Abstract
Background
The safety and effectiveness of a continuous, day-and-night automated glycaemic control system using insulin and glucagon has not been shown in a free-living, home-use setting. We aimed to assess whether bihormonal bionic pancreas initialised only with body mass can safely reduce mean glycaemia and hypoglycaemia in adults with type 1 diabetes who were living at home and participating in their normal daily routines without restrictions on diet or physical activity.Methods
We did a random-order crossover study in volunteers at least 18 years old who had type 1 diabetes and lived within a 30 min drive of four sites in the USA. Participants were randomly assigned (1:1) in blocks of two using sequentially numbered sealed envelopes to glycaemic regulation with a bihormonal bionic pancreas or usual care (conventional or sensor-augmented insulin pump therapy) first, followed by the opposite intervention. Both study periods were 11 days in length, during which time participants continued all normal activities, including athletics and driving. The bionic pancreas was initialised with only the participant's body mass. Autonomously adaptive dosing algorithms used data from a continuous glucose monitor to control subcutaneous delivery of insulin and glucagon. The coprimary outcomes were the mean glucose concentration and time with continuous glucose monitoring (CGM) glucose concentration less than 3·3 mmol/L, analysed over days 2-11 in participants who completed both periods of the study. This trial is registered with ClinicalTrials.gov, number NCT02092220.Findings
We randomly assigned 43 participants between May 6, 2014, and July 3, 2015, 39 of whom completed the study: 20 who were assigned to bionic pancreas first and 19 who were assigned to the comparator first. The mean CGM glucose concentration was 7·8 mmol/L (SD 0·6) in the bionic pancreas period versus 9·0 mmol/L (1·6) in the comparator period (difference 1·1 mmol/L, 95% CI 0·7-1·6; p<0·0001), and the mean time with CGM glucose concentration less than 3·3 mmol/L was 0·6% (0·6) in the bionic pancreas period versus 1·9% (1·7) in the comparator period (difference 1·3%, 95% CI 0·8-1·8; p<0·0001). The mean nausea score on the Visual Analogue Scale (score 0-10) was greater during the bionic pancreas period (0·52 [SD 0·83]) than in the comparator period (0·05 [0·17]; difference 0·47, 95% CI 0·21-0·73; p=0·0024). Body mass and laboratory parameters did not differ between periods. There were no serious or unexpected adverse events in the bionic pancreas period of the study.Interpretation
Relative to conventional and sensor-augmented insulin pump therapy, the bihormonal bionic pancreas, initialised only with participant weight, was able to achieve superior glycaemic regulation without the need for carbohydrate counting. Larger and longer studies are needed to establish the long-term benefits and risks of automated glycaemic management with a bihormonal bionic pancreas.Funding
National Institute of Diabetes and Digestive and Kidney Diseases of the National Institutes of Health, and National Center for Advancing Translational Sciences.Free full text

Home use of a bihormonal bionic pancreas versus insulin pump therapy in adults with type 1 diabetes: a multicentre randomised crossover trial
Summary
Background
The safety and effectiveness of a continuous, day-and-night automated glycaemic control system using insulin and glucagon has not been shown in a free-living, home-use setting. We aimed to assess whether bihormonal bionic pancreas initialised only with body mass can safely reduce mean glycaemia and hypoglycaemia in adults with type 1 diabetes who were living at home and participating in their normal daily routines without restrictions on diet or physical activity.
Methods
We did a random-order crossover study in volunteers at least 18 years old who had type 1 diabetes and lived within a 30 min drive of four sites in the USA. Participants were randomly assigned (1:1) in blocks of two using sequentially numbered sealed envelopes to glycaemic regulation with a bihormonal bionic pancreas or usual care (conventional or sensor-augmented insulin pump therapy) first, followed by the opposite intervention. Both study periods were 11 days in length, during which time participants continued all normal activities, including athletics and driving. The bionic pancreas was initialised with only the participant’s body mass. Autonomously adaptive dosing algorithms used data from a continuous glucose monitor to control subcutaneous delivery of insulin and glucagon. The coprimary outcomes were the mean glucose concentration and time with continuous glucose monitoring (CGM) glucose concentration less than 3·3 mmol/L, analysed over days 2–11 in participants who completed both periods of the study. This trial is registered with ClinicalTrials.gov, number NCT02092220.
Findings
We randomly assigned 43 participants between May 6, 2014, and July 3, 2015, 39 of whom completed the study: 20 who were assigned to bionic pancreas first and 19 who were assigned to the comparator first. The mean CGM glucose concentration was 7·8 mmol/L (SD 0·6) in the bionic pancreas period versus 9·0 mmol/L (1·6) in the comparator period (difference 1·1 mmol/L, 95% CI 0·7–1·6; p<0·0001), and the mean time with CGM glucose concentration less than 3·3 mmol/L was 0·6% (0·6) in the bionic pancreas period versus 1·9% (1·7) in the comparator period (difference 1·3%, 95% CI 0·8–1·8; p<0·0001). The mean nausea score on the Visual Analogue Scale (score 0–10) was greater during the bionic pancreas period (0·52 [SD 0·83]) than in the comparator period (0·05 [0·17]; difference 0·47, 95% CI 0·21–0·73; p=0·0024). Body mass and laboratory parameters did not differ between periods. There were no serious or unexpected adverse events in the bionic pancreas period of the study.
Interpretation
Relative to conventional and sensor-augmented insulin pump therapy, the bihormonal bionic pancreas, initialised only with participant weight, was able to achieve superior glycaemic regulation without the need for carbohydrate counting. Larger and longer studies are needed to establish the long-term benefits and risks of automated glycaemic management with a bihormonal bionic pancreas.
Funding
National Institute of Diabetes and Digestive and Kidney Diseases of the National Institutes of Health, and National Center for Advancing Translational Sciences.
Introduction
People with type 1 diabetes are at risk of life-threatening acute and chronic complications. Maintaining mean blood glucose concentrations near the non-diabetic range prevents complications of type 1 diabetes and reduces mortality.1–3 However, most people with type 1 diabetes are not able to maintain mean blood glucose in this range,4–6 and intensifying treatment to achieve therapeutic goals increases the risk of both symptomatic and life-threatening hypoglycaemia.7–11 Current treatments need painstaking effort by patients to count carbohydrates, closely monitor blood glucose, and make dosing decisions for insulin, a drug with a narrow therapeutic range and a low margin for error. An unmet need exists for better methods to manage glycaemia. We have investigated a strategy to automate glycaemic management with a bihormonal bionic pancreas that uses continuous glucose monitoring (CGM) and mathematical algorithms to automatically administer both insulin and glucagon.12–19
We have previously done day-and-night 5-day studies with a bihormonal bionic pancreas in outpatient settings.17, 18 Findings from these studies showed significantly reduced mean glucose concentrations and hypoglycaemia or the need to treat hypoglycaemia versus usual care in participants aged 6–76 years.17, 18 The bionic pancreas was initialised only with the participant body mass; there were no restrictions on diet or exercise, and meal announcements did not require carbohydrate counting. However, in the adult study,17 participants stayed in a hotel at night and had in-person monitoring by study staff during the day, and the studies in children17, 18 took place in a diabetes camp setting.
