Abstract
Unlabelled
Few plant pathogens have had a more profound effect on the evolution of disease management than Erysiphe necator, which causes grapevine powdery mildew. When the pathogen first spread from North America to England in 1845, and onwards to France in 1847, 'germ theory' was neither understood among the general populace nor even generally accepted within the scientific community. Louis Pasteur had only recently reported the microbial nature of fermentation, and it would be another 30 years before Robert Koch would publish his proofs of the microbial nature of certain animal diseases. However, within 6 years after the arrival of the pathogen, nearly 6 million grape growers in France were routinely applying sulphur to suppress powdery mildew on nearly 2.5 million hectares of vineyards (Campbell, 2006). The pathogen has remained a focus for disease management efforts ever since. Because of the worldwide importance of the crop and its susceptibility to the disease, and because conventional management with modern, organic fungicides has been compromised on several occasions since 1980 by the evolution of fungicide resistance, there has also been a renewed effort worldwide to explore the pathogen's biology and ecology, its genetics and molecular interactions with host plants, and to refine current and suggest new management strategies. These latter aspects are the subject of our review.Taxonomy
The most widely accepted classification follows. Family Erysiphaceae, Erysiphe necator Schw. [syn. Uncinula necator (Schw.) Burr., E. tuckeri Berk., U. americana Howe and U. spiralis Berk. & Curt; anamorph Oidium tuckeri Berk.]. Erysiphe necator var. ampelopsidis was found on Parthenocissus spp. in North America according to Braun (1987), although later studies revealed isolates whose host range spanned genera, making the application of this taxon somewhat imprecise (Gadoury and Pearson, 1991). The classification of the genera before 1980 was based on features of the mature ascocarp: (i) numbers of asci; and (ii) morphology of the appendages, in particular the appendage tips. The foregoing has been supplanted by phylogeny inferred from the internal transcribed spacer (ITS) of ribosomal DNA sequences (Saenz and Taylor, 1999), which correlates with conidial ontogeny and morphology (Braun et al., 2002).Host range
The pathogen is obligately parasitic on genera within the Vitaceae, including Vitis, Cissus, Parthenocissus and Ampelopsis (Pearson and Gadoury, 1992). The most economically important host is grapevine (Vitis), particularly the European grape, V. vinifera, which is highly susceptible to powdery mildew. Disease symptoms and signs: In the strictest sense, macroscopically visible mildew colonies are signs of the pathogen rather than symptoms resulting from its infection, but, for convenience, we describe the symptoms and signs together as the collective appearance of colonized host tissues. All green tissues of the host may be infected. Ascospore colonies are most commonly found on the lower surface of the first-formed leaves near the bark of the vine, and may be accompanied by a similarly shaped chlorotic spot on the upper surface. Young colonies appear whitish and those that have not yet sporulated show a metallic sheen. They are roughly circular, ranging in size from a few millimetres to a centimetre or more in diameter, and can occur singly or in groups that coalesce to cover much of the leaf. Senescent colonies are greyish, and may bear cleistothecia in various stages of development. Dead epidermal cells often subtend the colonized area, as natural mortality in the mildew colony, the use of fungicides, mycoparasites or resistance responses in the leaf result in the deaths of segments of the mildew colony and infected epidermal cells. Severely affected leaves usually senesce, develop necrotic blotches and fall prematurely. Infection of stems initially produces symptoms similar to those on leaves, but colonies on shoots are eventually killed as periderm forms, producing a dark, web-like scar on the cane (Gadoury et al., 2011). Inflorescences and berries are most susceptible when young, and can become completely coated with whitish mildew. The growth of the berry epidermal tissue stops when severely infected, which may result in splitting as young fruit expand. Berries in a transitional stage between susceptible and resistant (generally between 3 and 4 weeks after anthesis) develop diffuse, nonsporulating mildew colonies only visible under magnification. Diffuse colonies die as berries continue to mature, leaving behind a network of necrotic epidermal cells (Gadoury et al., 2007). Survival over winter as mycelium in buds results in a distinctive foliar symptom. Shoots arising from these buds may be heavily coated with fungal growth, stark white in colour and stand out like white flags in the vine, resulting in the term 'flag shoots'. More commonly, colonization of a flag shoot is less extensive, and infection of a single leaf, or of leaves on one side of the shoot only, is observed (Gadoury et al., 2011).Free full text

Grapevine powdery mildew (Erysiphe necator): a fascinating system for the study of the biology, ecology and epidemiology of an obligate biotroph
SUMMARY
Few plant pathogens have had a more profound effect on the evolution of disease management than Erysiphe necator, which causes grapevine powdery mildew. When the pathogen first spread from North America to England in 1845, and onwards to France in 1847, ‘germ theory’ was neither understood among the general populace nor even generally accepted within the scientific community. Louis Pasteur had only recently reported the microbial nature of fermentation, and it would be another 30 years before Robert Koch would publish his proofs of the microbial nature of certain animal diseases. However, within 6 years after the arrival of the pathogen, nearly 6 million grape growers in France were routinely applying sulphur to suppress powdery mildew on nearly 2.5 million hectares of vineyards (Campbell, 2006). The pathogen has remained a focus for disease management efforts ever since. Because of the worldwide importance of the crop and its susceptibility to the disease, and because conventional management with modern, organic fungicides has been compromised on several occasions since 1980 by the evolution of fungicide resistance, there has also been a renewed effort worldwide to explore the pathogen's biology and ecology, its genetics and molecular interactions with host plants, and to refine current and suggest new management strategies. These latter aspects are the subject of our review.
Taxonomy: The most widely accepted classification follows. Family Erysiphaceae, Erysiphe necator Schw. [syn. Uncinula necator (Schw.) Burr., E. tuckeri Berk., U. americana Howe and U. spiralis Berk. & Curt; anamorph Oidium tuckeri Berk.]. Erysiphe necator var. ampelopsidis was found on Parthenocissus spp. in North America according to Braun (1987), although later studies revealed isolates whose host range spanned genera, making the application of this taxon somewhat imprecise (Gadoury and Pearson, 1991). The classification of the genera before 1980 was based on features of the mature ascocarp: (i) numbers of asci; and (ii) morphology of the appendages, in particular the appendage tips. The foregoing has been supplanted by phylogeny inferred from the internal transcribed spacer (ITS) of ribosomal DNA sequences (Saenz and Taylor, 1999), which correlates with conidial ontogeny and morphology (Braun etal., 2002).
Host range: The pathogen is obligately parasitic on genera within the Vitaceae, including Vitis, Cissus, Parthenocissus and Ampelopsis (Pearson and Gadoury, 1992). The most economically important host is grapevine (Vitis), particularly the European grape, V. vinifera, which is highly susceptible to powdery mildew.
Disease symptoms and signs: In the strictest sense, macroscopically visible mildew colonies are signs of the pathogen rather than symptoms resulting from its infection, but, for convenience, we describe the symptoms and signs together as the collective appearance of colonized host tissues. All green tissues of the host may be infected. Ascospore colonies are most commonly found on the lower surface of the first‐formed leaves near the bark of the vine, and may be accompanied by a similarly shaped chlorotic spot on the upper surface. Young colonies appear whitish and those that have not yet sporulated show a metallic sheen. They are roughly circular, ranging in size from a few millimetres to a centimetre or more in diameter, and can occur singly or in groups that coalesce to cover much of the leaf. Senescent colonies are greyish, and may bear cleistothecia in various stages of development. Dead epidermal cells often subtend the colonized area, as natural mortality in the mildew colony, the use of fungicides, mycoparasites or resistance responses in the leaf result in the deaths of segments of the mildew colony and infected epidermal cells. Severely affected leaves usually senesce, develop necrotic blotches and fall prematurely. Infection of stems initially produces symptoms similar to those on leaves, but colonies on shoots are eventually killed as periderm forms, producing a dark, web‐like scar on the cane (Gadoury etal., 2011).
Inflorescences and berries are most susceptible when young, and can become completely coated with whitish mildew. The growth of the berry epidermal tissue stops when severely infected, which may result in splitting as young fruit expand. Berries in a transitional stage between susceptible and resistant (generally between 3 and 4 weeks after anthesis) develop diffuse, nonsporulating mildew colonies only visible under magnification. Diffuse colonies die as berries continue to mature, leaving behind a network of necrotic epidermal cells (Gadoury etal., 2007).
Survival over winter as mycelium in buds results in a distinctive foliar symptom. Shoots arising from these buds may be heavily coated with fungal growth, stark white in colour and stand out like white flags in the vine, resulting in the term ‘flag shoots’. More commonly, colonization of a flag shoot is less extensive, and infection of a single leaf, or of leaves on one side of the shoot only, is observed (Gadoury etal., 2011).
MORPHOLOGY AND REPRODUCTIVE BIOLOGY
Hyphae of Erysiphe necator are 4–5µm in diameter, hyaline and superficial on epidermal cells, with multilobed appressoria at regular intervals. A penetration hypha from the lower surface of the appressorium pierces the cuticle and epidermal cell wall and is subtended by a globose haustorium, which envaginates the epidermal cell membrane (Fig.
1).
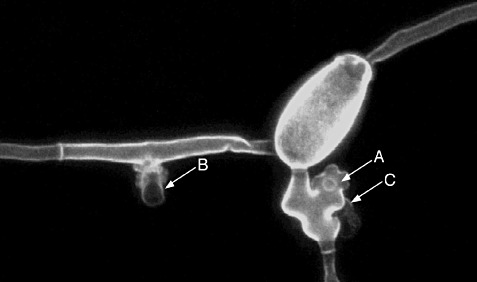
Laser scanning confocal micrograph of Erysiphe necator on an ontogenically susceptible leaf of Vitis vinifera at 72h post‐inoculation, stained with wheat germ agglutinin conjugated with Alexafluor‐488, showing the multilobed primary appressorium and penetration pore (A) and secondary germ tube with appressorium (B). The globose haustorium is faintly and partially visible at the lower left, beneath the primary appressorium (C).
Multiseptate conidiophores (10–400µm in height) form perpendicular to the epidermis, densely in rapidly growing colonies on susceptible tissue, less so on more resistant tissues, each producing a single hyaline, cylindro‐ovoid conidia (27–47
µm × 14–21
µm), with one to two large aqueous vacuoles per 24‐h cycle. Chains of conidia may accumulate in still air, the oldest conidium at the distal end. Long conidial chains are rarely seen under more turbulent field conditions. Conidia germinate via a single germ tube, which terminates in a lobed appressorium (Gadoury et
al., 2011).
Erysiphe necator has a bipolar‐heterothallic mating system (Evans etal., 1997; Gadoury and Pearson, 1991). At 14 days after pairing, mating within a collection of 35 isolates collected from several Vitis species, Parthenocissus quinquifolia and P. tricuspidata indicated the existence of two mutually exclusive mating types, which were present at similar frequencies (Gadoury and Pearson, 1991). However, in the same study, three of the 35 isolates retained the capacity to produce sparse, but fertile and functional, cleistothecia in protracted associations (i.e. 6–8 weeks after the initial pairing) with isolates of the same mating type. Thus, all isolates were readily compatible with an isolate of the opposite mating type. However, approximately 10% of the isolates produced a few fertile ascocarps when paired with a second isolate with which they were initially sexually incompatible. Gadoury and Pearson (1991) speculated that the delayed compatibility of these unusual isolates might explain the historical patterns of ascocarp development in Europe in the early 1900s.
Cleistothecia are initiated within 24h of contact between hyphae of compatible mating types at optimal temperatures (Gadoury and Pearson, 1988). Within 72
h, the hyaline ascocarp initial increases to 40
µm in diameter. Anchorage hyphae (Fig.
