Abstract
Free full text

Lysosome and endoplasmic reticulum quality control pathways in Niemann-Pick type C disease
Abstract
Lysosomal storage diseases result from inherited deficiencies of lysosomal hydrolytic activities or lipid transport. Collectively, these disorders are a common cause of morbidity in the pediatric population and are often associated with severe neurodegeneration. Among this group of diseases is Niemann-Pick type C, an autosomal recessive disorder of lipid trafficking that causes cognitive impairment, ataxia and death, most often in childhood. Here, we review the current knowledge of disease pathogenesis, with particular focus on insights gleaned from genetics and the study of model systems. Critical advances in understanding mechanisms that regulate intracellular cholesterol trafficking have emerged from this work and are highlighted. We review effects of disease-causing mutations on quality control pathways involving the lysosome and endoplasmic reticulum, and discuss how they function to clear the most common mutant protein found in Niemann-Pick type C patients, NPC1-I1061T. Finally, we summarize insights into the mechanisms that degrade misfolded transmembrane proteins in the endoplasmic reticulum and how manipulating these quality control pathways may lead to the identification of novel targets for disease-modifying therapies.
Introduction
Intracellular substrates from endocytosis, phagocytosis, and autophagy traffic to and mature within lysosomes. The acidic environment of the lysosome allows substrate degradation, thereby enabling the recycling of limited cellular constituents. In addition to participating in quality control, recent studies have established that lysosomes are important nodes in the homeostatic response to metabolic demands (reviewed in (Ferguson, 2015)). Given these essential functions, it is not surprising that more than fifty human diseases related to lysosomal dysfunction have been characterized. The pathology of these lysosomal storage disorders often manifests with the accumulation of proteins or lipids that are normally substrates for dysfunctional enzymes or transporters and with cellular toxicity (Micsenyi et al., 2013; Schultz et al., 2011). In this review, we focus on the lysosomal storage disorder Niemann-Pick type C disease. We summarize the effects of disease-causing mutations on cellular quality control pathways involving the lysosome and endoplasmic reticulum. In addition, we highlight how these quality control pathways have emerged as rational targets for the development of disease-modifying therapies.
Niemann-Pick Disease
The Niemann-Pick diseases are a group of autosomal recessive sphingolipid storage disorders that were first described by Albert Niemann and Ludwig Pick in the early 20th century (Pentchev, 2004). These disorders are clinically heterogeneous, affecting patients from early infancy to adulthood. Identification of causal mutations has simplified the categorization of Niemann-Pick disease into three types (Table 1). Types A and B are characterized by deficiency of the lysosomal enzyme acid sphingomyelinase, encoded by the sphingomyelin phosphodiesterase-1 (SMPD1) gene (Brady et al., 1966). These disease types are distinguished by differences in symptoms and pathology that likely represent a continuum resulting from loss of enzymatic activity. Patients with type A disease produce little (<1%) to no functional enzyme and exhibit severe disease with neurological manifestations that lead to death in infancy or early childhood. Type B patients have greater enzyme activity (often ~10%), develop little or no neurological disease, but show peripheral manifestations including heptosplenomegaly and can survive into adulthood. Patients with clinical manifestations that span the distinction between types A and B (so-called type A/B) have intermediate levels of enzyme activity.
Table 1
Niemann-Pick Diseases
Disease | Gene Mutation | Main accumulating substrate | Primary phenotype |
---|---|---|---|
NPA | SMPD1 (<1% of WT enzyme activity) | Sphingomyelin | Neurological and peripheral |
NPB | SMPD1 (≈10% of WT enzyme activity) | Sphingomyelin | Peripheral organ enlargement |
NPC | NPC1 or NPC2 | Unesterified Cholesterol | Neurological and peripheral |
In contrast to the severe loss of acid sphingomyelinase activity that characterizes types A and B disease (Brady et al., 1966), type C patients develop prominent intracellular accumulation of unesterified cholesterol in the setting of relatively preserved sphingomyelinase activity (Pentchev et al., 1985). Disease onset and clinical symptoms of type C patients are quite variable and do not closely correlate with the degree of cholesterol accumulation (Ribeiro et al., 2001). Symptom onset can occur shortly after birth, at which time hepatosplenomegaly is often present, or in adulthood, when psychiatric manifestations may be evident. Most type C patients develop progressive cognitive decline, seizures, dystonia, and abnormal eye movements (supranuclear gaze palsy) during childhood and die before age 20 (Higgins et al., 1992; Vanier and Millat, 2003).
Complementation studies and genetic analyses revealed that type C disease is caused by loss of function mutations in two distinct genes, NPC1 and NPC2 (Carstea et al., 1997; Morris et al., 1999; Vanier et al., 1996). Both NPC1 and NPC2 proteins have lysosomal localization (Naureckiene et al., 2000). While NPC2 is a soluble luminal protein, NPC1 is located in the lysosomal membrane and contains 13 transmembrane domains, three large cytoplasmic hydrophilic loops, and a short cytoplasmic tail (Fig. 1) (Davies and Ioannou, 2000). Mutations in either NPC1 or NPC2 result in the accumulation of unesterified cholesterol and sphingolipids within late endosomes and lysosomes, producing a clinically similar phenotype.
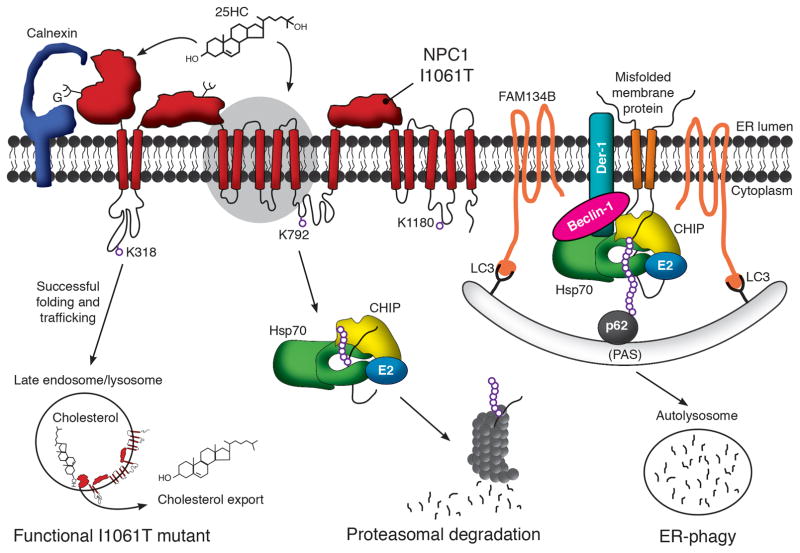
NPC1 is a multipass transmembrane glycoprotein whose folding is facilitated by the calnexin/calreticulin machinery. Folding is also promoted by binding cholesterol or oxysterols at sites in the NTD and perhaps in more C-terminal regions, such as the sterol sensing domain (highlighted by grey oval). The natively folded protein traffics through the Golgi to late endosome/lysosomes, where it facilitates efflux of unesterified cholesterol. Both WT and NPC1-I1061T, a missense mutant that causes disease, are degraded by the proteasome if they fail to properly fold. While components of the ERAD pathway that participate in this process have not been defined, cytosolic machinery including Hsp70 and CHIP have been implicated in the clearance process. Ubiquitination of NPC1 occurs on three lysine resides (318, 792 and 1180) which face the cytosol. Also implicated in the clearance of misfolded multipass transmembrane proteins is ER-phagy. Through this pathway, membrane proteins in the ER are engulfed by pre-autophagosome structures (PAS), which subsequently form autophagosomes, fuse with lysosomes, and lead to substrate degradation in autolysosomes. The extent to which this pathway contributes to NPC1 degradation is currently unknown.
