Abstract
Background and purpose
The nociceptin/orphanin FQ opioid peptide (NOP) receptor system plays a significant role in the regulation of pain. This system functions differently in the spinal cord and brain. The mechanism by which the NOP receptor agonists regulate pain transmission in these regions is not clearly understood. Here, we investigate the peripheral and spinal NOP receptor distribution and antinociceptive effects of intrathecal nociceptin/orphanin FQ (N/OFQ) in chronic neuropathic pain.Experimental approach
We used immunohistochemistry to determine changes in NOP receptor distribution triggered by spinal nerve ligation (SNL) using NOP-eGFP knock-in mice. Antinociceptive effects of intrathecal N/OFQ on SNL-mediated allodynia and heat/cold hyperalgesia were assessed in wild-type mice.Key results
NOP-eGFP immunoreactivity was decreased by SNL in the spinal laminae I and II outer, regions that mediate noxious heat stimuli. In contrast, immunoreactivity of NOP-eGFP was unchanged in the ventral border of lamina II inner, which is an important region for the development of allodynia. NOP-eGFP expression was also decreased in a large number of primary afferents in the L4 dorsal root ganglion (DRG) of SNL mice. However, SNL mice showed increased sensitivity, compared to sham animals to the effects of i.t administered N/OFQ with respect to mechanical as well as thermal stimuli.Conclusions and implications
Our findings suggest that the spinal NOP receptor system attenuates injury-induced hyperalgesia by direct inhibition of the projection neurons in the spinal cord that send nociceptive signals to the brain and not by inhibiting presynaptic terminals of DRG neurons in the superficial lamina.Free full text

Analysis of the distribution of spinal NOP receptors in a chronic pain model using NOP‐eGFP knock‐in mice
Abstract
Background and Purpose
The nociceptin/orphanin FQ opioid peptide (NOP) receptor system plays a significant role in the regulation of pain. This system functions differently in the spinal cord and brain. The mechanism by which the NOP receptor agonists regulate pain transmission in these regions is not clearly understood. Here, we investigate the peripheral and spinal NOP receptor distribution and antinociceptive effects of intrathecal nociceptin/orphanin FQ (N/OFQ) in chronic neuropathic pain.
Experimental Approach
We used immunohistochemistry to determine changes in NOP receptor distribution triggered by spinal nerve ligation (SNL) using NOP‐eGFP knock‐in mice. Antinociceptive effects of intrathecal N/OFQ on SNL‐mediated allodynia and heat/cold hyperalgesia were assessed in wild‐type mice.
Key Results
NOP‐eGFP immunoreactivity was decreased by SNL in the spinal laminae I and II outer, regions that mediate noxious heat stimuli. In contrast, immunoreactivity of NOP‐eGFP was unchanged in the ventral border of lamina II inner, which is an important region for the development of allodynia. NOP‐eGFP expression was also decreased in a large number of primary afferents in the L4 dorsal root ganglion (DRG) of SNL mice. However, SNL mice showed increased sensitivity, compared to sham animals to the effects of i.t administered N/OFQ with respect to mechanical as well as thermal stimuli.
Conclusions and Implications
Our findings suggest that the spinal NOP receptor system attenuates injury‐induced hyperalgesia by direct inhibition of the projection neurons in the spinal cord that send nociceptive signals to the brain and not by inhibiting presynaptic terminals of DRG neurons in the superficial lamina.
Abbreviations
- DRG
- dorsal root ganglion
- N/OFQ
- nociceptin/orphanin FQ
- NOP receptor
- nociceptin/orphanin FQ opioid receptor
Introduction
The http://www.guidetopharmacology.org/GRAC/ObjectDisplayForward?objectId=320 is the fourth member of opioid receptor family, for which nociceptin/orphanin FQ (http://www.guidetopharmacology.org/GRAC/LigandDisplayForward?ligandId=1681) is the endogenous ligand. This receptor system plays a significant role in diverse biological actions, such as regulation of pain, feeding, and drug and alcohol addictions (Schroder et al., 2014; Witkin et al., 2014; Toll et al., 2016). Interestingly, this receptor system shows both pro‐nociceptive and analgesic actions, which are dependent on the drug dosage, the route of administration and the type of pain stimulus. For example, when N/OFQ is injected i.c.v. into mice, it decreases hot plate escape jumping latency and tail flick latency suggesting a pro‐nociceptive response to radiant heat stimuli (Meunier et al., 1995; Reinscheid et al., 1995). Intracerebroventricular administration of N/OFQ also blocks both stress‐induced and opioid‐mediated antinociception (Grisel et al., 1996). In contrast, spinally injected N/OFQ shows an analgesic response (Tian et al., 1997; Yamamoto et al., 1997; Courteix et al., 2004). Small molecule agonists, such as http://www.guidetopharmacology.org/GRAC/LigandDisplayForward?ligandId=1684 and http://www.guidetopharmacology.org/GRAC/LigandDisplayForward?ligandId=8867), activate NOP receptors in both the brain and spinal cord, producing opposing effects with respect to nociception (Shoblock et al., 2005; Shoblock, 2007; Khroyan et al., 2011). Accordingly, the antinociceptive actions of systemically administered agonists depend upon pharmacokinetics and distribution. Neither selective agonist Ro 64‐6198 nor AT‐202 has antinociceptive activity in the tail flick assay in rats, though Ro 64‐6198 has modest activity in the hot plate assay, which might be due to sedation (Reiss et al., 2008; Toll et al., 2009). When administered systemically, AT‐202 blocks morphine analgesia (Ozawa et al., 2015). In non‐human primates, the effect of systemic administration of Ro 64‐6198 is unclear as one report demonstrated potent antinociceptive activity (Ko et al., 2009) while a second report found systemic administration of Ro 64‐6198 to be ineffective under similar conditions (Saccone et al., 2016). For chronic pain, the situation is different as systemic administration of NOP receptor agonists can block mechanical allodynia in various chronic and inflammatory pain models (Khroyan et al., 2011). The mechanism by which the NOP receptor system mediates these complicated pain‐associated effects and how the circuitry of the NOP receptor system in pain‐related tissues affects pain responses have not been clearly explained due to the lack of appropriate tools to interpret the actions of NOP receptor‐based analgesics at the cellular and neuronal circuitry levels.
To obtain a better understanding of opioid receptor biology, Scherrer et al. (2006) developed a http://www.guidetopharmacology.org/GRAC/ObjectDisplayForward?objectId=317 (delta‐eGFP) knock‐in mouse and determined the location of the receptor in the nervous system without specific δ receptor antibodies, proving that the knock‐in mice are indeed a valuable tool in mapping δ receptors both anatomically and functionally (Bardoni et al., 2014; Pradhan et al., 2015). Similar to these studies, we developed NOP‐eGFP knock‐in mice that express NOP receptors C‐terminally fused to eGFP (Ozawa et al., 2015). Biochemical and electrophysiological studies have proven that the NOP‐eGFP receptor in this mouse strain is functional in vitro. NOP receptor‐mediated inhibition of morphine analgesia was also equivalent in NOP‐eGFP knock‐in and wild‐type mice (Ozawa et al., 2015). Immunohistochemistry demonstrated that NOP‐eGFP receptors were expressed abundantly in the brain regions important to drug abuse and pain, as well as the spinal cord and dorsal root ganglia (DRG) (Ozawa et al., 2015). In the spinal cord, strong NOP‐eGFP immunoreactivity was observed in the specific spinal laminae that are important for the regulation of acute thermal and mechanical pain, injury‐induced allodynia, itch and touch. NOP‐eGFP receptors were present on both peptidergic C nociceptors and non‐peptidergic DRG neurons that are essential for acute heat pain and injury‐induced heat hyperalgesia and for acute mechanical pain respectively. These findings indicated that NOP receptors regulate the function of two major classes of C nociceptors that are critical to heat and mechanical pain modalities.
In this study, using the NOP‐eGFP knock‐in mice, we have investigated changes in the distribution of NOP‐eGFP receptors in the spinal cord and DRG neurons subsequent to spinal nerve ligation (SNL) as a chronic, neuropathic pain model. We also determined the effects of intrathecal N/OFQ on injury‐induced mechanical allodynia, and heat and cold hyperalgesia. The results have led us to discuss the potential spinal pain regulatory mechanism of the N/OFQ‐NOP system during chronic pain.
Methods
Antibodies
We used the following antibodies: rabbit anti‐GFP for DRG neurons (1:1000, Life Technologies, Carlsbad, CA, USA, Cat# A‐11122, RRID: AB_221569); chicken anti‐GFP (1:2500, Abcam, Cambridge, MA, USA, Cat# ab13970, RRID: AB_300798) for spinal cord; sheep anti‐CGRP (http://www.guidetopharmacology.org/GRAC/LigandDisplayForward?ligandId=695, 1:1500, Abcam, Cat# ab22560, RRID: AB_725809); and rabbit anti‐PKCγ, (http://www.guidetopharmacology.org/GRAC/ObjectDisplayForward?objectId=1484, 1:1500, Santa Cruz Biotechnology, Dallas, TX, USA, Cat# sc‐211, RRID: AB_632234). All of the secondary antibodies conjugated to fluorophore were obtained from either Life Technologies (donkey anti‐rabbit IgG Alexa Fluor 555, Cat# A31572, RRID: AB_162543; donkey anti‐sheep IgG Alexa Fluor 555, Cat# A21436, RRID: AB_10376163) or Jackson ImmunoResearch Laboratories (donkey anti‐rabbit IgG Alexa Fluor 488, Cat# 711‐545‐152, RRID: AB_2313584; donkey anti‐chicken IgY Alexa Fluor 488, Cat# 703‐545‐155, RRID: AB_2340375; donkey anti‐sheep IgG Alexa Fluor 647, Cat# 713‐605‐147, RRID: AB_2340751; West Grove, PA, USA).
Animals
All animal care and experimental methods complied with the National Institutes of Health Guide for the Care and Use of Laboratory Animals and were preapproved by the Institutional Animal Care and Use Committee at the Torrey Pines Institute for Molecular Studies (Port St. Lucie, FL, USA). Consistent with these guidelines, ongoing statistical testing of data collected was used to minimize the number of animals used, within the constraints of necessary statistical power. Animal studies are reported in compliance with the ARRIVE guidelines (Kilkenny et al., 2010; McGrath and Lilley, 2015).