Several randomised, controlled day-and-night outpatient studies have tested various insulin-only artificial pancreas systems for between 40 h and 12 weeks.20, 25 These studies were done in a supervised hotel-based setting,20 a diabetes camp,21 or in a unsupervised free-living home setting.23–25 In all of these studies, the artificial pancreas systems were initialised with information from each participant’s insulin regimen and required carbohydrate counting. Participants in two of the three home studies used the insulin-only artificial pancreas for 7 days and were restricted in their use of the artificial pancreas during vigorous exercise.23, 24 However, in the longest of these studies,25 lasting 12 weeks, participants had no restrictions on exercise after the first 2 weeks. Findings from this study showed increased time in the target blood glucose concentration range, decreased mean glucose concentration, and reduced hypoglycaemia exposure compared with sensor-augmented pump (SAP) treatment.25 In two studies26, 27 of a bihormonal artificial pancreas administering insulin and glucagon, participants were allowed to go home after an inpatient period of optimisation: for 2 days in one26 and for 3 days in the other.27 Diet and exercise were not restricted and no meal announcements were required. However, the system was initialised with a personalised insulin sensitivity factor that could be adjusted daily during the experiment by the investigators, and neither study showed improvements in the primary outcomes—time in target blood glucose concentration range26 or median glucose concentration27— relative to conventional insulin pump (CIP) treatment.
We did a multicentre, randomised, crossover trial to assess whether the bihormonal bionic pancreas initialised only with body mass can safely reduce both mean glycaemia and hypoglycaemia in adult volunteers with type 1 diabetes who were following their usual routines in a home-use setting without restrictions on diet or physical activity.
Methods
Study design and participants
We did this random-order crossover study at four sites in the USA: Massachusetts General Hospital (Boston, MA), University of Massachusetts Medical School (Worcester, MA), Stanford University (Palo Alto, CA), and the University of North Carolina at Chapel Hill (Chapel Hill, NC). All participants were at least 18 years old, had type 1 diabetes for at least 1 year, and were on insulin pump treatment for at least 6 months. They were required to work or study at one of the participating campuses and to live within a 30 min drive-time radius. Each participant had a designated contact at least 18 years old who lived with them and was willing to receive calls from the study staff regarding the participant’s welfare. The appendix (p 3) lists other eligibility and exclusion criteria.
The protocol was approved by the institutional review boards at each centre. All participants and designated contacts gave written informed consent before participation in the study.
Randomisation and masking
Participants completed two 11-day study periods in random order, during which they used the bihormonal bionic pancreas (intervention period) or their own insulin pump (and CGM if they used one) according to their usual practice (comparator period). Participants were randomly assigned (1:1) in blocks of two using sequentially numbered sealed envelopes produced by MAH and opened in the same order by KLM when participants were deemed eligible. Allocation assignment was not masked. Further details of masking are provided in the Procedures section. There was a washout period of 3 days or 10 days between study periods, depending on local scheduling constraints. Each study period included an in-person start-up visit on day 1, a shutdown visit on day 11, and two brief check-in calls on days 4 and 8.
Procedures
As described previously,17, 18 the bihormonal bionic pancreas used for automatic administration of insulin and glucagon consisted of an iPhone 4S (Apple, Cupertino, CA, USA) connected to a G4 Platinum CGM (Dexcom, San Diego, CA, USA). The insulin and glucagon control algorithms used were identical to the ones used in our previous study.18 These algorithms are similar to those used in our other previous studies,14–17 with the additional refinement of incorporating into the insulin dosing algorithm a feedback effect of the total glucagon dosing. The control algorithm and user interface ran as an app on the iPhone. The user interface allowed optional meal announcements, designated as “breakfast”, “lunch”, or “dinner”, of size “typical”, “more than typical”, “less than typical”, or “a small bite”. Participants were suggested, but not required, to use this feature immediately before eating the main meals of the day, but not snacks. Use of this feature triggered a partial meal-priming bolus that automatically adapted towards 75% of the 4 h prandial insulin need for that meal size and type. The first meal-priming bolus for each meal type, before adaptation, was based on body mass. For a “typical” meal the first weight-based meal-priming bolus was 0·05 U/kg. The meal-priming bolus was a third of that for a “typical” meal for a “small bite”, two-thirds for “less than typical”, and one and a third for “more than typical”. The bionic pancreas used two t:slim infusion pumps (Tandem Diabetes Care, San Diego, CA, USA) to deliver subcutaneous doses of insulin and glucagon up to every 5 min through insulin infusion sets (appendix p 15).
System initialisation was based solely on the participant’s body mass, without information about the usual insulin regimen. The bionic pancreas adapted dosing to each participant’s needs automatically. When CGM data were not available, the bionic pancreas invoked automatic basal insulin dosing on the basis of mean basal dosing delivered at corresponding times on previous days. Automatic correction doses of insulin or glucagon were delivered in response to manually entered blood glucose measurements that the system requested once per hour when CGM data were unavailable. No insulin was given other than by the bionic pancreas. Patients were asked not to use acetaminophen because of interference with CGM performance.28, 29
During the comparator period, all participants carried the CGM and iPhone portion of the bionic pancreas set to a mode in which CGM data were collected but not displayed to the participant, allowing collection of CGM data and remote telemetric monitoring for hypoglycaemia without altering their usual method of glycaemic management. During the comparator period, participants were also encouraged to use their own unmasked CGM, in addition to the masked study CGM, if that was part of their usual approach to glycaemic management (ie, if their usual care treatment was SAP). In both study periods, whenever CGM glucose concentrations were less than 2·8 mmol/L for more than 15 min, study staff were alerted and telephoned the participant to alert them. Remote telemetric monitoring was otherwise masked to glucose concentrations and to the actions of the bionic pancreas. In the bionic pancreas period, telemetric monitoring alerted study staff when the CGM or pumps were disconnected for 15 min, prompting a phone call to the participant for troubleshooting. Participants were instructed to send a text message to the monitor before driving, which changed the threshold for alerts from less than 2·8 mmol/L to less than 3·3 mmol/L during the trip. Participants calibrated the CGM twice daily using the StatStrip Xpress meter (Nova Biomedical, Waltham, MA, USA) and used this meter for all plasma glucose measurements in both study periods. Participants were asked to take capillary plasma glucose measurements at least four times daily.
During both treatment periods, participants used their usual insulin analogue and changed insulin infusion sets every 2 days. During the bionic pancreas period, participants reconstituted glucagon (Eli Lilly, Indianapolis, IN, USA) and used a new pump cartridge, tubing, and infusion set daily.
No restrictions were placed on diet or exercise. Participants were asked to limit alcohol intake to no more than two drinks in 1 h or four drinks in 1 day. Participants completed a questionnaire each evening via an automated email regarding the previous 24 h, including symptomatic hypoglycaemia, carbohydrate treatments for hypoglycaemia, and any nausea (intensity on a 10 cm Visual Analogue Scale, answers could be 0 or whole integers 1–10; appendix p 4).