2) form as short outgrowths of cells of the outer ascocarp wall, become intertwined in the subtending mildew colony, but form no functional connections or appressoria (Gadoury and Pearson, 1988). Ascocarps become yellow by day 7 as a result of accumulation of a pigmented lipid (Gadoury and Pearson, 1990a). The upright, bristle‐like and equatorially arranged appendages are initiated after about 3 weeks. Cells of the outer ascocarp wall eventually darken, as do the basal cells of appendages, which form their characteristic uncinate tips about 4 weeks after ascocarp initiation (Gadoury and Pearson, 1988). At this time, the ascocarp wall is heavily melanized and dark brown in colour. Anchorage hyphae and the parent hyphae die after 4–5 weeks under typical field temperatures during mid‐ to late summer, and a basal concavity forms in the ascocarp, effectively disconnecting it from the mildew colony (Gadoury and Pearson, 1988).
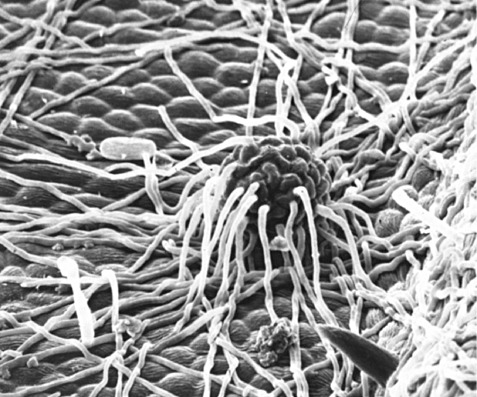
Immature cleistothecium of Erysiphe necator showing anchorage hyphae. These are distinct from the later‐forming and equatorially‐inserted appendages. The anchorage hyphae become intertwined in the mildew colony and serve to hold the developing ascocarp in place. Their necrosis is the first step towards detachment and dispersal of the cleistothecium to secondary substrates (principally the bark of the vine) for overwintering.
Cleistothecia contain four to six asci at maturity, each of which contains four to seven (usually four) hyaline, ovate to subglobose ascospores of 15–25µm × 10–14
µm (Gadoury et
al., 2011). Although ascocarps may reach morphological maturity in 4 weeks, physiological maturity may not be reached for several months, particularly in colder climates (Gadoury and Pearson, 1988; Gadoury et
al., 2011). Physiologically mature cleistothecia dehisce circumscissally when in contact with free water. No longer under the restriction of the ascocarp wall, the asci expand greatly and push back the upper half of the cleistothecium, and discharge ascospores forcefully through a slit‐like rupture of the ascus tip (Gadoury and Pearson, 1990a). Like conidia, ascospores germinate with a single germ tube, which terminates in a lobed appressorium (Gadoury and Pearson, 1990b).
EPIDEMIOLOGY
Many aspects of the epidemiology of grapevine powdery mildew were reviewed recently by Gadoury etal. (2011). In areas with relatively mild winters, dormant infected lateral buds give rise to flag shoots in the following spring (Hill, 1990; Pearson and Gärtel, 1985; Sall and Wyrsinski, 1982). The absence of flag shoots in colder viticultural regions, e.g. parts of Europe, eastern Washington State and New York State, USA, has been attributed to the reduced winter hardiness of the infected lateral buds (Hill, 1990; Pearson and Gadoury, 1992), as observed in the apple powdery mildew pathosystem (Spotts et
al., 1981); however, it is also possible that the pathogen itself is incapable of surviving low temperatures on dormant bud tissues in a nascent stage of establishment. Debilitation and hyphal mortality within colonies developing under low temperatures were recently demonstrated for nascent colonies on leaves (Moyer et
al., 2010).
The pathogen also overwinters in most viticultural areas as cleistothecia, providing an additional source of primary inoculum in regions in which flag shoots are common, and the principal to sole source where flag shoots are rare or absent. Cleistothecia are formed primarily on foliage, but also on berries, rachises or shoots (Pearson and Gadoury, 1987), once the disease severity has increased to a level at which pairing of compatible mating types on the same tissue becomes possible (Gadoury and Pearson, 1988). Morphologically mature cleistothecia are dispersed during rain events to either the bark of the vine or to the soil (Gadoury and Pearson, 1988). They may also be dispersed by extreme high winds (Grove, 2004). In areas in which cleistothecia develop by mid‐summer, e.g. the central coast of California, Italy and South Australia, ascospore release and resultant infection by some ascospores may occur late in the same growing season (Gadoury etal., 2011; Gee et
al., 2000; Rossi et
al., 2010). Otherwise, cleistothecia serve as overwintering fungal structures. Naked cleistothecia apparently do not survive the winter on or in the soil (Gadoury and Pearson, 1988). However, in climates with relatively low rainfall and mild winter temperatures (e.g. California, South Australia, Italy), they do not completely disperse from the leaves and can survive within leaf litter on the vineyard floor (Cortesi et
al., 1997; Magarey et
al., 1997). In the cold‐winter region of New York State, USA, survival appears to be confined to the bark of the vines.
In spring, ascospores are discharged when the ascocarps are wet by rain, irrigation or fog (Gadoury and Pearson, 1990a). Although ascospore release may be induced several weeks before bud break (Moyer etal., 2010), some are also conserved and released during rain events between bud break and bloom of the host. Similar periods of spring ascospore release have been reported in several other regions (Grove, 2004; Jailloux et
al., 1999; Rossi et
al., 2010; Rügner et
al., 2002). In the milder climates of California, Italy and South Australia, a significant proportion of cleistothecia may release ascospores during autumn or winter rains (Gadoury et
al., 2011; Gee et
al., 2000; Rossi et
al., 2010). Ascospore infection is favoured by precipitation in excess of 2–3
mm coincident with temperatures above 10
°C (Gadoury and Pearson, 1990b). Although free water is necessary for ascospore discharge, continued wetness is not required for ascospore germination and infection (1990a, 1990b). Definitive aerobiological studies have been limited by the relatively low density of ascospores in vineyard air, and the comparatively high threshold of detection of most mechanical samplers (Gadoury and Pearson, 1990a). However, the recent development of polymerase chain reaction (PCR)‐based detection methods (Falacy et
al., 2007) may allow more precise quantification of airborne ascospore dose at inoculum levels that typify the natural vineyard environment.
Where ascospores are the sole form of primary inoculum, new leaves may be infected as they form, but foliar disease severity does not increase markedly until after bloom (Gadoury etal., 1997). Where flag shoots serve as sources of primary inoculum, an additional level of complexity may be introduced into the development of the epidemic. A focus of disease develops around each flag shoot (Cortesi et
al., 2004), as intense conidial production spreads the disease to nearby shoots and vines. The infected lateral buds that give rise to flag shoots are typically infected at the early stages of an epidemic (Rumbolz and Gubler, 2004). Thus, early flag shoots also promote infection of new lateral buds, resulting in a tendency of flag shoots to appear repeatedly on the same vine or within the same area over consecutive years (2004, 2008; Sall and Wyrsinski, 1982; Ypema and Gubler, 2000).
Mildew colonies grow and sporulate most rapidly from 23 to 30°C, with an optimum of 26
°C (Delp, 1954). The latent period can be as short as 5 days under constant optimal temperatures, but increases substantially at lower temperatures, e.g. a minimum of 25 days at 9
°C, and under typical field conditions. The lower and upper limits for disease development are 6 and 32
°C, respectively (Delp, 1954). Germination of conidia is inhibited at 35
°C, and they are killed after sufficient exposure to 40
°C (Delp, 1954). Similarly, a substantial proportion of hyphae within mildew colonies may be killed by prolonged high temperatures, e.g. 10
h at 36
°C. However, some survival is common within the shaded interior of the canopy, and surviving portions of colonies may resporulate if temperatures again become favourable (Gadoury et
al., 2011).
The pathogen develops optimally at a relative humidity of approximately 85%, and progressively less so as air becomes drier (Carroll and Wilcox, 2003). Shading from direct sunlight increases disease, partly as a result of shielding from ultraviolet (UV) radiation to which E. necator is particularly vulnerable (Austin, 2010; Willocquet etal., 1996). Exposure to UV radiation reduces conidium germination, appressorium formation and subsequent colony expansion, and prolongs the latent period. Leaf surface temperatures in direct sunlight can be 10–15
°C higher than in shade, and can result in conditions lethal to the fungus on exposed vs. shaded tissues (Austin, 2010). Although free water is detrimental to conidial germination and may cause some conidia to lyse, this effect must be balanced against the favourable influences of moderate temperatures, limited direct sunlight and high humidity associated with rain events. Thus, the most severe mildew epidemics often accompany atypically rainy growing seasons or critical portions thereof (Gadoury et
al., 2011).
Ontogenic or age‐related resistance describes an increase in the ability of whole plants or plant tissues to resist pathogen infection as they age or mature (Develey‐Rivière and Galiana, 2007). For most grape cultivars, leaves are most susceptible to infection when half expanded, and susceptibility declines with further aging. However, leaves never become immune to infection, and older leaves can support substantial quantities of powdery mildew (Doster and Schnathorst, 1985b). In contrast, the period of fruit susceptibility is relatively brief. Individual berries of V. vinifera cultivars are highly susceptible to infection for the first 1–2 weeks after they have set (Gadoury etal., 2003). Ontogenic resistance is strongly expressed (Fig.
3) approximately 3–4 weeks after the completion of bloom in V. vinifera cultivars (2003, 2004; Gadoury et
al., 2003; Stark‐Urnau and Kast, 1999). Ontogenic resistance is broadly conserved across Vitis spp. (Gee et
al., 2008), but berries of less susceptible Vitis species and Vitis interspecific hybrids appear to express it 1–2 weeks earlier than those of V. vinifera (Gadoury et
al., 2001; Gee et
al., 2008). Diffuse powdery mildew colonies develop on berries as they transition to an ontogenically resistant state (Fig.
4), and are associated with increased severity of Botrytis bunch rot, elevated populations of epiphytic microorganisms and defects in wines (Gadoury et
al., 2007).
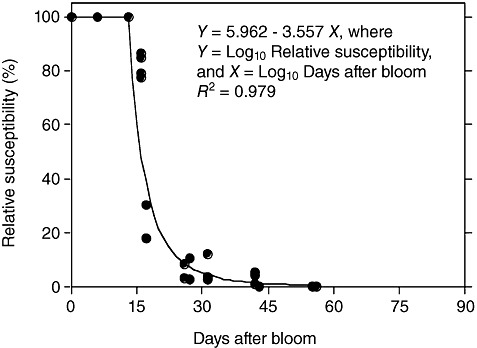
Development of ontogenic resistance to powdery mildew (Erysiphe necator) in berries of Vitis vinifera. Data from Gadoury etal. (2003).
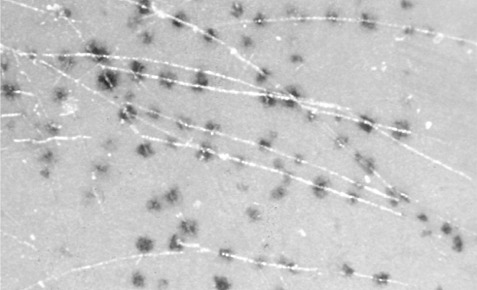
Epidermal necrosis at sites of appressorium formation following development of ontogenic resistance to Erysiphe necator in berries of Vitis vinifera. Berries infected at the transitional stage between susceptibility and resistance generally develop colonies of this phenotype, wherein infection results in a macroscopically invisible and nonsporulating colony that eventually dies as the epidermal tissues develop a high level of resistance to infection.