Experimental evidence from diverse model systems demonstrates that these two proteins function cooperatively in the efflux of cholesterol from late endosomes and lysosomes. Within the lysosome are a number of vesicular structures, known as inner lysosomal membranes, and lipids trafficked to the lysosome partition to these structures. NPC2 is thought to function by extracting cholesterol from these inner membranes and then transferring it to the amino-terminal domain (NTD) of NPC1. NPC2 facilitates the opening of the cholesterol binding pocket in NPC1’s NTD (Infante et al., 2008b; Kwon et al., 2009; Wang et al., 2010). NPC2 and NPC1 bind opposite ends of the cholesterol molecule, suggesting that a “hand off” mechanism enables cholesterol efflux (Infante et al., 2008a). While a crystal structure of the NPC1 and NPC2 cholesterol transfer has not been produced, data from multiple studies support this mechanism. Once transferred, it is unknown whether NPC1 inserts cholesterol into the limiting membrane of the lysosome or pumps it across the membrane to an acceptor.
Our current understanding of type C disease pathogenesis is guided by studies of mouse models that have defined several general principles. In Npc1 deficient mice, as in patients with this disorder, neurons accumulate lipids, abnormally swollen axons are frequent, and neuron loss occurs. These features are associated with impaired motor function and early death that model the degenerative phenotype of Niemann-Pick type C patients. While systemic manifestations also occur in both patients and mutant mice, progressive neurological impairment is due to loss of functional Npc1 protein in the nervous system (Loftus et al., 2002). Conditional deletion of the mouse Npc1 gene in neurons is sufficient to cause many features characteristic of Niemann-Pick type C neuropathology, including selective neuron loss that particularly involves Purkinje cells in anterior cerebellar lobules, delayed forebrain myelination, and reactive gliosis (Elrick et al., 2010; Yu et al., 2011; Yu and Lieberman, 2013). All of these features, except delayed myelination, occur following deletion of Npc1 in the adult mouse brain, indicating that most neuropathology is a consequence of degeneration rather than developmental impairment (Yu et al., 2011; Yu and Lieberman, 2013). Complementary studies demonstrated that transgenic over-expression of Npc1 only in neurons delays many of these features of CNS disease in Npc1−/− mice (Lopez et al., 2011). Taken together, these studies demonstrate that neurons but not astrocytes are important target cells for neurodegeneration in type C disease.
Niemann-Pick type C patients exhibit significant phenotypic variability (Ribeiro et al., 2001) despite the fact that ~95% of cases are caused by mutations in the NPC1 gene (Vanier, 2010). To date, over 250 disease-causing mutations have been identified throughout the NPC1 gene, the majority of which encode missense alleles. Of these, substitution of isoleucine 1061 to threonine (I1061T) is present in ~20% of patients of western European ancestry (Vanier, 2010). Patients homozygous for NPC1-I1061T typically present with juvenile-onset neurological disease. Because of the prevalence of this allele and the severity of disease with which it is associated, much work has investigated how the I1061T mutant protein is degraded. These studies have demonstrated that a fraction of over-expressed NPC1-I1061T escapes degradation, properly traffics, and functions in late endosomes/lysosomes (Gelsthorpe et al., 2008). Since the mutant protein is functional, efforts to increase its folding and trafficking through the secretory pathway have emerged as attractive therapeutic strategies. While cellular models such as patient fibroblasts have been used to test compounds that act through this mechanism, gene targeting has enabled the generation of mice expressing Npc1-I1061T so that proteostasis modulators can now be tested in vivo (Praggastis et al., 2015). Notably, NPC1-I1061T is only one of many disease-causing missense alleles, and it is likely that others may influence NPC1 trafficking and function in a distinct manner. Such complexity would be reminiscent of the diversity of effects seen as a result of mutations in the cystic fibrosis transmembrane conductance regulator in patients with cystic fibrosis, and will have significant implications for therapy development.
NPC1 proteostasis
Possibly due to its large size and complicated structure, it is estimated that slightly more than half of the synthesized wild type (WT) NPC1 protein misfolds and is degraded (Gelsthorpe et al., 2008; Nakasone et al., 2014). This is consistent with the notion that 30–75% of all proteins entering the ER fail to achieve a native or near-native state (Ellgaard and Helenius, 2003). Efforts to measure the half-life of the endogenous WT protein have revealed two different pools. One pool, with a shorter, 9 hour half-life, may represent misfolded forms which are rapidly degraded, while the long-lived fraction with a half-life of 42 hours may represent functional NPC1 in late endosomes/lysosomes. Other efforts to quantify NPC1 half-life in mouse or human cells have similarly detected the short-lived protein pool, but have had more difficultly detecting long-lived proteins (Nakasone et al., 2014) perhaps due to technical differences between approaches. Interestingly, cholesterol depletion causes rapid ubiquitination and degradation of NPC1 (Nakasone et al., 2014; Ohsaki et al., 2006). This observation suggests that binding of ligand to the newly synthesized protein in the ER may favor its folding and trafficking.
Although NPC1 mRNA levels are similar in WT and mutant cells, the steady-state level of NPC1-I1061T protein is reduced by up to 85% (Ribeiro et al., 2001; Yamamoto et al., 2000) indicating that the mutant protein is rapidly and efficiently degraded. Endogenous or over-expressed human or mouse NPC1-I1061T have half-lives measured between 4–6.5 hours (Gelsthorpe et al., 2008; Nakasone et al., 2014; Praggastis et al., 2015).These data are consistent with immunofluorescence analyses and endoglycosidase H sensitivity studies which revealed that NPC1-I1061T fails to traffic through the Golgi (Gelsthorpe et al., 2008; Praggastis et al., 2015), indicating that misfolded forms in the endoplasmic reticulum (ER) are targeted for degradation. However, it is possible that a small fraction of the remaining I1061T protein traffics to the lysosome and is functional. While this notion is suggested by the observation that Npc1-I1061T knock-in mice have a milder disease phenotype than null mice (Praggastis et al., 2015), NPC1-I1061T protein levels do not increase when human fibroblasts are treated with the lysosomal inhibitors chloroquine, NH4Cl or leupeptin (Gelsthorpe et al., 2008; Nakasone et al., 2014). A possible explanation of this discrepancy is that these small molecules are only weakly effective at altering lysosomal pH to inhibit protein degradation (Yoshimori et al., 1991).
The preponderance of data in the literature indicate that misfolded NPC1-I1061T is removed from the ER by ER associated degradation (ERAD) and degraded in the proteasome (Fig. 1). Although the precise components of the ERAD machinery that mediate NPC1 degradation remain to be defined, treatment of cells expressing NPC1-I1061T with proteasome inhibitors MG132 or lactacystin increases steady-state NPC1-I1061T protein levels by ~3.5 fold (Gelsthorpe et al., 2008; Nakasone et al., 2014). That defective folding drives the mutant protein to the proteasome for degradation is supported by incubating cells at reduced temperature or with the chemical chaperones 4-phenylbutyic acid or glycerol, manipulations which increase protein levels by 1.4–1.7 fold (Gelsthorpe et al., 2008; Nakasone et al., 2014). Moreover, mass spectrometry studies have revealed that NPC1 is ubiquitinated at lysine residues 318, 792, and 1180 (Fig. 1) (Nakasone et al., 2014). Immunoprecipitation of overexpressed and endogenous WT and NPC1-I1061T demonstrate interactions with the molecular chaperones Hsp70, Hsc70, Hsp90, and the chaperone-dependent E3 ubiquitin ligase CHIP (C terminus of Hsc70-interacting protein) as well as the ER-localized chaperone calnexin (Fig. 1). These finding indicate that NPC1 complexes with quality control machinery in both the cytosol and ER.
