Abstract
Free full text

ESR1 mutations as a mechanism for acquired endocrine resistance in breast cancer
Abstract
Most breast cancers are estrogen receptor α (ER)-positive (+) and are treated with endocrine therapies targeting ER activity. Despite efforts, the mechanisms of the frequent clinical resistance to these therapies remain largely unknown. Several recent parallel studies unveiled gain-of-function recurrent ESR1 mutations in up to 20% of patients with metastatic ER+ disease who all received endocrine therapies, which for more cases included an aromatase inhibitor. These mutations, clustered in a hotspot within the ligand-binding domain (LBD), lead to ligand independent ER activity and tumor growth, partial resistance to tamoxifen and fulvestrant, and potentially increased metastatic capacity. Together, these findings suggest that the ESR1 LBD mutations account for acquired endocrine resistance in a substantial fraction of patients with metastatic disease. The absence of detectable ESR1 mutations in treatment-naïve disease and the correlation with the number of endocrine treatments indicate a clonal expansion of rare mutant clones, selected under the pressure of treatment. New technologies to detect low/ultra rare ESR1 mutations together with tissue and liquid biopsies are required to fully expose their clinical relevance in prognosis and treatment. Pre-clinical and clinical development of rationale-based novel therapeutic strategies to inhibit these mutants has the potential to substantially improve treatment outcomes.
Introduction
Breast Cancer is a heterogeneous disease with different clinical, histopathological, and molecular subtypes. Approximately 70% of breast cancers express the estrogen receptor α (ER). ER-positive (ER+) tumors are primarily of the luminal molecular subtype, which consists of the more differentiated indolent and endocrine (anti-ER) therapy sensitive luminal A and the more aggressive and relatively endocrine-resistant luminal B subtype.1–3. A large number of clinical and experimental studies established the fundamental role of ER and its estrogen ligands in normal mammary gland development and in the etiology and progression of breast cancer.4–7 ER, encoded by the ESR1 gene, is predominantly a nuclear protein that functions as a ligand-dependent transcription factor (this is known as ER’s genomic activity). It belongs to the nuclear receptor superfamily8, sharing the typical functional/structural configuration of this family’s members. ER consists of two transcriptional activation domains, the N-terminal ligand-independent activation function (AF)-1 and the C-terminal ligand-dependent AF-2 domains, a ligand-binding domain (LBD) also residing in the C-terminal region, and the DNA-binding and hinge domains, positioned in the core of the protein.6 Ligand binding to the receptor leads to the recruitment of coregulatory proteins including coactivators and corepressors and the binding of the complex to regulatory DNA sites containing the estrogen responsive element (ERE) motif9 to regulate transcription of genes important in various physiological processes, tumorigenesis, and tumor progression (classical activity). By tethering to other transcription factors, such as AP-1 and NFκ-B, at their specific sites, the ER-co-regulator complex can also regulate the transcriptional activity of these transcription factors and their target genes10, 11. This non-classical ER transcriptional regulation was shown to be augmented under ligand-independent conditions by growth factor stimulation.12 ER also has non-nuclear/non-genomic activities that are less well understood in which it has been proposed to interact with various tyrosine kinase receptors or other signaling molecules to rapidly activate their downstream signaling pathways.13
Due to the central role of ER in breast cancer, endocrine therapy inhibiting this pathway has become the mainstay of prevention and treatment of ER+ breast cancers in all stages of the disease.14–18 Indeed, ER status is a strong predictor of response to endocrine therapy.6 Endocrine therapies include (1) direct inhibition of ER by selective estrogen modulators (SERMs) with mixed agonistic/antagonistic activities, such as tamoxifen19, (2) selective ER degraders (SERDs) that are more potent anti-estrogens such as fulvestrant20, and (3) deprivation of the receptor’s ligand by blocking estrogen production with strategies such as aromatase inhibitors21 or ovarian suppression. In the early disease setting, endocrine treatments reduce the risk of recurrence by close to 50%, and in metastatic disease, these treatments remain the most effective treatment for ER+ disease. Despite the effectiveness of endocrine therapy, however, intrinsic (de novo) and acquired endocrine resistance continues to be an important clinical challenge.18 About 20% of patients who present with early disease will develop resistance manifested as recurrences either during or after adjuvant endocrine treatments. In the metastatic setting, endocrine treatments lead to initial tumor regression in only approximately 30% of patients, and inevitably, in almost all patients resistant disease develops resulting in disease progression and eventually death.
Significant research efforts focusing on understanding the complex biology of ER and the basis for endocrine resistance have suggested multiple mechanisms mediating this resistance. Importantly, each mechanism may operate as the sole mechanism or in concert with others.22 These mechanisms have been extensively reviewed elsewhere’22, 23 and herein are only briefly discussed. Loss of ER expression, which could account for endocrine resistance, has been observed in ~ 15–20% of metastatic breast cancer patients.24, 25 In most cases of endocrine resistance, however, ER continues to be expressed and active, thus enabling response to sequential multiple lines of endocrine treatments in the advanced metastatic setting 26, 27. This continued ER activity has been shown to be mediated by several mechanisms, including increased expression of the receptor itself28 or of its co-activators29. Bidirectional cross talk between ER and growth factor receptors or cellular/stress-related kinase pathways, at least partly by increasing phosphorylation levels and activity of ER and ER-co-regulators, has also emerged as a key mechanism to maintain ligand-independent activation of the pathway and to mediate resistance to various endocrine therapies 29–31. Hyperactivation of these kinase pathways and amplification/overexpression of cell cycle regulators or anti-apoptotic factors can also provide alternative proliferative and survival signaling independent of ER, thus bypassing tumor inhibition under attenuated ER conditions. Finally, a role for various components of the tumor microenviroment and additional host-related factors has also been recognized in endocrine resistance.22, 23 Despite the advances in the field, mechanisms of clinical resistance, mainly acquired resistance, remain largely unknown.