PATHOGEN BIOLOGY AND ECOLOGY
Pathogenic specialization, aggressiveness and race specificity
Pathogenic specialization in E. necator, defined as the differential ability of isolates to infect a host species, has been demonstrated only on Parthenocissus spp. and muscadine grapes, V. rotundifolia (syn. Muscadinia rotundifolia), but not among other Vitis spp. Not surprisingly, most isolates collected from Vitis spp. and from P. tricuspidata and P. quincefolia by Gadoury and Pearson (1991) appeared to be best adapted to the host genus or species from which they were obtained. For example, most isolates collected from Vitis were not able to infect P. tricuspidata or P. quincefolia (Gadoury and Pearson, 1991). Of 35 isolates collected from Vitis spp., the three isolates that infected P. tricuspidata were moderately aggressive on both Parthenocissus spp. and Vitis spp., whereas 13 isolates that infected P. quincefolia had much longer latent periods (some did not sporulate) and slower colony expansion rates than on Vitis. Conversely, isolates from P. quincefolia and P. tricuspidata were either not pathogenic on different host species or were weakly virulent. Brewer and Milgroom (2010) reported that sequences for two genes from E. necator isolates sampled from P. quincefolia were sufficiently divergent from those of isolates from Vitis spp. to place them in a different taxon. Thus, populations of E. necator from P. quinquifolia, a common weed in eastern North American vineyards, seem unlikely to affect powdery mildew epidemics on Vitis. Pathogenic specialization was also found on V. rotundifolia, which is an important source of powdery mildew resistance in Vitis breeding programmes. Frenkel etal. (2010) found that, among 38 isolates collected from cultivated and wild Vitis spp. in the eastern USA, only the 10 isolates collected from V. rotundifolia were able to form colonies on V. rotundifolia. Vitis rotundifolia is cultivated and grows wild in the southeastern USA sympatrically with other Vitis species, but there appears to be limited gene flow of E. necator between V. rotundifolia and other Vitis hosts. For all of these studies, however, only a single host genotype was used to represent each species.
Race specificity is similar to pathogenic specialization, but is conferred by single resistance genes (R genes) absent in some individuals within a host species. Although race‐specific resistance to powdery mildews has been proven in several other host plants (e.g. Coffey etal., 2006; Leus et
al., 2006; Sacristán et
al., 2009), little direct evidence has been available for E. necator—seen primarily as inconsistent resistance ratings among host genotypes over time or between locations (Cadle‐Davidson et
al., 2010b). Although environmental factors could underlie these inconsistencies, more compelling evidence for race specificity in the grape powdery mildew pathosystem has developed recently. Two breeding populations of V. vinifera were shown to segregate for powdery mildew R genes introgressed from wild Vitis species. Ratings for some individual progeny varied on the basis of the pathogen population used. Therefore, progeny were screened with diverse isolates collected by Frenkel et
al. (2010), and individual isolates were differentiated in their ability to overcome the R genes (L. Cadle‐Davidson, D. Ramming and M. G. Milgroom, unpublished data). As predicted by previous work on pathogenic specialization (Frenkel et
al., 2010; Gadoury and Pearson, 1991), the isolates that overcame each resistance were collected from the same host species as the source of resistance. This raises the question of whether the pathogenic specialization observed previously was a result of the action of single R genes, i.e. race specificity, present in only some genotypes of a species, or to more complex nonhost barriers of all host genotypes of a given species. Genetic studies in V. rotundifolia and Parthenocissus spp., or a broader survey of host genotypes, is needed to address this question.
Most isolates from Vitis appear to be equally aggressive on highly susceptible Vitis hosts. All isolates from a diverse collection of Vitis spp. in the USA were pathogenic on the Vitis interspecific hybrid cultivar Chancellor and on V. vinifera seedlings, but no variation in aggressiveness could be demonstrated (Gadoury and Pearson, 1991). However, on the more resistant V. labruscana, isolates differed greatly in the rate of colony expansion. Frenkel etal. (2010) found a similar relationship when comparing colony expansion and latent period among 60 isolates from various wild and cultivated Vitis species from the eastern and central USA. Thus, apparent differences in aggressiveness among isolates may actually reflect their differential responses to substantial host resistance. It is possible that aggressiveness on resistant hosts is not the result of an intrinsically robust growth of the individual isolate, but rather the ability to overcome quantitative host resistance (Gadoury and Pearson, 1991).
Prior vegetative growth, inoculum density, mating and light effects on conidiation
The initiation of asexual sporulation in E. necator is preceded by a period of superficial vegetative growth. Gadoury etal. (2010) reported that signalling in E. necator was promulgated at the colony centre and stimulated sporulation throughout the colony. Removal of the colony centre after signal promulgation had no impact on the timing of sporulation by 48‐h‐old hyphae at the colony margin. However, removal of the colony centre before signalling nearly doubled the latent period. A relationship between inoculum density and latent period was also observed, with latent period decreasing as the number of conidia deposited per square millimetre was increased. Rouse et
al. (1984) reported a similar relationship for E. graminis f. sp. tritici, wherein the number of spores produced per colony increased as the number of colonies per leaf increased. In E. necator, the effect was most pronounced at the lowest inoculum densities, with little decrease in the latent period as the density of inoculation increased above 10 spores/mm2. Light was also shown to be necessary to subsequently initiate the conidiation of competent colonies. Finally, hyphal contact between compatible mating types promulgated a signal with the opposite effect: sporulation ceased. The cessation of conidial production occurred simultaneously throughout the colony, even at points remote from the site of ascocarp initiation, including that from existing conidiophores.
Population biology of E. necator
Until recently, studies on the population genetics of E. necator were confined mostly to Europe and Australia, with a few small samples analysed from India, Tunisia and Israel (Délye etal., 1997b). In Europe and Australia, two genetically distinct groups, A and B (or I and III), were originally identified because of their marked differentiation with anonymous, dominant, multilocus genetic markers [e.g. random amplification of polymorphic DNAs (RAPDs) and amplified fragment length polymorphisms (AFLPs)] (Amrani and Corio‐Costet, 2006; Délye et
al., 1997b; Miazzi et
al., 2003; Núñez et
al., 2006; Péros et
al., 2005; Stummer et
al., 2000). Attempts to develop simpler markers to identify each group resulted in a few sequence‐characterized amplified region (SCAR) markers (Hajjeh et
al., 2005) and single‐nucleotide polymorphisms (SNPs) (Amrani and Corio‐Costet, 2006; 1997a, 1999; Péros et
al., 2005), which correlate with the two genetic groups.
The biological significance of groups A and B is unclear, but general patterns have emerged. Group A is often associated with flag shoots and is usually found early in the epidemic. Group A subpopulations tend to have little diversity, with most isolates being of the same mating type. Group B can also be associated with flag shoots or ascospore infections; it is frequently found later in the epidemic and subpopulations are more diverse than group A, with mating‐type ratios close to 1:1. These differences have led some authors to speculate that group A subpopulations are clonal, whereas group B subpopulations are sexual (Amrani and Corio‐Costet, 2006; Délye etal., 1997b; Montarry et
al., 2009; Péros et
al., 2005). Both groups can overwinter as mycelium in buds, and thus reference to group A as a ‘flag shoot biotype’ (Délye and Corio‐Costet, 1998; Délye et
al., 1997b) is imprecise. Flag shoot subpopulations in Italy may be highly clonal with a single mating type (Cortesi et
al., 2008), or have high genotypic diversity and 1:1 mating‐type ratios (Cortesi et
al., 2004; Miazzi et
al., 2003).
The two genetic groups are not randomly distributed, either spatially or temporally. Erysiphe necator populations in most of the vineyards sampled in Italy and France are composed of only one group (Miazzi etal., 2008; 2008, 2009). Where both occur in the same vineyard, group A is rarely found late in the epidemic. The cause of this pattern is unclear, but Miazzi et
al. (2008) speculated that it could be related to fungicide application and selection for fungicide resistance. The two groups differ in aggressiveness (measured by the components of spore germination, infection efficiency, latent period, lesion size and spore production), but group A is more aggressive for some components, whereas group B is more aggressive for others (Miazzi et
al., 2008; Montarry et
al., 2008). Overall, however, disease severity on leaves and clusters is positively correlated with the initial frequency of group B, possibly because it is more prevalent when berries are susceptible to infection (Montarry et
al., 2009).
Genetic differentiation between groups A and B reflects a lack of recombination between groups, even though they are interfertile and produce viable ascospores when mated in the laboratory (2003, 2008; Schneider etal., 1999; Stummer and Scott, 2003). One explanatory hypothesis is that temporal isolation is a prezygotic barrier to mating in the field (Miazzi et
al., 2008; Montarry et
al., 2008). An alternative hypothesis is postzygotic isolation, in which recombinants are less fit. The occurrence of mostly parental genotypes among progeny from a cross between the two genetic groups (Stummer and Scott, 2003) suggests a barrier to recombination, which would be consistent with a postzygotic isolation mechanism. Additional research is needed to determine the mechanisms responsible for reproductive isolation of the two groups.
Recent advances in the population genetics of E. necator have included the development of three new sets of molecular markers and the analysis of diverse populations in North America. The first set of genetic markers has extended the number of SNPs by multilocus sequencing of genes conserved among ascomycetes (Brewer and Milgroom, 2010). For the second, microsatellite markers (or simple sequence repeats, SSRs) were developed by mining transcriptome sequences (Frenkel etal., 2011). These first two sets of markers confirmed the existence of genetic groups A and B in Europe and Australia, and have opened up new possibilities for the study of the evolution of E. necator. The third marker is for idiomorphs at the mating‐type locus, MAT1, which have been identified and partially sequenced (Brewer et
al., 2011). Mating type can now be determined by PCR in E. necator and named according to the homology of MAT1 genes in ascomycetes. Degenerate primers designed for conserved regions of mating‐type genes have also been developed and are available to identify mating‐type genes in other powdery mildew fungi (Brewer et
al., 2011).
Diverse populations in eastern North America have been studied recently using new sets of genetic markers. If North America was the source of the introductions of E. necator to Europe, which was widely assumed (Weltzien, 1978), groups A and B should both be found in this putative source population. To test this hypothesis, Brewer and Milgroom (2010) sampled E. necator from cultivated grapevines and four wild Vitis species in the eastern USA and obtained partial nucleotide sequences from three genes. Populations in the eastern USA are significantly more diverse than introduced populations in Australia, France, Italy and the west coast of the USA. Isolates with haplotypes identical to genetic group A were common in the southeastern USA, whereas the only isolates with haplotypes of group B in the USA were from the west coast. The haplotypes closest to group B in the eastern USA were two nucleotide substitutions different from those of group B isolates from Europe. The positions of eastern USA haplotypes in the centre of a haplotype network, and group A and B haplotypes at the tips, on opposite sides of the network (Fig.5), indicates that the eastern USA is the likely ancestral population and potential source for two separate introductions of groups A and B to other populations.
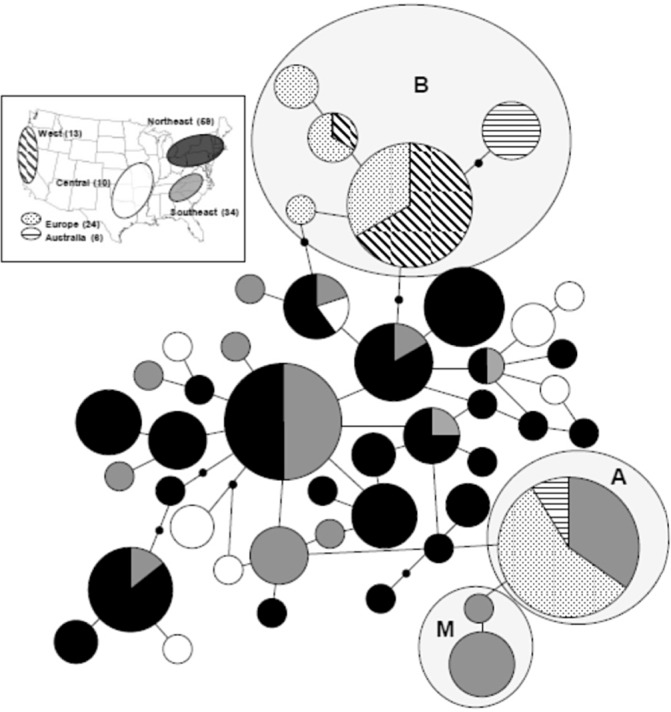
Multilocus haplotype network for Erysiphe necator sampled from North America, Europe and Australia (adapted from Brewer and Milgroom, 2010). Each haplotype is represented as a circle proportional in size to the number of isolates in each haplotype. Line segments connecting haplotypes represent single mutations; inferred intermediate haplotypes are represented by small solid dots. Geographical origins of isolates in each haplotype are proportionally represented in pie charts for each haplotype by patterns shown in the key at the top left; sample sizes for each geographical origin are also shown in the key. The haplotypes that include group A and group B isolates and isolates from muscadine grapes (Vitis rotundifolia) are enclosed in shaded ellipses labelled ‘A’, ‘B’ and ‘M’, respectively.