Within the ER, the calnexin/calreticulin machinery appears to play an important role in folding of glycosylated NPC1-I1061T. Calnexin overexpression or ryanodine receptor antagonists, small molecule inhibitors of ryanodine-dependent ER calcium efflux channels, promote protein trafficking and rescue function in patient fibroblasts (Yu et al., 2012). Similarly, survival of iPSC derived NPC1-I1061T neural stem cells is increased by treatment with the ryanodine receptor antagonist dantrolene (Efthymiou et al., 2015). These observations raise the possibility that NPC1 deficiency alters ER calcium levels, similar to alterations of lysosomal calcium that have been observed by several groups (Lloyd-Evans et al., 2008; Shen et al., 2012). In the ER, calcium dysregulation could impact mutant NPC1 folding, favoring a state in which missense mutants are targeted for degradation.
Lysosomal quality control
Macroautophagy
The degradation of misfolded proteins and dysfunctional organelles through the lysosomal quality control pathway of autophagy has garnered considerable attention in the lysosomal storage diseases (for review (Lieberman et al., 2012)). Impairments of autophagy have been implicated as contributing to the pathogenesis of many of these disorders. In Niemann-Pick type C disease, NPC1 deficiency results in the marked accumulation of autophagosomes in neurons of mutant mice and in primary fibroblasts from patients. This is attributable, in part, to the beclin-1 dependent induction of macroautophagy (Ko et al., 2005; Pacheco and Lieberman, 2007). This autophagy induction has also been observed in Npc1−/− mice, where significantly elevated levels of LC3-II and cholesterol accumulation occur at 4 weeks of age (Liao et al., 2007). The induction of autophagy is accompanied by a relatively modest increase in autophagic flux as measured by the degradation of long-lived proteins (Pacheco and Lieberman, 2007). The limited increase in the rate of protein degradation along with the accumulation of ubiquitinated, p62-positive protein aggregates (Elrick et al., 2012) and fragmented mitochondria in neurons (Ordonez et al., 2012) indicates that disease is also associated with diminished autophagic flux. Studies in NPC1 deficient cells have identified several distinct points at which substrate degradation is impaired to varying degrees, including defective amphisome formation caused by a failure in the SNARE machinery (Sarkar et al., 2013) and diminished lysosomal cathepsin activity (Elrick et al., 2012). Despite this block in autophagic flux, several reports have suggested that upregulation of macroautophagy may be beneficial to mutant cells (Maetzel et al., 2014; Sarkar et al., 2013).
ER-phagy (ER autophagy)
While lysosomal quality control pathways may be disrupted in Niemann-Pick disease, it is also possible that they contribute to the degradation of NPC1 missense mutants. In instances where ERAD is inefficient at removing protein substrates for degradation, an alternative pathway termed ER-phagy can be activated which targets substrates to the lysosome for degradation (Fig. 1) (Houck et al., 2014; Lipatova and Segev, 2015). In yeast, where ER-phagy is best characterized, it is estimated that up to 20–50% of endogenous membrane proteins and up to 90% of over-expressed membrane proteins are degraded by ER-phagy (Lipatova and Segev, 2015). The components of this pathway and its contributions to quality control in mammalian cells are still being defined. Unlike proteins targeted to classical ERAD, current models suggest that misfolded transmembrane proteins in mammalian cells targeted to ER-phagy are not extracted from the membrane. Instead, these substrates are recognized by a complex of Hsp70, beclin-1, derlin-1, and FAM134B (Fig. 1) (Houck et al., 2014; Khaminets et al., 2015; Mochida et al., 2015). FAM134B, a recently defined adapter protein for this pathway, binds the canonical autophagy modifiers GABARAP and LC3, triggering autophagosome formation and eventual degradation in the lysosome (Khaminets et al., 2015). Although the extent to which this pathway degrades misfolded NPC1 is currently unknown, its importance in yeast quality control suggests that it may play a similar role in the clearance of misfolded transmembrane proteins in mammalian cells.
Lysosomal membrane permeabilization
Lysosomal damage in storage disorders may trigger lysosomal membrane permeabilization, resulting in the release of enzymes into the cytosol and triggering a cascade that can lead to cell death. This process may contribute to the accumulation of ubiquitinated proteins in the cytosol of neurons in the diseased brain (Micsenyi et al., 2013). Experimental evidence suggests the occurrence of lysosomal membrane permeabilization in models of both type A, B, and type C disease. In both instances, cathepsin mislocalization outside lysosomes has been demonstrated by biochemical fractionation and immunohistochemical studies of brain tissue (Amritraj et al., 2009; Chung et al., 2016; Gabande-Rodriguez et al., 2014). In a mouse model of type C disease, genetic deletion of cystatin b, an endogenous inhibitor of lysosomal cathepsins, was found to trigger apoptosis and markedly enhance neurodegeneration (Chung et al., 2016). These findings suggest that damage to lysosomes and leakage of their contents into the cytosol of neurons is an important component of neuropathology, and one that needs to be considered when developing therapies designed to target the lysosomal quality control pathways.
Potential strategies to target quality control pathways
The growing knowledge of Niemann-Pick disease pathogenesis and the importance of quality control pathways in regulating folding and trafficking of disease-causing missense mutants, such as NPC1-I1061T, have prompted studies to identify novel therapeutic approaches. These efforts are distinct from those aimed at developing cholesterol removing agents such as hydroxypropyl-beta-cyclodextrin (cyclodextrin). Systemic administration of cyclodextrin reduces peripheral organ lipid storage but low blood-brain barrier permeability hinders central nervous system correction (Liu et al., 2009; Ramirez et al., 2010). Direct central nervous system administration of cyclodextrin corrects neurological phenotypes (Aqul et al., 2011; Vite et al., 2015) but results in severe hearing loss (Ward et al., 2010). This toxicity, along with the challenges associated with intrathecal administration of the drug to NPC patients, spurred investigations of alternative therapeutics (overview in Table 2). Here we summarize emerging approaches and targets for modulating NPC lipid storage through autophagy or correcting NPC1 folding and trafficking as paths to therapy development.
Table 2
Overview of potential NPC therapeutics discussed in this review
Therapeutic | Potential Mechanism of Action | Status |
---|---|---|
Cyclodextrin | Directly removes cholesterol | In clinical trial |
Chlorpromazine | Induction of autophagy | In vitro |
rHSP70 | Prevents lysosomal membrane permeability; stabilizes NPC1 folding | In vitro |
Geranylgeranylacetone | Induces Hsp70 expression | In vitro |
Arimoclomol | Induces Hsp70 expression | Entering clinical trial |
SAHA | Histone deacetylase inhibitor | In clinical trial |
Oxysterols | Chemical chaperone to stabilize NPC1 folding | In vitro |
Autophagy induction
It has been proposed that many neurological diseases could be improved by the induction of macroautophagy (Rubinsztein et al., 2007). This notion is perhaps most strongly supported by studies of age-dependent protein aggregation disorders, in which cytosolic protein aggregates are cleared through this pathway. For many lysosomal storage disorders, the effects of autophagy induction are more difficult to predict because of demonstrated impairments in autophagic flux. Nonetheless, if alternative pathways exist to facilitate clearance of the accumulating substrate, if lysosomal exocytosis is beneficial, or if lysosomal localization of a therapeutic is required for its activity, then modulating this pathway could be beneficial.