Animals were group‐housed (three to five mice per cage, depending on group allocation) in plastic cages with conventional bedding and provided with food and water ad libitum under a 12 h day/night cycle (lights on at 07:00 h). The colonies for Oprl1‐eGFP‐homozygous (NOPeGFP/eGFP; NOP‐eGFP) mice on a C57/BL6/J background have been routinely maintained in‐house (Ozawa et al., 2015). To match the genetic background, male and female C57BL6/J wild‐type mice (4 weeks old) were obtained from the Jackson Laboratory (Bar Harbor, ME, USA). All behavioural studies were conducted in C57BL6/J wild‐type mice from the Jackson Laboratory in order to minimize the potential impact of the C‐terminally attached eGFP on the functionality of NOP receptors, though the NOP‐eGFP mice showed a normal nociceptive response to the thermal stimulation in the tail flick tests (Ozawa et al., 2015). Rather than using wild‐type littermates of the NOP‐eGFP mice, we used commercially available C57BL6/J mice in order to test sufficient number of animals of the same age. This also confers consistency on behavioural experiments. As we observed consistent changes in NOP receptors when measuring protein in NOP‐eGFP mice and mRNA in wild‐type mice, there are no anticipated problems in using wild‐type mice to conduct the pain behavioural experiments in the current study.
Spinal nerve ligation
SNL surgery at L4 and L5 mouse spinal nerves, which most closely mimics L5/L6 ligation in rats (Kim and Chung, 1992), was carried out in 5–8‐week‐old mice (male NOP‐eGFP mice, 20–25 g; female and male C57BL6/J wild‐type, 13–20 g), following previously reported procedures (Ye et al., 2015). Under isoflurane anaesthesia (5% for induction and 2% for maintenance in 10% O2), animals were placed in a prone position. A dorsal midline incision was made to expose the lumbar L6 transverse process. Cutting of L6 transverse process allowed visualization of the L5 spinal nerve and the L3 and L4 spinal nerves joining each other. L4 and L5 spinal nerves were then isolated and tightly ligated with 6–0 silk suture. Muscles were sutured using absorbable sutures and skin tightly glued. In sham‐operated animals, the surgical procedure was identical to the SNL surgery except that spinal nerves were not ligated. Body temperature was maintained at 37°C throughout the surgery using a heating pad. At the end of the procedure, mice were monitored until full recovery from anaesthesia. Two weeks after surgery, we applied gentle tactile stimuli to the ipsilateral hindpaw of un‐anaesthetized mice in order to define the tactile allodynia established by SNL using a set of von Frey filaments as described below.
Immunohistochemistry
Two and four weeks after surgery, sham‐operated and SNL NOP‐eGFP mice (N = 4 male mice per group) were anesthetized with an overdose of isoflurane for 1 min and transcardially perfused in 4% paraformaldehyde in PBS. Anaesthetic depth was ensured by testing the pedal withdrawal reflex (pinching on both hindpaws) before perfusion. The DRG (L4, L5 and L6) and spinal cord (lumbar cord) were dissected and cryoprotected in 30% sucrose in PBS. Tissues were then frozen in OCT (Sakura Finetek, INC., Torrence, CA, USA) and stored in −80°C until used. DRG from the ipsilateral and contralateral sides were collected separately. DRG from the same animal group were then pooled for cryoprotection and frozen in the same mould. Tissue sections (40 μm for spinal cord; and 10 μm for DRG) were prepared by using a cryostat (Leica Biosystems, Buffalo Grove, IL, USA) and blocked with PBS containing 5% normal donkey serum and 0.3% Triton X‐100 for 1 h at room temperature. The sections were then incubated with primary antibodies, indicated in each figure, at 4°C, overnight. For the chicken anti‐GFP antibody, the incubation was performed at 37°C for 2 h. After extensive washing with PBS containing 1% normal donkey serum and 0.3% Triton X‐100, sections were incubated with appropriate secondary antibody conjugated to AlexaFluor (1:1000) for 2 h at room temperature.
Image analysis and quantification
Images were collected under a Leica TCS SP5II confocal microscope and LAS AF Lite software (Leica Microsystems) or an A1R confocal microscope and NIS‐Elements Imaging software (images shown in Figure 1C, D; Nikon, Melville, NY, USA). All of the images directly compared were collected using the same laser intensities for the corresponding colour channels. Image analysis was carried out by using Fiji (National Institute of Health) by an experimenter, blinded to group conditions. Brightness and contrast were adjusted equally among the images compared when necessary. Images from four sections per animal were collected for analysis. Cell counts, immunoreactive intensities and percentage obtained for each section were averaged. To investigate the effect of chronic pain on NOP receptor distribution in areas of the spinal cord relevant for nociceptive processing, immunofluorescent measurements were carried out at the ipsilateral and contralateral spinal laminae I to IIo shown in Figure 1A. Using Fiji, the region of interest (ROI) was manually drawn with freehand line tool, and the intensity and size of the regions were measured using the ROI manager. The fluorescent intensity obtained from each ROI was then divided by its own area size. Values [NOP‐eGFP immunoreactivity (%)] for ipsilateral and contralateral sides of SNL mice and for contralateral side of sham‐operated mice were calculated relative to ipsilateral of sham group. To ensure NOP‐eGFP‐positive DRG neurons, background measurement was carried out at three low immunoreactive areas on the DRG in each image and the intensity was averaged. All DRG neurons that exceeded twice the background intensity were counted as NOP‐eGFP positive. To quantify the immunoreactivity of NOP‐eGFP in DRG neurons, NOP‐eGFP‐positive DRG neurons were first selected, and their fluorescent intensity and size were measured using the freehand line tool and ROI manager in Fiji. Fluorescent intensities and sizes were summed separately, and the immunoreactivity of NOP‐eGFP per image was calculated as [(total immunoreactive intensity of NOP‐eGFP‐positive cells)/(total area size of NOP‐eGFP‐positive cells)]. Immunoreactivity of NOP‐eGFP for ipsilateral and contralateral sides of SNL mice were then compared with the corresponding side of sham‐operated mice and represented as a percentage of sham group.
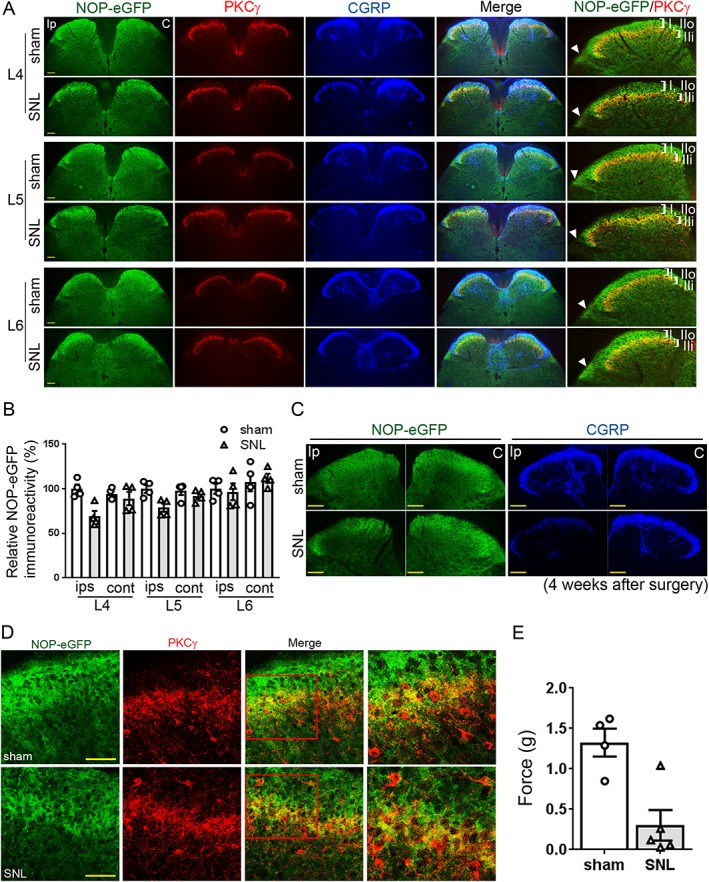
Changes in the distribution of NOP‐eGFP receptors in SNL mice. NOP‐eGFP receptor distribution was investigated 14 days post SNL of the L4/L5 spinal nerves. (A) Representative images show that NOP‐eGFP (green) is concentrated in the inner part of lamina II (IIi), where PKCγ interneurons (red) are located in the L4 and L5 ipsilateral spinal cord of the SNL mice (observed in yellow), while the immunoreactivity in laminae I and II outer (IIo) is decreased. In contralateral spinal cord, slightly lower immunoreactivity of NOP‐eGFP was observed in SNL mice, but it is not significant compared to sham‐operated mice. No changes were observed in the L6 segment. CGRP (blue), a marker for the spinal laminae I and IIo. All of the representative images were prepared from the same sham‐operated or SNL mice. Roman numerals in zoomed images for the ipsilateral side indicate lamina. White arrowhead depicts the position of the lateral spinal nucleus. Ip, Ipsilateral; C, Contralateral. (B) Quantification of immunoreactivity of NOP‐eGFP in the ipsilateral and contralateral laminae I and IIo. N = 4 mice per group. (C) Representative images of the L4 spinal dorsal horns 4 weeks after surgery. As in the results in panel (A), a decrease in NOP‐eGFP immunoreactivity was observed in the ipsilateral spinal cord of SNL mice. Note that images for ipsilateral and contralateral were obtained from the same section. In the same section, CGRP staining was also carried out as a reference to verify the effect of SNL in the ipsilateral spinal dorsal horn. (D) Representative high‐power images of the ipsilateral L4 spinal cord derived from sham‐operated and SNL mice. Right panel, an expanded image of the red square in the merged images. No PKCγ interneurons co‐expressed NOP‐eGFP. (E) Effect of SNL on mechanical allodynia measured by von Frey (N = 4 for sham and N = 5 for SNL male NOP‐eGFP mice). After initial von Frey testing, one mouse from SNL group was eliminated from the immunostaining experiments because it did not develop mechanical allodynia. For all quantifications, data shown are means ± SEM. Scale bars: (A) and (C), 100 μm; (D), 50 μm.
