Tumor growth of MCA205 in SPF (A) or GF (B) mice treated with five injections (compare the arrows) of 9D9 or isotype control (Iso Ctrl) Ab. (C) Tumor growth as in (A) and (B) in the presence (left) of ACS or (right) of single-antibiotic regimen in >20 mice per group. Flow cytometric analyses of (D) Ki67 and ICOS expression and (E) TH1 cytokines on splenic CD4+Foxp3−Tcells (D) and TILs (E) 2 days after the third administration of 9D9 or Iso Ctrl Ab. Each dot represents one mouse in two to three independent experiments of five mice per group. P values corrected for interexperimental baseline variation between three individual experiments in (D). *P < 0.05; **P < 0.01; ***P < 0.001; ns, not significant.
We next addressed the impact of the gut micro-biota on the incidence and severity of intestinal lesions induced by CTLA-4 Ab treatment. A “subclinical colitis” dependent on the gut microbiota was observed at late time points (figs. S2 to S5). However, shortly (by 24 hours) after the first administration of CTLA-4 Ab, we observed increased cell death and proliferation of intestinal epithelial cells (IECs) residing in the ileum and colon, as shown by immunohistochemistry using Ab-cleaved caspase-3 and Ki67 Ab, respectively (Fig. 2A and fig. S6A). The CTLA-4 Ab–induced IEC proliferation was absent in RegIIIβ-deficient mice (fig. S6A). Concomitantly, the transcription levels of Il17a, Ifng, Ido1, type 1 Ifn-related gene products and Ctla4 (but not Il6), which indicate ongoing inflammatory processes, significantly increased by 24 hours in the distal ileum of CTLA-4 Ab–treated mice (fig. S6, B to D). Depletion of T cells, including intraepithelial lymphocytes (IELs) (by injection of Abs specific for CD4 and CD8), abolished the induction of IEC apoptosis by CTLA-4–specific Ab (Fig. 2A). When crypt-derived three-dimensional small intestinal enteroids (6) were exposed to Toll-like receptor (TLR) agonists (which act as microbial ligands in this assay) and subsequently admixed with IELs harvested from mice treated with Ab against CTLA-4 (but not isotype Ctl), IECs within the enteroids underwent apoptosis (Fig. 2B). Hence, CTLA-4 Ab compromises the homeostatic IEC-IEL equilibrium, favoring the apoptotic demise of IEC in the presence of microbial products.
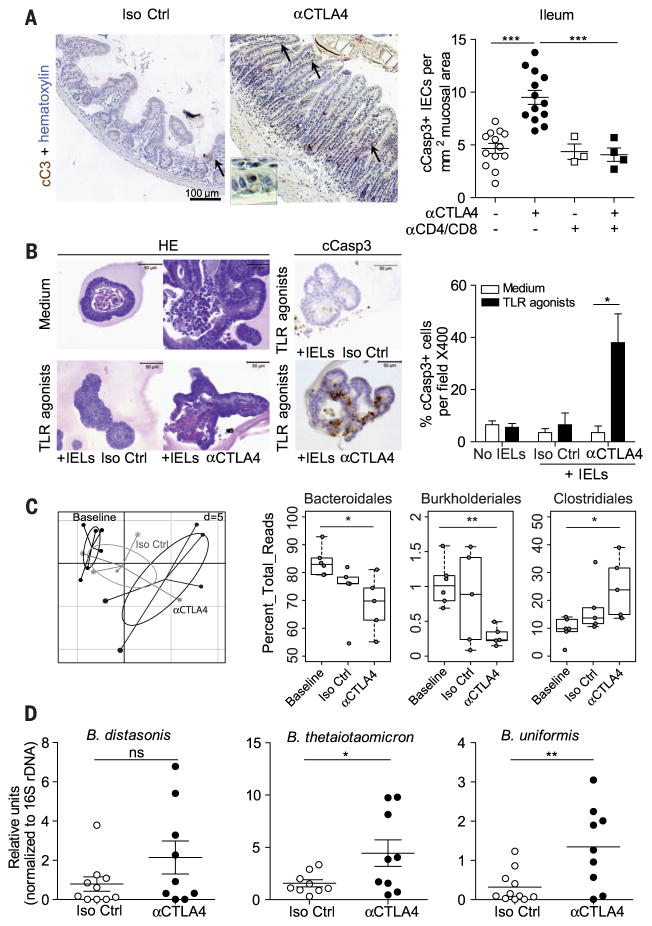
(A) (left) Representative micrograph pictures of distal ileum after staining with Ab-cleaved caspase 3 (cCasp3) Ab 24 hours after one injection of 9D9 (or Iso Ctrl) Ab in naïve mice with or without prior depletion of CD4+ and CD8+ T cells. Inset enlarged 18-fold. (Right) Concatanated data of two experiments. (B) (left) Representative micrographs of 3D enteroid cocultures stimulated (or not) with TLR agonists and incubated with IELs harvested from 9D9 (or Iso Ctrl) Ab–treated mice in hematoxylin and eosin (H&E), then (middle) stained with cCasp3-specific Ab. (Right) Data concatenated from two experiments counting the means ± SEM percentages of apoptotic cells to organoid in 20 organoids. (C) Sequencing of 16S rRNA gene amplicons of feces from