An example of the complexities of targeting autophagy in lysosomal diseases is provided by work on Pompe disease, a glycogen storage disorder caused by deficiency of acid alpha-glucosidase (GAA), a soluble enzyme that converts glycogen into glucose. Enzyme replacement therapy (ERT) partially ameliorates the Pompe phenotype in a null mouse model, with a robust rescue in cardiac muscle but a poor response in skeletal muscle (Fukuda et al., 2006). This correlates with an accumulation of autophagic structures in skeletal muscle due to impairment of autophagosome-lysosome fusion, and entrapment of recombinant enzyme in autophagosomes following ERT (reviewed in (Lim et al., 2014)). Strikingly, genetic disruption of autophagosome formation in skeletal muscle by cell specific deletion of Atg5 or Atg7 improves the response to ERT (Raben et al., 2010). In contrast, analysis of muscle biopsies from subjects with milder, childhood/juvenile Pompe disease indicates that autophagic flux is important for successful ERT, perhaps by affecting GAA maturation (Nascimbeni et al., 2012). These data suggest that the extent of lysosomal dysfunction may vary with disease severity and thereby impact the therapeutic response (Lim et al., 2014).
The extent to which autophagy induction would be therapeutically beneficial in Niemann-Pick disease animal models or patients remains uncertain. Studies in cellular models have suggested that low dose cyclodextrin in combination with an autophagy inducer may be beneficial in overcoming defects in autophagic flux and clearing cholesterol storage (Sarkar et al., 2013). Alternatively, activation of minor pathways that bypass loss of NPC1 function may provide an avenue to therapeutics. For example, NPC1 interacts with and transfers cholesterol to the cytoplasmic ER linker protein ORP5 (Yu et al., 2014b). Interestingly, deletion of ORP5 in cells phenocopies NPC1 deficiency by triggering the accumulation of unesterified cholesterol in the lysosome (Du et al., 2011). Although oxysterol binding proteins such as ORP5 work directly with NPC1, it was found that an interaction of RIDα and ORP5 could bypass NPC1 function in the presence of NPC2 (Cianciola et al., 2013). The RIDα/ORP5 pathway appears to be a minor route for cholesterol egress from lysosomes, but its modulation could prove to be therapeutically relevant.
Evidence for a contribution of autophagy defects to the systemic pathology that arises in Niemann-Pick type C patients was recently provided. Schwerd et al studied a cohort of type C patients who developed inflammatory bowel disease and suggested a critical role for impaired intracellular bacterial clearance (Schwerd et al., 2016). Macrophages treated with U18666A, a small molecule that triggers lipid accumulation in late endosomes and lysosomes, exhibit decreased ability to clear bacteria (Schwerd et al., 2016). While cyclodextrin did not correct bacterial handling, treatment with the autophagy activator chlorpromazine restored bacterial clearance in U18666A-treated macrophages (Schwerd et al., 2016). Since U18666A was recently shown to directly bind to and inhibit NPC1 (Lu et al., 2015), differential effects of cyclodextrin and chlorpromazine suggest defects in autophagy may be upstream of cholesterol accumulation. Surprisingly, other autophagy activators such as rapamycin or trehalose did not correct bacterial clearance (Schwerd et al., 2016). Exactly how chlorpromazine upregulates autophagy is not known, but in macrophages chlorpromazine is well described as an inhibitor of clathrin-dependent endocytosis and phagocytosis (Elferink, 1979; Hussain et al., 2011). Therefore, chlorpromazine could function both by reducing bacterial uptake and by activating autophagy to degrade the bacteria that have invaded macrophages. The extent to which this strategy can be used to alleviate symptoms in type C patients is an important question that requires further investigation.
Hsc/Hsp70 targeted approaches
The molecular chaperones of the Hsp70 family have emerged as potential therapeutic targets based on their role in quality control pathways that regulate triage decisions which determine whether mutant proteins are either (re)folded or targeted for degradation. Exposed hydrophobic residues are bound by Hsc/Hsp70 to facilitate (re)folding, and if this is unsuccessful, recruit ubiquitination machinery. Although Hsc70 and Hsp70 have high sequence homology, Hsc70 is constitutively expressed while Hsp70 expression is induced by an HSF1 (heat shock transcription factor 1) dependent heat shock response. The potential role of Hsp70s in NPC1 quality control is highlighted by their interactions with WT and I1061T protein as well as with CHIP (Nakasone et al., 2014). Most frequently, Hsc/Hsp70 work on substrates identically, promoting either folding or degradation; however, a recent study suggests that Hsc70 may promote degradation of NPC1 through the proteasome while Hsp70 stabilizes it (Nakasone et al., 2014). Data indicating that the Hsp70s play an important role in the quality control of NPC1 indicate that modulating their expression or activity may be a viable therapeutic approach.
Hsp70 has also attracted attention because of its potential role in stabilizing lysosomal membranes. Hsp70 that has trafficked to the lumen of lysosomes prevents cathepsin release and cell death (Nylandsted et al., 2004). Supporting the protective role of Hsp70 in the lysosome are data from cellular models of Niemann-Pick type A and B disease. Recombinant Hsp70 has been shown to bind lysosomal bis(monoacylglycero)phosphate, stabilize lysosomal membranes, and reduce sphingomyelin levels by ~20% (Kirkegaard et al., 2010). Studies indicating that lysosomal membrane permeabilization is also a component of neurodegeneration in type C disease (Amritraj et al., 2009; Chung et al., 2016) suggest that recombinant Hsp70 may be broadly beneficial to Niemann-Pick patients. Interestingly, an isoform of Hsp70, ly-hsc73, is predominantly localized to the lysosome. In addition to having a pI of 5.3 and enhanced lysosomal stability, serum starvation readily mobilizes ly-hsc73 to the lysosome, where it facilitates protein degradation (Agarraberes et al., 1997). Whether over-expression of the ly-hsc73 isoform is effective in alleviating Niemann-Pick cellular phenotypes is not known.
Small molecules targeting Hsp70 function or expression have been generated and recently demonstrated to have efficacy in models of protein aggregation disorders. These compounds variably function as allosteric agents that favor the ADP-bound conformation of Hsp70 or by upregulating Hsp70 expression (Katsuno et al., 2005; Wang et al., 2013). Interestingly, a small molecule inducer of Hsp70, geranylgeranylacetone, increases NPC1-I1061T protein levels and trafficking, and reduces cholesterol accumulation in a cellular model of type C disease (Nakasone et al., 2014). A similar mechanism of action has been suggested for arimoclomol. This drug is an analog of bimiclomol, an inducer of heat shock proteins which has been tested in mouse models of amyotrophic lateral sclerosis (Kieran et al., 2004) and spinal and bulbar muscular atrophy (Malik et al., 2013). Although there are no published preclinical data suggesting efficacy of arimoclomol in models of Niemann-Pick disease, it has been approved for a phase 1 clinical trial in type C patients (NCT02612129). Notably, it is uncertain whether chronic activation of an HSF1-dependent stress response would have untoward effects, although such a possibility has been suggested to underlie a maladaptive stress response that exacerbates disease states (Lamech and Haynes, 2015).