At the start and end of each treatment period, body mass and blood pressure were measured and blood was taken for a chemistry panel and a complete blood count (appendix p 3).
Outcomes
The coprimary outcomes were the mean CGM glucose concentration and the time with CGM glucose concentration less than 3·3 mmol/L during days 2–11 in each period. Although the bionic pancreas continues to adapt beyond day 1, glycaemic data collected on days 2–11 are expected to be more representative of long-term system performance than day 1 data.16–18
Secondary outcomes included the percentage of time CGM glucose concentrations were in clinically relevant ranges (<2·8 mmol/L, <3·9 mmol/L, 3·9–10·0 mmol/L, and >10·0 mmol/L); number of symptomatic hypoglycaemic events; number of carbohydrate interventions for hypoglycaemia; and amount of carbohydrates taken for treatment of hypoglycaemia (appendix p 4). All glycaemic outcome measures derived from CGM data were calculated for the full 24 h period and for the night-time period (2300 h to 0700 h). The number of participants with a mean CGM glucose concentration 8·6 mmol/L or lower (secondary endpoint) was also calculated because this is the estimated mean glucose concentration that corresponds to a glycated haemoglobin (HbA1c) of 7·0%.30–32 Other secondary outcomes were insulin and glucagon total daily dose.
Safety outcomes were nausea reported on the daily surveys, change in body mass, and change in laboratory measurements from the beginning to end of each study period (days 1–11).
Statistical analysis
In our previous outpatient study in adults,17 the difference between the mean CGM glucose concentration in the bionic pancreas and usual care periods was 1·4 mmol/L (bionic pancreas mean 7·4 mmol/L [ SD 0·7] vs usual care 8·8 mmol/L [1·7]) and the difference in time with CGM glucose concentration less than 3·3 mmol/L was 2·2% (1·5% [1·7] vs 3·7% [3·3], SD of the difference 3·7%). The sample size needed to detect the same difference in time with CGM glucose concentration less than 3·3 mmol/L with a power of 95% and a significance level of 5% using a one-sided paired t test assuming a SD of 4% to be conservative was 38 participants. This number of participants was predicted to provide more than 99% power to detect the difference in mean CGM found in the previous study17 using the same statistical method. This sample size was larger than that needed to detect the previously observed difference in mean CGM glucose. We aimed to have ten participants complete the study at each of the four sites and we replaced participants who withdrew.
All data from participants who completed both periods of the study, including data from periods when the bionic pancreas was not in use, were included in the analyses. Data were analysed using Excel for Mac 2011 (version 14.7.0) and SAS (version 9.4). We used the paired t test to compare study periods, and report two-sided p values. For the two coprimary outcomes, we used a repeated measures model allowing within-patient random effects fitted using the generalised estimating equations method to analyse the period and treatment main effects, and used the interaction between them to test for a carryover effect. We also used multivariable models to analyse the effect, in separate analyses (one variable per analysis) of study site, participant age, duration of diabetes, baseline HbA1c concentration, insulin total daily dose, and CGM use in the comparator period. For analysis of laboratory measures, we used the paired sample Student’s t test to establish whether the difference from the beginning to the end of each period was different between the two periods. Additionally, we used the Wilcoxon signed rank test to assess whether there was a difference in the total number of laboratory measurements that changed from normal to abnormal during the two periods. We used McNemar’s test to assess whether the difference in number of participants achieving a mean CGM glucose 8·6 mmol/L or lower in the two periods was statistically significant. The Pearson correlation coefficient was calculated to establish whether there was a correlation between the number of meal announcements and the effect on mean CGM glucose and time with CGM glucose concentration less than 3·3 mmol/L. p values were not adjusted for multiple comparisons.
This trial is registered with ClinicalTrials.gov, number NCT02092220.
Role of the funding source
The funders of the study had no role in study design, data collection, data analysis, data interpretation, writing of the report, or the decision to submit this manuscript for publication. FHE-K, ERD, and SJR had full access to all the data in the study, and FHE-K, DMH, BAB, JBB, ERD, and SJR had final responsibility for the decision to submit for publication.
Results
We randomly assigned 43 participants between May 6, 2014, and July 3, 2015 (figure 1). Among 21 participants randomly assigned to the bionic pancreas period first, one had an episode of severe hypoglycaemia during the comparator period and was removed from the study according to the protocol. Among 22 participants randomly assigned to the comparator period first, two withdrew during the comparator period before participation in the bionic pancreas period because of unrelated illnesses, and one withdrew during the bionic pancreas period because of nausea, anxiety, and inconvenience. Therefore, there were 39 assessable participants. The washout period between study periods was 3 days for 30 participants and 10 days for nine participants. Table 1 lists baseline data for the 39 participants who completed both treatment periods and were included in the analyses. During the comparator period, 22 (56%) of 39 participants used their own unmasked CGM devices as a part of SAP treatment in addition to the masked CGM used to collect study data. Among those who used SAP treatment, the CGM was used on a mean of 87·2% of comparator days (SD 21·9; IQR 81·8–100). Overall, SAP treatment was used during 211 (49%) of 429 days during the comparator period.
Table 1
Baseline characteristics
Adult participants with type 1 diabetes (n=39) | |
---|---|
Sex | |
Male | 18 (46%) |
Female | 21 (54%) |
Age (years) | 33·3 (11·1,19·7–61·3) |
Bodyweight (kg) | 75·8 (15·8,47·5–116·9) |
Body-mass index (kg/m2) | 25·9 (4·8,18.2·384) |
Diabetes duration (years) | 16·9(9·6, 2·2–36·8) |
Daily insulin dose (U/kg) | 0·60 (0·14,0·31–0·93) |
Using sensor-augmented pump | 23 (59%) |
Dexcom G4 | 15 (38%) |
Minimed Enlite | 8 (21%) |
Glycated haemoglobin (%) | 7·7 (1·2,5·4–10·8) |
Estimated mean glucose Concentration (mmol/L)* | 9·7(1·8, 6·0–14·6) |
In terms of the coprimary outcomes, on days 2–11, the mean CGM glucose concentration was 7·8 mmol/L (SD 0·6) in the bionic pancreas period versus 9·0 mmol/L (1·6) in the comparator period (difference 1·1 mmol/L, 95% CI 0·7–1·6; p<0·0001), and the mean time with CGM glucose concentration less than 3·3 mmol/L was 0·6% (0·6) versus 1·9% (1·7; difference 1·3%, 95% CI 0·8–1·8; p<0·0001; figure 2; table 2). In the repeated measures model, we did not detect a significant period effect or carryover effect for either the mean CGM glucose concentration (period effect p=0·34 and carryover effect p=0·72) or the time with CGM glucose concentration less than 3·3 mmol/L (p=0·48 and p=0·81). In the multivariable analyses for both mean CGM glucose and time with CGM glucose concentration less than 3·3 mmol/L, we did not detect an effect of study site (data not shown), or of participant age or insulin total daily dose at baseline on the treatment effect (appendix p 5). For the mean CGM glucose concentration outcome, a higher baseline HbA1c concentration was associated with a larger treatment effect (p=0·0026; appendix p 5). For time with CGM glucose concentration less than 3·3 mmol/L, a longer duration of diabetes was associated with a larger treatment effect (p=0·016) and a higher baseline HbA1c was associated with a smaller treatment effect (p=0·035; appendix p 5). Participants’ use of their own unmasked CGM as a component of SAP treatment, quantitated as the percentage of days during which CGM was used, did not affect the treatment effect either for mean CGM glucose (p=0·62) or for time with CGM glucose concentration less than 3·3 mmol/L (p=0·69; appendix p 5). In a post-hoc analysis, participants were divided into groups according to whether they used CGM (SAP, n=22) or not (CIP, n=17) during the comparator period. The bionic pancreas was associated with reductions in mean CGM glucose in the SAP subgroup (7·9 mmol/L [SD 0·6] in the bionic pancreas period vs 9·0 mmol/L [1·9] in the comparator period; difference 1·2 mmol/L, 95% CI 0·5–1·8; p=0·0026) and in the CIP subgroup (7·8 mmol/L [0·4] vs 8·9 mmol/L [1·0]; 1·1 mmol/L, 0·6–1·6; p=0·00057). Likewise, the bionic pancreas was associated with similar reductions in time with CGM glucose concentration less than 3·3 mmol/L in the SAP subgroup (0·6% [SD 0·7] in the bionic pancreas period vs 1·9% [2·0] in the comparator period; difference 1·3%, 95% CI 0·6–2·1; p=0·0028) and in the CIP subgroup (0·6% [0·4] vs 1·8% [1·4]; 1·2%, 0·6–1·8; p=0·00071).