tumor bearers before and 48 hours after one administration of 9D9 or Iso Ctrl Ab. (Left) Principal component analysis (PCA) on a relative abundance matrix of genus repartition highlighting the clustering between baseline, Iso Ctrl Ab–, and 9D9 Ab–treated animals after one injection (five to six mice per group). Ellipses are presented around the centroids of the resulting three clusters. The first two components explain 34.41% of total variance (Component 1: 20.04%; Component 2: 14.35%) (Monte-Carlo test with 1000 replicates, P = 0.0049). (C) (right) Means ± SEM of relative abundance for each three orders for five mice per group are shown. (D) QPCR analyses targeting three distinct Bacteroides spp. in ileal mucosae performed 24 to 48 hours after Ab introduction. Results are represented as 2−ΔΔCt × 103, normalized to 16S rDNA and to the basal time point (before treatment). Each dot represents one mouse in two gathered experiments. *P < 0.05; ** P < 0.01; ***P < 0.001; ns, not significant.
To explore whether this T cell–dependent IEC death could induce perturbations of the microbiota composition, we performed high-throughput pyrosequencing of 16S ribosomal RNA (rRNA) gene amplicons of feces. The principal component analysis indicated that a single injection of CTLA-4 Ab sufficed to significantly affect the microbiome at the genus level (Fig. 2C). CTLA-4 blockade induced a rapid underrepresentation of both Bacteroidales and Burkholderiales, with a relative increase of Clostridiales, infeces (Fig. 2C and table S1). Quantitative polymerase chain reaction (QPCR) analyses targeting the Bacteroides genus and species (spp.) in small intestine mucosa and feces contents showed a trend toward a decreased relative abundance of such bacteria in the feces, which contrasted with a relative enrichment in particular species [such as B. thetaiotaomicron (Bt) and B. uniformis] in the small intestine mucosa 24 to 48 hours after one CTLA-4 Ab injection (Fig. 2D and fig. S7). One of the most regulatory Bacteroides isolates, B. fragilis (Bf) (7–10), was detectable by PCR in colon mucosae but was not significantly increased with CTLA-4 Ab (fig. S7).
Next, to establish a cause-and-effect relationship between the dominance of distinct Bacteroides spp. in the small intestine and the anticancer efficacy of CTLA-4 blockade, we recolonized ACS-treated and GF mice with several bacterial species associated with CTLA-4 Ab–treated intestinal mucosae as well as Bf. ACS-treated mice orally fed with Bt, Bf, Burkholderia cepacia (Bc), or the combination of Bf and Bc, recovered the anticancer response to CTLA-4 Ab, contrasting with all the other isolates that failed to do so (table S2 and Fig. 3A). Similarly, oral feeding with Bf, which colonized the mucosal layer of GF mice (fig. S8) (11), induced T helper 1 (TH1) immune responses in the tumor-draining lymph nodes and promoted the maturation of intratumoral dendritic cells (DCs), which culminated in the restoration of the therapeutic response of GF tumor bearers to CTLA-4 Ab (Fig. 3B and fig. S9, A and B).