Intrathecal drug administration
By following the method of Hylden and Wilcox (1980), intrathecal (i.t.) administration of N/OFQ (3 and 10 nmol) or vehicle was performed, using doses based on a previously reported study (Rizzi et al., 2015). Briefly, an awake mouse was held firmly by the pelvic girdle in one hand. A disposable 30 gauge 0.5 inch needle attached to a 10 μL Hamilton syringe was then inserted into the vertebrae between L5 and L6 of the mouse spinal column. A characteristic tail flick was confirmed as the penetration of the subarachnoid space. N/OFQ was administered in a total volume of 5 μL per mouse. Changes in each pain modality described below were determined 10 min after the injection of N/OFQ in SNL mice as compared to sham‐operated mice. For the antagonist test, 10 mg·kg−1 of http://www.guidetopharmacology.org/GRAC/LigandDisplayForward?ligandId=1693 was i.p. administered to mice 30 min prior to N/OFQ or vehicle (Toll et al., 2009; Khroyan et al., 2011).
Assessment of tactile allodynia
Tactile allodynia was measured in mice that received either SNL or sham surgery as hindpaw withdrawal response to a set of von Frey filaments with forces of 0.04, 0.07, 0.16, 0.4, 0.6, 1.0 and 2.0 g (Stoelting, Wood Dale, IL, USA) using the up/down method of Chaplan et al. (1994). Prior to the test, mice were habituated for 30 min in individual chambers placed on a wire‐mesh floor. The test began with a von Frey filament that had a buckling weight of 0.4 g. The von Frey filament was applied to the plantar surface of the ipsilateral hindpaw (left hindpaw) of the animal with continuous pressure for approximately 5 s. A positive response was recognized as a sharp withdrawal of the testing hindpaw. If the animal lifted its paw, the next filament with lower force was then applied. If the animal did not lift its paw, the next filament with higher force was used. Each response was recorded, and the experiment ended once the animal had made five responses after the initial positive response. The 50% paw withdrawal threshold was calculated (Dixon, 1980). If the animal made four consecutive positive responses, a score of 0.04 g was assigned, whereas if the animal had five consecutive negative responses, then a score of 2.0 g was assigned.
Assessment of heat and cold hyperalgesia
Heat hyperalgesia was measured using a hot/cold plate (IITC, Woodland Hills, CA, USA) with the surface temperature maintained at 55°C. Mice were placed on the hot plate, and the latency for withdrawal of the hindpaw or jumping from the metal plate was measured. A cut‐off latency of 30 s was used to avoid tissue damage. For cold hyperalgesia, mice were placed on the metal plate with the surface temperature maintained at 2°C. The number of flicks of the ipsilateral hindpaw was counted during an observation period of 5 min.
Quantification of NOP receptor mRNA expression
Total RNA for the spinal cord and DRG neurons was isolated from wild‐type mice that received SNL or sham surgery (2 and 6 weeks after the surgery) using RNAeasy Mini kit (Qiagen, Hilden, Germany). mRNA analysis for the 6‐week group was carried out with mice (three female and three male) previously used for pharmacological testing. Mice were deeply anesthetized with isoflurane and killed by cervical dislocation. The lumbar portion of the spinal cord (from L4 to L5 segments) as well as ipsilateral and contralateral L4‐L5 DRG was dissected on ice. L4 and L5 DRG for each animal were pooled together for analysis. For dissecting the spinal cord, the spinal column was carefully removed from neck to tail in order to expose the dorsal side. The cranial and the caudal ends as well as L4 and L5 segments of the spinal column were marked for reference. The lumbar cord was carefully removed from the spinal column. The spinal cord was carved at the centre towards the caudal end by following the midline reference in order to separate ipsilateral and contralateral portions and the L4‐L5 were then excised. Total RNA was subjected to reverse transcription with a QuantiTect Reverse Transcription Kit (Qiagen). To measure mRNA levels, real‐time quantitative RT‐PCR was performed with the iCycler IQ5 multicolor Real‐Time PCR detection system (Bio‐Rad Laboratories, Hercules, CA, USA) using QuantiFast SYBR Green PCR Kit (Qiagen); 95°C for 3 min to activate the HotStart enzyme followed by 45 cycles of amplification and quantification (10 s at 95°C; 30 s at 60°C) each with a single FAM fluorescence measurement. Melting curve analysis (60–95°C) was also performed in order to verify reaction specificity for each PCR products. Gene‐specific primers for NOP receptor and GAPDH (an endogenous reference gene) were designed by the NCBI primer‐blast. GAPDH was used as a reference gene to normalize NOP receptor mRNA expression due to the previous evidence showing stable expression in DRG and spinal cord between normal and nerve‐injured animals (Laedermann et al., 2014; Jiang et al., 2015). All of the primers were purchased from Eurofins (Louisville, KY, USA). Primers for NOP receptor and GAPDH were as follows: NOP_F459, 5′‐ATGTCATCCTCAGGCACACC‐3′; NOP_R573, 5′‐AAATGGCCAGAAGCCCAGAA‐3′, GAPDH_F581, 5′‐GTGGAAGGGCTCATGACCAC‐3′; and GAPDH_R698, 5′‐ATGCAGGGATGATGTTCTGG‐3′. Data were calculated as the mean ΔCt relative to the house‐keeping gene GAPDH. The ΔΔCt method (Livak and Schmittgen, 2001) was employed to calculate percentage expression of SNL mice relative to sham‐operated mice [(relative NOP mRNA level for individual mouse)/(averaged relative NOP mRNA level of sham‐operated mice)×100].
Experimental design
Assessment of tactile allodynia was carried out in all the mice used for molecular and behavioural analysis, at 2 weeks after surgery. Animals that were not deemed healthy after surgery were excluded from the experiments. Prior to pharmacological tests, 8 and 10 mice from each group were randomly selected and subjected to cold and hot plate, respectively, in order to measure the basal response to each pain modality. Beginning 3 weeks after surgery, mice received i.t. injections of N/OFQ (3 or 10 nmol) or vehicle and were examined on each behavioural test described above. The behavioural tests were carried out in the following order: mechanical allodynia (3 weeks post‐surgery), heat hyperalgesia (4 weeks post‐surgery) and cold hyperalgesia (5 weeks post‐surgery). After all the behavioural tests, tissues from six mice per group were dissected for the RNA preparation. Additionally, in a separate cohort of SNL mice, i.t N/OFQ (10 nmol) was examined in the presence or absence of SB‐612111 (10 mg·kg−1, i.p.) for mechanical allodynia (3 weeks post‐surgery) and heat hyperalgesia (4 weeks post‐surgery). The exact number of animals tested in different treatments in the study is indicated in the Methods section or in the figure legends. A sample size for behavioural experiments was determined by a power analysis done with G*Power 3.1.9.2. Animals in each group (sham‐operated and SNL mice; female and male) were randomized before treatments. All pain behavioural experiments were conducted by experimenters blinded to the condition and treatments used.
Data and statistical analysis
The data and statistical analysis comply with the recommendations on experimental design and analysis in pharmacology (Curtis et al., 2015). All of the results are shown as mean ± SEM. Data were tested for statistical significance using unpaired t‐test or two‐way ANOVA that used two between‐subject factors (‘ligation’ and ‘treatment’ for the N/OFQ antinociceptive effect on SNL‐mediated allodynia, and heat/cold hyperalgesia; ‘pretreatment’ × ‘treatment’ for SB‐612111 test on the N/OFQ‐mediated antinociceptive effects). When interaction of factors was statistically significant (P values less than 0.05), pairwise comparisons were conducted by using Newman–Keuls post hoc test (Statistica 7.0 software). Any data point that was detected as an outlier (Grubbs, α = 0.05, P < 0.05) was excluded from unpaired t‐test or two‐way ANOVA.
Materials
N/OFQ and http://www.guidetopharmacology.org/GRAC/LigandDisplayForward?ligandId=1693, an NOP receptor antagonist, were provided by the National Institute on Drug Abuse Drug Supply Program and were dissolved in sterile PBS and 2% DMSO in 0.5% aqueous hydroxypropyl cellulose (HPC) respectively. All solutions were prepared freshly on the test day.
Nomenclature of targets and ligands
Key protein targets and ligands in this article are hyperlinked to corresponding entries in http://www.guidetopharmacology.org, the common portal for data from the IUPHAR/BPS Guide to PHARMACOLOGY (Harding et al., 2018), and are permanently archived in the Concise Guide to PHARMACOLOGY 2017/18 (Alexander et al., 2017a,b).
Results
SNL alters the distribution of NOP receptors in the spinal dorsal laminae
To obtain a better understanding of the plasticity of NOP receptors due to chronic neuropathic pain, we investigated the changes in the laminar location of spinal NOP receptors in NOP‐eGFP mice subsequent to SNL. SNL caused a marked increase in the response to mechanical stimulation of the ipsilateral paw by von Frey filaments (Figure 1E). In the spinal cord of SNL mice 2 weeks after the surgery, as compared to sham‐operated mice, the immunoreactivity of NOP‐eGFP receptors was decreased, but not completely eliminated, in the spinal dorsal lamina I, a region generally responsible for noxious heat stimulation (Figure 1A, D). Specifically, a decrease in NOP‐eGFP immunoreactivity was observed in the ipsilateral L4 and L5 segments of the spinal cord when SNL animals were compared to sham‐operated animals (Figure 1B). Note that L4 ipsilateral spinal segment displayed a larger decrease in NOP‐eGFP immunoreactivity than that of L5 ipsilateral spinal segment. Likewise, a difference in NOP‐eGFP immunoreactivity was detected between ipsilateral and contralateral sides of L4 and L5 (Figure 1B). We found similar results in the spinal cord derived from the SNL mice 4 weeks after the surgery (Figure 1C), suggesting that NOP receptor level was consistently down‐regulated due to chronic pain. There was no difference in immunostaining of the contralateral side of the L4 and L5 superficial lamina in SNL mice compared to those of sham‐operated mice. Based on the previous neurochemical studies (Honore et al., 2000), we also used CGRP as a molecular marker to confirm the effect of SNL in the ipsilateral spinal dorsal horn. Consistent with the previous report (Honore et al., 2000), a decrease in CGRP immunoreactivity was observed in the ipsilateral dorsal horn in SNL mice, compared to sham‐operated mice. These results clearly demonstrate that a chronic pain state caused by SNL indeed caused changes in NOP‐eGFP distribution in the ipsilateral spinal dorsal horn. In the L6 segment, similar immunoreactivity was observed between sham‐operated and SNL mice and between ipsilateral and contralateral sides. In contrast to lamina I, the immunoreactivity of NOP‐eGFP receptor was unchanged in the ventral border of lamina II inner (IIi), where NOP‐eGFP receptors overlap with http://www.guidetopharmacology.org/GRAC/ObjectDisplayForward?objectId=1484 interneurons, a region important for developing injury‐induced allodynia (Figure 1A, D) (Neumann et al., 2008). Interestingly, we found no PKCγ interneurons that co‐express NOP‐eGFP in either sham or SNL mice (Figure 1D). These results indicate that NOP‐eGFP expression is on other spinal neurons in laminae IIi and III that are involved in the development of mechanical allodynia. In addition to the results seen in the spinal dorsal horn, a decreased immunoreactivity of NOP‐eGFP was also observed in other regions of the spinal cord throughout lamina X to the ventral parts. Interestingly, decreased immunostaining of NOP‐eGFP in the lateral spinal nucleus that does not receive input from primary afferents (Cliffer et al., 1988) was also observed (Figure 1A), indicating that the NOP‐eGFP receptors are also expressed on neurons intrinsic to the cord that do not have cell bodies in the DRG neurons.