Further analysis of the E. necator population in the eastern USA compared genetic structure with geographical locations, host habitat (wild vs. cultivated) and host species with multilocus sequence data (Brewer and Milgroom, 2010). Multilocus sequencing revealed significant differentiation between populations in the northeast, southeast and central USA. However, this differentiation was not apparent when multilocus microsatellite haplotypes were assigned to populations in a Bayesian analysis (Frenkel etal., 2011). Genetic differentiation among host species was evident with both analyses, but only because of unique haplotypes in isolates collected from muscadine grapes, V. rotundifolia. No genetic differentiation was found among E. necator isolates from other Vitis hosts. Analyses based on either multilocus sequences or microsatellites failed to detect genetic differentiation between subpopulations from wild and cultivated hosts. Within all North American populations, both mating types are present at ratios close to 1:1 (Brewer et
al., 2011).
Genetics and genomics
Despite the economic importance of grape powdery mildew, few classical or molecular genetic resources have been developed. Although mating can be achieved easily under controlled conditions (Gadoury and Pearson, 1991), to our knowledge, only one study has genetically analysed progeny from a controlled cross (Stummer and Scott, 2003). The 18 progeny obtained in this cross exhibited anomalous segregation, possibly because the cross was between parents in genetic groups A and B. A definitive analysis of crosses will require recovery of mature ascospores in sufficiently large numbers to meaningfully study inheritance. Cleistothecia undergo a lengthy maturation period during overwintering, with few external clues of readiness to dehisce or discharge germinable ascospores (Gadoury and Pearson, 1990a, 1990b). Further progress in this area will require the development of methods to ‘farm’ larger numbers of cleistothecia and efficiently capture, establish and grow the progeny for analysis.
Genomic resources are similarly scarce for E. necator. Only a handful of nucleotide sequences are publicly available for E. necator, most of which have been described above because of their use as genetic markers. In part, this is a result of the inherent limitations of working with an obligate parasite. Recent advances in the isolation of RNA specifically from E. necator (Cadle‐Davidson etal., 2010a) have enabled the development of a reference transcriptome (Wakefield et
al., 2011), which has resulted in the identification of the mating‐type locus (MAT1) (Brewer et
al., 2011), ABC transporter genes putatively associated with fungicide resistance, microsatellite markers (Frenkel et
al., 2011) and candidate effector genes (L. Cadle‐Davidson and M. G. Milgroom, unpublished data). This transcriptome sequencing effort has served as a launching point for the annotation of differentially expressed transcript fragments previously identified by cDNA‐AFLP during E. necator growth and development (Wakefield et
al., 2011). In a broader context, a preliminary analysis of genes identified in the transcriptome of E. necator has shown that, as with other powdery mildew fungi (Spanu et
al., 2010), there are approximately 100 missing ascomycete core genes (MACGs) that are broadly conserved in yeasts and other ascomycetes. These 100 MACGs match almost perfectly with the MACGs absent from draft genomes of Blumeria graminis f. sp. hordei, E. pisi and Golovinomyces orontii. This reduction in gene number probably reflects the deletion of unnecessary genes for functions provided by the host in an obligate biotrophic interaction.
Although genome sequencing holds great promise for the identification of candidate genes, the biggest challenge for E. necator molecular genetics will be in testing gene function. For many traits, such as sporulation, gene function will need to be tested within the context of the E. necator genome. Thus far, however, only limited success has been achieved in transforming powdery mildew fungi (Chaure etal., 2000; Christiansen et
al., 1995). Although biolistic transformation has been reported for E. necator by Smith et
al. (1992), the lack of candidate genes until recently precluded further development of this method. Additional studies are needed to determine the stability of transformants.
DISEASE MANAGEMENT THROUGH CHEMICAL AND CULTURAL PRACTICES
Vitis is a genus composed of numerous widely dispersed and diverse taxa, yet nearly all of the world's commercial grape production is concentrated within a single species, V. vinifera, which is native to Europe and Asia Minor. Vitis vinifera thus evolved in isolation from E. necator, and the species is, with the rare exceptions described below (e.g. Ren1), highly susceptible to powdery mildew, with relatively minor differences among cultivars and selections. Many non‐vinifera grape species endemic to North America display varying levels of resistance to powdery mildew (Pearson and Gadoury, 1992). However, the cultivation of vines utilizing these sources of resistance is presently a viable management option only for commercial growers or hobbyists in regions in which end‐users will support the production of non‐vinifera grape species (e.g. V. rotundifolia) or interspecific hybrids that satisfy this criterion. The principal barriers to the exploitation of available genetic resources are thus market driven, and have much to do with perceived industry and consumer acceptance, and the quality of fruit and/or wine prepared from interspecific hybrid cultivars.
Although the control of powdery mildew in commercial vineyards generally requires the significant use of fungicides, vine canopy management is also an important component of integrated disease management programmes. Pruning and training systems that facilitate ventilation in the fruit cluster zone, as well as the physical removal of leaves immediately surrounding clusters, reduce microclimate humidity and promote sunlight exposure on berries (thereby subjecting the pathogen to solar radiation), and significantly reduce disease development (Austin and Wilcox, 2011; Austin etal., 2011). Numerous organic and inorganic fungicides are used to control E. necator. Sulphur is still widely used throughout the world because of its efficacy, low cost, lack of pathogen resistance and perception as a ‘natural’ substance. Sulphur provides protection and activity against established colonies. The superficial hyphae of E. necator render the pathogen susceptible to topical applications of an assortment of other materials that have little, if any, direct activity against pathogens other than powdery mildews, including botanical and petroleum‐based oils, inorganic salts and hydrogen peroxide. Fungicides used against E. necator include benzimidazoles, ergosterol biosynthesis inhibitors [both the sterol demethylation inhibitor (DMI) and morpholine subgroups], quinone‐outside inhibitor (QoI) compounds, such as the strobilurins, quinolines and the succinate dehydrogenase inhibitor (SDHI) group. Many of the foregoing fungicides have single‐site modes of action and resistance can develop from single base pair mutations. Erysiphe necator has exhibited a propensity to develop resistance to these materials when they are used repeatedly. Resistance to DMIs can evolve as the result of mutation in the 14‐α‐demethylase gene, CYP51 (Délye et
al., 1997a); however, quantitative variation in resistance phenotypes cannot be explained entirely by a single gene (Erickson and Wilcox, 1997; Ypema and Gubler, 1997). Some highly resistant isolates found in the eastern USA do not have the mutation in CYP51 typically found in resistant isolates (O. Frenkel, W. F. Wilcox, L. Cadle‐Davidson and M. G. Milgroom, unpublished data), suggesting the presence of additional mechanisms and genes involved in resistance to this class of compounds, perhaps including the efflux of fungicides by ABC transporters, for example, as found in other fungi (Kretschmer et
al., 2009). The timing of fungicide applications may be based on calendar days, phenology or weather‐driven advisory models (Gadoury et
al., 2011), the most widely deployed of which was described by Gubler et
al. (1999).
Temporal dynamics of ontogenic resistance (Fig.3) are now reflected in many management programmes. For example, the relatively brief period of high disease susceptibility for berries suggests that fungicide programmes should be especially diligent during this time. Such an approach has been validated experimentally (Gadoury et
al., 2003) and is now a standard recommendation to producers (Wilcox and Wolf, 2008). Similarly, leaf‐pulling treatments imposed 2 weeks after anthesis (whilst the berries are still highly susceptible to infection) have consistently reduced disease development on fruit clusters, whereas those imposed 5 weeks post‐anthesis (after berries have attained ontogenic resistance) have not (Austin and Wilcox, 2011).
NATURAL ENEMIES AND BIOLOGICAL CONTROL
There has been limited application of biological controls for grapevine powdery mildew. Several studies have indicated that natural enemies may have a substantial impact on pathogen survival in natural systems, but not to a degree that results in consistent and commercially relevant levels of disease suppression. The biological fungicide AQ10 is composed of propagules of a proprietary strain of the fungus Ampelomyces quisqualis, and exhibits modest efficacy against E. necator. In commercial vineyards, A. quisqualis commonly parasitizes mildew colonies late in the epidemic cycle (Falk etal., 1995b). Under high moisture conditions, it may destroy the capacity of mildew colonies to sporulate and can also infect and kill immature cleistothecia (Falk et
al., 1995a). This natural spread of the mycoparasite occurs too late in the epidemic cycle to affect the need for earlier fungicide sprays.
Orthotydeus lambi is a mycophagous mite that commonly inhabits wild riverbank grapevines (V. riparia) in eastern North America (English‐Loeb etal., 2005). In unmanaged vines, grazing of mildew colonies by O. lambi can reduce mildew to trace levels. Although the mite can also increase to disease‐suppressive levels in commercial vineyards, its populations are generally too low to control powdery mildew as a consequence of the routine use of sulphur, mancozeb and carbaryl, all of which are highly toxic to O. lambi (English‐Loeb et
al., 2007; Melidossian et
al., 2005). Until materials are available that control diseases other than powdery mildew, and are less harmful to mites, O. lambi is unlikely to be exploited as a biological control agent in commercial viticulture.
HOST RESISTANCE
Genetic variation in powdery mildew resistance across different Vitis species
North American Vitis spp. are more resistant to powdery mildew than most European V. vinifera cultivars (2010a, 2010b; Doster and Schnathorst, 1985a, 1985b; Eibach, 1994; Staudt, 1997). As a result, grape breeders in the late 1800s began to introduce genetic resistance from North American Vitis spp. into V. vinifera, resulting in many Vitis interspecific ‘French–American’ hybrids. Unfortunately, the perceived reduced quality of wine made from these resistant hybrids has significantly limited their adoption.
Based on research with Arabidopsis and barley, plants have apparently evolved a two‐layer strategy to combat powdery mildew infection (Dry etal., 2009). The first strategy, referred to as pathogen‐associated molecular pattern (PAMP)‐triggered immunity (PTI), involves the detection of the fungal PAMP chitin by a plasma membrane receptor‐like kinase (Miya et
al., 2007; Wan et
al., 2008). This, in turn, activates the PTI pathway, resulting in the secretion of cell wall‐reinforcing and antimicrobial molecules into the extracellular compartment to block powdery mildew penetration (Kwon, 2010). Powdery mildew species adapted to a particular host are presumed to suppress PTI through the secretion of effectors. In response, host plants have evolved a second layer of resistance, referred to as effector‐triggered immunity (ETI), whereby these PTI‐suppressing effector molecules are detected by an R gene that, in turn, activates a number of defence responses, including programmed cell death (PCD), which stop fungal development after successful penetration. Thus, insights into the mechanism of resistance can be gained from the quantification of the conidial fate (i.e. incidence of successful penetration and of colony establishment) 48–72
h post‐inoculation.
A good example of ETI against grapevine powdery mildew is found in V. rotundifolia, a native to the southeastern USA. Although this species is generally resistant to E. necator (Olmo, 1986), within‐species variation of resistance has been noted in several Vitis spp. (2010a, 2010b). One source of resistance from V. rotundifolia has been successfully introgressed into V. vinifera and shown to be conferred by a single dominant locus, designated Run1 (resistance to Uncinula necator 1; Table1), which provides complete resistance to E. necator in the V. vinifera background (Dry et
al., 2009; Pauquet et
al., 2001). Analysis of the Run1‐mediated resistance response indicates that it involves the induction of PCD within the penetrated epidermal cell, approximately 12–24
h following penetration, halting fungal development after the formation of a short secondary hypha (Fig.