Histone deacetylase inhibitors
Histone deacetylase inhibitors (HDACi) are a class of small molecules that have broad effects on gene expression by inhibiting the enzymes that deacetylate histones and other proteins. The acetylation of histones on lysine residues diminishes chromatin compaction and increases gene expression, a state that is favored by inhibiting the enzymes that remove these modifications. Initial reports indicated that valproic acid, a broad spectrum HDACi, diminishes cholesterol accumulation in NPC1 null neural stem cells (Kim et al., 2007; Pipalia et al., 2011; Yang et al., 2006). Subsequent genetic and small molecule screens corroborated the beneficial effects of HDACi in cellular models of Niemann-Pick type C disease (Munkacsi et al., 2011; Pipalia et al., 2011; Praggastis et al., 2015; Wehrmann et al., 2012). These studies and additional reports showed that HDACi were effective at reducing lipid storage in NPC1 deficient cells. Moreover, in models expressing NPC1-I1061T, these compounds increased steady-state protein levels and promoted proper trafficking of the mutant protein (Munkacsi et al., 2011; Pipalia et al., 2011; Praggastis et al., 2015; Wehrmann et al., 2012). Taken together, these reports demonstrate that HDACi have significant beneficial effects on promoting folding and restoring function of NPC1-I1061T, however the mechanism underlying this activity is poorly understood.
The HDACi that has been most intensively studied in models of Niemann-Pick type C disease is SAHA (suberanilohydroxamic acid), also known as vorinostat. SAHA has shown efficacy in yeast models and type C patient fibroblasts (Munkacsi et al., 2011; Pipalia et al., 2011; Praggastis et al., 2015; Wehrmann et al., 2012), but not in induced neural stem cells derived from NPC1-I1061T compound heterozygous patient fibroblasts (Yu et al., 2014a). This observation raises the possibility that SAHA’s efficacy might be cell type dependent. Recent work has demonstrated therapeutic benefit from SAHA in Npc1nmf164 mice, an animal model that contains an Npc1 missense allele. Treatment with SAHA alone failed to improve animal survival suggesting poor blood-brain barrier penetration (Alam et al., 2016). However, complexing SAHA with cyclodextrin and polyethylene glycol enhanced access of the drug to the CNS, promoted survival, delayed behavioral abnormalities, and reduced Purkinje cell death (Alam et al., 2016). These changes coincided with increased Npc1 mRNA and protein levels (Alam et al., 2016). An ongoing phase 1/2 clinical trial (NCT02124083) of SAHA in adult Niemann-Pick type C patients may provide an indication of the likelihood that this drug will be beneficial to patients.
Chemical chaperones
Chemical chaperones are small molecules that promote folding by binding to a protein’s active site to stabilize its native state. Because of their small size, many chemical chaperones cross the blood brain barrier, making them especially useful for the treatment of neurological diseases (Schultz et al., 2011). Chemical chaperones are in development for a variety of lysosomal diseases including Tay-Sachs, gangliosidosis (Maegawa et al., 2007; Suzuki, 2008) and Gaucher disease (Sawkar et al., 2005). These successes have prompted investigators in the Niemann-Pick field to actively pursue this strategy for therapy development, taking advantage of the cholesterol binding site in NPC1 to identify potential chemical chaperones.
Work on chemical chaperones has focused on oxygenated derivatives of cholesterol, or oxysterols. These molecules have diverse roles in cholesterol metabolism, as reviewed in (Schroepfer, 2000), and have emerged as biomarkers in type C disease (Jiang et al., 2011; Porter et al., 2010). The oxysterol 25-hydroxycholesterol (25-HC) out-competes cholesterol for binding to the NPC1 NTD (Infante et al., 2008a; Infante et al., 2008b; Kwon et al., 2009). Based on the knowledge that 25-HC strongly binds NPC1, 25-HC was used as a chemical chaperone to modulate folding of the mutant protein. Treatment of cells with 25-HC was found to correct NPC1-I1061T trafficking to the lysosomal compartment and rescue lipid accumulation in a dose-dependent manner (Ohgane et al., 2013). One concern is that high doses of oxysterol increased cholesterol accumulation in cells, suggesting that they may be inhibitory to NPC1 function (Ohgane et al., 2013). More potent (40 fold) derivatives of oxysterols have been discovered utilizing high content NPC1-I1061T trafficking assays (Ohgane et al., 2014), but care is warranted to ensure that oxysterol-derivative binding is reversible.
Conclusions
Significant advances in our understanding of Niemann-Pick disease have provided critical insights into the mechanisms regulating intracellular cholesterol trafficking. Moreover, the consequences of disease-causing mutations in NPC1 have highlighted the important role of lipid trafficking in lysosomal quality control pathways and membrane stability. Recent characterization of the mechanisms regulating the folding, trafficking and degradation of NPC1 encoded by missense alleles provides insights into previously unanticipated targets for therapeutic intervention. Despite these advances, our knowledge of Niemann-Pick disease pathogenesis is rudimentary in many areas, and critical questions related to basic cell biology and therapeutic strategies remain unanswered. It is our expectation that on-going work in Niemann-Pick and related lysosomal diseases will continue to yield advances in our understanding of pathogenic mechanisms so that rational strategies for treatment can be developed.
Acknowledgments
We are greatful to Kayla Capper for creating the scientific illustration. Supported by the National Institutes of Health (T32 NS007222 to M.L.S. and R01 NS063967 to A.P.L.).