Large-scale genomics efforts, such as The Cancer Genome Atlas (TCGA), have led to new insights in the landscape and complexity of breast cancer genomics.32 Results from the TCGA show that while luminal primary breast cancers harbor the most diverse and recurrent mutations, the overall mutation rate in luminal cancers is lower when compared to basal-like and HER2-enriched subtypes.32 Of note, among the luminal tumors, only 8 genes were found to be mutated with a frequency above 5% (PIK3CA, TP53, MAP3K1, MAP2K4, GATA3, MLL3, CDH1, PTEN). Despite the central role of ER in luminal breast cancers, updated TCGA data from 962 breast cancer samples reports ESR1 mutations in only 0.5% of cases and ESR1 amplification in 2.6% of the cases.33 These results are consistent with previous studies from the 1990’s, which also showed that ESR1 mutations are a very rare event in primary breast cancers.34, 35 Thus, results from primary untreated tumors do not support the notion of ESR1 mutations as major drivers of carcinogenesis. In contrast, recent reports on metastatic ER+ breast cancers reveal a higher frequency of ESR1 mutations.36–40. Strikingly, the vast majority of these are point mutations clustered within hotspots of the ER LBD, rendering the receptor a ligand-independent constitutive activator. This review will summarize ESR1-related genomic alterations and their functional characteristics and potential in endocrine resistance, with a focus on these recently identified recurrent ESR1 LBD missense activating mutations, in metastatic endocrine-resistant ER+ breast cancer. This review will elaborate on the endocrine-resistant phenotype of these ESR1 LBD mutations, the underlying mechanisms, sensitive methods of detection, their prognostic and predictive implications, and new therapeutic strategies to circumvent resistance rendered by these mutations.
ESR1 genomic alterations in breast cancer
Acquired resistance to various targeted therapies has been attributed to acquired genetic aberrations that alter the target protein itself, additional components of its pathway, or other compensatory pathways to resist the inhibitory effect of the drug.41–44 Several types of ESR1 related genomic abnormalities have been described, including copy number changes (especially gene amplification), genomic rearrangements, and missense point mutations. Most of these alterations, in particular those largely reported in primary breast cancers, will be discussed only briefly. This will be followed by an in-depth discussion of the recent data from endocrine resistant metastatic tumors, which highlights the role of acquired genomic ESR1 point mutations that mainly modify the activity of the ER protein and its hormonal sensitivity.
ESR1 amplifications
Gene amplification is a mechanism by which certain cancer cells increase the expression of genes that provide a survival advantage. Overexpression of amplified genes has also been described as a mechanism for anti-tumor treatment failure and acquired resistance.43, 45, 46 Interestingly, in prostate cancer AR amplification was also reported in seven of 23 (30%) tumors that recurred during androgen deprivation therapy but in none of the matching specimens obtained prior to therapy.47 Despite advances in technologies to assess gene amplifications, the prevalence and the functional consequences of ESR1 amplifications remain controversial topics. Initial studies of ESR1 amplifications in early stage breast cancer reported a relatively high amplification rate of 20%.48, 49 In these studies ESR1 amplification correlated with high ER expression and favorable outcomes.48, 49 However, subsequent studies revealed a lower ESR1 amplification rate ranging between 1.5%–6% and an association with high-grade cancers and poor outcomes.50 The discrepancies between the studies may be attributed to the different technical assays, scoring systems, and the possibility that pre-mRNA artifacts may cause ESR1 FISH analysis artifacts. More recently, studies applying next-generation sequencing detected an ESR1 amplification rate of approximately 2% in both primary and metastatic tumors32, 40, suggesting that ESR1 amplification may not have a major role in the development of endocrine resistance. Nonetheless, emerging data from a small neoadjuvant study of endocrine treatment demonstrates the acquisition of a new amplicon containing ESR1 after 6 months of treatment.51 ESR1 gene amplification driving ligand-independent tumor growth was also reported by Li et al. in an ER+ patient-derived xenograft established from a patient with metastatic endocrine-refractory tumors.36 Thus, the clinical significance of ESR1 amplifications in early and recurrent diseases is still unclear.
ESR1 genomic rearrangements
Genomic rearrangements result in transcriptional dysregulation or the generation of fusion gene transcripts/proteins of truncated two distinct genes. The list of clinically significant recurrent gene fusions in solid tumors including breast cancer is continually growing52 and references therein, though no clear evidence for functional rearrangements involving the ESR1 gene have been reported in previous years. Recently, however, a new report identified and characterized a recurrent genomic rearrangement event involving the ESR1 gene and its chromosomal neighbor CCDC170.53 The identified ESR1-CCDC170 fusions exemplify a gain-of-function alteration, in which N-terminally truncated CCDC170 forms are fused to and utilize the ESR1 constitutive promoter to increase their expression. The truncated CCDC170 forms are associated with increased motility, tumorigenicity, and endocrine resistance properties. A second intriguing report recently described a YAP1/ESR1 translocation event in a metastatic ER+ breast cancer, where the C-terminal end of the ESR1 protein product is truncated and fused to the C-terminus of YAP1.36 This mutant leads to ligand-independent tumor growth and complete resistance to fulvestrant, since the ESR1 LBD is absent from this chimeric protein. Ongoing studies are expected to soon provide the more complete spectrum of the various infrequent and more recurrent ESR1 fusions and their role in pathology and endocrine resistance of primary and metastatic ER+ breast cancer.
Point missense mutations
Recurrent gain-of-function missense mutations have been reported in multiple oncogenes or key signaling molecules involved in tumor development and progression.32 As mentioned above, these reports did not include significant recurrent mutations in the ESR1 gene. In contrast, an earlier report by Fuqua et al. described a highly frequent gain-of-function ESR1 mutation in a third of hyperplastic lesions and one half of ER+ primary breast cancer.54 This somatic mutation, K303R (lysine to arginine)54, resides within the ER hinge domain and was recently comprehensively reviewed.55 In ER+ breast cancer cell lines, overexpression of this mutation was reported to confer estrogen hypersensitivity54 and a decrease in endocrine treatment sensitivity when engaged in crosstalk with growth factor signaling pathways.56–58 Additionally, the same group reported an association between the K303R mutation and poor outcome in untreated breast cancer patients.59 However, the presence and frequency of this mutation remains an open question since only two additional studies could detect this mutation albeit at a lower frequency (5%–10%)60, 61, while several other studies could not identify this mutation in primary or metastatic tumors including the large TCGA dataset.32, 36–40, 62–64 Future studies should resolve these controversies, which may be related to the sequencing methods in the different studies to date.55 As mentioned above, the following parts of this review will focus on the recently discovered recurrent ESR1 LBD mutations in metastatic ER+ breast cancer.