6). Positional cloning of the Run1 locus has revealed the presence of a cluster of seven putative Toll/interleukin‐1 receptor‐nucleotide binding‐leucine‐rich repeat (TIR‐NB‐LRR)‐type resistance proteins (Barker et
al., 2005; Dry et
al., 2009). Although this locus appears to confer non‐race‐specific resistance in Europe and Australia, pathogenic specialization of isolates collected from the natural range of V. rotundifolia suggests that some individual R genes can be overcome by isolates of E. necator (Ramming et
al., 2011), raising questions about the durability of ETI resistance sources, such as Run1.
Table 1
Major powdery mildew resistance loci identified in grapevine.
Locus | Chromosome | Source of resistance | Origin of R loci | Reference |
---|---|---|---|---|
Ren1 | 13 | Vitis vinifera cv. ‘Kishmish vatkana’ | Central Asia |
Hoffmann et![]() |
Coleman et![]() | ||||
Ren2 | 14 | Vitis cinerea | North America |
Dalbo et![]() |
Ren3 | 15 | ‘Regent’* | North America |
Welter et![]() |
Ren4 | 18 | Vitis romanetti | Eastern Asia | D. W. Ramming and L. Cadle‐Davidson, unpublished data |
Run1 | 12 | Muscadinia rotundifolia | North America |
Pauquet et![]() |
Barker et![]() | ||||
Run2 | 18 | Muscadinia rotundifolia | North America |
Riaz et![]() |
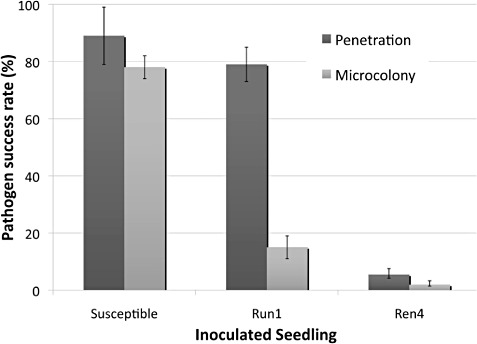
Percentage of successful penetration and microcolony formation of total counted conidia. Conidia penetrate and form colonies on susceptible Vitis vinifera, penetrate but are stopped within 48h post‐inoculation (hpi) prior to microcolony formation by Run1 (resistance to Uncinula necator 1) introgressed as a modified BC5 into Vitis vinifera, and fail to penetrate by 72
hpi on modified BC2 progeny carrying markers for Ren4 (resistance to Erysiphe necator 4) on chromosome 18.
A number of accessions of wild Chinese Vitis species also show significant resistance to E. necator (Wan etal., 2007). For example, a single dominant resistance locus, designated Ren4 (resistance to Erysiphe necator 4), from V. romanetii has been introgressed into V. vinifera and maps to chromosome 18 (Table
1; Mahanil et
al., 2011; Ramming et
al., 2011), which has the highest density of NB‐LRR genes in the grape genome. This dominant locus is unique among powdery mildew resistances in that it confers non‐race‐specific resistance that prevents hyphal growth (Fig.
6), reminiscent of PTI recessive R genes, such as mlo (mildew resistance locus o) (Buschges et
al., 1997). It will be intriguing to discover whether resistance in other Chinese grape species operates via reduced penetration, effector‐triggered induction of PCD, or a combination of both.
For two V. vinifera cultivars originating from Uzbekistan in Central Asia, ‘Kishmish vatkana’ and ‘Dzhandzhal kara’, resistance to E. necator has been reported to result from elevated levels of PCD in penetrated cells (Hoffmann etal., 2008). This resistance has been mapped to a single dominant locus (Ren1) on chromosome 13 that contains an NB‐LRR gene cluster (Coleman et
al., 2009).
Although the above three examples apparently fit into PTI or ETI phenotypes, partial resistance conferred by quantitative trait loci (QTLs) is poorly understood. Two loci which confer partial resistance to grapevine powdery mildew have been identified in North American Vitis species and map to a different chromosomal location. Dalbo etal. (2001) identified a major QTL (Ren2) for powdery mildew resistance derived from the V. cinerea parent in the interspecific hybrid ‘Illinois 547–1’. A second major QTL (Ren3) for powdery mildew resistance originating from an undetermined North American Vitis sp. has also been mapped in the Vitis interspecific hybrid ‘Regent’ (Welter et
al., 2007).
Contribution of other grapevine defence pathways to powdery mildew resistance
Various genes encoding grape defence‐related proteins, including pathogenesis‐related (PR) proteins, germin‐like proteins (GLPs) and stilbene synthases, are induced in response to powdery mildew infection in susceptible V. vinifera cultivars (Ficke etal., 2004; Fung et
al., 2008; Godfrey et
al., 2007; Jacobs et
al., 1999). Extractable levels of the PR proteins chitinase and β‐1,3‐glucanase were significantly increased in powdery mildew‐infected grape leaves and preveraison berries, and preparations of these proteins were shown to cause lysis of E. necator germ tubes in an in vitro bioassay (Giannakis et
al., 1998).
In cereals, certain members of the GLP gene family have been proposed to contribute to defence against Blumeria graminis infection, although the mode of action is not clear. Genes of the GLP subfamily 4 of barley (HvGLP4) and wheat (TaGLP4) were specifically expressed in powdery mildew‐penetrated epidermal cells (Schweizer etal., 1999; Wei et
al., 1998). Furthermore, transient RNAi silencing of HvGER4 and TaGLP4 reduced resistance against B. graminis in both cereals (Christensen et
al., 2004). Similarly, Ficke et
al. (2004) showed that the GLP gene, VvGLP3, was induced in ontogenically resistant (older), but not ontogenically susceptible (younger), grapevine berries following inoculation with E. necator. Godfrey et
al. (2007) showed that the induction of VvGLP3 was specific to infection by E. necator, and was not induced by other grapevine fungal pathogens or wounding, also suggesting a role in defence.
Not only are putative defence proteins synthesized in grapevine in response to powdery mildew infection, but there is also a significant increase in the transcription of genes involved in defence signal perception and signal transduction, including ENHANCED DISEASE SUSCEPTIBILITY1, mitogen‐activated protein kinases and WRKY transcription factors (Fung etal., 2008)
Despite the induction of multiple defence pathways in grapevine in response to powdery mildew infection, none of these defence proteins or pathways is capable of completely restricting E. necator growth and development on V. vinifera cultivars. Effects are presumed to be quantitative in nature, thereby explaining the minor differences in the susceptibility of different V. vinifera cultivars (Eibach, 1994). There may be a stronger role of these defence proteins in the reduced susceptibility of certain North American Vitis species exhibiting constitutively elevated levels of chitinase and β‐1,3‐glucanase in uninfected leaf tissues (Fung etal., 2008; Giannakis et
al., 1998).
Evidence for putative powdery mildew ‘susceptibility’ genes in grapevine
MLO proteins belong to a large family of seven‐transmembrane‐domain proteins found only in plants (Devoto etal., 1999). Although the biological function of most MLO family members remains elusive, there is now a large body of evidence suggesting that certain members of the MLO family predispose plants to powdery mildew infection, thereby acting as ‘susceptibility’ factors. For example, mutations in specific members of the MLO gene family from barley, tomato and Arabidopsis (either through natural genetic variation or targeted gene silencing) result in broad‐spectrum resistance to compatible powdery mildew isolates (Bai et
al., 2008; Consonni et
al., 2006; Jørgensen, 1992). Three of the 17 putative MLO genes in grapevine show a high degree of similarity, in terms of nucleotide sequence and transcriptional regulation, to the MLO‘susceptibility’ genes from barley, Arabidopsis and tomato, suggesting that they may have a role in regulating the susceptibility of grapevine to E. necator (Feechan et
al., 2008; Winterhagen et
al., 2008).
Developmental changes in host resistance
Both grapevine leaves and berries display ontogenic resistance to powdery mildew, although to differing degrees. Leaves of several Vitis species and interspecific hybrids display slightly reduced penetration and hyphal development of E. necator as they mature (Doster and Schnathorst, 1985b; Stein etal., 1985). Similarly, immature grape berries are highly susceptible to powdery mildew, but become immune to new infections within 2–4 weeks after bloom (Ficke et
al., 2003; Gadoury et
al., 2001). Interestingly, ontogenic resistance is sometimes expressed differently in separate tissues of the cluster, e.g. rachis, pedicels and berries (2001, 2003; Gee et
al., 2008). Previous studies on developing grape berries have shown that ontogenic resistance reduces the proportion of successful penetration, inhibits the growth of new and extant colonies and, through its effects on latent period and sporophore density, reduces the potential for secondary cycles of disease development (Ficke et
al., 2003). Conidial attachment, germination and appressorium formation were not affected by berry age. However, successful penetration of berries, formation of haustoria and development of secondary hyphae often declined to either nil or trace levels once ontogenic resistance was fully expressed in older berries (Ficke et
al., 2003; Gee et
al., 2008). The progress of E. necator was halted by ontogenic resistance in many cases before the formation of visible penetration pores. Hence, the mechanisms of ontogenic resistance operated at, or very near, the interface between the appressorium and the berry cuticle, and during the first few hours of the infection process. Neither cuticle nor cell wall thickness was causally associated with changes in ontogenic resistance (Ficke et
al., 2004). In the same study, polyphenolic synthesis and oxidation were responses to wounding during successful infection, and were therefore primarily restricted to young susceptible tissues and were not a principal cause of ontogenic resistance. Likewise, papillum formation beneath the first‐formed appressoria at 24
h post‐inoculation was primarily a response observed on susceptible berries coincident with effective penetration and establishment of the pathogen in susceptible berries, i.e. it did not completely contain the pathogen. In contrast, the pathogen was stopped prior to the penetration of resistant berries, and this thwarting of penetration most frequently occurred without subtending papillae (Ficke et
al., 2004).
Environmentally mediated changes in host resistance
As noted above, leaves of V. vinifera cultivars are most susceptible to infection by E. necator when they are approximately 50% expanded. However, recent field and laboratory studies have revealed that brief exposure of these otherwise susceptible leaves to nonfreezing low temperatures increases their resistance to infection (Moyer etal., 2010). Abiotic stresses, such as salt and heat, have also been reported to reduce host susceptibility in other powdery mildew pathosystems (Keller et
al., 2003; Schweizer et
al., 1995; Wiese et
al., 2004). Thomashow (1999) reported that the host response to cold temperature stress was similar to water stress in Arabidopsis. Other work has indicated the stimulation of the salicylic acid (SA) pathway in response to chilling (Wang and Hua, 2009). In the particular case of V. vinifera and E. necator, preinoculation exposure of susceptible V. vinifera leaves to temperatures of 2–4
°C for 2–8
h reduced subsequent infection efficiency and colony expansion. In separate experiments, nascent colonies exposed to similar cold events post‐inoculation exhibited hyphal mortality, reduced expansion and increased latent periods. Historical weather data and an analysis of the radiational cooling properties of leaf tissue have indicated that early‐season cold events capable of inducing the foregoing responses occur frequently across many, if not most, viticultural regions worldwide, and may partially explain: (i) the unexpectedly slow development of powdery mildew during the first month after budbreak (Gadoury et
al., 1997; Seem, 1984); and (ii) the sudden increase in epidemic development once seasonal temperatures remain above the threshold effecting acute cold responses (Moyer et
al., 2010).