Expression of NOP receptors in DRG neurons is lowered in SNL mice
We next addressed the expression pattern of NOP‐eGFP receptor in the DRG neurons derived from sham‐operated and SNL mice. Similar to the results seen in the spinal cord, the total NOP‐eGFP receptor expression was decreased in the L4 and L5 ipsilateral DRG of SNL mice, compared to the sham‐operated mice (Figure 2A, C). No changes in NOP‐eGFP immunoreactivity were observed in the L6 ipsilateral DRG or any of the contralateral DRG (Figure 2A–D). In addition, the percentage of small NOP‐eGFP‐positive neurons (100–200 and 200–300 μm2) was reduced in L4 DRG subsequent to SNL surgery (Figure 2G). NOP‐eGFP receptor expression disappeared in about 50% of small‐sized DRG neurons in SNL mice. In contrast, an increase in NOP‐eGFP‐positive cell number was observed in medium‐sized L4 neurons (Figure 2G; 400–500 μm2). Small NOP‐eGFP‐positive DRG neurons (<300 μm2) co‐expressing CGRP (NOP‐eGFP+ CGRP+) were rarely found in L4 ipsilateral DRG of SNL mice (Figure 2A, I), suggesting that the expression of NOP receptors might be down‐regulated specifically in peptidergic C‐nociceptors during chronic pain. A decrease in the number of cells expressing CGRP was selectively observed in the ipsilateral L4 and L5 DRG of SNL mice compared to sham‐operated mice (Figures 2E, F), which also agrees with previous neurochemical analyses conducted in SNL mice (Honore et al., 2000). In L5 DRG, surprisingly, there was not a comparable difference in the percentage of NOP‐eGFP‐positive cells between sham‐operated and SNL mice at any neuron sizes (Figure 2H) nor in the percentage of small NOP‐eGFP+ neurons expressing CGRP. As smaller changes in NOP‐eGFP immunoreactivity were observed in L5 ipsilateral DRG, compared to L4 ipsilateral DRG (Figure 2C), these results might indicate that reduced expression is a function of fewer receptors per neuron rather than certain types of neurons being completely devoid of NOP receptors in L5 DRG.
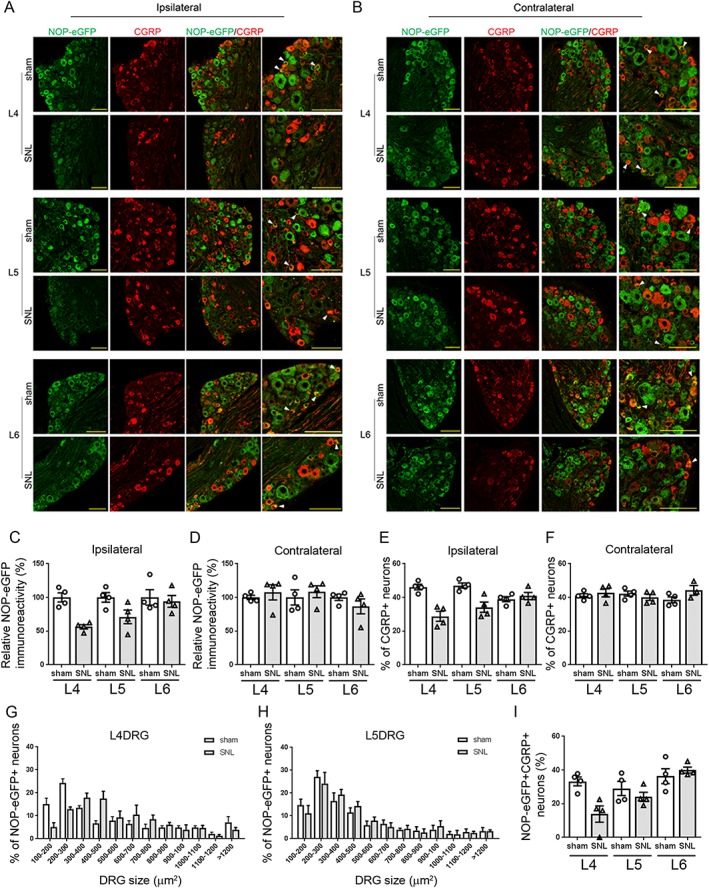
Expression of NOP‐eGFP receptors in the DRG neurons of SNL mice. (A, B) Representative images of L4, L5 and L6 ipsilateral and contralateral DRG neurons derived from sham‐operated and SNL mice. Tissue sections were incubated with anti‐GFP (green) and anti‐CGRP (red) antibodies. Right panel, an expanded image of the merged images. White arrowheads denote the cells co‐expressing NOP‐eGFP and CGRP in small DRG neurons. These cells were rarely observed in the L4 DRG in SNL mice. As DRG tissues were pooled for cryoprotection, the representative images for each section were prepared from all of the animals in the corresponding group. Scale bars, 100 μm. (C) Decreased expression level in NOP‐eGFP receptors was observed in the L4 and L5 ipsilateral DRG neurons of SNL mice compared to sham‐operated mice. NOP‐eGFP expression in the L6 ipsilateral DRG neurons was not affected by SNL. (D) No difference between sham and SNL mice in NOP‐eGFP immunoreactivity was observed in the contralateral DRG neurons. (E, F) Population of the CGRP‐expressing neurons in the L4, L5 and L6 ipsilateral and contralateral DRG. Percentage of CGRP‐positive neurons was calculated by comparing with the total number of DRG neurons. (G, H) Size profiling of NOP‐eGFP+ neurons in L4 and L5 ipsilateral DRG. (I) Population of small NOP‐eGFP‐positive neurons (<300 μm2) expressing CGRP (NOP‐eGFP+ CGRP+) in the L4, L5 and L6 ipsilateral DRG. The percentage of NOP‐eGFP+ CGRP+ neurons was determined by comparing with the total number of small CGRP‐positive neurons; [(number of small NOP‐eGFP+ CGRP+ neurons)/(number of small CGRP+ neurons)×100]. For all quantifications, data shown are means ± SEM. N = 4 mice for each group.
mRNA levels in DRG and spinal cord of wild‐type sham‐operated and SNL mice were also determined (Figure 3). Consistent with a decrease in NOP receptor protein, mRNA levels were also reduced in both ipsilateral DRG and spinal cord of SNL wild‐type mice at 2 and 6 week after the SNL surgery (Figure 3A, C). Changes in mRNA expression level of NOP receptor were not observed in either contralateral spinal cord or DRG (Figure 3B, D). These data together with spinal NOP receptor distribution discussed above suggest that an important role of specific subsets of NOP receptor expressing neurons in the spinal cord and DRG may affect the development of NOP receptor‐based analgesics.
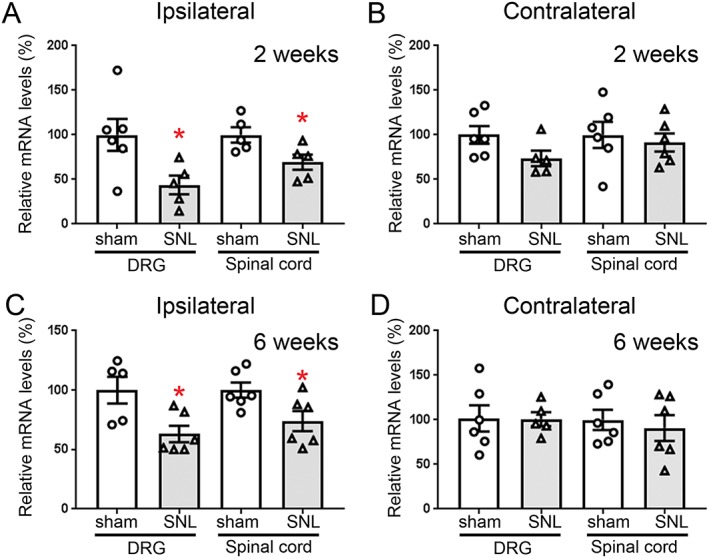
NOP receptor mRNA expression in the spinal cord and DRG of SNL wild‐type mice. Relative mRNA expression of NOP receptor in the ipsilateral (A, C) and contralateral (B, D) spinal cord and DRG neurons were determined by quantitative RT‐PCR, 2 (A, B) and 6 weeks (C, D) after surgery. (A, B) Two weeks after surgery: Ipsilateral DRG: sham (N = 6, three female and three male mice); SNL (N = 5, three female and two male mice). Ipsilateral spinal cord: sham (N = 5, three female and two male mice); SNL (N = 5, two female and three male mice). Contralateral DRG: sham (N = 6, three female and three male mice); SNL (N = 5, three female and two male mice). Contralateral spinal cord: sham (N = 6, three female and three male mice); SNL (N = 6, three female and three male mice). Outliers eliminated: One male SNL mouse from ipsilateral DRG and contralateral DRG; one sham mouse from ipsilateral spinal cord; one SNL female mouse from ipsilateral spinal cord. (C, D) Six weeks after surgery: Ipsilateral DRG: sham (N = 5, two female and three male mice); SNL (N = 6, three female and three male mice). Ipsilateral spinal cord: sham (N = 6, three female and three male mice); SNL (N = 6, three female and three male mice). Contralateral DRG: sham (N = 6, three female and three male mice); SNL (N = 5, two female and three male mice). Contralateral spinal cord: sham (N = 6, three female and three male mice); SNL (N = 6, three female and three male mice). Outliers eliminated: One sham female mouse from ipsilateral DRG; one SNL female mouse from contralateral DRG. Tissues were collected from the mice 2 and 6 weeks after surgery. Results similar to the immunostaining shown in Figures 1 and and22 were observed in the spinal cord and DRG. Data are represented as mean ± SEM. *P < 0.05, significantly different from sham‐operated mice; unpaired t‐test, Welch's correction.