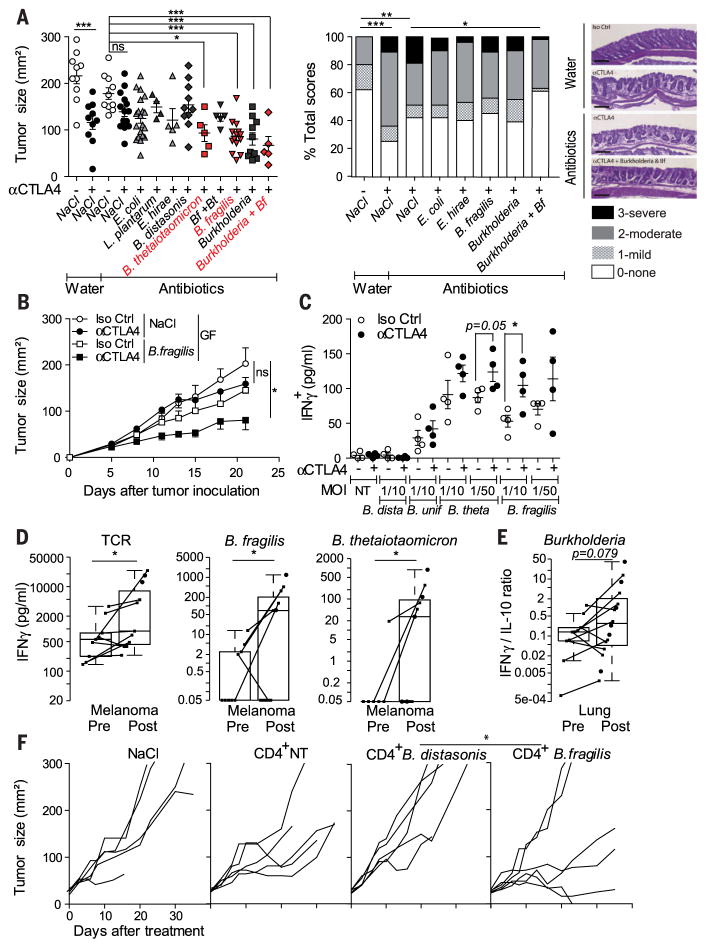
(A and B) Tumoricidal effects of Bf, Bt, and/or B. cepacia (Bc) administered by oral feeding of ACS-treated or GF mice (also refer to fig. S8A). (A) (left) Tumor sizes at day 15 after 9D9 or Iso Ctrl Ab treatment are depicted. Each dot represents one tumor, and graphs depict two to three experiments of five mice per group. (Middle) Histopathological score of colonic mucosae in ACS-treated tumor bearers receiving 9D9 Ab after oral gavage with various bacterial strains, assessed on H&E-stained colons monitoring microscopic lesions as described in materials and methods at day 20 after treatment in five animals per group on at least six independent areas. (Right) Representative micrographs are shown; scale bar, 100 μm. (B) Tumor-icidal effects of Bf in GF mice as indicated. (C to E) Recall responses of CD4+ T cells in mice and patients to various bacterial strains after CTLA-4 blockade. DCs loaded with bacteria of the indicated strain were incubated with CD4+ T cells, 2 days after three intraperitoneal (ip) CTLA-4 Ab in mice, and after at least two injections of ipilimumab (ipi) in patients. The graphs represent interferon-γ (IFN-γ) concentrations from coculture supernatants at 24 hours in mice (C) and 48 hours in MM patients (D). (E) IFN-γ/IL-10 ratios were monitored in DC–T cell cocultures of NSCLC patients at 48 hours. No cytokine release was observed in the absence of bacteria or Tcells (fig. S11 with HV). Each dot represents one patient or mouse. Paired analyses are represented by linking dots pre- and post-ipi. (F) Tcells harvested from spleens of mice exposed to CTLA-4 Ab and restimulated with Bf versus B. distasonis or bone marrow DCs alone (CD4+ NT) were infused intravenously in day 6 MCA205 tumor-bearing GF mice. A representative experiment containing five to six mice per group is shown. *P < 0.05; **P < 0.01; ***P < 0.001; ns, not significant.
We analyzed the dynamics of memory T cell responses directed against distinct bacterial species in mice and humans during CTLA-4 blockade. CD4+ T cells harvested from spleens of CTLA-4 Ab–treated mice (Fig. 3C) or from blood taken from individuals with MM or non–small cell lung carcinoma (NSCLC) patients after two administrations of ipilimumab (Fig. 3, D and E, and table S3) tended to recover a TH1 phenotype (figs. S10 and S11). The functional relevance of such T cell responses for the anticancer activity of CTLA-4 Ab was further demonstrated by the adoptive transfer of memory Bf-specific (but not B. distasonis-specific) TH1 cells into GF or ACS-treated tumor bearers (Fig. 3F and fig. S12), which partially restored the efficacy of the immune checkpoint blocker.