FUTURE PROSPECTS AND CHALLENGES
Genetics and genomics
There are considerable challenges in advancing our knowledge of the genetics and genomics of E. necator. Classical genetics and linkage mapping cannot be accomplished until methods are optimized for maturing cleistothecia and for obtaining ascospore progeny from crosses. Association mapping might prove to be a viable alternative to linkage mapping, which will require the genotyping of isolates from natural recombining populations to find correlations between genotypes and phenotypes. Although E. necator is heterothallic, it remains to be seen whether populations are randomly mating in the eastern USA—this is a key assumption for association mapping.
Genomic studies of E. necator will also present significant challenges. The genomes of powdery mildew fungi are three to five times larger than those of most other ascomycetes because of the abundance of retroelements (Spanu etal., 2010), which greatly complicates sequencing and the assembly of genome sequences. Until significantly more funds or more efficient sequencing technologies for de novo genome assembly are available, researchers of E. necator must be content to work with transcriptome sequences. Although this has the advantage of targeting expressed genes, it has the disadvantage of missing genes that are expressed in the conditions or isolates used for mRNA isolation. It also means that promoter regions or other sequences that are not expressed cannot be determined, although these regulatory regions may be of interest in the understanding of gene expression.
Although genome sequencing holds great promise for the identification of candidate genes, the biggest challenge for molecular genetics will be in proving gene function within the context of the E. necator genome. Although biolistic transformation was demonstrated for E. necator in the early 1990s (Smith etal., 1992), and later for Blumeria graminis f. sp. hordei (Chaure et
al., 2000), stable transformants have not been produced in powdery mildew fungi beyond these original descriptions of the methods. Additional technical hurdles will need to be overcome to achieve these goals in developing stable transformation systems for E. necator.
Disease management: new technologies and approaches
Host resistance remains the ‘Holy Grail’ for the economically and environmentally sustainable management of powdery mildew and attendant production of high‐quality grapes. The reality that one or two genes conferring ETI may not be sufficient for durable resistance has been discussed above. Durability will be key to any successful disease‐resistance breeding strategy, as planting any cultivar entails an economic commitment of 20 or more years to the vineyard. The very limited success of previous conventional breeding efforts to impart powdery mildew resistance to V. vinifera through interspecific hybridization has also been discussed. A molecular breeding approach will be required to introgress and combine complementary mechanisms of resistance, whilst unlinking negative fruit quality traits. In addition, biotechnology provides the potential for the rapid development of resistant cultivars by the addition or deletion of one or a few genes, whilst maintaining the specific qualities and brand identities of current individual cultivars—a concept perhaps more commercially important for wine grapes than for nearly all other agricultural commodities. Candidate R genes for breeding or biotechnology will require functional characterization. As grapevine transformation is technically challenging and slow, Arabidopsis mutants that support E. necator penetration may provide an opportunity for the rapid characterization of host gene function in a heterologous system. For some period of time, however, the technical ability to produce disease‐resistant cultivars using the tools of biotechnology may outpace the commercial acceptance of the technology.
Other desirable disease management approaches will apply knowledge of fungal biology to reduce or specifically target fungicide applications. The potential exists to develop new biorational fungicides targeted at specific phases in the pathogen's life cycle. For example, studies being conducted on signalling pathways for synchronous conidiation within a powdery mildew colony might lead to the discovery of compounds that interfere with conidiation, thereby reducing secondary disease spread and epidemic development. Advances in our understanding of abiotic stress impacts on powdery mildew pathogenesis provide opportunities for both new manipulations to slow epidemic progress or improved predictive capabilities to know when to spray. Fungicide application will continue to be a prominent tool for disease management. Our improved knowledge of the molecular basis of fungicide resistance, and our consequent ability to detect and monitor the presence of resistant genotypes before they reach damaging frequencies, could help growers maintain effective fungicide programmes.
Because control tactics exert a selection pressure favouring pathogen individuals genetically capable of withstanding them, improved knowledge of the genetic diversity and evolutionary potential of powdery mildew populations should provide a strong warning about the prevention of the movement of powdery mildew from the eastern USA to other grape‐growing regions. Even with a subset of the E. necator genetic diversity, the major grape‐growing regions outside of the eastern USA struggle to manage powdery mildew sustainably, a struggle that would probably intensify with increased pathogen diversity.
REFERENCES
- Amrani, L. and Corio‐Costet, M.F. (2006) A single nucleotide polymorphism in the beta tubulin gene distinguishing two genotypes of Erysiphe necator expressing different symptoms on grapevine. Plant Pathol. 55, 505–512. [Google Scholar]
- Austin, C.N. (2010) Sunlight's influence on grapevine powdery mildew: direct effects on pathogen development and attendant consequences of canopy management and vineyard variability. PhD Thesis, Cornell University, Ithaca, NY.
- Austin, C.N. and Wilcox, W.F. (2011) Effects of fruit zone leaf removal, training system, and variable irrigation on powdery mildew development on Vitis vinifera L. Chardonnay. Am. J. Enol. Vitic. 10.5344/ajev.2010.10084. [Google Scholar]
- Austin, C.N. , Meyers, J. , Grove, G.G. and Wilcox, W.F. (2011) Quantification of powdery mildew severity as a function of canopy variability and associated impacts on sunlight penetration and spray coverage within the fruit zone. Am. J. Enol. Vitic. 62, 23–31. [Google Scholar]
- Bai, Y. , Pavan, S. , Zheng, Z. , Zappel, N.F. , Reinstädler, A. , Lotti, C. , De Giovanni, C. , Ricciardi, L. , Lindhout, P. , Visser, R. , Theres, K. and Panstruga, R. (2008) Naturally occurring broad‐spectrum powdery mildew resistance in a Central American tomato accession is caused by loss of Mlo function. Mol. Plant–Microbe Interact. 21, 30–39. [Abstract] [Google Scholar]
- Barker, C.L. , Donald, T. , Pauquet, J. , Ratnaparkhe, M.B. , Bouquet, A. , Adam‐Blondon, A.F. , Thomas, M.R. and Dry, I. (2005) Genetic and physical mapping of the grapevine powdery mildew resistance gene, Run1, using a bacterial artificial chromosome library. Theor. Appl. Genet. 111, 370–377. [Abstract] [Google Scholar]
- Braun, U. (1987) A monograph of the Erysiphales (powdery mildews). Beih. Nova Hedwigia 89, 1–700. [Google Scholar]
- Braun, U. , Cook, R.T.A. , Inman, A.J. and Shin, H.‐D. (2002) The taxonomy of the powdery mildew fungi In: The Powdery Mildews (Belanger R.R., Bushnell W.R., Dik A.J. and Carver T.L.W., eds), pp. 13–55. St. Paul, MN: APS Press. [Google Scholar]
- Brewer, M.T. and Milgroom, M.G. (2010) Phylogeography and population structure of the grape powdery mildew fungus, Erysiphe necator, from diverse Vitis species. BMC Evol. Biol. 10, 268. [Europe PMC free article] [Abstract] [Google Scholar]
- Brewer, M.T. , Cadle‐Davidson, L. , Cortesi, P. , Spanu, P.D. and Milgroom, M.G. (2011) Identification and structure of the mating‐type locus and development of PCR‐based markers for mating type in powdery mildew fungi. Fungal. Genet. Biol. 10.1016/j.fgb.2011.04.004. [Abstract] [Google Scholar]
- Buschges, R. , Hollricher, K. , Panstruga, R. , Simons, G. , Wolter, M. , Frijters, A. , van Daelen, R. , van der Lee, T. , Diergaarde, P. , Groenendijk, J. , Topsch, S. , Vos, P. , Salamini, F. and Schulze‐Lefert, P. (1997) The barley Mlo gene: a novel control element of plant pathogen resistance. Cell, 88, 695–705. [Abstract] [Google Scholar]
- Cadle‐Davidson, L. , Wakefield, L. , Seem, R.C. and Gadoury, D.M. (2010a) Specific isolation of RNA from the grape powdery mildew pathogen Erysiphe necator, an epiphytic, obligate parasite. J. Phytopathol. 158, 69–71. [Google Scholar]
- Cadle‐Davidson, L. , Chicoine, D.R. and Consolie, N.H. (2010b) Variation within and between Vitis species for foliar resistance to the powdery mildew pathogen Erysiphe necator . Plant Dis. 95, 202–211. [Abstract] [Google Scholar]
- Campbell, C. (2006) The Botanist and the Vintner. Chapel Hill, NC: Algonquin Books. [Google Scholar]
- Carroll, J.E. and Wilcox, W.F. (2003) Effects of humidity on the development of grapevine powdery mildew. Phytopathology, 93, 1137–1144. [Abstract] [Google Scholar]
- Chaure, P. , Gurr, S. and Spanu, P. (2000) Stable transformation of Erysiphe graminis, an obligate biotrophic pathogen of barley. Nat. Biotechnol. 18, 205–207. [Abstract] [Google Scholar]
- Christensen, A.B. , Thordal‐Christensen, H. , Zimmermann, G. , Gjetting, T. , Lyngkjær, M.F. , Dudler, R. and Schweizer, P. (2004) The germin‐like protein GLP4 exhibits superoxide dismutase activity and is an important component of quantitative resistance in wheat and barley. Mol. Plant–Microbe Interact. 17, 109–117. [Abstract] [Google Scholar]
- Christiansen, S.K. , Knudsen, S. and Giese, H. (1995) Biolistic transformation of the obligate plant pathogenic fungus, Erysiphe graminis f sp hordei . Curr. Genet. 29, 100–102. [Abstract] [Google Scholar]
- Coffey, M.D. , McCreight, J.D. and Miller, T. (2006) New races of the cucurbit powdery mildew Podosphaera xanthii present in California. Phytopathology, 96, S25. [Google Scholar]
- Coleman, C. , Copetti, D. , Cipriani, G. , Hoffman, S. , Kozman, P. , Kovacs, L. , Morgante, M. , Testolin, R. and Di Gaspero, G. (2009) The powdery mildew resistance gene REN1 co segregates with an NBS‐LRR gene cluster in two Central Asian grapevines. BMC Genet. 10, 89. [Europe PMC free article] [Abstract] [Google Scholar]