Effects of i.t. N/OFQ on mechanical allodynia and heat and cold hyperalgesia in SNL mice
The decrease in NOP receptors in spinal laminae I and II outer suggests that NOP receptor activation could be less effective in attenuating noxious heat pain, but not mechanical pain, when N/OFQ is administered intrathecally, to the spinal cord of animals in a chronic pain state. To address this hypothesis, we investigated the effect of spinally administered N/OFQ on mechanical allodynia and both heat and cold hyperalgesia induced by SNL. Figure 4B–D shows a significant difference in the basal pain responses to mechanical and thermal stimulations observed between sham‐operated and SNL mice at 2 or 3 weeks following surgery, as shown by the von Frey, hot plate and cold plate tests. As seen in Figure 4E, i.t. treatment with N/OFQ produced changes in pain threshold. ANOVA showed significant ‘treatment’ × ‘ligation’ interaction (F (2,53) = 8.7). Post hoc analysis indicated that N/OFQ reduced sensitivity to mechanical stimuli in SNL mice at 10 nmol. These results are similar to previously reported spinal N/OFQ effects in SNL rats and other chronic pain models (Yamamoto et al., 1997; Corradini et al., 2001; Courteix et al., 2004; Ju et al., 2013). In contrast, no drug effect was detected in sham‐operated mice, although there was an unexpected trend towards increased sensitivity at the 3 nmol dose.
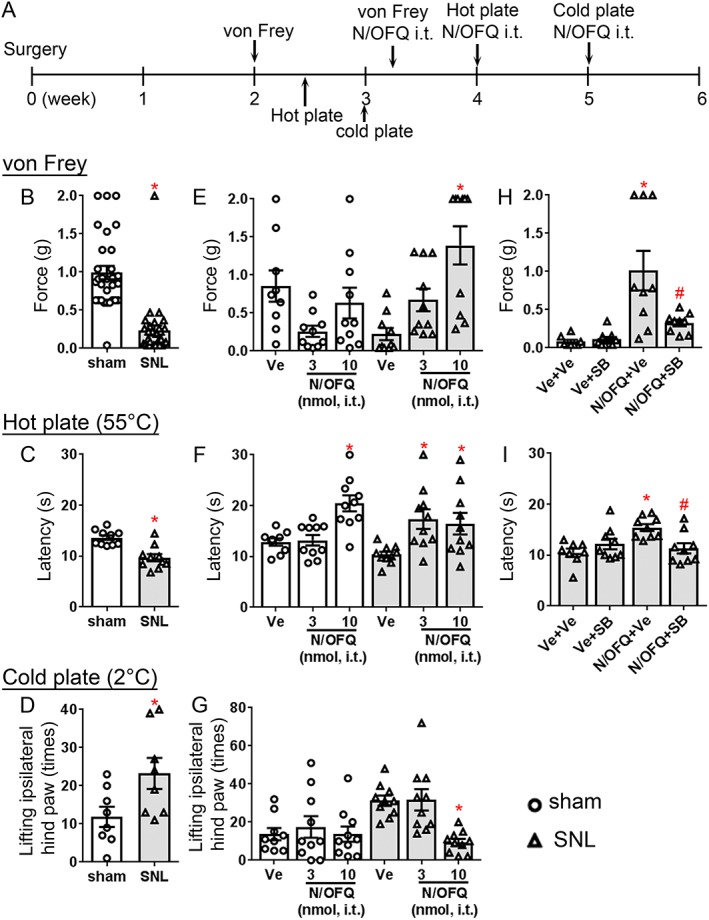
Spinal N/OFQ effects on mechanical allodynia, heat and cold hyperalgesia mediated by SNL in wild‐type mice. (A) Schematic of the experimental design. (B–D) Effect of L4/L5 SNL on mechanical allodynia (N = 30 mice per group; 15 female and 15 male mice per group) and heat and cold mediated hyperalgesia (hot plate, N = 10 mice per group; five female and five male mice per group; and cold plate, N = 8 mice per group; four female and four male mice per group). These experiments were conducted 2 or 3 weeks after ligation (von Frey and hot plate, 2 weeks post‐surgery; and cold plate, 3 weeks post‐surgery). Data are represented as mean ± SEM. *P < 0.05, significantly different from sham‐operated mice; unpaired t‐test, Welch's correction). One sham‐operated and two SNL mice were not deemed healthy and eliminated from the behavioural experiments after initial von Frey tests. Total of 29 sham‐operated mice (14 female and 15 male mice) and 28 SNL mice (14 female and 14 male mice) were then used in the following antinociceptive assays. (E–G) Effect of spinally administered N/OFQ on mechanical allodynia, heat and cold hyperalgesia in sham‐operated mice (vehicle, N = 9, four female and five male mice; 3 nmol N/OFQ, N = 10, five female and five male mice; 10 nmol N/OFQ, N = 10, five female and five male mice) and SNL mice (vehicle, N = 9, four female and five male mice; 3 nmol N/OFQ, N = 9, five female and four male mice; 10 nmol N/OFQ, N = 10, five female and five male mice). (H, I) Administration of both N/OFQ (10 nmol, i.t.) and SB‐612111 (10 mg·kg−1, i.p) in SNL mice. Ve + Ve (N = 8 mice, four female and four male mice), PBS + 2% DMSO, 0.5% HPC; Ve + SB (N = 9 mice, five female and four male mice), PBS + SB‐612111; N/OFQ + Ve (N = 9 mice, four female and five male mice), N/OFQ + 2% DMSO, 0.5% HPC; and N/OFQ + SB (N = 9 mice, five female and four male mice), N/OFQ + SB‐612111. One SNL mouse was not deemed healthy and was eliminated from the behavioural experiments. Total of 35 SNL mice (18 female and 17 male mice) were used in the SB‐612111 tests. *P < 0.05, significantly different from vehicle‐treated animals; # P < 0.05, , significantly different from N/OFQ‐treated animals. Data shown are means ± SEM.
Heat hyperalgesia (Figure 4F) was also significantly blocked by spinal N/OFQ treatment ‘treatment’ × ‘ligation’ interaction (F (2,53) = 4.9). On post hoc analysis, N/OFQ increased latency to hot plate responses at 3 nmol as well as 10 nmol in SNL mice. In contrast, 10 nmol but not 3 nmol N/OFQ attenuated noxious acute heat pain in sham‐operated mice, which is similar to previous tail flick studies with naïve mice (Rizzi et al., 2015). Note that this antinociceptive action in sham‐operated mice is with respect to acute heat pain, not injury‐induced heat hyperalgesia, as there is no development of hyperalgesia in sham‐operated mice. Cold plate assays conducted following N/OFQ treatment led to similar results with ANOVA showing significant ‘treatment’ × ‘ligation’ interaction (F (2,52) = 3.9). Cold hyperalgesia was potently reduced by 10 nmol N/OFQ in SNL mice, while N/OFQ did not alter the sensitivity to cold in sham‐operated mice at any dose examined (Figure 4G).
Next, we examined the spinal N/OFQ effect in SNL mice in the presence of SB‐612111, a selective NOP receptor antagonist. The overall ANOVA indicated that there was a significant effect of SB‐612111 on the N/OFQ‐mediated antiallodynic and antihyperalgesic effects ‘pretreatment’ × ‘treatment’ interaction (von Frey, [F (1,33) = 7.4]; hot plate, [F (1,32) = 11.4]). Post hoc analysis showed that i.t. N/OFQ attenuated mechanical allodynia in SNL mice at 10 nmol (Figure 4H,), which was consistent with the results in Figure 4E. In contrast, systemic administration of SB‐612111 (10 mg·kg−1, i.p.) reversed the analgesic effect mediated by i.t. N/OFQ (Figure 4H). Similarly, antihyperalgesia to heat, mediated by 10 nmol N/OFQ, was potently reduced by systemically administered SB‐612111 (10 mg·kg−1, i.p., Figure 4I). These results demonstrate that the antiallodynic and antihyperalgesic effects mediated by spinally administered N/OFQ are via NOP receptors.
Discussion
Immunohistochemistry with NOP‐eGFP mice revealed that NOP‐eGFP receptors were selectively decreased in spinal laminae I and IIo, as well as DRG neurons, in mice that had undergone SNL. Similarly, a decrease in NOP receptor mRNA levels in spinal cord and DRG was observed in SNL wild‐type mice. Interestingly, spinal (i.t.) N/OFQ administration still elicited antinociceptive effects against injury‐induced heat and cold hyperalgesia as well as mechanical allodynia. These N/OFQ‐mediated effects were completely blocked by an NOP receptor antagonist, SB‐612111. These results are the first to visualize NOP receptor distribution in spinal cord and DRG, in the absence of problematic NOP receptor antibodies, to clearly demonstrate a chronic pain‐induced reduction in NOP receptor protein in specific spinal laminae and subpopulations of DRG neurons. Although these anatomical and pharmacological results are not apparently consistent, they demonstrate NOP receptor alterations along the pain pathway induced by SNL that must be reconciled with the behavioural results.