ESR1 LBD activating mutations
In metastatic endocrine-resistant disease
Over the past 18 months five studies published the identification of point mutations in the LBD of ESR1 in metastatic ER+ breast cancers (Figure 1).36–40 The first study36 detected ESR1 mutations in 3 of 7 (43%) patient-derived xenografts that were established from metastatic tumor samples from patients with metastatic ER+ disease. The ESR1 mutations were detected by whole genome sequencing and were confirmed in the originating tumors as well. In the second published study, ER+ metastatic tumor samples from two patient cohorts were sequenced by targeted next-generation sequencing, including 36 patients that had progressive disease after at least 3 months of hormonal therapy and 44 patients enrolled in the BOLERO-2 clinical trial.37 The BOLERO-2 trial studied patients who had disease refractory to an aromatase inhibitor, defined as disease recurrence within 12 months of completion of adjuvant treatment with an AI or with progressive disease during treatment for metastatic disease.65 In both cohorts a total of 14 (17.5%) ESR1 LBD mutations were detected. The analysis of patients from the BOLERO-2 trial also included the sequencing of the primary tumors of 183 of the patients enrolled in the trial. ESR1 mutations were detected in 3% of the primary cases. Because of the small number of cases with ESR1 mutations, a definitve association between ESR1 mutations and response to treatment in the BOLERO-2 study could not be confirmed, but the presence of the mutation did not seem to affect response to exemestane, presumably due to the fact that all the patients in the study had already progressed on a previous aromatase inhibitor treatment.37 In two additional small studies by Robinson et al.38 and Merenbakh-Lamin et al.39, 11 and 13 metastatic ER+ tumor samples were sequenced detecting ESR1 LBD mutations in 6 (54%) and 5 (38%) patients, respectively. Finally, in the second larger study40, 76 metastatic ER+ tumor samples were sequenced to high coverage using next-generation targeted sequencing, and 11 ESR1 LBD mutations were detected. Conversely, in the ER-negative control samples there were no LBD mutations. In this study, among 58 primary tumors that were sequenced, no ESR1 LBD mutations were detected. These primary tumors included two samples that had matched metastatic samples harboring the LBD ESR1 mutations40.
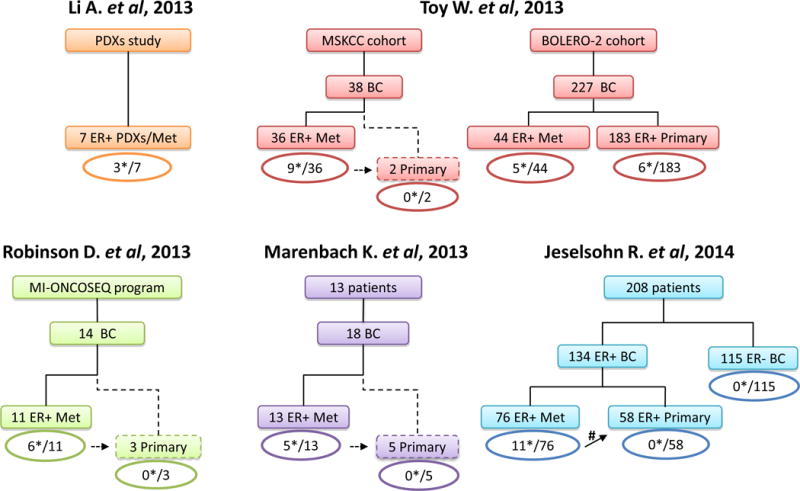
Numbers of sequenced metastatic and primary breast cancer samples and reported ESR1 LBD point mutations (*) are indicated. The dashed lines indicate primary tumors that were sequenced subsequently to the detection of the ESR1 LBD mutation in their matched metastatic specimens. The ER+ BC cohort from Jeselsohn’s study included upfront 37 matched ER+ metastatic and primary sample pairs, of which in 2 of these pairs (#) ESR1 LBD mutations were detected in the metastatic but not in the primary tumors. Abbreviations: BC, breast cancer; BOLERO-2, Breast cancer trials of OraL EveROlimus-2; Met, metastatic samples; MI-ONCOSEQ, the MIchigan ONCOlogy SEQuencing program; MSKCC, Memorial Sloan Kettering Cancer Center; PDXs, Patient-derived xenografts.
Together, in all studies a total of 187 metastatic ER+ tumors from patients who received at least 1 line of endocrine treatment were sequenced and 39 ESR1 LBD mutations were found.36–40 The prevalence of the mutations in the different studies ranges between 14%–54%. This wide range may be due to the small patient cohorts in some of the studies and to the differences in the patient population and their treatment course. Indeed, the study of Jeselsohn et al. showed a correlation between the prevalence of the hotspot LBD mutations and the number of lines of endocrine treatment.40 The mutations were detected in tumor samples obtained from different organ sites, including lymph nodes, skin, lung, and liver36–40, suggesting that these mutations do not display specific oragonotropism. The majority of the LBD mutations in these studies were missense mutations in residues Y537 and D538 (Figure 2). The most common mutations included Y537S (11), Y537N (6) and Y537C (4), and D538G (13) missense mutations, a finding that is consistent with an early single report that documented the Y537N in one metastatic breast cancer.66 Additional mutations found in this hotspot region were L536Q, L536R, P535H, and V534E. In addition, 3 double mutants were detected within the same tumor (Y537N/D538G, S436P/D538G, S436P/Y537N), though it is not clear if these mutations reside within the same ER molecule, if they co-occur within the same cell, or if they represent different cell subpopulations within a heterogeneous tumor. Another LBD mutation outside of the hotspot is E380Q, found in three tumor samples36, 37, 40, while no additional recurrent alterations in other domains of the ER protein were observed.