The microbiota-dependent immunostimulatory effects induced by CTLA-4 blockade depended on the mobilization of lamina propria CD11b+ DC that can process zwitterionic poly-saccharides (9) and then mount interleukin-12 (IL-12)–dependent cognate TH1 immune responses against Bf capsular polysaccharides (figs. S13 and S14). However, they did not appear to result from TLR2/TLR4-mediated innate signaling (7, 8) in the context of a compromised gut tolerance (figs. S15 to S19).
To address the clinical relevance of these findings, we analyzed the composition of the gut microbiome before and after treatment with ipilimumab in 25 individuals with MM (table S4). A clustering algorithm based on genus composition of the stools (12, 13) distinguished three clusters (Fig. 4A and table S5) with Al-loprevotella or Prevotella driving cluster A and distinct Bacteroides spp. driving clusters B and C (Fig. 4B). During ipilimumab therapy, the proportions of MM patients falling into cluster C increased, at the expense of those belonging to cluster B (Fig. 4B and fig. S20A). We next performed fecal microbial transplantation of feces harvested from different MM patients from each cluster, 2 weeks before tumor inoculation into GF mice that were subsequently treated with anti–CTLA-4 Ab. Tumors growing in mice that had been transplanted with feces from cluster C patients markedly responded to CTLA-4 blockade, contrasting with absent anticancer effects in mice transplanted with cluster B–related feces (Fig. 4C). QPCR analyses revealed that, although bacteria from the Bacteroidales order equally colonized the recipient murine intestine, stools from cluster C (but not A or B) individuals specifically facilitated the colonization of the immunogenic bacteria Bf and Bt (7–10, 14, 15) (Fig. 4D). Moreover, after CTLA-4 Ab therapy, only cluster C (not A or B) recipient mice had outgrowth of Bf (fig. S20B). Note that the fecal abundance of Bf (but not B. distasonis or B. uniformis) negatively correlated with tumor size after CTLA-4 blockade in cluster C–recipient mice (Fig. 4E and fig. S20C). Hence, ipilimumab can modify the abundance of immunogenic Bacteroides spp. in the gut, which in turn affects its anticancer efficacy.
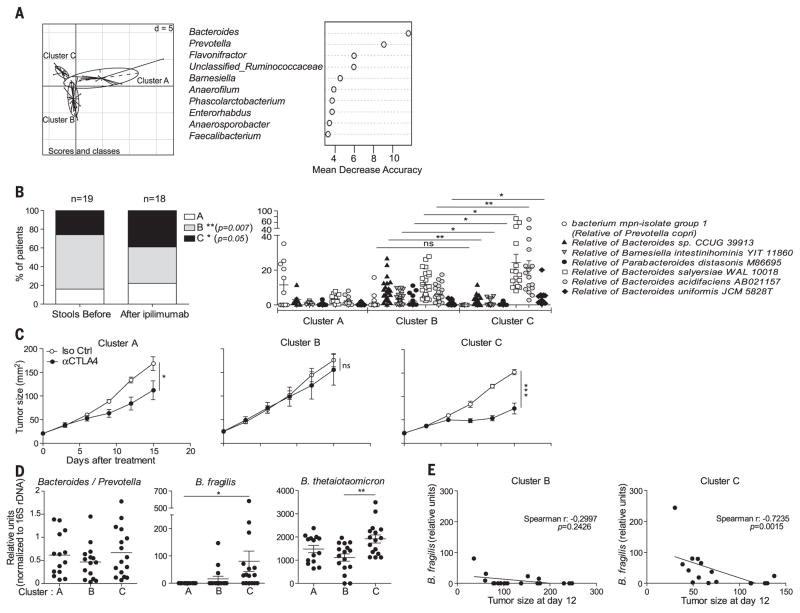
The k means clustering algorithm was applied on the basis of genus composition before and during ipilimumab treatment in 25 MM patients, validated using the Calinski-Harabasz index (14), and showed good performance in recovering three clusters before and after therapy (interclass PCA); (A) (left) (Monte-Carlo test, P = 0.000199). (A) (right) Random Forest analysis was applied to decipher the main genera responsible for this significant clustering. (B) (right) The relative abundance of main Bacteroides spp. significantly differed between clusters B and C. (B) (left) The proportions of patients falling into each cluster were analyzed in a nonpaired manner before versus after ipi injections regardless of the time point (fig. S20A). (C) Fecal microbial transplantation after introduction of ipilimumab from eight patients falling into each of the three clusters (stool selection for fecal microbial transplantation marked with an asterisk * in fig. S20A) into GF animals. One representative experiment out of three is shown with means ± SEM of tumor sizes depicted for each cluster over time. (D) QPCR analyses of feces DNA of the recipient before (2 weeks postcolonization) and 2 weeks after ipi, targeting Bacteroidales and Bacteroides spp. Results are represented as 2−ΔCt x 103, normalized to 16S rDNA. No significant difference in the relative abundance of Bf was detectable in the donors of cluster B versus C before colonization (not shown). (E) Spearman correlations between the amount of Bf in stools 15 days after treatment with 9D9 Ab and tumor sizes across cluster B- and C-recipient mice. *P < 0.05; **P < 0.01; ***P < 0.001.