Plasticity of the NOP receptor system in response to pain has been investigated using a number of chronic pain models (Schroder et al., 2014). In the rat chronic constriction injury (CCI) model, spinally administered N/OFQ inhibited both thermal hyperalgesia and mechanical allodynia, while it had no effect on mechanical pain thresholds in naïve rats (Courteix et al., 2004). Similarly, Ro64‐6198, a selective NOP receptor agonist, inhibited mechanical and cold allodynia after peripheral and spinal administration in CCI rats, while it had no effect on naïve animals (Obara et al., 2005). One explanation for these pharmacological results could be that expression of NOP receptors is up‐regulated in tissues essential for transmitting pain signals. In a previous study with the rat CCI model, an up‐regulation of NOP receptor mRNA in lumbar spinal cord and ipsilateral L5‐L6 DRG was reported (Briscini et al., 2002). However, we found that SNL elicited a down‐regulation of NOP receptor mRNA in both spinal cord and DRG. In the earlier study, mRNA expression level was measured by a ‘semi‐quantitative’ method that requires step‐wise post‐PCR processing, which can lead to high intra‐ and inter‐assay variability in endpoint detection assays. Our mRNA analyses were quantitatively carried out in the same test tube, thereby, reducing potential sources of errors. Additionally, mRNA samples were prepared at different post‐surgery time points in the earlier studies (Briscini et al., 2002) compared to the data discussed here. Perhaps, the two chronic pain models lead to different neurochemical changes in the NOP receptor system due to temporal differences.
In addition to NOP receptor transcript level in chronic pain models, NOP receptor and N/OFQ were reportedly down‐regulated in certain subsets of L4 DRG neurons in rats subsequent to partial nerve ligation and complete Freund's adjuvant injection (Chen and Sommer, 2006). This is consistent with our immunohistochemistry data with SNL NOP‐eGFP mice. However, the study reported an overall increase in NOP receptor immunoreactivity in DRG neurons of neuropathic animals. This study used NOP receptor antibodies, for which high background staining may have made quantification difficult. Although they found the overall percentage of NOP receptor containing cells in DRG was comparable to the value found here (33% compared to approximately 40% in NOP‐eGFP mice), it is possible that inadequate primary antibodies against NOP receptors made quantification difficult. GPCR antibodies have been notoriously problematic, which is the case for NOP receptor antibodies. One study was withdrawn after similar immunohistochemical staining patterns were observed in wild‐type and NOP receptor knockout mice (Corrigendum, 1999). However, as noted above, the difference might be a function of different pain models used. Further investigations are required with NOP‐eGFP mice to determine how NOP distribution and/or expression are altered by each pain model and at different post‐surgery times.
Histological studies have also provided information regarding where opioid receptors are expressed and where agonists modulate pain signals in the brain, spinal cord and DRG. For example, spinal http://www.guidetopharmacology.org/GRAC/ObjectDisplayForward?objectId=319 are expressed in heat‐sensitive and peptidergic nociceptors that project to spinal laminae I and IIo, which are responsible for acute heat pain and injury‐induced heat hyperalgesia (Scherrer et al., 2009). In contrast, δ opioid receptors, preferentially expressed by mechanosensitive nociceptors that project to lamina IIi, transmit mechanical pain signals including injury‐induced allodynia (Scherrer et al., 2009). NOP receptors are highly expressed in spinal laminae I through III, areas that regulate heat, cold and mechanical pain and are expressed in a variety of DRG neurons including ones responsible for thermal and mechanical pain (Ozawa et al., 2015).
Current studies have demonstrated considerable plasticity in NOP receptors. SNL induced a substantial decrease in immunoreactivity of NOP receptors in spinal laminae I and IIo but not from lamina IIi and lamina III, which contain PKCγ‐positive excitatory interneurons. This is consistent with a decrease in the number of NOP receptor‐containing small DRG neurons in SNL mice compared to sham‐operated mice, since some of these small DRG neurons are C‐nociceptors, which have axon terminals in laminae I and II. Almost all peptidergic nociceptors, which express CGRP and modulate heat pain, co‐express μ opioid receptors (Scherrer et al., 2009). However, only a smaller percentage (~30%) of μ receptor‐positive peptidergic C‐nociceptors co‐express NOP receptors (Ozawa et al., 2015). Furthermore, after SNL, there are very few NOP‐eGFP+ small DRG neurons expressing CGRP. We hypothesized that this pattern of NOP receptor expression, in SNL mice, would result in NOP agonists being less effective for treatment of heat pain when administered i.t. This would be similar to analgesic actions shown for δ opioid receptors in chronic pain mice (Scherrer et al., 2009). Surprisingly, our antinociceptive tests revealed N/OFQ to be equally or more effective for attenuation of thermal hyperalgesia in SNL mice, suggesting two possibilities. Either there are C‐fibres that contain NOP receptors that modulate heat and cold pain that do not co‐express CGRP and are not affected by SNL, allowing N/OFQ to maintain effectiveness; or more likely, NOP receptors can be found on spinal projection neurons that transmit heat pain signals to the brain (Figure 5). In this way, i.t. administration of N/OFQ would still block hotplate‐induced heat pain despite NOP receptors missing from a significant portion of potential nociceptors. On the other hand, in naïve animals, N/OFQ and NOP agonists are known to presynaptically inhibit C‐fibre‐evoked responses in the spinal dorsal horn (Lai et al., 1997; Liebel et al., 1997; Wang et al., 1999; Carpenter et al., 2000), in order to express an antinociceptive action. The present study suggests that in SNL mice, spinal NOP receptor activation directly inhibits nociceptive‐specific projection neurons in the dorsal horn to exert analgesia on injury‐induced heat and cold hyperalgesia. Electrophysiological experiments are planned to address this question directly.
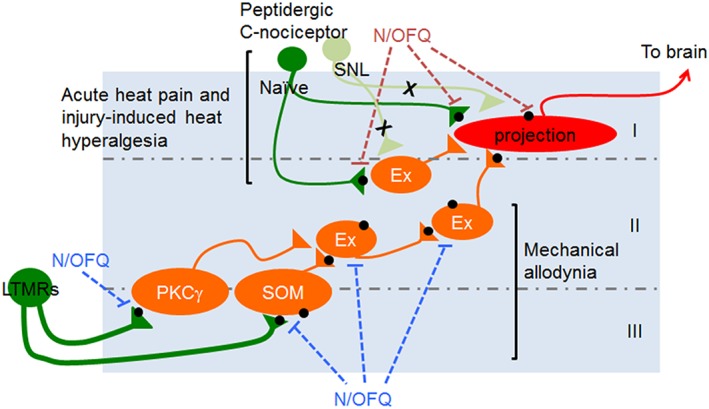
Diagram of possible antinociceptive mechanisms of spinally administered N/OFQ for acute and chronic pain. Acute heat pain and injury‐induced heat hyperalgesia: In naïve mice, i.t. N/OFQ is capable of attenuating acute heat pain presynaptically at the terminals of primary afferents and on the projection neurons (Lai et al., 1997; Liebel et al., 1997; Wang et al., 1999; Carpenter et al., 2000). Because of the diminished NOP receptor expression in the peptidergic C‐nociceptors caused by a chronic pain state, this presynaptic antinociceptive pathway via the peptidergic C‐nociceptors might be no longer available for the N/OFQ‐NOP receptor system (shown in light green). Although the expression of NOP receptor on the projection neuron in spinal lamina I (shown in red) is unknown, there is a possibility that NOP receptor agonists target the projection neurons in spinal lamina I that directly send the nociceptive signals to the brain, in order to attenuate heat hyperalgesia. Mechanical allodynia: i.t. N/OFQ may presynaptically inhibit the excitability of low‐threshold mechanoreceptors (LTMRs) that innervate the excitatory interneurons such as PKCγ and somatostatin‐positive excitatory neurons in lamina IIi, which critically contribute to establish tactile allodynia; alternatively, N/OFQ may inhibit the excitability of the mechanical allodynia circuitry at its neighbouring excitatory neurons present downstream of the PKCγ or somatostatin (SOM) pathway (Neumann et al., 2008; Duan et al., 2014; Peirs et al., 2015; Petitjean et al., 2015). A black circle indicates NOP receptor. Roman numerals depict lamina. Ex, excitatory neurons.
Our anatomical study has shown that NOP receptors may localize to myelinated low‐threshold mechanoreceptors, which are normally responsible for innocuous stimuli like touch (Ozawa et al., 2015). Spinal NOP receptors have a distribution similar to that of δ‐receptors, overlapping in lamina locations that are important for development of injury‐induced allodynia and receive input from low‐threshold myelinated primary afferents (Scherrer et al., 2009; Ozawa et al., 2015). In fact, the number of medium‐sized NOP‐eGFP+ DRG neurons is increased in SNL mice. These data are consistent with our previous demonstration that systemically administered small molecule NOP receptor agonists are effective in attenuating chronic neuropathic and inflammatory mechanical pain but not acute nociceptive pain (Toll et al., 2009; Khroyan et al., 2011). Electrophysiological studies previously revealed that cutaneous mechano‐sensitive A fibres contribute to transmission of allodynic pain signals to the spinal cord (Torsney and MacDermott, 2006). These pathways are under tonic inhibition that is likely derived from glycinergic interneurons located in deeper laminae under normal conditions (Takazawa and MacDermott, 2010). But upon injury, this inhibitory mechanism is reduced (thus disinhibiting nociceptive circuitry) in the spinal cord, enabling touch sensations to engage and activate nociceptive‐specific projection neurons (Torsney and MacDermott, 2006). In fact, certain circuitries underlying the disinhibitory mechanism for mechanical allodynia have been experimentally proven by recent studies (Figure 5; Duan et al., 2014; Peirs et al., 2015; Petitjean et al., 2015). The particular circuits for which spinal N/OFQ‐NOP system attenuates mechanical allodynia have yet to be uncovered. We speculate that spinal N/OFQ attenuates injury‐induced mechanical hypersensitivity by hyperpolarizing one or more of these disinhibited neurons along the nociceptive pathway. Altogether, to better develop NOP‐based analgesics for treatment of chronic pain, ongoing studies will characterize subpopulations of NOP receptor expressing neurons in DRG and spinal cord and determine how spinally administered NOP agonists inhibit specific spinal and/or peripheral neurons.
In conclusion, we have demonstrated down‐regulation of NOP‐eGFP levels in selective spinal laminae and a subset of DRG neurons that might be important for heat pain. However, the chronic pain‐induced changes observed in these tissues do not affect spinal N/OFQ antinociceptive actions against thermal hyperalgesia or mechanical allodynia subsequent to SNL. The present study has also demonstrated the usefulness of NOP‐eGFP mice in dissecting location and plasticity of receptors altered by specific pathophysiological states and interpreting the correspondence between anatomical changes and pharmacological outcomes.