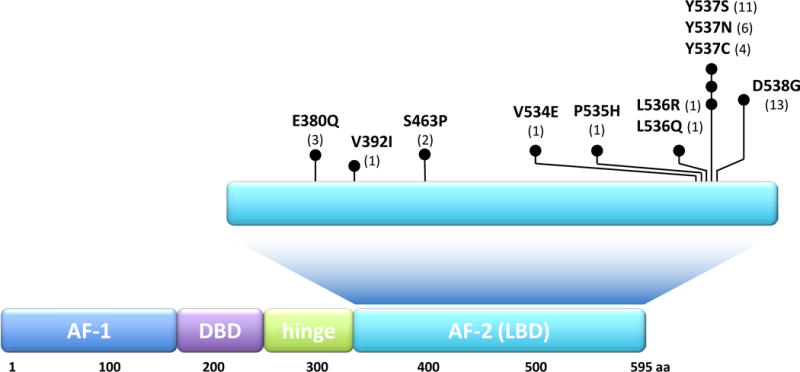
Black circles indicate each mutation at the specific protein position; numbers in parentheses indicate the total number of samples reported to harbor the specific indicated mutations. Abbreviations: AF-1, activation function-1; DBD, DNA-binding domain; AF-2, activation function-2.
In treatment-naïve early disease
To better understand genetic mechanisms of ER+ metastatic disease, Toy et al.37 compared the prevalence of the mutations found in the metastatic samples of their first cohort of relapsed tumors with the prevalence of these mutations in primary luminal tumors reported by TCGA. The prevalence of the mutations of three of the most commonly altered genes (TP53, PIK3CA, GATA3) was comparable. In contrast, the prevalence of mutations in ESR1, as well as RPTOR and ERBB3, was significantly higher in the metastatic tumors. Applying a similar approach, the study of Jeselsohn et al. compared the mutation prevalence in the metastatic versus primary ER+ breast cancers.40 Of the most frequently mutated genes, all but ESR1 mutations exhibited similar prevalence across primary and metastatic tumors. Collectively, these comparisons suggest a role for the ESR1 LBD mutations in the development of metastatic disease and endocrine resistance. Noteworthy and somewhat contradictory to this notion, are the findings of Toy et al. showing that the ESR1 LBD mutations were detected in 6/183 (3%) of the primary tumors from the BOLERO-2 clinical trial. This may be due to the fact that this was a selective cohort of primary tumors from patients who eventually all developed relatively early disease recurrence with a less than 5 year median time to relapse. Of note, 1 of the 6 mutations found by Toy et al.37 in the primary tumors (E380Q), is also present in 1/932 primary tumors in the updated TCGA dataset.33
Resistance and cellular phenotypes
Consistent with earlier studies66–68, all five recent papers36–40 showed that the ESR1 LBD mutations within the hotspot region, particularly the point mutations in residues Y537 and D538, are gain-of-function mutations with ligand independent activity and enhanced ligand-stimulated activity (Figure 3). Overexpression of the mutants induced ligand independent and enhanced ligand-dependent expression of exogenous and endogenous genes, such as PR, GREB1, and TTF1, driven by the classical estrogen responsive element (ERE).36–40 Fulvestrant and tamoxifen were both able to inhibit mutant ER activity, although substantially higher doses were required, suggesting partial but not complete resistance to these hormonal agents.37–40 Microarray gene expression analysis of ER+ breast cancer cells overexpressing the ER mutants or wild type (WT) ER as controls under hormone-depleted conditions showed increased expression of known estrogen-responsive genes in mutant cells and also identified an additional set of genes uniquely expressed by mutant cells that are not known as classic ER-target genes.37 Hence, the transcriptional program mediated by mutant ER is ligand-independent and may not be limited to the known estrogen-induced genes but rather includes a new set of direct or indirect targets (Figure 3).
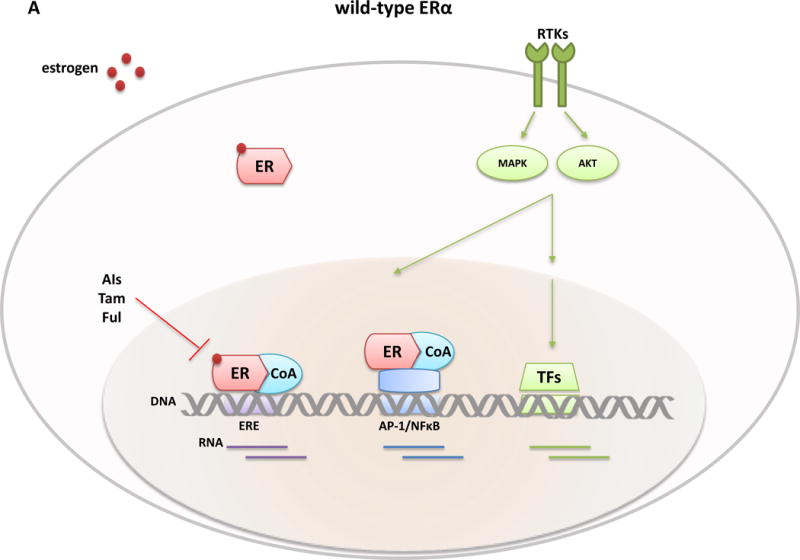
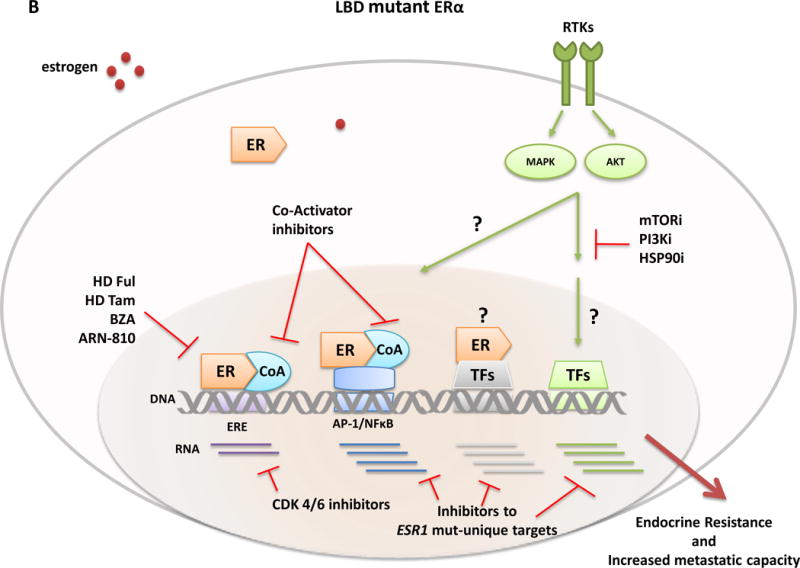
Classical ER transcription activity is mediated by ER binding to DNA at the consensus ERE sites, while in the non-classical ER activity mode, the receptor is tethered to other transcriptional factors such as AP-1 or NFkB and regulates gene expression from their sites. The transcriptional activity of ER and other TFs is further modulated by RTKs and other signaling pathway-induced kinases (e.g., MAPK and AKT) that phosphorylate ER, its coregulatory proteins (e.g., CoA), and other components of the transcriptional machinery to control the overall transcriptional program needed for tumor development and progression. Differential expression profiles between wild-type and mutant ERs suggest an augmented non-classical genomic activity of ER mutants, which may enhance RTK signaling and the metastatic capacity of the tumor cells. Breast cancer cells with wild-type ER (A) are largely sensitive to standard endocrine therapies (aromatase inhibitors, tamoxifen, fulvestrant). In contrast, ESR1 LBD mutant cells (B) display an endocrine resistant phenotype and current findings suggest the need of alternative therapeutic strategies such as higher doses of fulvestrant or tamoxifen, more potent or mutant specific SERMS or SERDs (bazedoxifene and ARN-810, respectively), agents targeting ER co-activators and ER gene products such as, cyclinD1 blockade by CDK4/6 inhibitors, or other signaling pathways and kinase inhibitors (e.g., mTOR, PI3Kinase, and HSP90 inhibitors) alone or in combination with ER inhibitors. Abbreviation: AIs, aromatase inhibitors; BZD, bazedoxifen; CoA, co-activator; ER, estrogen receptor; ERE, estrogen responsive element; Ful, fulvestrant; i, inhibitor; LBD, ligand-binding domain; RTKs, tyrosine kinase receptors; Tam, tamoxifen; TFs, transcription factors.