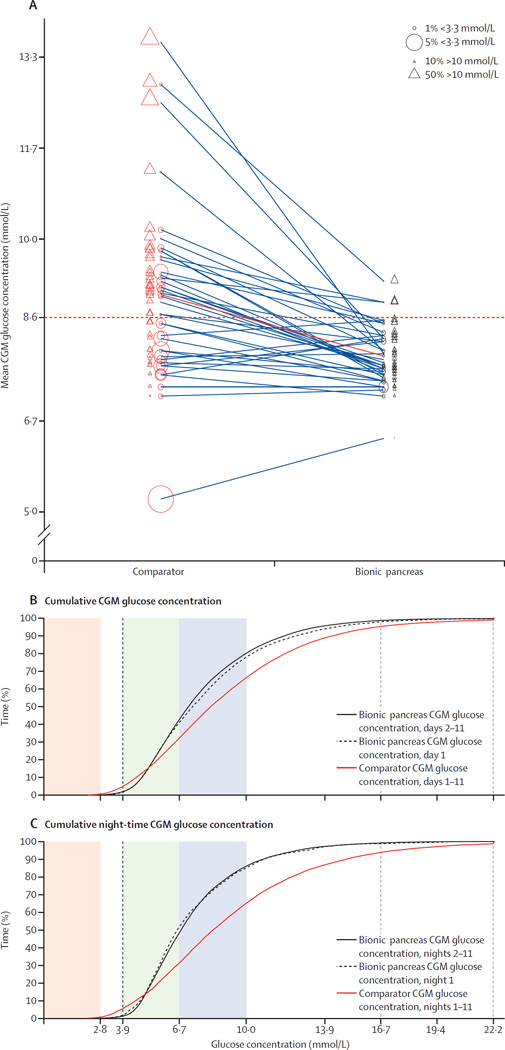
(A) Mean CGM glucose concentration in each participant on days 2–11 of the comparator period (centre of red circles), connected by a line to the corresponding mean CGM glucose concentration during the bionic pancreas period (centre of black circles). The diameter of each circle is proportional to the percentage of time that the patient spent with a CGM glucose concentration less than 3·3 mmol/L. The solid red line represents the aggregate mean for all study participants. The dashed horizontal red line shows a mean CGM glucose concentration of 8·6 mmol/L. (B) Cumulative CGM glucose concentrations during the bionic pancreas period (day land days 2–11) and during the 11-day comparator period, and (C) corresponding cumulative night-time CGM glucose concentrations. In (B) and (C), the shaded regions correspond to CGM glucose concentrations less than 2·8 mmol/L (red), 3·9–6·7 mmol/L (green), and 67–10·0 mmol/L (blue). CGM=continuous glucose monitoring.
Table 2
Summary results of all 11-day experiments
Bionic pancreas | Comparator | Difference (95% CI) | p value | |
---|---|---|---|---|
Day and night CGM glucose concentration on days 2–11 | ||||
Overall mean* | 7·8 (0·6, 6·3–9·2) | 9·0 (1·6, 5·2–13·6) | 1·1 (0·7–1·6) | <0·0001 |
<3·3 mmol/L (% of time)* | 0·6% (0·6, 0·0–2·8) | 1·9% (1·7, 0·0–7·7) | 1·3% (0·8–1·8) | <0·0001 |
3·9–10·0 mmol/L (% of time) | 78·4% (6·0, 66·3–98·6) | 61·9% (14·4, 19·6–88·3) | 16·5% (12·5–20·6) | <0·0001 |
>10·0 mmol/L (% of time) | 19·8% (6·1, 1·2–33·3) | 33·6% (16·4, 0·8–80·3) | 13·8% (9·3–18·3) | <0·0001 |
Participant-level SD (mmol/L) | 2·8 (0·5, 1·4–3·6) | 3·5 (0·8, 1·6–5·9) | 0·6 (0·4–0·9) | <0·0001 |
Coefficient of variation (%) | 35·9% (5·1, 22–45) | 38·8% (6·0, 26–53) | 2·9% (0·7–5·0) | 0·013 |
Daily differences (mmol/L) | 0·9 (0·4, 0·2–2·1) | 1·6 (0·7, 0·6–4·1) | 0·7 (0·5–0·9) | <0·0001 |
Number of carbohydrate interventions per day | 0·4 (0·3, 0·0–1·3) | 0·9 (0·7, 0·0–2·8) | 0·5 (0·3–0·8) | <0·0001 |
Night-time CGM glucose concentration on nights 2–11 | ||||
Overall mean (mmol/L) | 7·4 (0·8, 5·9–9·9) | 9·2 (2·1, 4·9–15·3) | 1·7 (1·2–2·3) | <0·0001 |
<3·3 mmol/L (% of time) | 0·3% (0·4, 0·0–1·7) | 1·9% (2·7, 0·0–13·3) | 1·6% (0·8–2·5) | 0·0008 |
3·9–10·0 mmol/L (% of time) | 84·8% (8·6, 60·1–99·3) | 60·3% (16·9, 14·6–97·7) | 24·6% (20·0–29·1) | <0·0001 |
>10·0 mmol/L (% of time) | 14·1% (8·4, 0·5–39·6) | 34·8% (19·0, 0·0–85) | 20·7% (15·6–25·8) | <0·0001 |
Participant-level SD (mmol/L) | 2·4 (0·6, 1·2–3·8) | 3·5 (0·9, 1·6–5·9) | 0·6 (0·4–0·9) | <0·0001 |
Coefficient of variation (%) | 32·1% (7·1, 20–51) | 37·0% (8·8, 23–57) | 4·9% (1·4–8·3) | 0·0092 |
Data are mean (SD, range), unless otherwise specified. CGM=continuous glucose monitoring.