Author contributions
A.O., G.B. and L.T. designed the study; A.O., G.B., A.C., N.T. and J.S. performed experiments; A.O., G.B., A.C., N.T. and L.T. analysed data: B.L.K. supplied materials and analytic tools; A.O., G.B., A.C. and L.T. wrote the paper; A.O. and G.B. contributed equally to this study.
Declaration of transparency and scientific rigour
This http://onlinelibrary.wiley.com/doi/10.1111/bph.13405/article acknowledges that this paper adheres to the principles for transparent reporting and scientific rigour of preclinical research recommended by funding agencies, publishers and other organisations engaged with supporting research.
Acknowledgements
This work was supported by the National Institute on Drug Abuse grant DA023281 to L.T. We thank Shannon Ortiz‐Umpierre and Roman Ramirez for maintaining the colony for NOP‐eGFP mice; Reona Asano, Yoshitaka Iwayama and Masako Nagai for excellent technical assistance; and the Optical Workshop and Light Microscopy Facility Center at the MAX PLANCK Florida Institute and the Nikon Center of Excellence at the Florida Atlantic University Brain Institute for the use of the microscopes.
Notes
Ozawa A., Brunori G., Cippitelli A., Toll N., Schoch J., Kieffer B. L., and Toll L. (2018) Analysis of the distribution of spinal NOP receptors in a chronic pain model using NOP‐eGFP knock‐in mice. British Journal of Pharmacology, 175: 2662–2675. 10.1111/bph.14225. [Europe PMC free article] [Abstract] [Google Scholar]
References
- Alexander SPH, Christopoulos A, Davenport AP, Kelly E, Marrion NV, Peters JA et al (2017a). The Concise Guide to PHARMACOLOGY 2017/18: G protein‐coupled receptors. Br J Pharmacol 174 (Suppl 1): S17–S129. [Europe PMC free article] [Abstract] [Google Scholar]
- Alexander SPH, Fabbro D, Kelly E, Marrion NV, Peters JA, Faccenda E et al (2017b). The Concise Guide to PHARMACOLOGY 2017/18: Enzymes. Br J Pharmacol 174 (Suppl 1): S272–S359. [Europe PMC free article] [Abstract] [Google Scholar]
- Bardoni R, Tawfik VL, Wang D, Francois A, Solorzano C, Shuster SA et al (2014). Delta opioid receptors presynaptically regulate cutaneous mechanosensory neuron input to the spinal cord dorsal horn. Neuron 81: 1312–1327. [Europe PMC free article] [Abstract] [Google Scholar]
- Briscini L, Corradini L, Ongini E, Bertorelli R (2002). Up‐regulation of ORL‐1 receptors in spinal tissue of allodynic rats after sciatic nerve injury. Eur J Pharmacol 447: 59–65. [Abstract] [Google Scholar]
- Carpenter KJ, Vithlani M, Dickenson AH (2000). Unaltered peripheral excitatory actions of nociceptin contrast with enhanced spinal inhibitory effects after carrageenan inflammation: an electrophysiological study in the rat. Pain 85: 433–441. [Abstract] [Google Scholar]
- Chaplan SR, Bach FW, Pogrel JW, Chung JM, Yaksh TL (1994). Quantitative assessment of tactile allodynia in the rat paw. J Neurosci Methods 53: 55–63. [Abstract] [Google Scholar]
- Chen Y, Sommer C (2006). Nociceptin and its receptor in rat dorsal root ganglion neurons in neuropathic and inflammatory pain models: implications on pain processing. J Peripher Nerv Syst 11: 232–240. [Abstract] [Google Scholar]
- Cliffer KD, Urca G, Elde RP, Giesler GJ Jr (1988). Studies of peptidergic input to the lateral spinal nucleus. Brain Res 460: 356–360. [Abstract] [Google Scholar]
- Corradini L, Briscini L, Ongini E, Bertorelli R (2001). The putative OP(4) antagonist, [Nphe(1)]nociceptin(1‐13)NH(2), prevents the effects of nociceptin in neuropathic rats. Brain Res 905: 127–133. [Abstract] [Google Scholar]
- Corrigendum (1999). Corrigendum. J Comp Neurol 412: 708. [Abstract] [Google Scholar]
- Courteix C, Coudore‐Civiale MA, Privat AM, Pelissier T, Eschalier A, Fialip J (2004). Evidence for an exclusive antinociceptive effect of nociceptin/orphanin FQ, an endogenous ligand for the ORL1 receptor, in two animal models of neuropathic pain. Pain 110: 236–245. [Abstract] [Google Scholar]
- Curtis MJ, Bond RA, Spina D, Ahluwalia A, Alexander SP, Giembycz MA et al (2015). Experimental design and analysis and their reporting: new guidance for publication in BJP. Br J Pharmacol 172: 3461–3471. [Europe PMC free article] [Abstract] [Google Scholar]
- Dixon WJ (1980). Efficient analysis of experimental observations. Annu Rev Pharmacol Toxicol 20: 441–462. [Abstract] [Google Scholar]
- Duan B, Cheng L, Bourane S, Britz O, Padilla C, Garcia‐Campmany L et al (2014). Identification of spinal circuits transmitting and gating mechanical pain. Cell 159: 1417–1432. [Europe PMC free article] [Abstract] [Google Scholar]
- Grisel JE, Mogil JS, Belknap JK, Grandy DK (1996). Orphanin FQ acts as a supraspinal, but not a spinal, anti‐opioid peptide. Neuroreport 7: 2125–2129. [Abstract] [Google Scholar]
- Harding SD, Sharman JL, Faccenda E, Southan C, Pawson AJ, Ireland S et al (2018). The IUPHAR/BPS Guide to PHARMACOLOGY in 2018: updates and expansion to encompass the new guide to IMMUNOPHARMACOLOGY. Nucleic Acids Res 46: D1091–D1106. [Europe PMC free article] [Abstract] [Google Scholar]
- Honore P, Rogers SD, Schwei MJ, Salak‐Johnson JL, Luger NM, Sabino MC et al (2000). Murine models of inflammatory, neuropathic and cancer pain each generates a unique set of neurochemical changes in the spinal cord and sensory neurons. Neuroscience 98: 585–598. [Abstract] [Google Scholar]
- Hylden JL, Wilcox GL (1980). Intrathecal morphine in mice: a new technique. Eur J Pharmacol 67: 313–316. [Abstract] [Google Scholar]
- Jiang BC, Sun WX, He LN, Cao DL, Zhang ZJ, Gao YJ (2015). Identification of lncRNA expression profile in the spinal cord of mice following spinal nerve ligation‐induced neuropathic pain. Mol Pain 11: 43. [Europe PMC free article] [Abstract] [Google Scholar]
- Ju J, Shin DJ, Na YC, Yoon MH (2013). Role of spinal opioid receptor on the antiallodynic effect of intrathecal nociceptin in neuropathic rat. Neurosci Lett 542: 118–122. [Abstract] [Google Scholar]
- Khroyan TV, Polgar WE, Orduna J, Montenegro J, Jiang F, Zaveri NT et al (2011). Differential effects of nociceptin/orphanin FQ (NOP) receptor agonists in acute versus chronic pain: studies with bifunctional NOP/mu receptor agonists in the sciatic nerve ligation chronic pain model in mice. J Pharmacol Exp Ther 339: 687–693. [Europe PMC free article] [Abstract] [Google Scholar]
- Kilkenny C, Browne W, Cuthill IC, Emerson M, Altman DG (2010). Animal research: reporting in vivo experiments: the ARRIVE guidelines. Br J Pharmacol 160: 1577–1579. [Europe PMC free article] [Abstract] [Google Scholar]
- Kim SH, Chung JM (1992). An experimental model for peripheral neuropathy produced by segmental spinal nerve ligation in the rat. Pain 50: 355–363. [Abstract] [Google Scholar]
- Ko MC, Woods JH, Fantegrossi WE, Galuska CM, Wichmann J, Prinssen EP (2009). Behavioral effects of a synthetic agonist selective for nociceptin/orphanin FQ peptide receptors in monkeys. Neuropsychopharmacology 34: 2088–2096. [Europe PMC free article] [Abstract] [Google Scholar]
- Laedermann CJ, Pertin M, Suter MR, Decosterd I (2014). Voltage‐gated sodium channel expression in mouse DRG after SNI leads to re‐evaluation of projections of injured fibers. Mol Pain 10: 19. [Europe PMC free article] [Abstract] [Google Scholar]
- Lai CC, Wu SY, Dun SL, Dun NJ (1997). Nociceptin‐like immunoreactivity in the rat dorsal horn and inhibition of substantia gelatinosa neurons. Neuroscience 81: 887–891. [Abstract] [Google Scholar]
- Liebel JT, Swandulla D, Zeilhofer HU (1997). Modulation of excitatory synaptic transmission by nociceptin in superficial dorsal horn neurones of the neonatal rat spinal cord. Br J Pharmacol 121: 425–432. [Europe PMC free article] [Abstract] [Google Scholar]
- Livak KJ, Schmittgen TD (2001). Analysis of relative gene expression data using real‐time quantitative PCR and the 2(−Delta Delta C(T)) Method. Methods 25: 402–408. [Abstract] [Google Scholar]
- McGrath JC, Lilley E (2015). Implementing guidelines on reporting research using animals (ARRIVE etc.): new requirements for publication in BJP. Br J Pharmacol 172: 3189–3193. [Europe PMC free article] [Abstract] [Google Scholar]
- Meunier JC, Mollereau C, Toll L, Suaudeau C, Moisand C, Alvinerie P et al (1995). Isolation and structure of the endogenous agonist of opioid receptor‐like ORL1 receptor. Nature 377: 532–535. [Abstract] [Google Scholar]
- Neumann S, Braz JM, Skinner K, Llewellyn‐Smith IJ, Basbaum AI (2008). Innocuous, not noxious, input activates PKCgamma interneurons of the spinal dorsal horn via myelinated afferent fibers. J Neurosci 28: 7936–7944. [Europe PMC free article] [Abstract] [Google Scholar]
- Obara I, Przewlocki R, Przewlocka B (2005). Spinal and local peripheral antiallodynic activity of Ro64‐6198 in neuropathic pain in the rat. Pain 116: 17–25. [Abstract] [Google Scholar]