The ligand-independent activity of the ER mutants also conferred an in vitro cell proliferation advantage for cells grown in estrogen-deprived conditions or in the presence of fulvestrant and tamoxifen.36, 39, 40 Increased ligand-independent tumor growth was also observed in vivo using xenograft models of ER+ breast cancer cells overexpressing the mutant ER.37 Patient-derived xenografts (PDXs) from a human metastatic breast tumor harboring one of the ESR1 mutation residing within the LBD hotspot region also exhibited ligand-independent growth.36 However, the growth pattern of the PDX harboring the recurrent E380Q LBD ER mutant was ligand-dependent36. Of note, this mutation was also detected in primary and metastatic breast tumors by other groups33, 37, 40 and resides outside of the LBD hotspot cluster region. The E380Q mutant may therefore improve tumor fitness through a different mechanism than the constitutive activity observed with the Y537 and D538 mutants.
Finally, breast cancer cells overexpressing the D538 LBD mutation exhibited an increased migration capacity.39 This finding may suggest an increased invasion property, which could contribute to increased metastatic progression and could partly explain why the mutations were detected mainly in metastatic lesions. In keeping with this hypothesis, it should be noted that some of the genes uniquely expressed in the cells overexpressing the ER-LBD mutants have been indicated to have roles in related migratory processes (e.g., MMP1169, WNT1170, and RET.71, 72 Additional studies are needed to confirm the potential role of these molecular effectors for this migratory phenotype and to fully characterize the “invasiveness” properties of the ER mutants.
Mechanistic insights to resistance
In the absence of ligand, WT ER is protected from proteosomal degradation by interaction with the HSP90 chaperone complex.6 Binding of estrogen to ER induces a conformational change, known as the agonistic active conformation.73, 74 This conformational change consists of ER dissociation from the chaperone complex, dimerization, and the formation of a complex with co-regulatory proteins, and depends on the agonistic activity of its C-terminal helix 12. Previous structural crystal studies of the WT ER and the Y537S mutant reveal the stabilization of the mutant ER helix 12 in the agonistic conformation similar to the estrogen-bound WT ER. These seminal studies provide the molecular mechanism underlying the ligand-independent activity of this mutant ER.37, 39, 73 More recently, molecular dynamics simulations of the novel D538G mutation and the Y537S mutation37, 39 bound to co-activators confirm that the mutations lead to increased stability of helix 12 in the agonist conformation. Additional evidence for the stabilization of the LBD-ER mutant in the agonist conformation is provided by protein immunoprecipitation studies revealing increased co-precipitation of ER LBD mutant with the SRC-3 co-activator compared to WT-ER in hormone depleted conditions.37 In addition, a ligand-independent interaction between D538G ER and SRC-1 co-activator was observed.39 As a more global approach, a nuclear receptor-co-regulator interaction microarray assay containing 154 nuclear receptor cofactor motifs showed mutant ER ligand-independent recruitment of a large number of co-regulators.75 Taken together, the constitutive coactivator recruitment in the absence of ligand, due to the constitutive agonistic position of Helix 12 in the mutants, provides a strong molecular mechanism for the hormone-independent activity of the mutant receptors.
The ER LBD mutants display relative resistance to tamoxifen and fulvestrant.36–38, 40 Studies to directly evaluate the affinity of tamoxifen and fulvestrant to all these ER LBD mutants have not been conducted. However, it is highly plausible that the changes in the dynamics between the agonist and antagonist conformation of the mutant LBD in the absence of ligand is the underlying mechanism of this resistance. An indication of this possibility is provided by the finding of increased co-regulator binding of mutant ER after tamoxifen treatment when compared to WT ER.75 Additionally, the abrogated degradation of mutant ER by fulvestrant offers mechanistic proof for the relative resistance to fulvestrant.40
Tumor evolution and clinical relevance
Breast cancers, like many other types of cancers, display an extensive degree of intratumor heterogeneity with genetic, epigenetic, and histopathological phenotypic diversity among the tumor epithelial cells.76, 77 The interplay between genetic instability and the resultant continuous accumulation of somatic mutations in tumor cells on one hand, and, on the other hand, the need for these cells to resist and adapt to constraints from the tumor microenvironment or other exogenous factors provides the basis for a dynamic clonal evolution in cancers, which largely operates according to the Darwinian evolutionary model.78, 79 Multiple clinical and preclinical studies, which strongly support this phenomenon, also provide compelling evidence to suggest that acquired resistance in many cases reflects the outgrowth and expansion of pre-existing resistant rare clones in response to the selective pressure of targeted therapies.78, 79 Given the ligand-independent endocrine resistant property of the ESR1 LBD mutants, and their detection almost exclusively in acquired endocrine-resistant metastatic breast cancer, but not in primary treatment-naïve tumors, suggests a clonal selection of rare resistant clones only under the pressure of endocrine treatment (Figure 4). Interestingly, the allele frequencies of these mutations reported by Toy et al.37 ranged between 0.06 to 0.84 in the metastatic tumor samples, indicating continued tumor heterogeneity which may be essential even after clonal selection.