The bionic pancreas was also associated with an increase in time with CGM glucose 3·9–10·0 mmol/L of 16·5% (95% CI 12·5–20·6; 78·4% [SD 6·0] in the bionic pancreas period vs 61·9% [14·4] in the comparator period; p<0·0001), a reduction in time with CGM glucose more than 10·0 mmol/L of 13·8% (9·3–18·3%, 19·8% [6·1] vs 33·6% [16·4]; p<0·0001), a reduction in time with CGM glucose concentration less than 3·9 mmol/L of 2·7% (1·6–3·9%, 1·8% [1·4] vs 4·5% [3·8]; p<0·0001), and a reduction in time with CGM glucose concentration less than 2·8 mmol/L of 0·4% (0·3–0·6, 0·1% [0·2] vs 0·6% [0·6]; p<0·0001; table 2; figure 2).
During the night-time hours, the bionic pancreas was associated with a reduction in mean CGM glucose concentration of 1·7 mmol/L (95% CI 1·2–2·3 mmol/L; 7·4 mmol/L [SD 0·8] in the bionic pancreas period vs 9·2 mmol/L [2·1] in the comparator period; p<0·0001), a reduction in time with CGM glucose concentration less than 3·3 mmol/L of 1·6% (0·8–2·5%; 0·3% [0·4] vs 1·9% [2·7]; p=0·0008), and an increase in the time within 3·9–10·0 mmol/L of 24·6% (20·0–29·1%; 84·8% [8·6] vs 60·3% [16·9]; p<0·0001; table 2; figure 2). The effect of the bionic pancreas to decrease mean glucose concentration was greater during the night-time than over the full 24 h day by 0·6 mmol/L (95% CI 0·3–0·9; p<0·0001); the effect of the bionic pancreas on reduction in time with CGM glucose concentration less than 3·3 mmol/L was not significantly different at night than over the full 24 h day (p=0·19). The increase in time with CGM glucose within 3·9–10·0 mmol/L was greater during the night-time hours than during the full 24 h day by 8·0% (95% CI 5·5–10·6; p<0·0001).
The aggregate mean and SD of CGM glucose concentrations, as well as the means of daily differences,33 showed lower glucose variability on the bionic pancreas period, both for the full day and at night relative to the comparator (table 2; figure 3). The bionic pancreas achieved a mean CGM glucose concentration less than 8·6 mmol/L during days 2–11 in 36 (92%) of 39 participants versus 16 (41%) of 39 participants during the comparator period (p<0·0001; figure 2; figure 4). There was no difference in the mean CGM glucose concentration during days 2–11 versus during day 1 in the bionic pancreas period (p=0·91) or in the comparator period (p=0·98). Likewise, there was no difference in the time with CGM glucose concentration less than 3·3 mmol/L during days 2–11 versus during day 1 in the bionic pancreas period (p=0·98) or in the comparator period (p=0·92).
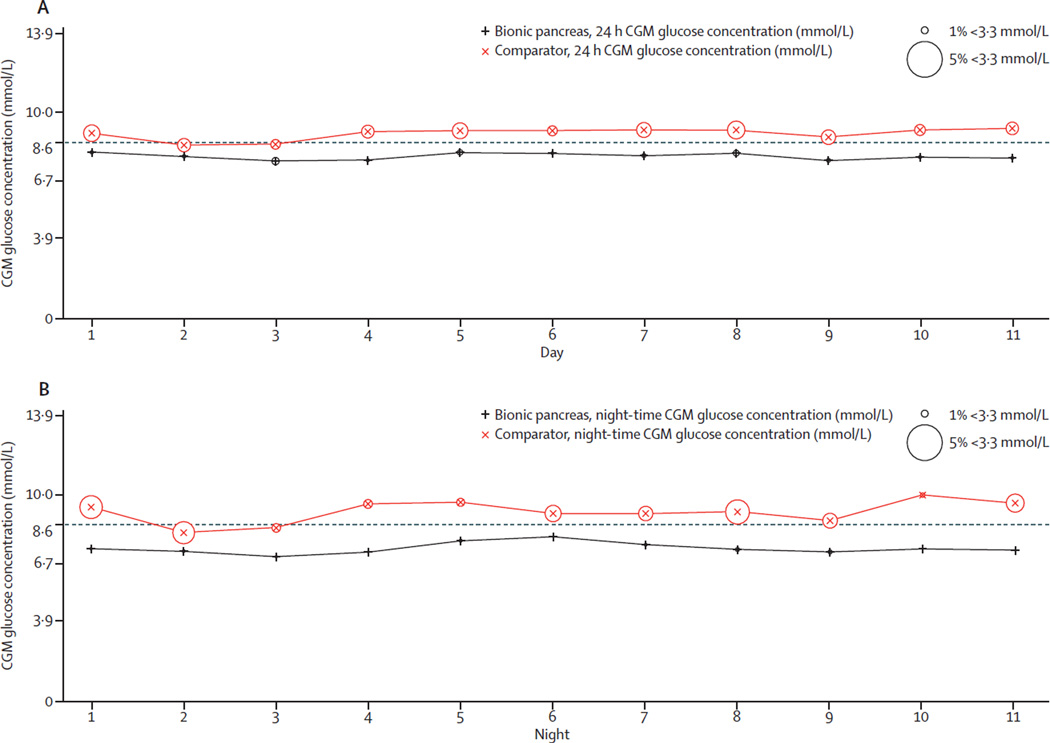
Daily mean CGM glucose concentrations and percentages of time with CGM glucose concentration less than 3·3 mmol/L over 11 days during the full study period (A) and during the night-time period (B). CGM=continuous glucose monitoring.