- Consonni, C. , Humphry, M.E. , Hartmann, H.A. , Livaja, M. , Durner, J. , Westphal, L. , Vogel, J. , Lipka, V. , Kemmerling, B. , Schulze‐Lefert, P. , Somerville, S.C. and Panstruga, R. (2006) Conserved requirement for a plant host cell protein in powdery mildew pathogenesis. Nat. Genet. 38, 716–720. [Abstract] [Google Scholar]
- Cortesi, P. , Bisiach, M. , Ricciolini, M. and Gadoury, D.M. (1997) Cleistothecia of Uncinula necator: an additional source of inoculum in Italian vineyards. Plant Dis. 81, 922–926. [Abstract] [Google Scholar]
- Cortesi, P. , Ottaviani, M.‐P. and Milgroom, M.G. (2004) Spatial and genetic analysis of a flag shoot subpopulation of Erysiphe necator . Phytopathology, 94, 544–550. [Abstract] [Google Scholar]
- Cortesi, P. , Pizzatti, C. , Bertocchi, D. and Milgroom, M.G. (2008) Persistence and spatial autocorrelation of clones of Erysiphe necator overwintering as mycelium in dormant buds in an isolated vineyard in northern Italy. Phytopathology, 98, 148–152. [Abstract] [Google Scholar]
- Dalbo, M.A. , Ye, G.N. , Weeden, N.F. , Wilcox, W.F. and Reisch, B.I. (2001) Marker assisted selection for powdery mildew resistance in grapes. J. Am. Soc. Hortic. Sci. 126, 83–89. [Google Scholar]
- Delp, C.J. (1954) Effects of temperature and humidity on the grape powdery mildew fungus. Phytopathology, 44, 615–626. [Google Scholar]
- Délye, C. and Corio‐Costet, M.F. (1998) Origin of primary infections of grape by Uncinula necator: RAPD analysis discriminates two biotypes. Mycol. Res. 102, 283–288. [Google Scholar]
- Délye, C. , Laigret, F. and Corio‐Costet, M.F. (1997a) A mutation in the 14‐alpha demethylase gene of Uncinula necator that correlates with resistance to a sterol biosynthesis inhibitor. Appl. Environ. Microbiol. 63, 2966–2970. [Europe PMC free article] [Abstract] [Google Scholar]
- Délye, C. , Laigret, F. and Corio‐Costet, M.F. (1997b) RAPD analysis provides insight into the biology and epidemiology of Uncinula necator . Phytopathology, 87, 670–677. [Abstract] [Google Scholar]
- Délye, C. , Ronchi, V. , Laigret, F. and Corio‐Costet, M.F. (1999) Nested allele‐specific PCR primers distinguish genetic groups of Uncinula necator . Appl. Environ. Microbiol. 65, 3950–3954. [Europe PMC free article] [Abstract] [Google Scholar]
- Develey‐Rivière, M.‐P. and Galiana, E. (2007) Resistance to pathogens and host developmental stage: a multifaceted relationship within the plant kingdom. New Phytol. 175, 405–416. [Abstract] [Google Scholar]
- Devoto, A. , Piffanelli, P. , Nilsson, I. , Wallin, E. , Panstruga, R. , von Heijne, G. and Schulze Lefert, P. (1999) Topology, subcellular localization, and sequence diversity of the MLO family in plants. J. Biol. Chem. 274, 34993–35004. [Abstract] [Google Scholar]
- Doster, M.A. and Schnathorst, W.C. (1985a) Comparative susceptibility of various grapevine cultivars to the powdery mildew fungus Uncinula necator . Am. J. Enol. Vitic. 36, 101–104. [Google Scholar]
- Doster, M.A. and Schnathorst, W.C. (1985b) Effects of leaf maturity and cultivar resistance on development of the powdery mildew fungus of grapevines. Phytopathology, 75, 318–321. [Google Scholar]
- Dry, I.B. , Feechan, A. , Anderson, C. , Jermakow, A.M. , Bouquet, A. , Anne, F. , Adam Blondon, A.‐F. and Thomas, M.R. (2009) Molecular strategies to enhance the genetic resistance of grapevines to powdery mildew. Aust. J. Grape Wine Res. 16, 94–105. [Google Scholar]
- Eibach, R. (1994) Investigations about the genetic‐resources of grapes with regard to resistance characteristics to powdery mildew (Oidium‐Tuckeri). Vitis, 33, 143–150. [Google Scholar]
- English‐Loeb, G. , Norton, A.P. , Gadoury, D.M. , Seem, R. and Wilcox, W. (2005) Tri‐trophic interactions among grapevines, a fungal pathogen, and a mycophagous mite. Ecol. Applications, 15, 1679–1688. [Google Scholar]
- English‐Loeb, G. , Norton, A.P. , Gadoury, D.M. , Seem, R.C. and Wilcox, W.F. (2007) Biological control of grape powdery mildew using mycophagous mites. Plant Dis. 91, 421–429. [Abstract] [Google Scholar]
- Erickson, E.O. and Wilcox, W.F. (1997) Distributions of sensitivities to three sterol demethylation inhibitor fungicides among populations of Uncinula necator sensitive and resistant to triadimefon. Phytopathology, 87, 784–791. [Abstract] [Google Scholar]
- Evans, K.J. , Scott, E.S. and Whisson, D.L. (1997) Heterothallism among South Australian clonal lines of Uncinula necator . Australas. Plant Pathol. 26, 10–20. [Google Scholar]
- Falacy, J.S. , Grove, G.G. , Mahaffee, W. , Larsen, R.C. , Vandermark, G.J. , Glawe, D.A. and Galloway, H. (2007) Detection of Erysiphe necator (Uncinula necator) with polymerase chain reaction and species‐specific primers. Phytopathology, 97, 1290–1297. [Abstract] [Google Scholar]
- Falk, S.P. , Gadoury, D.M. , Cortesi, P. , Pearson, R.C. and Seem, R.C. (1995a) Parasitism of cleistothecia of Uncinula necator by the mycoparasite Ampelomyces quisqualis . Phytopathology, 85, 794–800. [Google Scholar]
- Falk, S.P. , Gadoury, D.M. , Pearson, R.C. and Seem, R.C. (1995b) Partial control of grape powdery mildew by the mycoparasite Ampelomyces quisqualis . Plant Dis. 79, 483–490. [Google Scholar]
- Feechan, A. , Jermakow, A.M. , Torregrosa, L. , Panstruga, R. and Dry, I.B. (2008) Identification of grapevine MLO gene candidates involved in susceptibility to powdery mildew. Funct. Plant Biol. 35, 1255–1266. [Abstract] [Google Scholar]
- Ficke, A. , Gadoury, D.M. , Seem, R.C. and Dry, I.B. (2003) Effects of ontogenic resistance upon establishment and growth of Uncinula necator on grape berries. Phytopathology, 93, 556–563. [Abstract] [Google Scholar]
- Ficke, A. , Gadoury, D.M. , Seem, R.C. , Godfrey, D. and Dry, I.B. (2004) Host barriers and responses to Uncinula necator in developing grape berries. Phytopathology, 94, 438–445. [Abstract] [Google Scholar]
- Frenkel, O. , Brewer, M.T. and Milgroom, M.G. (2010) Variation in pathogenicity and aggressiveness of Erysiphe necator from different Vitis spp. and geographic origins in the eastern United States. Phytopathology, 100, 1185–1193. [Abstract] [Google Scholar]
- Frenkel, O. , Portillo, I. , Brewer, M.T. , Peros, J.‐P. , Cadle‐Davidson, L. and Milgroom, M.G. (2011) Development of microsatellite markers from the transcriptome of Erysiphe necator for analyzing population structure in North America and Europe. Plant Pathol. (in press). [Google Scholar]
- Fung, R.W.M. , Gonzalo, M. , Fekete, C. , Kovacs, L.G. , He, Y. , Marsh, E. , McIntyre, L.M. , Schachtman, D.P. and Qiu, W.P. (2008) Powdery mildew induces defense oriented reprogramming of the transcriptome in a susceptible but not in a resistant grapevine. Plant Physiol. 146, 236–249. [Abstract] [Google Scholar]
- Gadoury, D.M. and Pearson, R.C. (1988) Initiation, development, dispersal, and survival of cleistothecia of Uncinula necator in New York vineyards. Phytopathology, 78, 1413–1421. [Google Scholar]
- Gadoury, D.M. and Pearson, R.C. (1990a) Ascocarp dehiscence and ascospore discharge in Uncinula necator . Phytopathology, 80, 393–401. [Google Scholar]
- Gadoury, D.M. and Pearson, R.C. (1990b) Germination of ascospores and infection of Vitis by Uncinula necator . Phytopathology, 80, 1198–1203. [Google Scholar]
- Gadoury, D.M. and Pearson, R.C. (1991) Heterothallism and pathogenic specialization in Uncinula necator . Phytopathology, 81, 1287–1293. [Google Scholar]
- Gadoury, D.M. , Seem, R.C. , Magarey, P.A. , Emmett, R. and Magarey, R. (1997) Effects of environment and fungicides on epidemics of grape powdery mildew: considerations for practical model development and disease management. Vitic. Enol. Sci. 52, 225–229. [Google Scholar]
- Gadoury, D.M. , Seem, R.C. , Ficke, A. and Wilcox, W.F. (2001) The epidemiology of powdery mildew on Concord grapes. Phytopathology, 91, 948–955. [Abstract] [Google Scholar]
- Gadoury, D.M. , Seem, R.C. , Ficke, A. and Wilcox, W.F. (2003) Ontogenic resistance to powdery mildew in grape berries. Phytopathology, 93, 547–555. [Abstract] [Google Scholar]
- Gadoury, D.M. , Seem, R.C. , Wilcox, W.F. , Henick‐Kling, T. , Conterno, L. , Day, A. and Ficke, A. (2007) Effects of diffuse colonization of grape berries by Uncinula necator on bunch rots, berry microflora, and juice and wine quality. Phytopathology, 97, 1356–1365. [Abstract] [Google Scholar]
- Gadoury, D.M. , Wakefield, L.M. , Seem, R.C. , Cadle‐Davidson, L. and Dry, I.B. (2010) Effect of prior vegetative growth, inoculum density and light on conidiation in Erysiphe necator In: Proceedings of the 6th International Workshop on Grapevine Downy and Powdery Mildew (Calonnec A., Delmotte F., Emmett R., Gadoury D., Gessler C., Gubler W.D., Kassemeyer H.‐H., Magarey P., Raynal M. and Seem R., eds), pp. 51–53. San Michele, Italy: Istituto Agrario San Michele all'Adige. [Google Scholar]
- Gadoury, D.M. , Wilcox, W.F. , Rumbolz, J. and Gubler, W.D. (2011) Powdery mildew In: Compendium of Grapevine Diseases (2nd edn.) (Wilcox W.F., Gubler W.D. and Uyemoto J., eds). APS Press; (in press). [Google Scholar]
- Gee, C.T. , Gadoury, D.M. and Cadle‐Davidson, L. (2008) Ontogenic resistance to Uncinula necator varies by genotype and tissue type in a diverse collection of Vitis spp. Plant Dis. 92, 1067–1073. [Abstract] [Google Scholar]
- Gee, L.M. , Stummer, B.E. , Gadoury, D.M. , Biggins, L.T. and Scott, E.S. (2000) Maturation of cleistothecia of Uncinula necator (powdery mildew) and release of ascospores in southern Australia. Aust. J. Grape Wine Res. 6, 13–20. [Google Scholar]
- Giannakis, C. , Bucheli, C.S. , Skene, K.G.M. , Robinson, S.P. and Scott, N.S. (1998) Chitinase and beta‐1,3‐glucanase in grapevine leaves: a possible defence against powdery mildew infection. Aust. J. Grape Wine Res. 4, 14–22. [Google Scholar]
- Godfrey, D. , Able, A.J. and Dry, I.B. (2007) Induction of a grapevine germin‐like protein (VvGLP3) gene is closely linked to the site of Erysiphe necator infection: a possible role in defence. Mol. Plant–Microbe Interact. 20, 1112–1125. [Abstract] [Google Scholar]
- Grove, G.G. (2004) Perennation of Uncinula necator in vineyards of Eastern Washington. Plant Dis. 88, 242–247. [Abstract] [Google Scholar]
- Gubler, W.D. , Rademacher, M. , Vasquez, S.J. and Thomas, C.S. (1999) Control of powdery mildew using the UC Davis powdery mildew risk index. American Phytopathological Society. Available at http://www.apsnet.org/online/feature/pmildew/top.html[accessed 15 August 2010].