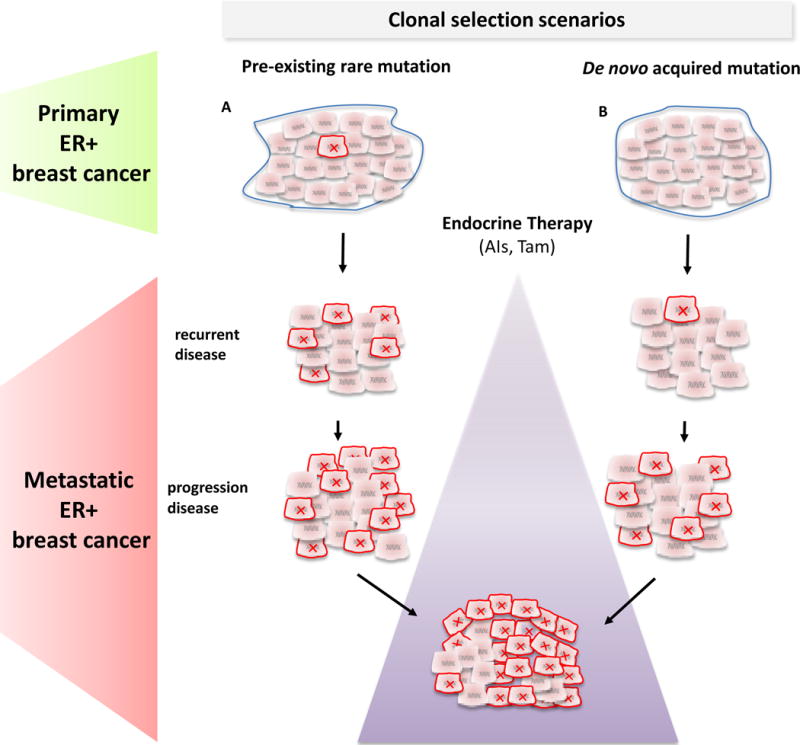
Two possible scenarios for the origin of the ESR1 LBD mutations detected endocrine resistant metastatic breast cancer are proposed: Pre-existing rare ESR1 LBD mutant subclones (red x) in treatment-naïve primary tumors (A) or acquired de novo ESR1 mutations during therapy (B). In both cases, a selection and an expansion of the ESR1 mutant clones occurs over multiple lines of endocrine therapy (violet triangle) leading to an acquired endocrine-resistant phenotype.
The rare mutant ESR1 LBD cells may arise from a rare and undetectable pre-existing clone in the primary treatment-naïve tumors, or may have been acquired de novo during the course of the treatment (Figure 4). In any case, the rare mutant clones, possessing a selective advantage over other endocrine-sensitive clones, expand to become a more predominant clone over the course of successive lines of endocrine therapy. Nonetheless, in both scenarios, early as possible detection of their appearance is required for prognosis and clinical decision-making (see below). The distinction between these two scenarios has several clinical implications: First, the timing of the appearance of these mutations will dictate when patients should be screened. Screening in early stage disease will likely require more sensitive techniques. Second, if the mutations can be detected in primary tumors it will be important to reveal their prognostic and predictive significance and their effect on the interval to disease recurrence (early versus late recurrences) by retrospective and prospective studies. Alternatively, if the ESR1 mutations are acquired during treatment, it would be important to monitor for their appearance as will be discussed below.
In this context, it is important to reiterate that most patients in the five studies received an AI prior to the detection of the mutation36–40, which raises the question whether the ESR1 LBD mutations possess a superior advantage under estrogen deprivation treatments (AIs) compared to other endocrine treatment modalities. Whether the observation that the frequency of ESR1 LBD mutations correlates with the increase in the number of endocrine treatment lines is related merely to the longer pressure time enabling expansion of the mutated clones or should be attributed to the necessity for an acquired second hit, remains an unanswered question. An additional open question is whether and how more effective endocrine therapies or longer duration of adjuvant endocrine therapy, by increasing the selection pressure and its time-span, will affect the time of the emergence and the frequency of ESR1 LBD mutations.
Emerging detection platforms
Massively parallel next-generation sequencing techniques allow the quantification of the proportion of cells harboring a given mutation in cancer, but their inherent error rate of up to 1%80 limits the sensitivity to detect infrequent mutations. Even technologies using targeted sequencing that allow “deeper” sequencing are still limited by this error rate. Improved technologies that can detect mutation presence in a very small fraction of cells, which may be the case for ESR1 mutations in primary disease or at the time their first emergence in advanced disease, are essential to discern the full complexity and heterogeneity of breast cancers and to identify potentially actionable rare mutations. Low cellularity, which is commonly found in many tumor specimens, further challenges the detection of these rare mutations. A few emerging promising new technologies that dramatically decrease the sequencing error rate have the potential to become useful tools to overcome these limitations. These technologies include the Safe-Sequencing System (Safe-SeqS)81, which tags each template molecule to allow confident identification of rare variants, and the ultra sensitive Duplex sequencing82, which independently tags and sequences each of the two strands of a DNA duplex. With these improved technologies, the potential background error rate is less than one error per 109 nucleotides sequenced, thus allowing the detection of ultra-rare mutations within heterogeneous cellular populations.82 Digital droplet PCR83, 84, which emulsifies the DNA in thousands to millions of droplets to encapsulate single DNA molecules, designed with mutant-specific primers, is a second emerging platform to allow the detection and quantification of rare mutations. The current incorporation of these technologies to clinical research and eventually their transition to the clinical setting will improve our capacity to detect rare ESR1 and other genetic alterations over the course of disease and will allow the development of clinical studies to reveal their full clinical utility.