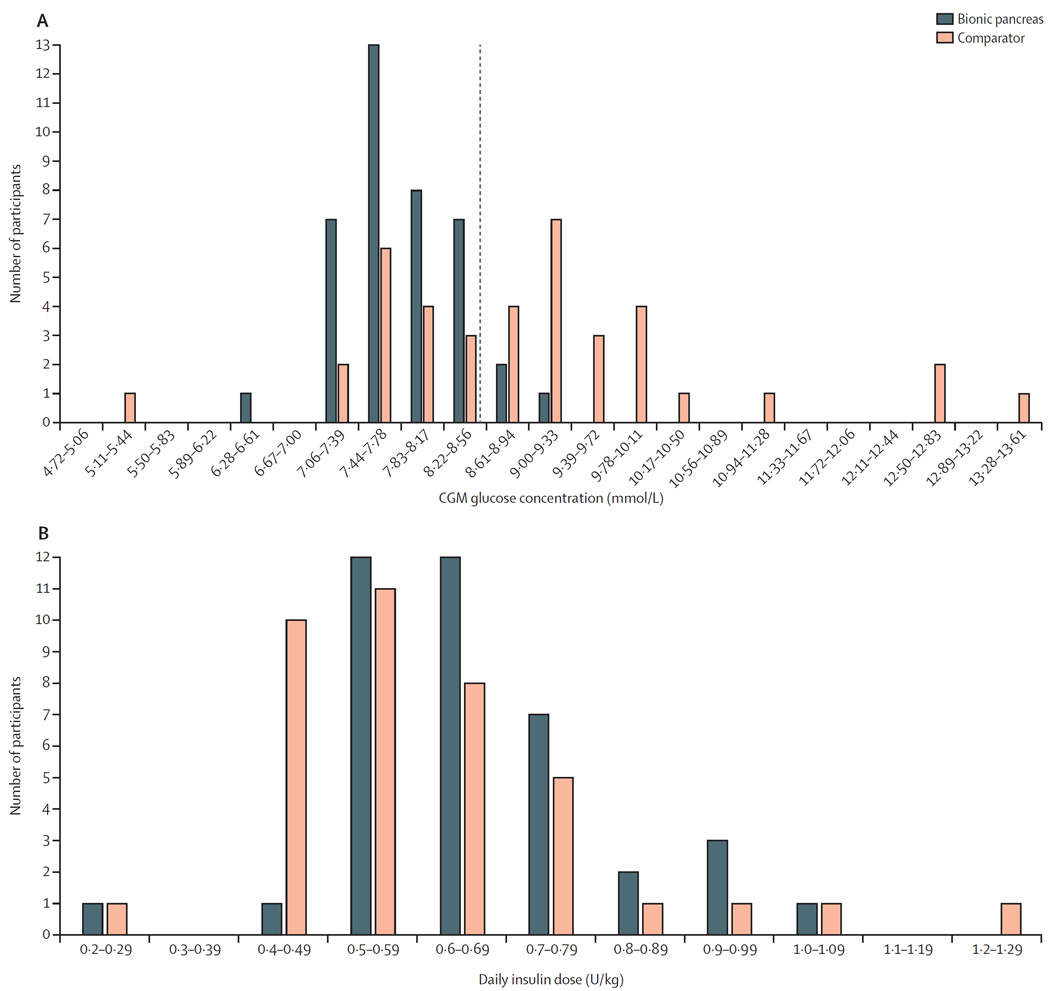
(A) Distribution of the mean CGM glucose concentrations per participant during the bionic pancreas and comparator periods with mean CGM glucose concentrations on days 2–11 divided into intervals of 0·39 mmol/L. The dashed red line shows a mean glucose concentration of 8·6 mmol/L. (B) Distribution of total daily doses of insulin, divided into intervals of 0·1 U/kg of body mass per day. CGM=continuous glucose monitoring.
In response to the daily automated survey, participants reported that, over the entire study period, the bionic pancreas was associated with a reduction in the mean number of symptomatic hypoglycaemia events by 0·31 events per day (95% CI 0·07–0·56; 0·59 events per day [0·56] in the bionic pancreas period vs 0·90 events per day [0·64] in the comparator period; p=0·023), the number of times carbohydrates were taken for hypoglycaemia by 0·5 events per day (95% CI 0·3–0·8; 0·4 events per day [0·3] vs 0·9 events per day [0·7]; p<0·0001; table 2; appendix p 6), and grams of carbohydrates per day consumed to treat hypoglycaemia by 12·8 g/day (8·4–17·2; 22 g/day [14] vs 35 g/day [16]; p=0·00033). Participants completed 425 (99%) of the 429 possible daily surveys in the bionic pancreas period and 416 (97%) in the comparator period.
The mean total daily dose of insulin delivered by the bionic pancreas varied widely between participants (0·26–1·07 U/kg per day), and the mean total daily insulin dose was greater during the bionic pancreas period than during the comparator period by 0·042 U/kg per day (95% CI 0·010–0·074; 0·66 U/kg per day [SD 0·15] in the bionic pancreas period vs 0·62 U/kg per day [0·18] in the comparator period; p=0·014; figure 4). We hypothesised that increased insulin use in the bionic pancreas period could be related to insufficient insulin dosing during the comparator period for participants not meeting glycaemic goals for treatment. In a post-hoc analysis, we found no difference in the mean insulin total daily dose between the bionic pancreas and comparator periods among participants with a mean CGM glucose of 8·6 mmol/L or lower in the comparator period (0·61 U/kg per day [SD 0·15] in the bionic pancreas period vs 0·60 U/kg per day [0·18] in the comparator period; 95% CI −0·027 to 0·044 U/kg per day; p=0·65; appendix p 7). The mean insulin given as adaptive meal-priming boluses by the bionic pancreas in response to meal announcements was 23% (SD 8) of the total insulin delivered over days 2–11. Participants self-administered boluses during the comparator period a mean of 5·6 times per day (SD 2·2) for meals and corrections, whereas during the bionic pancreas period all corrections were automatically done by the bionic pancreas and participants initiated partial meal-priming boluses from the bionic pancreas a mean of 2·6 times per day (SD 0·8; mean range by participant 1·3–5·5) by providing qualitative meal announcements without carbohydrate counting (reduction of 3·0 interactions resulting in insulin delivery per day, 95% CI 2·2–3·7; p<0·0001; appendix p 8). The mean number of meal announcements per day varied by participant from 1·3 per day to 5·5 per day. In a post-hoc analysis, we found no correlation between number of meal announcements during the bionic pancreas period and the treatment effect for either of the coprimary outcomes (p=1·0, R2=0·00028 for mean CGM and p=0·96, R2=0·00778 for time with CGM glucose concentration <3·3 mmol/L). The mean total daily dose of glucagon over days 2–5 was 0·51 mg/day (SD 0·19, range 0·23–0·90), or 6·8 µg/kg per day (2·4; appendix p 17). Participants were asked to take at least four capillary plasma glucose measurements during the bionic pancreas period, but took fewer plasma glucose measurements in the bionic pancreas period than in the comparator period by 1·2 measurements per day (95% CI 0·6–1·7; 4·1 measurements per day [SD 1·7] vs 5·3 measurements per day [1·8]; p=0·0001). Plasma glucose values were entered into the bionic pancreas on 14 occasions (mean 0·4 occasions per participant) when the CGM signal was unavailable; the bionic pancreas responded with bolus insulin on three of those occasions and bolus glucagon on no occasions.
No episodes of severe hypoglycaemia occurred in the bionic pancreas period. One participant had an episode of severe hypoglycaemia in the comparator period and was removed from the study according to the protocol (appendix p 57).
We found no difference in the self-reported mean minutes per day engaged in exercise in the bionic pancreas and comparator periods (39 min/day [SD 28] in the bionic pancreas period vs 39 min/day [32] in the comparator period; 95% CI −8·9 to 8·0; p=0·99), or in alcohol consumption between the two periods (0·5 drinks per day [0·5] vs 0·5 drinks per day [0·5]; –0·1 to 0·2; p=0·35).