- Ozawa A, Brunori G, Mercatelli D, Wu J, Cippitelli A, Zou B et al (2015). Knock‐in mice with NOP‐eGFP receptors identify receptor cellular and regional localization. J Neurosci 35: 11682–11693. [Europe PMC free article] [Abstract] [Google Scholar]
- Peirs C, Williams SP, Zhao X, Walsh CE, Gedeon JY, Cagle NE et al (2015). Dorsal horn circuits for persistent mechanical pain. Neuron 87: 797–812. [Europe PMC free article] [Abstract] [Google Scholar]
- Petitjean H, Pawlowski SA, Fraine SL, Sharif B, Hamad D, Fatima T et al (2015). Dorsal horn parvalbumin neurons are gate‐keepers of touch‐evoked pain after nerve injury. Cell Rep 13: 1246–1257. [Europe PMC free article] [Abstract] [Google Scholar]
- Pradhan AA, Tawfik VL, Tipton AF, Scherrer G (2015). In vivo techniques to investigate the internalization profile of opioid receptors. Methods Mol Biol 1230: 87–104. [Europe PMC free article] [Abstract] [Google Scholar]
- Reinscheid RK, Nothacker HP, Bourson A, Ardati A, Henningsen RA, Bunzow JR et al (1995). Orphanin FQ: a neuropeptide that activates an opioidlike G protein‐coupled receptor. Science 270: 792–794. [Abstract] [Google Scholar]
- Reiss D, Wichmann J, Tekeshima H, Kieffer BL, Ouagazzal AM (2008). Effects of nociceptin/orphanin FQ receptor (NOP) agonist, Ro64‐6198, on reactivity to acute pain in mice: comparison to morphine. Eur J Pharmacol 579: 141–148. [Abstract] [Google Scholar]
- Rizzi A, Sukhtankar DD, Ding H, Hayashida K, Ruzza C, Guerrini R et al (2015). Spinal antinociceptive effects of the novel NOP receptor agonist PWT2‐nociceptin/orphanin FQ in mice and monkeys. Br J Pharmacol 172: 3661–3670. [Europe PMC free article] [Abstract] [Google Scholar]
- Saccone PA, Zelenock KA, Lindsey AM, Sulima A, Rice KC, Prinssen EP et al (2016). Characterization of the discriminative stimulus effects of a NOP receptor agonist Ro 64‐6198 in Rhesus Monkeys. J Pharmacol Exp Ther 357: 17–23. [Europe PMC free article] [Abstract] [Google Scholar]
- Scherrer G, Imamachi N, Cao YQ, Contet C, Mennicken F, O'Donnell D et al (2009). Dissociation of the opioid receptor mechanisms that control mechanical and heat pain. Cell 137: 1148–1159. [Europe PMC free article] [Abstract] [Google Scholar]
- Scherrer G, Tryoen‐Toth P, Filliol D, Matifas A, Laustriat D, Cao YQ et al (2006). Knockin mice expressing fluorescent delta‐opioid receptors uncover G protein‐coupled receptor dynamics in vivo . Proc Natl Acad Sci U S A 103: 9691–9696. [Europe PMC free article] [Abstract] [Google Scholar]
- Schroder W, Lambert DG, Ko MC, Koch T (2014). Functional plasticity of the N/OFQ‐NOP receptor system determines analgesic properties of NOP receptor agonists. Br J Pharmacol 171: 3777–3800. [Europe PMC free article] [Abstract] [Google Scholar]
- Shoblock JR (2007). The pharmacology of Ro 64‐6198, a systemically active, nonpeptide NOP receptor (opiate receptor‐like 1, ORL‐1) agonist with diverse preclinical therapeutic activity. CNS Drug Rev 13: 107–136. [Europe PMC free article] [Abstract] [Google Scholar]
- Shoblock JR, Wichmann J, Maidment NT (2005). The effect of a systemically active ORL‐1 agonist, Ro 64‐6198, on the acquisition, expression, extinction, and reinstatement of morphine conditioned place preference. Neuropharmacology 49: 439–446. [Abstract] [Google Scholar]
- Takazawa T, MacDermott AB (2010). Synaptic pathways and inhibitory gates in the spinal cord dorsal horn. Ann N Y Acad Sci 1198: 153–158. [Europe PMC free article] [Abstract] [Google Scholar]
- Tian JH, Xu W, Fang Y, Mogil JS, Grisel JE, Grandy DK et al (1997). Bidirectional modulatory effect of orphanin FQ on morphine‐induced analgesia: antagonism in brain and potentiation in spinal cord of the rat. Br J Pharmacol 120: 676–680. [Europe PMC free article] [Abstract] [Google Scholar]
- Toll L, Bruchas MR, Calo G, Cox BM, Zaveri NT (2016). Nociceptin/orphanin fq receptor structure, signaling, ligands, functions, and interactions with opioid systems. Pharmacol Rev 68: 419–457. [Europe PMC free article] [Abstract] [Google Scholar]
- Toll L, Khroyan TV, Polgar WE, Jiang F, Olsen C, Zaveri NT (2009). Comparison of the antinociceptive and antirewarding profiles of novel bifunctional nociceptin receptor/mu‐opioid receptor ligands: implications for therapeutic applications. J Pharmacol Exp Ther 331: 954–964. [Europe PMC free article] [Abstract] [Google Scholar]
- Torsney C, MacDermott AB (2006). Disinhibition opens the gate to pathological pain signaling in superficial neurokinin 1 receptor‐expressing neurons in rat spinal cord. J Neurosci 26: 1833–1843. [Europe PMC free article] [Abstract] [Google Scholar]
- Wang XM, Zhang KM, Long LO, Mokha SS (1999). Orphanin FQ (nociceptin) modulates responses of trigeminal neurons evoked by excitatory amino acids and somatosensory stimuli, and blocks the substance P‐induced facilitation of N‐methyl‐D‐aspartate‐evoked responses. Neuroscience 93: 703–712. [Abstract] [Google Scholar]
- Witkin JM, Statnick MA, Rorick‐Kehn LM, Pintar JE, Ansonoff M, Chen Y et al (2014). The biology of Nociceptin/Orphanin FQ (N/OFQ) related to obesity, stress, anxiety, mood, and drug dependence. Pharmacol Ther 141: 283–299. [Europe PMC free article] [Abstract] [Google Scholar]
- Yamamoto T, Nozaki‐Taguchi N, Kimura S (1997). Effects of intrathecally administered nociceptin, an opioid receptor‐like1 (ORL1) receptor agonist, on the thermal hyperalgesia induced by unilateral constriction injury to the sciatic nerve in the rat. Neurosci Lett 224: 107–110. [Abstract] [Google Scholar]
- Ye GL, Savelieva KV, Vogel P, Baker KB, Mason S, Lanthorn TH et al (2015). Ligation of mouse L4 and L5 spinal nerves produces robust allodynia without major motor function deficit. Behav Brain Res 276: 99–110. [Abstract] [Google Scholar]
Articles from British Journal of Pharmacology are provided here courtesy of The British Pharmacological Society
Full text links
Read article at publisher's site: https://doi.org/10.1111/bph.14225
Read article for free, from open access legal sources, via Unpaywall:
https://bpspubs.onlinelibrary.wiley.com/doi/pdfdirect/10.1111/bph.14225
Citations & impact
Impact metrics
Citations of article over time
Alternative metrics
Smart citations by scite.ai
Explore citation contexts and check if this article has been
supported or disputed.
https://scite.ai/reports/10.1111/bph.14225
Article citations
Functional and anatomical analyses of active spinal circuits in a mouse model of chronic pain.
Pain, 165(3):685-697, 10 Oct 2023
Cited by: 1 article | PMID: 37820238
The Downregulation of Opioid Receptors and Neuropathic Pain.
Int J Mol Sci, 24(6):5981, 22 Mar 2023
Cited by: 11 articles | PMID: 36983055 | PMCID: PMC10053236
Review Free full text in Europe PMC
Regulation of N-type calcium channels by nociceptin receptors and its possible role in neurological disorders.
Mol Brain, 15(1):95, 24 Nov 2022
Cited by: 2 articles | PMID: 36434658 | PMCID: PMC9700961
Review Free full text in Europe PMC
The nociceptin receptor promotes autophagy through NF-kB signaling and is transcriptionally regulated by E2F1 in HCC.
Cell Death Discov, 8(1):165, 05 Apr 2022
Cited by: 6 articles | PMID: 35383175 | PMCID: PMC8983730
Current and Future Therapeutic Options in Pain Management: Multi-mechanistic Opioids Involving Both MOR and NOP Receptor Activation.
CNS Drugs, 36(6):617-632, 26 May 2022
Cited by: 10 articles | PMID: 35616826 | PMCID: PMC9166888
Review Free full text in Europe PMC
Go to all (16) article citations
Similar Articles
To arrive at the top five similar articles we use a word-weighted algorithm to compare words from the Title and Abstract of each citation.
Effects of spinally administered bifunctional nociceptin/orphanin FQ peptide receptor/μ-opioid receptor ligands in mouse models of neuropathic and inflammatory pain.
J Pharmacol Exp Ther, 346(1):11-22, 07 May 2013
Cited by: 40 articles | PMID: 23652222 | PMCID: PMC3684842
Knock-In Mice with NOP-eGFP Receptors Identify Receptor Cellular and Regional Localization.
J Neurosci, 35(33):11682-11693, 01 Aug 2015
Cited by: 40 articles | PMID: 26290245 | PMCID: PMC4540802
Nociceptin/orphanin FQ peptide receptor antagonist JTC-801 reverses pain and anxiety symptoms in a rat model of post-traumatic stress disorder.
Br J Pharmacol, 172(2):571-582, 01 Jul 2014
Cited by: 34 articles | PMID: 24666365 | PMCID: PMC4292969
The biology of Nociceptin/Orphanin FQ (N/OFQ) related to obesity, stress, anxiety, mood, and drug dependence.
Pharmacol Ther, 141(3):283-299, 01 Nov 2013
Cited by: 109 articles | PMID: 24189487 | PMCID: PMC5098338
Review Free full text in Europe PMC
Funding
Funders who supported this work.
NIDA NIH HHS (1)
Grant ID: R01 DA023281
National Institute on Drug Abuse (1)
Grant ID: DA023281