As mentioned above, the pattern of the tumor evolution of the ESR1 mutations that was unveiled in the recent publications emphasizes the importance of studying these and other genetic alterations over the natural course of breast cancer. Testing for these mutations should take place at the time of initial diagnosis and at the time of each recurrence and disease progression, ideally by obtaining a tissue specimen. However, obtaining tissue biopsies from metastatic disease is limited, and is even more challenging in cases of multiple synchronous metastatic lesions. Therefore, alternative approaches to detect the mutations in “liquid biopsies”, including circulating tumor cells or cell-free DNA from blood samples85, along with additional ultrasensitive methods86, are in development. Implementation of the more sensitive assays to detect rare mutations along with systematic collection of patient samples from all disease stages will enable the identification of patients for stratification in prospective clinical trials that have yet to be performed studying the clinical significance of the ESR1 mutations in disease progression, resistance to standard endocrine treatments, and response to emerging therapeutic strategies.
Novel therapeutic strategies
The preclinical studies show relative resistance of the activating mutations to tamoxifen and fulvestrant but effective inhibition with higher doses of these agents36–40 (Figure 3). Together with the results of the structural studies, the current findings suggest that higher doses of fulvestrant or tamoxifen or more potent or mutant specific SERMS or SERDs may benefit patients whose tumors harbor an ER LBD mutant. Given the results of the CONFIRM study that showed increased survival with higher doses of fulvestrant87, it is important to test if high dose fulvestrant can inhibit these ER mutations. Another consideration will be to test the efficacy of high dose tamoxifen, specifically in metastatic patients with ER LBD mutations, particularly since an acceptable toxicity profile was reported in several small studies.88, 89 New generation SERMs and SERDs, such as bazedoxifene90 and ARN-81091, are currently extensively being studied in the preclinical setting for their efficacy in the inhibition of the ER-LBD mutants and are expected to guide the clinical development of these agents. Indeed, an open phase I/IIa clinical trial with ARN-810 for post-menopausal patients with locally advanced or metastatic ER+ breast cancer plans to include an expansion arm of 30 patients confirmed to have ESR1 LBD mutations (clinicaltrials.gov: NCT 01823835).
Since the mutant ER is still highly dependent on the recruitment of co-activators, new agents targeting ER co-activators, such as the recently described SRC-3 small molecule inhibitors92, 93, may offer another approach to target the ER mutants and should be tested alone and in combination with available and new ER antagonists (Figure 3).
The constitutive ligand-independent activity of the ER mutants suggests targeting classical ER gene products (such as cyclinD1 blockade by CDK4/6 inhibitors94), but also gene products unique to the ESR1 mutants alone or in combination with an ER antagonist (Figure 3). Furthermore, better understanding of the unique mutant ER downstream effectors will likely reveal other innovative targets. In addition, pathways that crosstalk with and activate ER transcriptional activity, such as growth factors and their downstream signaling pathways, are also of interest, especially considering the elevated phosphorylation levels of the serine-118 residue in the ligand-independent AF-1 domain of the mutant ER.37 Finally, as has been suggested for other commonly mutated oncogenes95–97, it will be important to study the mutant ER protein as a target for immunotherapy.
Since the ER mutants are associated with other genetic and epigenetic alterations, testing of these novel therapeutic agents and their combinations in preclinical models that include different authentic genetic backgrounds is relevant and highlights the potential of patient-derived xenografts36 and ex-vivo cultures of CTCs from patients with ER+ metastatic disease98. Indeed, a recent report successfully utilized ex-vivo cultures of CTCs harboring ER mutants to tailor different therapeutic combinations. In this study, which included two mutant ER models, multiple pathway inhibitors alone or in combination with ER inhibitors were queried. While the results were highly complex and varied among the two available models, the study identified the efficacy of mTOR, PI3Kinase, and HSP90 inhibitors, especially in combination with fulvestrant.98
Conclusions
The recent discovery of recurrent ESR1 mutations within the LBD in ER+ endocrine resistant metastatic breast cancer across multiple publications36–40 sheds new light on the mechanisms of clinical endocrine resistance. The mutations were found in about 15–20% of patients, the majority of whom received an AI, with increasing frequencies detected in patients who received multiple endocrine treatments. Functional and mechanistic studies demonstrated that the mutations confer a ligand-independent agonistic conformation of the LBD resulting in ligand independent and enhanced ER transcriptional activity and tumor growth, and enhanced migratory properties. The ESR1 mutants displayed relative resistance to tamoxifen and fulvestrant, which could be reversed with high doses of these agents. These mutations are seldom detected in primary treatment-naïve tumors, suggesting either the clonal selection of very rare resistant clones or their later acquisition under the pressure of endocrine treatments as a new and major mechanism of resistance.
Collectively, these exciting findings introduce new clinical challenges and opportunities (Figure 5). Innovative platforms allowing the detection of rare subclonal mutations are a key component to elucidating the accurate prevalence and the clinical predictive and prognostic consequences of ESR1 LBD mutations in early and advanced stages of ER+ breast cancer. In addition to the analysis of retrospectively collected tissues from large clinical trials, the systematic collections of tumor tissues or liquid biopsies throughout the course of disease including at initial diagnosis and at disease recurrence and progression, together with these emerging technologies, will eventually enable the stratification of patients in clinical trials based on the ESR1 mutations. In addition, detection of such mutants in liquid biopsies might offer an approach to early detection of resistant occult micrometastatic residual disease during or after adjuvant endocrine treatment, though the clinical significance of such a finding would remain to be established. These types of studies will eventually disclose the overall contribution of ESR1 genetic aberrations including amplifications and rearrangements to endocrine resistance.