There was no difference in the change in body mass from day 1 to day 11 between the bionic pancreas and comparator periods (0·4 kg [SD 1·0] in the bionic pancreas period vs 0·1 kg [1·1] in the comparator period; 95% CI −0·1 to 0·8; p=0·17). Likewise, from day 1 to day 11, there was no difference between the bionic pancreas and comparator periods in the mean change in systolic (−2·2 mm Hg [12] vs −1·9 mm Hg [16]; 95% CI −6·3 to 4·0; p=0·93) or diastolic blood pressure (−1·1 mm Hg [8·0] vs −1·9 mm Hg [10·0]; −12·3 to 5·9; p=0·70). When participants were asked to report nausea on a Visual Analogue Scale, the number reporting a score greater than 0 on at least one day was 21 in the bionic pancreas period and five in the comparator period.
The mean nausea score on the Visual Analogue Scale was greater during the bionic pancreas period (0·52 [SD 0·83]) than in the comparator period (0·05 [0·17]; difference 0·47, 95% CI 0·21–0·73; p=0·0024; appendix p 9). Two participants reported vomiting in the bionic pancreas period and one in the comparator period. There was no difference in the mean change in any haematology parameter or in 22 of the 25 chemistry tests from day 1 to day 11 between the bionic pancreas and comparator periods. The mean change differed significantly between periods in three of the tests, but these were not safety concerns because the values remained within the normal range (appendix p 10). Of 1443 possible instances (ie, pairs of before and after values for the same test), laboratory test results changed from normal to abnormal between days 1 and 11 in 48 instances during the bionic pancreas period and in 44 instances during the comparator period (p=0·98).
The mean absolute relative difference between plasma glucose concentrations and the closest CGM glucose value within 2·5 min of a plasma glucose measurement was 18·5% (mean of 91·1 plasma glucose–CGM measurement pairs per participant; n=3554), with a mean negative bias (CGM glucose concentration below the plasma glucose concentration) of 1·1%. The mean absolute relative difference did not differ between the bionic pancreas and comparator periods (19·2% vs 18·0%; 95% CI −0·7 to 3·2; p=0·22).
The insulin pumps lost wireless connectivity to the bionic pancreas a mean of 3·9% (SD 2·5) of the time and the glucagon pumps 4·1% (2·5%) of the time. The CGM signal was unavailable 3·4% of the time during the bionic pancreas period and 3·7% of the time during the comparator period (95% CI −0·78 to 0·30; p=0·39). 11 (6%) of 200 insulin infusion site changes in the bionic pancreas period and 15 (9%) of 175 in the comparator period were unscheduled (appendix p 11). 14 (3%) of 422 glucagon infusion site changes were unscheduled (appendix p 11). During the bionic pancreas period, the device was being worn and working properly for at least 23 h a day on 393 (95·2%) of 413 days for which surveys were returned. During the comparator period, the bionic pancreas monitoring configuration (with only the masked CGM active) was being worn and working properly for at least 23 h a day on 396 (96·8%) of 409 days for which surveys were returned (appendix pp 12–14).
Discussion
In this study, the bionic pancreas reduced mean CGM glucose concentrations, hypoglycaemia, symptomatic hypoglycaemia, and carbohydrates given to treat hypoglycaemia relative to the comparator period. This finding occurred despite participants taking fewer capillary plasma glucose measurements and triggering fewer insulin boluses during the bionic pancreas period. When participants did interact with the bionic pancreas to announce a meal, the interaction required no carbohydrate counting.
By contrast with other automated insulin delivery systems,20–25 the bionic pancreas tested in this study automated delivery of both insulin and glucagon and required no information for initialisation other than the participant’s body mass.20–27 In this study, the participants’ insulin requirements varied by more than four times during the bionic pancreas period, yet the bionic pancreas adapted automatically to each participant’s insulin needs and was able to manage glycaemia with no information about the participants’ past insulin-pump regimens. Whether artificial pancreas systems can be used as the initial treatment, without a preliminary period of CIP or SAP treatment, in patients newly diagnosed with type 1 diabetes remains to be established.
Debate is ongoing regarding what is the best control group for studies of artificial pancreas systems: usual care or the most sophisticated treatment available (ie, SAP treatment).34 In this study, more than half of participants used SAP treatment, and the effect of the bionic pancreas was similar in participants who used SAP and CIP treatments. Future studies should include participants who are naive to advanced diabetes technology,34 since many patients with type 1 diabetes do not use pumps (30–60% are pump users)35, 36 and an even smaller proportion use CGM (~11% used CGM according to a 2015 survey).35, 36 The small amount of input required from the user by the bionic pancreas is likely to make it particularly useful in patients who have no previous experience with advanced diabetes technology.
This study had several limitations. The number of participants was small and their glycaemic control was better than usually found in patients with type 1 diabetes at baseline.4–6 The study duration was short and a longer trial is needed to establish whether patients will consistently use the bionic pancreas for longer periods. This was a crossover trial, so there was the possibility of both period and carryover effects; although no such effects were detected, the trial was not powered to detect them. At the request of the US Food and Drug Administration, we monitored participants remotely in both periods of the study for severe biochemical hypoglycaemia (CGM glucose <2·8 mmol/L) and alerted participants who had episodes below this threshold lasting more than 15 min. The percentage of time with CGM glucose concentration less than 3·3 mmol/L and less than 2·8 mmol/L were both reduced in the bionic pancreas period compared with the comparator period, but these differences might have been larger if no remote monitoring had been done. We also monitored remotely for loss of wireless communication between the iPhone and the pump that lasted for more than 15 min. Results with this version of the bionic pancreas would probably have been worse without monitoring for connectivity. Longer duration day-and-night home-use studies of an insulin-only artificial pancreas system have been done successfully without remote monitoring.23–25 The next generation of our bionic pancreas integrates both of the pumps, the CGM receiver, and the algorithms into a single unit, thereby eliminating the need for wireless communication with the pumps, and this device will need to be tested in studies without remote monitoring. Our study did not compare the bihormonal bionic pancreas with an insulin-only configuration. Other investigators have directly compared insulin-only and bihormonal artificial pancreas systems in night-time-only studies.37, 38
The version of the bionic pancreas device used in this study has several limitations. Acetaminophen can lead to overestimation of blood glucose by the current generation Dexcom CGM, so we requested that participants refrain from using acetaminophen.39, 40 The next generation of the Dexcom CGM sensors will be resistant to interference by acetaminophen. Other CGM sensor technologies available in Europe but not the USA (Abbott FreeStyle Flash, Abbott Diabetes Care, Abbot Park, IL, USA; and Senseonics Eversense, Senseonics, Germantown, MD, USA) are resistant to such interference.40, 41 The glucagon reservoir had to be filled daily because of the unstable nature of glucagon formulations. Stable formulations and analogues of glucagon have been developed42–46 that should obviate the need for daily glucagon reservoir changes; however, the extent to which the use of glucagon will increase the cost of treatment is unknown.