Finally, intestinal reconstitution of ACS-treated animals with the combination of Bf and Bc did not increase but rather reduced histopathological signs of colitis induced by CTLA-4 blockade (Fig. 3A). This efficacy-toxicity uncoupling effect was not achieved with vancomycin, which could boost the antitumor effects of CTLA-4 blockade (presumably by inducing the overrepresentation of Bacteroidales at the expense of Clostridiales) but worsened the histopathological score (fig. S21). In support of this notion, Bf maintained its regulatory properties in the context of CTLA-4 blockade (fig. S22) (7).
Hence, the efficacy of CTLA-4 blockade is influenced by the microbiota composition (B. fragilis and/or B. thetaiotaomicron and Burkholderiales). The microbiota composition affects interleukin 12 (IL-12)–dependent TH1 immune responses, which facilitate tumor control in mice and patients while sparing intestinal integrity. In accord with previous findings (16), colitis (observed in the context of IL-10 deficiency and CTLA-4 blockade) (fig. S17) could even antagonize anti-cancer efficacy. Several factors may dictate why such commensals could be suitable “anticancer probiotics.” The geodistribution of Bf in the mucosal layer of the intestine (fig. S8) and its association with Burkholderiales—recognized through the pyrin–caspase-1 inflammasome (17) and synergizing with TLR2/TLR4 signaling pathways (fig. S15) —may account for the immunomodulatory effects of CTLA-4 Ab. Future investigations will determine whether a potential molecular mimicry between distinct commensals and/or pathobionts and tumor neoantigens could account for the toxicity and/or efficacy of immune checkpoint blockers. Prospective studies in MM and/or NSCLC may validate the relevance of the enterotypes described herein in the long-term efficacy of immune checkpoint blockers, with the aim of compensating cluster B–driven patients with live and immunogenic or recombinant Bacteroides spp. (18) or fecal microbial transplantation from cluster C–associated stools to improve their antitumor immune responses.
Acknowledgments
We are grateful to the staff of the animal facility of Gustave Roussy and Institut Pasteur. The data presented in this manuscript are tabulated in the main paper and in the supplementary materials. L.Z., M.V., and P.L. have filed patent application no. EP 14190167 that relates to the following: Methods and products for modulating microbiota composition for improving the efficacy of a cancer treatment with an immune checkpoint blocker. M.V. and J.M.P. were supported by La Ligue contre le cancer and ARC, respectively. L.Z. received a special prize from the Swiss Bridge Foundation and ISREC. G.K. and L.Z. were supported by the Ligue Nationale contre le Cancer (Equipes labelisées), Agence Nationale pour la Recherche (ANR AUTOPH, ANR Emergence), European Commission (ArtForce), European Research Council Advanced Investigator Grant (to G.K.), Fondation pour la Recherche Médicale (FRM), Institut National du Cancer (INCa), Fondation de France, Cancéropôle Ile-de-France, Fondation Bettencourt-Schueller, Swiss Bridge Foundation, the LabEx Immuno-Oncology, the Institut national du cancer (SIRIC) Stratified Oncology Cell DNA Repair and Tumor Immune Elimination (SOCRATE); the SIRIC Cancer Research and Personalized Medicine (CARPEM), and the Paris Alliance of Cancer Research Institutes (PACRI). S.M. was supported by NIH (R01 CA161879, as Principal Investigator). M.C. was supported by the Fondation pour la Recherche Médicale, the Fondation ARC pour la recherche sur le cancer, and Institut Nationale du Cancer. N.W. is a recipient of a Postdoctoral Fellowship from the Agence Nationale de la Recherche. A.S. was supported by BMSI YIG 2014. F.G. is supported by SIgN core funding. L.Z., M.C., and I.B.G. are all sponsored by Association pour la Recherche contre le Cancer (PGA120140200851). F.C. was supported by INCA-DGOS (GOLD H78008). N.C. was supported by INCA-DGOS (GOLD study; 2012-1-RT-14-IGR-01). L’Oreal awarded a prize to M.V. We are grateful to the staff of the animal facility of Gustave Roussy and Institut Pasteur. We thank P. Gonin, B. Ryffel, T. Angelique, N. Chanthapathet, H. Li, and S. Zuberogoitia for technical help. DNA sequence reads from this study have been submitted to the NCBI under the Bioproject IDPRJNA299112 and are available from the Sequence Read Archive (SRP Study accession SRP065109; run accession numbers SRR2758006, SRR2758031, SRR2758178, SRR2758179, SRR2758180, SRR2758181, SRR2768454, and SRR2768457.