References
- Agarraberes FA, Terlecky SR, Dice JF. An intralysosomal hsp70 is required for a selective pathway of lysosomal protein degradation. J Cell Biol. 1997;137:825–34. [Europe PMC free article] [Abstract] [Google Scholar]
- Alam MS, Getz M, Haldar K. Chronic administration of an HDAC inhibitor treats both neurological and systemic Niemann-Pick type C disease in a mouse model. Sci Transl Med. 2016;8:326ra23. [Abstract] [Google Scholar]
- Amritraj A, et al. Increased activity and altered subcellular distribution of lysosomal enzymes determine neuronal vulnerability in Niemann-Pick type C1-deficient mice. Am J Pathol. 2009;175:2540–56. [Europe PMC free article] [Abstract] [Google Scholar]
- Aqul A, et al. Unesterified cholesterol accumulation in late endosomes/lysosomes causes neurodegeneration and is prevented by driving cholesterol export from this compartment. J Neurosci. 2011;31:9404–13. [Europe PMC free article] [Abstract] [Google Scholar]
- Brady RO, et al. The metabolism of sphingomyelin. II. Evidence of an enzymatic deficiency in Niemann-Pick diseae. Proc Natl Acad Sci U S A. 1966;55:366–9. [Europe PMC free article] [Abstract] [Google Scholar]
- Carstea ED, et al. Niemann-Pick C1 disease gene: homology to mediators of cholesterol homeostasis. Science. 1997;277:228–31. [Abstract] [Google Scholar]
- Chung C, et al. Genetic and pharmacological evidence implicate cathepsins in Niemann-Pick C cerebellar degeneration. Human Molecular Genetics 2016 [Europe PMC free article] [Abstract] [Google Scholar]
- Cianciola NL, et al. Adenovirus RIDalpha uncovers a novel pathway requiring ORP1L for lipid droplet formation independent of NPC1. Mol Biol Cell. 2013;24:3309–25. [Europe PMC free article] [Abstract] [Google Scholar]
- Davies JP, Ioannou YA. Topological analysis of Niemann-Pick C1 protein reveals that the membrane orientation of the putative sterol-sensing domain is identical to those of 3-hydroxy-3-methylglutaryl-CoA reductase and sterol regulatory element binding protein cleavage-activating protein. J Biol Chem. 2000;275:24367–74. [Abstract] [Google Scholar]
- Du X, et al. A role for oxysterol-binding protein-related protein 5 in endosomal cholesterol trafficking. J Cell Biol. 2011;192:121–35. [Europe PMC free article] [Abstract] [Google Scholar]
- Efthymiou AG, et al. Rescue of an in vitro neuron phenotype identified in Niemann-Pick disease, type C1 induced pluripotent stem cell-derived neurons by modulating the WNT pathway and calcium signaling. Stem Cells Transl Med. 2015;4:230–8. [Europe PMC free article] [Abstract] [Google Scholar]
- Elferink JG. Chlorpromazine inhibits phagocytosis and exocytosis in rabbit polymorphonuclear leukocytes. Biochem Pharmacol. 1979;28:965–8. [Abstract] [Google Scholar]
- Ellgaard L, Helenius A. Quality control in the endoplasmic reticulum. Nat Rev Mol Cell Biol. 2003;4:181–91. [Abstract] [Google Scholar]
- Elrick MJ, et al. Conditional Niemann-Pick C mice demonstrate cell autonomous Purkinje cell neurodegeneration. Hum Mol Genet. 2010;19:837–47. [Europe PMC free article] [Abstract] [Google Scholar]
- Elrick MJ, et al. Impaired proteolysis underlies autophagic dysfunction in Niemann-Pick type C disease. Hum Mol Genet. 2012;21:4876–87. [Europe PMC free article] [Abstract] [Google Scholar]
- Ferguson SM. Beyond indigestion: emerging roles for lysosome-based signaling in human disease. Curr Opin Cell Biol. 2015;35:59–68. [Europe PMC free article] [Abstract] [Google Scholar]
- Fukuda T, et al. Autophagy and mistargeting of therapeutic enzyme in skeletal muscle in Pompe disease. Mol Ther. 2006;14:831–9. [Europe PMC free article] [Abstract] [Google Scholar]
- Gabande-Rodriguez E, et al. High sphingomyelin levels induce lysosomal damage and autophagy dysfunction in Niemann Pick disease type A. Cell Death Differ. 2014;21:864–75. [Europe PMC free article] [Abstract] [Google Scholar]
- Gelsthorpe ME, et al. Niemann-Pick type C1 I1061T mutant encodes a functional protein that is selected for endoplasmic reticulum-associated degradation due to protein misfolding. J Biol Chem. 2008;283:8229–36. [Europe PMC free article] [Abstract] [Google Scholar]
- Higgins JJ, et al. A clinical staging classification for type C Niemann-Pick disease. Neurology. 1992;42:2286–90. [Abstract] [Google Scholar]
- Houck SA, et al. Quality control autophagy degrades soluble ERAD-resistant conformers of the misfolded membrane protein GnRHR. Mol Cell. 2014;54:166–79. [Europe PMC free article] [Abstract] [Google Scholar]
- Hussain KM, et al. The essential role of clathrin-mediated endocytosis in the infectious entry of human enterovirus 71. J Biol Chem. 2011;286:309–21. [Europe PMC free article] [Abstract] [Google Scholar]
- Infante RE, et al. Purified NPC1 protein: II. Localization of sterol binding to a 240-amino acid soluble luminal loop. J Biol Chem. 2008a;283:1064–75. [Abstract] [Google Scholar]
- Infante RE, et al. NPC2 facilitates bidirectional transfer of cholesterol between NPC1 and lipid bilayers, a step in cholesterol egress from lysosomes. Proc Natl Acad Sci U S A. 2008b;105:15287–92. [Europe PMC free article] [Abstract] [Google Scholar]
- Jiang X, et al. A sensitive and specific LC-MS/MS method for rapid diagnosis of Niemann-Pick C1 disease from human plasma. J Lipid Res. 2011;52:1435–45. [Europe PMC free article] [Abstract] [Google Scholar]
- Katsuno M, et al. Pharmacological induction of heat-shock proteins alleviates polyglutamine-mediated motor neuron disease. Proc Natl Acad Sci U S A. 2005;102:16801–6. [Abstract] [Google Scholar]
- Khaminets A, et al. Regulation of endoplasmic reticulum turnover by selective autophagy. Nature. 2015;522:354–8. [Abstract] [Google Scholar]
- Kieran D, et al. Treatment with arimoclomol, a coinducer of heat shock proteins, delays disease progression in ALS mice. Nat Med. 2004;10:402–5. [Abstract] [Google Scholar]
- Kim SJ, et al. Defective cholesterol traffic and neuronal differentiation in neural stem cells of Niemann-Pick type C disease improved by valproic acid, a histone deacetylase inhibitor. Biochem Biophys Res Commun. 2007;360:593–9. [Abstract] [Google Scholar]
- Kirkegaard T, et al. Hsp70 stabilizes lysosomes and reverts Niemann-Pick disease-associated lysosomal pathology. Nature. 2010;463:549–53. [Abstract] [Google Scholar]
- Ko DC, et al. Cell-autonomous death of cerebellar purkinje neurons with autophagy in Niemann-Pick type C disease. PLoS Genet. 2005;1:81–95. [Europe PMC free article] [Abstract] [Google Scholar]
- Kwon HJ, et al. Structure of N-terminal domain of NPC1 reveals distinct subdomains for binding and transfer of cholesterol. Cell. 2009;137:1213–24. [Europe PMC free article] [Abstract] [Google Scholar]
- Lamech LT, Haynes CM. The unpredictability of prolonged activation of stress response pathways. J Cell Biol. 2015;209:781–7. [Europe PMC free article] [Abstract] [Google Scholar]
- Liao G, et al. Cholesterol accumulation is associated with lysosomal dysfunction and autophagic stress in Npc1 −/− mouse brain. Am J Pathol. 2007;171:962–75. [Europe PMC free article] [Abstract] [Google Scholar]