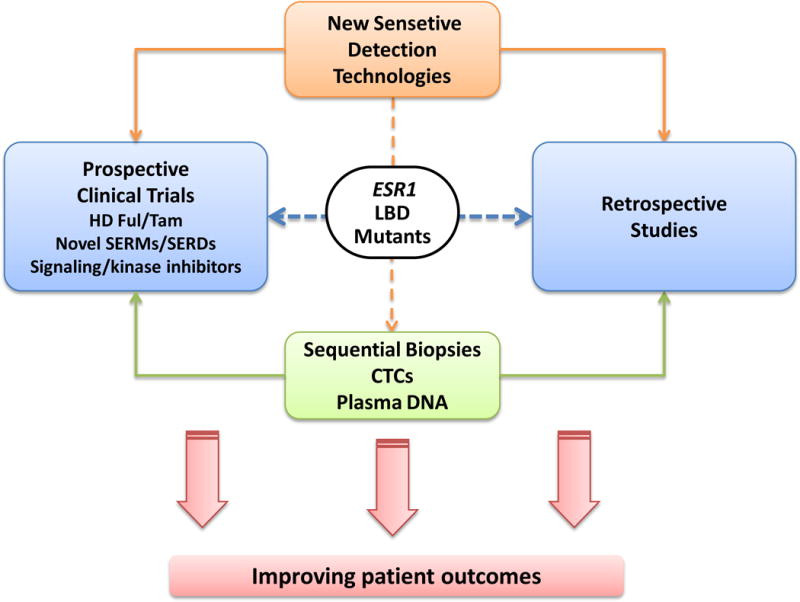
Future clinical directions in understanding the clinical significance of the ESR1 LBD mutations and novel therapeutics.
Based on the available studies, new therapeutic strategies should be first studied in pre-clinical models that accurately recapitulate the genomic complexity of patient tumors. Putative strategies include high dose fulvestrant or tamoxifen, inhibitors of ER co-activator proteins, and novel SERDs and SERMs as single agents or in combination with novel agents inhibiting targets that either activate or are activated by the WT and mutant ER-signaling axis. If successfully translated to the clinical setting, our abilities to better detect the mutations, predict resistance, and effectively treat tumors harboring these mutations will have a substantial impact on patient outcome. Implementation of these strategies in early stage disease holds the promise to improve cure rates.
Acknowledgments
Grant Support
This work was supported in part by NCI grants P50 CA58183, P50CA186784-01 (R.S.) (SPORE) and P30 CA125123 (R.S.), and P50CA168504 (SPORE) Career Development Award (R.J), as well as by Susan G. Komen for the Cure Promise Grant PG12221410 (R.S.), the Breast Cancer Research Foundation (R.S.), Cancer Prevention and Research Institute of Texas (CPRIT) program RP140102-Baylor College of Medicine Comprehensive Cancer Training Program (C.DA), and the Claudia Adams Barr Awar (R.J)
Footnotes
Competing Interests
RS has received research funding from AstraZenca and GlaxoSmithKline. MB has received a commercial research grant and is a consultant/advisory board member for Novartis Pharmaceuticals.
Authors Contributions
All authors contributed substantially to the discussion, content, writing, and review/editing of the manuscript.References
Full text links
Read article at publisher's site: https://doi.org/10.1038/nrclinonc.2015.117
Read article for free, from open access legal sources, via Unpaywall:
https://europepmc.org/articles/pmc4911210?pdf=render
Citations & impact
Impact metrics
Citations of article over time
Alternative metrics
Smart citations by scite.ai
Explore citation contexts and check if this article has been
supported or disputed.
https://scite.ai/reports/10.1038/nrclinonc.2015.117
Article citations
Genomic and clinical landscape of metastatic hormone receptors-positive breast cancers carrying ESR1 alterations.
ESMO Open, 9(10):103731, 01 Oct 2024
Cited by: 0 articles | PMID: 39357123 | PMCID: PMC11480226
Improving the Prognostic and Predictive Value of Circulating Tumor Cell Enumeration: Is Longitudinal Monitoring the Answer?
Int J Mol Sci, 25(19):10612, 02 Oct 2024
Cited by: 0 articles | PMID: 39408942 | PMCID: PMC11476589
Review Free full text in Europe PMC
Therapeutic Opportunities for Biomarkers in Metastatic Spine Tumors.
Cancers (Basel), 16(18):3152, 14 Sep 2024
Cited by: 0 articles | PMID: 39335124 | PMCID: PMC11430692
Review Free full text in Europe PMC
Estrogen-Receptor Loss and ESR1 Mutation in Estrogen-Receptor-Positive Metastatic Breast Cancer and the Effect on Overall Survival.
Cancers (Basel), 16(17):3025, 30 Aug 2024
Cited by: 0 articles | PMID: 39272884 | PMCID: PMC11394627
Exploring pathogenic SNPs and estrogen receptor alpha interactions in breast cancer: An in silico approach.
Heliyon, 10(17):e37297, 31 Aug 2024
Cited by: 0 articles | PMID: 39286133 | PMCID: PMC11403482
Go to all (300) article citations
Data
Similar Articles
To arrive at the top five similar articles we use a word-weighted algorithm to compare words from the Title and Abstract of each citation.
The association between type of endocrine therapy and development of estrogen receptor-1 mutation(s) in patients with hormone-sensitive advanced breast cancer: A systematic review and meta-analysis of randomized and non-randomized trials.
Biochim Biophys Acta Rev Cancer, 1872(2):188315, 21 Oct 2019
Cited by: 25 articles | PMID: 31647985
Review
The prevalence of estrogen receptor-1 mutation in advanced breast cancer: The estrogen receptor one study (EROS1).
Cancer Treat Res Commun, 19:100123, 21 Feb 2019
Cited by: 11 articles | PMID: 30826563
Recurrent hyperactive ESR1 fusion proteins in endocrine therapy-resistant breast cancer.
Ann Oncol, 29(4):872-880, 01 Apr 2018
Cited by: 62 articles | PMID: 29360925 | PMCID: PMC5913625
Activating ESR1 mutations in hormone-resistant metastatic breast cancer.
Nat Genet, 45(12):1446-1451, 03 Nov 2013
Cited by: 641 articles | PMID: 24185510 | PMCID: PMC4009946
Funding
Funders who supported this work.
NCI NIH HHS (8)
Grant ID: P50 CA058183
Grant ID: P50 CA58183
Grant ID: P50 CA186784
Grant ID: P50 CA168504
Grant ID: K08 CA191058
Grant ID: P30 CA125123
Grant ID: P50 CA186784-01
Grant ID: P50 CA168508