Several findings from this trial warrant further investigation. More participants experienced nausea in the bionic pancreas period than in the comparator period, although the absolute amount was low. In this study, but not in our two previous studies in adolescents and preadolescents,17, 18 more insulin was used in the bionic pancreas period than in the comparator period. The increment was small and there was no difference in insulin total daily dose among participants who achieved a mean glucose concentration in the comparator period (8·6 mmol/L) predicted to give an HbA1c of 7% or lower.30, 31 This result is consistent with the bionic pancreas delivering additional insulin to participants who were not taking enough insulin during the comparator period. Likewise, small changes in several laboratory values were associated with the use of the bionic pancreas, but none seemed to raise safety concerns. Whether these findings are of clinical significance, and whether the balance of benefits, risks, and costs associated with the use of glucagon will be favourable overall, requires further study.47
Relative to CIP treatment and SAP treatments, the bihormonal bionic pancreas, initialised only with participant body mass, was able to achieve superior glycaemic regulation in this free-living home-use trial, without the need for carbohydrate counting. A fully integrated device should be tested in much larger studies of longer duration to establish the long-term benefits and risks of automated glycaemic management with a bihormonal bionic pancreas.
Acknowledgments
This study was funded by the National Institute of Diabetes and Digestive and Kidney Diseases of the National Institutes of Health under contracts R01DK097657 and DP3DK101084 (both to ERD and SJR), and through the following National Center for Advancing Translational Sciences clinical and translational science awards: UL1TR001453 (to University of Massachusetts Medical School); UL1TR001085 (to Stanford University); and UL1TR001111 (to University of North Carolina). The content of this Article is solely the responsibility of the authors and does not necessarily represent the official views of the National Institutes of Health. We thank the volunteers, their families, and their designated contacts for their participation and enthusiasm. We thank Eli Lilly, USA, for donating the glucagon used in this study; Mary Larkin, Camille Collings, Irene Orzechowski, Nancy Kingori, Patricia Cannon, Janet Framiglio, Jill Cunnup, Khadija Tlaiti, Lisa Hubacz, Nancy Shaffer, Karen Gallagher-Dorval, Joseph Largay, Elizabeth Debnam, Christian Bennett, Michelle Duclos, Kim Josey, and Natalie Broadway for organisational and logistical support; the remote monitoring staff at each of the four clinical sites for their dedication and careful attention to telemetric monitoring and equipment maintenance; George Zamanakos, Justin Schumacher, Liam Pender, Vance Swanson, and James Sidwell for hardware and software support, technical advice, and support in kind; Niall Kavanagh, Hilary Orenberg, and Trevor Macdowell for user interface software support; Fyodor Wolf, Inderpreet Singh, and Murugesh Vyravanadan for remote monitoring software support; Sheila Ramerman for software and hardware documentation support; Deborah Wexler, Geoffrey Walford, Nancy Wei, and John Brooks III for serving on the data safety and monitoring board; and the members of the Partners Human Research Committee, the Institutional Review Boards of Boston University Charles River Campus, the University of Massachusetts Medical School, Stanford University, and the University of North Carolina for their oversight of the study.
Footnotes
Contributors
FHE-K, DMH, BB, JBB, ERD, and SJR designed the study and wrote the manuscript. FHE-K and ERD designed and built the closed-loop control algorithm and the bionic pancreas device. FHE-K, CB, RRS, ERD, and SJR collated, analysed, and interpreted the data. AE and HZ did additional statistical analyses. DMH, BAB, JBB, and SJR supervised the clinical study and SJR led the clinical team. All of the authors reviewed the draft report for content.Declaration of interests
FHE-K, ERD, and RRS have equity in Beta Bionics. FHE-K, ERD, and SJR have received loaned equipment, support in kind, and technical assistance from Dexcom and Tandem Diabetes Care. FHE-K and ERD hold a patent related to a fully automated control system for type 1 diabetes (US 7 806 854) and pending patent applications related to a blood glucose control system (PCT/US 11/058 688 and PCT/US 13/870 634), all assigned to Boston University. ERD has received lecture fees from Dexcom, Tandem Diabetes Care, and Eli Lilly, and serves on the board of directors of Beta Bionics. SJR has received lecture fees from Tandem Diabetes Care, Sanofi Aventis, Eli Lilly, and Abbott Diabetes Care; serves on the scientific advisory boards for Tandem Diabetes Care and Companion Medical; and holds a pending patent application for a blood glucose control system (PCT/US 13/870 634), assigned to Partners HealthCare and Massachusetts General Hospital. All other authors declare no competing interests.
References
Full text links
Read article at publisher's site: https://doi.org/10.1016/s0140-6736(16)32567-3
Read article for free, from open access legal sources, via Unpaywall:
https://europepmc.org/articles/pmc5358809?pdf=render
Citations & impact
Impact metrics
Article citations
Carbohydrate Counting: A Bibliometric Analysis with a Focus on Research.
Nutrients, 16(19):3249, 26 Sep 2024
Cited by: 0 articles | PMID: 39408216 | PMCID: PMC11478275
Diabetes technology in people with diabetes and advanced chronic kidney disease.
Diabetologia, 67(10):2129-2142, 08 Aug 2024
Cited by: 0 articles | PMID: 39112642 | PMCID: PMC11446991
Review Free full text in Europe PMC
Approaches of wearable and implantable biosensor towards of developing in precision medicine.
Front Med (Lausanne), 11:1390634, 18 Jul 2024
Cited by: 0 articles | PMID: 39091290 | PMCID: PMC11293309
Review Free full text in Europe PMC
14. Children and Adolescents: Standards of Care in Diabetes-2024.
Diabetes Care, 47(suppl 1):S258-S281, 01 Jan 2024
Cited by: 14 articles | PMID: 38078582
Review
Randomized Trial of the Insulin-Only iLet Bionic Pancreas for the Treatment of Cystic Fibrosis- Related Diabetes.
Diabetes Care, 47(1):101-108, 01 Jan 2024
Cited by: 3 articles | PMID: 37874987
Go to all (122) article citations
Data
Data behind the article
This data has been text mined from the article, or deposited into data resources.
BioStudies: supplemental material and supporting data
Clinical Trials
- (2 citations) ClinicalTrials.gov - NCT02092220
Similar Articles
To arrive at the top five similar articles we use a word-weighted algorithm to compare words from the Title and Abstract of each citation.
Day and night glycaemic control with a bionic pancreas versus conventional insulin pump therapy in preadolescent children with type 1 diabetes: a randomised crossover trial.
Lancet Diabetes Endocrinol, 4(3):233-243, 03 Feb 2016
Cited by: 97 articles | PMID: 26850709 | PMCID: PMC4799495
Outpatient glycemic control with a bionic pancreas in type 1 diabetes.
N Engl J Med, 371(4):313-325, 15 Jun 2014
Cited by: 300 articles | PMID: 24931572 | PMCID: PMC4183762
Comparison of dual-hormone artificial pancreas, single-hormone artificial pancreas, and conventional insulin pump therapy for glycaemic control in patients with type 1 diabetes: an open-label randomised controlled crossover trial.
Lancet Diabetes Endocrinol, 3(1):17-26, 27 Nov 2014
Cited by: 118 articles | PMID: 25434967
Funding
Funders who supported this work.
NCATS NIH HHS (3)
Grant ID: UL1 TR001453
Grant ID: UL1 TR001111
Grant ID: UL1 TR001085
NIDDK NIH HHS (3)
Grant ID: T32 DK007028
Grant ID: DP3 DK101084
Grant ID: R01 DK097657