- Lieberman AP, et al. Autophagy in lysosomal storage disorders. Autophagy. 2012;8:719–30. [Europe PMC free article] [Abstract] [Google Scholar]
- Lim JA, Li L, Raben N. Pompe disease: from pathophysiology to therapy and back again. Front Aging Neurosci. 2014;6:177. [Europe PMC free article] [Abstract] [Google Scholar]
- Lipatova Z, Segev N. A Role for Macro-ER-Phagy in ER Quality Control. PLoS Genet. 2015;11:e1005390. [Europe PMC free article] [Abstract] [Google Scholar]
- Liu B, et al. Reversal of defective lysosomal transport in NPC disease ameliorates liver dysfunction and neurodegeneration in the npc1−/− mouse. Proc Natl Acad Sci U S A. 2009;106:2377–82. [Europe PMC free article] [Abstract] [Google Scholar]
- Lloyd-Evans E, et al. Niemann-Pick disease type C1 is a sphingosine storage disease that causes deregulation of lysosomal calcium. Nat Med. 2008;14:1247–55. [Abstract] [Google Scholar]
- Loftus SK, et al. Rescue of neurodegeneration in Niemann-Pick C mice by a prion-promoter-driven Npc1 cDNA transgene. Hum Mol Genet. 2002;11:3107–14. [Abstract] [Google Scholar]
- Lopez ME, et al. Anatomically defined neuron-based rescue of neurodegenerative Niemann-Pick type C disorder. J Neurosci. 2011;31:4367–78. [Europe PMC free article] [Abstract] [Google Scholar]
- Lu F, et al. Identification of NPC1 as the target of U18666A, an inhibitor of lysosomal cholesterol export and Ebola infection. Elife. 2015:4. [Europe PMC free article] [Abstract] [Google Scholar]
- Maegawa GH, et al. Pyrimethamine as a potential pharmacological chaperone for late-onset forms of GM2 gangliosidosis. J Biol Chem. 2007;282:9150–61. [Europe PMC free article] [Abstract] [Google Scholar]
- Maetzel D, et al. Genetic and chemical correction of cholesterol accumulation and impaired autophagy in hepatic and neural cells derived from Niemann-Pick Type C patient-specific iPS cells. Stem Cell Reports. 2014;2:866–80. [Europe PMC free article] [Abstract] [Google Scholar]
- Malik B, et al. Co-induction of the heat shock response ameliorates disease progression in a mouse model of human spinal and bulbar muscular atrophy: implications for therapy. Brain. 2013;136:926–43. [Europe PMC free article] [Abstract] [Google Scholar]
- Micsenyi MC, et al. Lysosomal membrane permeability stimulates protein aggregate formation in neurons of a lysosomal disease. J Neurosci. 2013;33:10815–27. [Europe PMC free article] [Abstract] [Google Scholar]
- Mochida K, et al. Receptor-mediated selective autophagy degrades the endoplasmic reticulum and the nucleus. Nature. 2015;522:359–62. [Abstract] [Google Scholar]
- Morris JA, et al. The genomic organization and polymorphism analysis of the human Niemann-Pick C1 gene. Biochem Biophys Res Commun. 1999;261:493–8. [Abstract] [Google Scholar]
- Munkacsi AB, et al. An “exacerbate-reverse” strategy in yeast identifies histone deacetylase inhibition as a correction for cholesterol and sphingolipid transport defects in human Niemann-Pick type C disease. J Biol Chem. 2011;286:23842–51. [Europe PMC free article] [Abstract] [Google Scholar]
- Nakasone N, et al. Endoplasmic reticulum-associated degradation of Niemann-Pick C1: evidence for the role of heat shock proteins and identification of lysine residues that accept ubiquitin. J Biol Chem. 2014;289:19714–25. [Europe PMC free article] [Abstract] [Google Scholar]
- Nascimbeni AC, et al. Impaired autophagy contributes to muscle atrophy in glycogen storage disease type II patients. Autophagy. 2012;8:1697–700. [Europe PMC free article] [Abstract] [Google Scholar]
- Naureckiene S, et al. Identification of HE1 as the second gene of Niemann-Pick C disease. Science. 2000;290:2298–301. [Abstract] [Google Scholar]
- Nylandsted J, et al. Heat shock protein 70 promotes cell survival by inhibiting lysosomal membrane permeabilization. J Exp Med. 2004;200:425–35. [Europe PMC free article] [Abstract] [Google Scholar]
- Ohgane K, et al. Discovery of oxysterol-derived pharmacological chaperones for NPC1: implication for the existence of second sterol-binding site. Chem Biol. 2013;20:391–402. [Abstract] [Google Scholar]
- Ohgane K, et al. Structure-activity relationships of oxysterol-derived pharmacological chaperones for Niemann-Pick type C1 protein. Bioorg Med Chem Lett. 2014;24:3480–5. [Abstract] [Google Scholar]
- Ohsaki Y, et al. Cholesterol depletion facilitates ubiquitylation of NPC1 and its association with SKD1/Vps4. J Cell Sci. 2006;119:2643–53. [Abstract] [Google Scholar]
- Ordonez MP, et al. Disruption and therapeutic rescue of autophagy in a human neuronal model of Niemann Pick type C1. Hum Mol Genet. 2012;21:2651–62. [Europe PMC free article] [Abstract] [Google Scholar]
- Pacheco CD, Lieberman AP. Lipid trafficking defects increase Beclin-1 and activate autophagy in Niemann-Pick type C disease. Autophagy. 2007;3:487–9. [Abstract] [Google Scholar]
- Pentchev PG, et al. A defect in cholesterol esterification in Niemann-Pick disease (type C) patients. Proc Natl Acad Sci U S A. 1985;82:8247–51. [Europe PMC free article] [Abstract] [Google Scholar]
- Pentchev PG. Niemann-Pick C research from mouse to gene. Biochim Biophys Acta. 2004;1685:3–7. [Abstract] [Google Scholar]
- Pipalia NH, et al. Histone deacetylase inhibitor treatment dramatically reduces cholesterol accumulation in Niemann-Pick type C1 mutant human fibroblasts. Proc Natl Acad Sci U S A. 2011;108:5620–5. [Europe PMC free article] [Abstract] [Google Scholar]
- Porter FD, et al. Cholesterol oxidation products are sensitive and specific blood-based biomarkers for Niemann-Pick C1 disease. Sci Transl Med. 2010;2:56ra81. [Europe PMC free article] [Abstract] [Google Scholar]
- Praggastis M, et al. A murine Niemann-Pick C1 I1061T knock-in model recapitulates the pathological features of the most prevalent human disease allele. J Neurosci. 2015;35:8091–106. [Europe PMC free article] [Abstract] [Google Scholar]
- Raben N, et al. Suppression of autophagy permits successful enzyme replacement therapy in a lysosomal storage disorder--murine Pompe disease. Autophagy. 2010;6:1078–89. [Europe PMC free article] [Abstract] [Google Scholar]
- Ramirez CM, et al. Weekly cyclodextrin administration normalizes cholesterol metabolism in nearly every organ of the Niemann-Pick type C1 mouse and markedly prolongs life. Pediatr Res. 2010;68:309–15. [Europe PMC free article] [Abstract] [Google Scholar]
- Ribeiro I, et al. Niemann-Pick type C disease: NPC1 mutations associated with severe and mild cellular cholesterol trafficking alterations. Hum Genet. 2001;109:24–32. [Abstract] [Google Scholar]
- Rubinsztein DC, et al. Potential therapeutic applications of autophagy. Nat Rev Drug Discov. 2007;6:304–12. [Abstract] [Google Scholar]
- Sarkar S, et al. Impaired autophagy in the lipid-storage disorder Niemann-Pick type C1 disease. Cell Rep. 2013;5:1302–15. [Europe PMC free article] [Abstract] [Google Scholar]
- Sawkar AR, et al. Gaucher disease-associated glucocerebrosidases show mutation-dependent chemical chaperoning profiles. Chem Biol. 2005;12:1235–44. [Abstract] [Google Scholar]
- Schroepfer GJ., Jr Oxysterols: modulators of cholesterol metabolism and other processes. Physiol Rev. 2000;80:361–554. [Abstract] [Google Scholar]