Footnotes
www.sciencemag.org/content/350/6264/1079/suppl/DC1 Materials and Methods
References (19–35)
REFERENCES AND NOTES
Full text links
Read article at publisher's site: https://doi.org/10.1126/science.aad1329
Read article for free, from open access legal sources, via Unpaywall:
https://europepmc.org/articles/pmc4721659?pdf=render
Citations & impact
Impact metrics
Citations of article over time
Alternative metrics
Article citations
Neoadjuvant vidutolimod and nivolumab in high-risk resectable melanoma: A prospective phase II trial.
Cancer Cell, 42(11):1898-1918.e12, 31 Oct 2024
Cited by: 0 articles | PMID: 39486411
Long-term survival following anti-PD-(L)1 monotherapy in advanced urothelial cancer and an assessment of potential prognostic clinical factors: a multicentre observational study.
BJC Rep, 2(1):84, 23 Oct 2024
Cited by: 0 articles | PMID: 39516359 | PMCID: PMC11523962
Gut microbiome metabolites, molecular mimicry, and species-level variation drive long-term efficacy and adverse event outcomes in lung cancer survivors.
EBioMedicine, 109:105427, 30 Oct 2024
Cited by: 0 articles | PMID: 39471749 | PMCID: PMC11550776
Predictive biomarkers for immune checkpoint inhibitors therapy in lung cancer.
Hum Vaccin Immunother, 20(1):2406063, 16 Oct 2024
Cited by: 0 articles | PMID: 39415535 | PMCID: PMC11487980
Review Free full text in Europe PMC
Integrating clinical and genomic landscape analysis of perineural invasion identify ACTA1 as an oncogene for oral squamous cell carcinoma.
Front Cell Dev Biol, 12:1458879, 15 Oct 2024
Cited by: 0 articles | PMID: 39474352 | PMCID: PMC11519412
Go to all (1,704) article citations
Data
Data behind the article
This data has been text mined from the article, or deposited into data resources.
BioStudies: supplemental material and supporting data
Nucleotide Sequences (Showing 9 of 9)
- (1 citation) ENA - SRP065109
- (1 citation) ENA - SRR2758031
- (1 citation) ENA - SRR2758178
- (1 citation) ENA - SRR2758006
- (1 citation) ENA - SRR2768454
- (1 citation) ENA - SRR2758179
- (1 citation) ENA - SRR2758180
- (1 citation) ENA - SRR2768457
- (1 citation) ENA - SRR2758181
Show less
Similar Articles
To arrive at the top five similar articles we use a word-weighted algorithm to compare words from the Title and Abstract of each citation.
IMMUNOTHERAPY. Could microbial therapy boost cancer immunotherapy?
Science, 350(6264):1031-1032, 01 Nov 2015
Cited by: 20 articles | PMID: 26612936
Ctla-4 blockade plus adoptive T-cell transfer promotes optimal melanoma immunity in mice.
J Immunother, 38(2):54-61, 01 Feb 2015
Cited by: 24 articles | PMID: 25658614 | PMCID: PMC4321765
Tumour immunology: Intestinal bacteria are in command.
Nat Rev Immunol, 16(1):5, 14 Dec 2015
Cited by: 2 articles | PMID: 26655627
Immunotherapy for malignant melanoma.
Curr Stem Cell Res Ther, 7(3):217-228, 01 May 2012
Cited by: 11 articles | PMID: 22329580
Review