- Schultz ML, et al. Clarifying lysosomal storage diseases. Trends Neurosci. 2011;34:401–10. [Europe PMC free article] [Abstract] [Google Scholar]
- Schwerd T, et al. Impaired antibacterial autophagy links granulomatous intestinal inflammation in Niemann-Pick disease type C1 and XIAP deficiency with NOD2 variants in Crohn’s disease. Gut 2016 [Europe PMC free article] [Abstract] [Google Scholar]
- Shen D, et al. Lipid storage disorders block lysosomal trafficking by inhibiting a TRP channel and lysosomal calcium release. Nat Commun. 2012;3:731. [Europe PMC free article] [Abstract] [Google Scholar]
- Suzuki Y. Chemical chaperone therapy for GM1-gangliosidosis. Cell Mol Life Sci. 2008;65:351–3. [Abstract] [Google Scholar]
- Vanier MT, et al. Genetic heterogeneity in Niemann-Pick C disease: a study using somatic cell hybridization and linkage analysis. Am J Hum Genet. 1996;58:118–25. [Europe PMC free article] [Abstract] [Google Scholar]
- Vanier MT, Millat G. Niemann-Pick disease type C. Clin Genet. 2003;64:269–81. [Abstract] [Google Scholar]
- Vanier MT. Niemann-Pick disease type C. Orphanet J Rare Dis. 2010;5:16. [Europe PMC free article] [Abstract] [Google Scholar]
- Vite CH, et al. Intracisternal cyclodextrin prevents cerebellar dysfunction and Purkinje cell death in feline Niemann-Pick type C1 disease. Sci Transl Med. 2015;7:276ra26. [Europe PMC free article] [Abstract] [Google Scholar]
- Wang AM, et al. Activation of Hsp70 reduces neurotoxicity by promoting polyglutamine protein degradation. Nat Chem Biol. 2013;9:112–8. [Europe PMC free article] [Abstract] [Google Scholar]
- Wang ML, et al. Identification of surface residues on Niemann-Pick C2 essential for hydrophobic handoff of cholesterol to NPC1 in lysosomes. Cell Metab. 2010;12:166–73. [Europe PMC free article] [Abstract] [Google Scholar]
- Ward S, et al. 2-hydroxypropyl-beta-cyclodextrin raises hearing threshold in normal cats and in cats with Niemann-Pick type C disease. Pediatr Res. 2010;68:52–6. [Europe PMC free article] [Abstract] [Google Scholar]
- Wehrmann ZT, et al. Quantitative comparison of the efficacy of various compounds in lowering intracellular cholesterol levels in Niemann-Pick type C fibroblasts. PLoS One. 2012;7:e48561. [Europe PMC free article] [Abstract] [Google Scholar]
- Yamamoto T, et al. Genotype-phenotype relationship of Niemann-Pick disease type C: a possible correlation between clinical onset and levels of NPC1 protein in isolated skin fibroblasts. J Med Genet. 2000;37:707–12. [Europe PMC free article] [Abstract] [Google Scholar]
- Yang SR, et al. NPC1 gene deficiency leads to lack of neural stem cell self-renewal and abnormal differentiation through activation of p38 mitogen-activated protein kinase signaling. Stem Cells. 2006;24:292–8. [Abstract] [Google Scholar]
- Yoshimori T, et al. Bafilomycin A1, a specific inhibitor of vacuolar-type H(+)-ATPase, inhibits acidification and protein degradation in lysosomes of cultured cells. J Biol Chem. 1991;266:17707–12. [Abstract] [Google Scholar]
- Yu D, et al. Niemann-Pick Disease Type C: Induced Pluripotent Stem Cell-Derived Neuronal Cells for Modeling Neural Disease and Evaluating Drug Efficacy. J Biomol Screen. 2014a;19:1164–1173. [Europe PMC free article] [Abstract] [Google Scholar]
- Yu T, et al. Temporal and cell-specific deletion establishes that neuronal Npc1 deficiency is sufficient to mediate neurodegeneration. Hum Mol Genet. 2011;20:4440–51. [Europe PMC free article] [Abstract] [Google Scholar]
- Yu T, et al. Ryanodine receptor antagonists adapt NPC1 proteostasis to ameliorate lipid storage in Niemann-Pick type C disease fibroblasts. Hum Mol Genet. 2012;21:3205–14. [Europe PMC free article] [Abstract] [Google Scholar]
- Yu T, Lieberman AP. Npc1 acting in neurons and glia is essential for the formation and maintenance of CNS myelin. PLoS Genet. 2013;9:e1003462. [Europe PMC free article] [Abstract] [Google Scholar]
- Yu XH, et al. NPC1, intracellular cholesterol trafficking and atherosclerosis. Clin Chim Acta. 2014b;429:69–75. [Abstract] [Google Scholar]
Full text links
Read article at publisher's site: https://doi.org/10.1016/j.brainres.2016.03.035
Read article for free, from open access legal sources, via Unpaywall:
https://europepmc.org/articles/pmc5542880?pdf=render
Citations & impact
Impact metrics
Citations of article over time
Alternative metrics
Smart citations by scite.ai
Explore citation contexts and check if this article has been
supported or disputed.
https://scite.ai/reports/10.1016/j.brainres.2016.03.035
Article citations
Overexpression of long noncoding RNA DUXAP8 inhibits ER-phagy through activating AKT/mTOR signaling and contributes to preeclampsia.
Cell Mol Life Sci, 81(1):336, 09 Aug 2024
Cited by: 0 articles | PMID: 39120751 | PMCID: PMC11335266
The cholesterol transporter NPC1 is essential for epigenetic regulation and maturation of oligodendrocyte lineage cells.
Nat Commun, 14(1):3964, 05 Jul 2023
Cited by: 4 articles | PMID: 37407594 | PMCID: PMC10322873
Modified 5-aminolevulinic acid photodynamic therapy suppresses cutaneous squamous cell carcinoma through blocking Akt/mTOR-mediated autophagic flux.
Front Pharmacol, 14:1114678, 17 Mar 2023
Cited by: 4 articles | PMID: 37007013 | PMCID: PMC10063783
Lysosomal positioning diseases: beyond substrate storage.
Open Biol, 12(10):220155, 26 Oct 2022
Cited by: 11 articles | PMID: 36285443 | PMCID: PMC9597170
Review Free full text in Europe PMC
Species-specific differences in NPC1 protein trafficking govern therapeutic response in Niemann-Pick type C disease.
JCI Insight, 7(23):e160308, 08 Dec 2022
Cited by: 3 articles | PMID: 36301667 | PMCID: PMC9746915
Go to all (26) article citations
Data
Data behind the article
This data has been text mined from the article, or deposited into data resources.
BioStudies: supplemental material and supporting data
Clinical Trials (2)
- (1 citation) ClinicalTrials.gov - NCT02124083
- (1 citation) ClinicalTrials.gov - NCT02612129
Similar Articles
To arrive at the top five similar articles we use a word-weighted algorithm to compare words from the Title and Abstract of each citation.
Histone deacetylase inhibitors correct the cholesterol storage defect in most Niemann-Pick C1 mutant cells.
J Lipid Res, 58(4):695-708, 13 Feb 2017
Cited by: 37 articles | PMID: 28193631 | PMCID: PMC5392745
High-content screen for modifiers of Niemann-Pick type C disease in patient cells.
Hum Mol Genet, 27(12):2101-2112, 01 Jun 2018
Cited by: 18 articles | PMID: 29659804 | PMCID: PMC5985738
Mutant induced neurons and humanized mice enable identification of Niemann-Pick type C1 proteostatic therapies.
JCI Insight, 9(20):e179525, 29 Aug 2024
Cited by: 0 articles | PMID: 39207850 | PMCID: PMC11530122
Finding pathogenic commonalities between Niemann-Pick type C and other lysosomal storage disorders: Opportunities for shared therapeutic interventions.
Biochim Biophys Acta Mol Basis Dis, 1866(10):165875, 06 Jun 2020
Cited by: 13 articles | PMID: 32522631
Review
Funding
Funders who supported this work.
NINDS NIH HHS (2)
Grant ID: T32 NS007222
Grant ID: R01 NS063967
National Institutes of Health (2)
Grant ID: T32 NS007222
Grant ID: R01 NS063967