- Hajjeh, H. , Miazzi, M. , De Guido, M.A. and Faretra, F. (2005) Specific scar primers for the ‘flag shoot’ and ‘ascospore’ biotypes of the grape powdery mildew fungus Erysiphe necator . J. Plant Pathol. 87, 71–74. [Google Scholar]
- Hill, G.K. (1990) The influence of annual weather patterns on epidemics of Uncinula necator in Rheinhessen. Vitic. Enol. Sci. 45, 43–46. [Google Scholar]
- Hoffmann, S. , Di Gaspero, G. , Kovács, L. , Howard, S. , Kiss, E. , Galbács, Z. , Testolin, R. and Kozma, P. (2008) Resistance to Erysiphe necator in the grapevine ‘Kishmish vatkana’ is controlled by a single locus through restriction of hyphal growth. Theor. Appl. Genet. 116, 427–438. [Abstract] [Google Scholar]
- Jacobs, A.K. , Dry, I.B. and Robinson, S.P. (1999) Powdery mildew infection and ethylene treatment induce different pathogenesis‐related cDNAs in grapevine. Plant Pathol. 48, 325–336. [Google Scholar]
- Jailloux, F. , Willocquet, L. , Chapuis, L. and Froidefond, G. (1999) Effect of weather factors on the release of ascospores of Uncinula necator, the cause of grape powdery mildew, in Bordeaux region. Can. J. Bot. 77, 1044–1051. [Google Scholar]
- Jørgensen, J.H. (1992) Discovery, characterization and exploitation of Mlo powdery mildew resistance in barley. Euphytica, 63, 141–152. [Google Scholar]
- Keller, M. , Rogiers, S.Y. and Schultz, H.R. (2003) Nitrogen and ultraviolet radiation modify grapevines' susceptibility to powdery mildew. Vitis, 42, 87–94. [Google Scholar]
- Kretschmer, M. , Leroch, M. , Mosbach, A. , Walker, A.S. , Fillinger, S. , Mernke, D. , Schoonbeek, H.‐J. , Pradier, J.‐M. , Leroux, P. , De Waard, M.A. and Hahn, M. (2009) Fungicide‐driven evolution and molecular basis of multidrug resistance in field populations of the grey mould fungus Botrytis cinerea . Plos. Pathogens, 5, e1000696. [Europe PMC free article] [Abstract] [Google Scholar]
- Kwon, C. (2010) Plant defense responses coming to shape. Plant Physiol. J. 26, 115–120. [Google Scholar]
- Leus, L. , Dewitte, A. , Van Huylenbroeck, J. , Vanhoutte, N. , Van Bockstaele, E. and Hofte, M. (2006) Podosphaera pannosa (syn. Sphaerotheca pannosa) on Rosa and Prunus spp.: characterization of pathotypes by differential plant reactions and ITS sequences. J. Phytopathol. 154, 23–28. [Google Scholar]
- Magarey, P.A. , Gadoury, D.M. , Emmett, R.W. , Biggins, L.T. , Clarke, K. , Wachtel, M.F. , Wicks, T.J. and Seem, R.C. (1997) Cleistothecia of Uncinula necator in Australia. Vitic. Enol. Sci. 52, 210–218. [Google Scholar]
- Mahanil, S. , Lagerholm, S. , Garris, A. , Owens, C.L. , Ramming, D.W. and Cadle‐Davidson, L. (2011) Development of molecular markers for powdery mildew resistance in grapevines. Acta Hortic. (in press). [Google Scholar]
- Melidossian, H.S. , Seem, R.C. , English‐Loeb, G. , Wilcox, W.F. and Gadoury, D.M. (2005) Suppression of grapevine powdery mildew by a mycophagous mite. Plant Dis. 89, 1331–1338. [Abstract] [Google Scholar]
- Miazzi, M. , Hajjeh, H. and Faretra, F. (2003) Observations on the population biology of the grape powdery mildew fungus Uncinula necator . J. Plant Pathol. 85, 123–129. [Google Scholar]
- Miazzi, M. , Hajjeh, H. and Faretra, F. (2008) Occurrence and distribution of two distinct genetic groups in populations of Erysiphe necator Schw. in southern Italy. J. Plant Pathol. 90, 563–573. [Google Scholar]
- Miya, A. , Albert, P. , Shinya, T. , Desaki, Y. , Ichimura, K. , Shirasu, K. , Narusaka, Y. , Kawakami, N. , Kaku, H. and Shibuya, N. (2007) CERK1, a LysM receptor kinase, is essential for chitin elicitor signaling in Arabidopsis. Proc. Natl. Acad. Sci. USA, 104, 19613–19618. [Europe PMC free article] [Abstract] [Google Scholar]
- Montarry, J. , Cartolaro, P. , Delmotte, F. , Jolivet, J. and Willocquet, L. (2008) Genetic structure and aggressiveness of Erysiphe necator populations during grapevine powdery mildew epidemics. Appl. Environ. Microbiol. 74, 6327–6332. [Europe PMC free article] [Abstract] [Google Scholar]
- Montarry, J. , Cartolaro, P. , Richard‐Cervera, S. and Delmotte, F. (2009) Spatio‐temporal distribution of Erysiphe necator genetic groups and their relationship with disease levels in vineyards. Eur. J. Plant Pathol. 123, 61–70. [Google Scholar]
- Moyer, M.M. , Gadoury, D.M. , Cadle‐Davidson, L. , Dry, I.B. , Magarey, P.A. , Wilcox, W.F. and Seem, R.C. (2010) Effects of acute low temperature events on development of Erysiphe necator and susceptibility of Vitis vinifera . Phytopathology, 100, 1240–1249. [Abstract] [Google Scholar]
- Núñez, Y. , Gallego, J. , Ponz, F. and Raposo, R. (2006) Analysis of population structure of Erysiphe necator using AFLP markers. Plant Pathol. 55, 650–656. [Google Scholar]
- Olmo, H.P. (1986) The potential role of (vinifera ×rotundifolia) hybrids in grape variety improvement. Experientia, 42, 921–926. [Google Scholar]
- Pauquet, J. , Bouquet, A. , This, P. and Adam‐Blondon, A.‐F. (2001) Establishment of a local map of AFLP markers around the powdery mildew resistance gene Run1 in grapevine and assessment of their usefulness for marker assisted selection. Theor. Appl. Genet. 103, 1201–1210. [Google Scholar]
- Pearson, R.C. and Gadoury, D.M. (1987) Cleistothecia, the source of primary inoculum for grape powdery mildew in New York. Phytopathology, 77, 1509–1514. [Google Scholar]
- Pearson, R.C. and Gadoury, D.M. (1992) Grape powdery mildew In: Plant Diseases of International Importance, Vol. III. Diseases of Fruit Crops (Kumar J., Chaube H.S., Singh U.S. and Mukhopadhyay A.N., eds), pp. 129–146. Englewood Cliffs, NJ: Prentice Hall. [Google Scholar]
- Pearson, R.C. and Gärtel, W. (1985) Occurrence of hyphae of Uncinula necator in buds of grapevine. Plant Dis. 69, 149–151. [Google Scholar]
- Péros, J.‐P. , Troulet, C. , Guerriero, M. , Michel‐Romiti, C. and Notteghem, J.L. (2005) Genetic variation and population structure of the grape powdery mildew fungus, Erysiphe necator, in southern France. Eur. J. Plant Pathol. 113, 407–416. [Google Scholar]
- Ramming, D.W. , Gabler, F. , Smilanick, J. , Cadle‐Davidson, M. , Barba, P. , Mahanil, S. and Cadle‐Davidson, L. (2011) A single dominant locus Ren4 confers non‐race‐specific penetration resistance to grapevine powdery mildew. Phytopathology, 101, 502–508. [Abstract] [Google Scholar]
- Riaz, S. , Tenscher, A.C. , Ramming, D.W. and Walker, M.A. (2011) Using a limited mapping strategy to identify major QTLs for resistance to grapevine powdery mildew (Erysiphe necator) and their use in marker‐assisted breeding. Theor. Appl. Genet. 122, 1059–1073. [Europe PMC free article] [Abstract] [Google Scholar]
- Rossi, V. , Caffi, T. and Leger, S.E. (2010) Dynamics of ascospore maturation and discharge in Erysiphe necator, the causal agent of grape powdery mildew. Phytopathology, 100, 1321–1329. [Abstract] [Google Scholar]
- Rouse, D.I. , MacKenzie, D.R. and Nelson, R.R. (1984) Density dependent sporulation of Erysiphe graminis f. sp. tritici . Phytopathology, 74, 1176–1180. [Google Scholar]
- Rügner, A. , Rumbolz, J. , Huber, B. , Bleyer, G. , Gisi, G. , Kassemeyer, H. and Guggenheim, R. (2002) Formation of overwintering structures of Uncinula necator and colonization of grapevine under field conditions. Eur. J. Plant Pathol. 21, 322–330. [Google Scholar]
- Rumbolz, J. and Gubler, W.D. (2004) Susceptibility of grapevine buds to infection by grapevine powdery mildew fungus Uncinula necator (Schw.) Burr. Plant Pathol. 54, 535–548. [Google Scholar]
- Sacristán, S. , Vigouroux, M. , Pedersen, C. , Skamnioti, P. , Thordal‐Christensen, H. , Micali, C. , Brown, J.K.M. and Ridout, C.J. (2009) Coevolution between a family of parasite virulence effectors and a class of LINE‐1 retrotransposons. PLoS ONE, 4, 10. [Europe PMC free article] [Abstract] [Google Scholar]
- Saenz, G.S. and Taylor, J.W. (1999) Phylogeny of the Erysiphales (powdery mildews) inferred from internal transcribed spacer ribosomal DNA sequences. Can. J. Bot. 77, 150–168. [Google Scholar]
- Sall, M.A. and Wyrsinski, J. (1982) Perennation of powdery mildew in buds of grapevines. Plant Dis. 66, 678–679. [Google Scholar]
- Schneider, S. , Stummer, B. , Kassemeyer, H.‐H. , Blaich, R. , Scott, E. , Seem, R.C. and Gadoury, D.M. (1999) Heterothallism and compatibility of ascospore‐ and flag shoot‐derived isolates of Uncinula necator In: Proceedings of the 1st International Conference on Powdery Mildews, Avignon, France (Belanger R.R., Bushnell W.R., Carver T.L.W., Dik A.J., Kunoh H., Nocot P. and Schmitt A., eds), p. 26 Avignon, France: INRA‐Station de Pathologie Végétale. [Google Scholar]
- Schweizer, P. , Vallélian‐Bindschedler, L. and Mösinger, E. (1995) Heat‐induced resistance in barley to the powdery mildew fungus Erysiphe graminis f. sp hordei . Physiol. Mol. Plant Pathol. 47, 51–66. [Google Scholar]
- Schweizer, P. , Christoffel, A. and Dudler, R. (1999) Transient expression of members of the germin‐like gene family in epidermal cells of wheat confers disease resistance. Plant J. 20, 541–552. [Abstract] [Google Scholar]
- Seem, R.C. (1984) Disease incidence and severity relationships. Annu. Rev. Phytopathol. 22, 133–150. [Google Scholar]
- Smith, F.D. , Gadoury, D.M. , Harpending, P.R. and Sanford, J.C. (1992) Transformation of a powdery mildew, Uncinula necator, by microprojectile bombardment. Phytopathology, 82, 247. [Google Scholar]
- Spanu, P.D. , Abbott, J.C. , Amselem, J. , Burgis, T.A. , Soanes, D.M. , Stüber, K. , Ver Loren van Themaat, E. , Brown, J.K.M. , Butcher, S.A. , Gurr, S.J. , Lebrun, M.‐H. , Ridout, C.J. , Schulze‐Lefert, P. , Talbot, N.J. , Ahmadinejad, N. , Ametz, C. , Barton, G.R. , Benjdia, M. , Bidzinski, P. , Bindschedler, L.V. , Both, M. , Brewer, M.T. , Cadle‐Davidson, L. , Cadle‐Davidson, M.M. , Collemare, J. , Cramer, R. , Francisco, L.‐R. , Frenkel, O. , Godfrey, D. , Harriman, J. , Hoede, C. , King, B.C. , Klages, S. , Kleemann, J. , Knoll, D. , Koti, P.S. , Kreplak, J. , Lu, X. , Maekawa, T. , Mahanil, S. , Milgroom, M.G. , Montana, G. , Noir, S. , O'Connell, R.J. , Oberhaensli, S. , Parlange, F. , Pedersen, C. , Quesneville, H. , Reinhardt, R. , Rott, M. , Sacristán, S. , Schmidt, S.M. , Schön, M. , Skamnioti, P. , Sommer, H. , Stephens, A. , Takahara, H. , Thordal‐Christensen, H. , Vigouroux, M. , Weßling, R. , Wicker, T. and Panstruga, R. (2010) Genome expansion and gene loss in powdery mildew fungi reveal functional tradeoffs in extreme parasitism. Science, 330, 1543–1546. [Abstract] [Google Scholar]
- Spotts, R.A. , Covey, R.P. and Chen, P.M. (1981) Effects of low temperature on survival of apple buds infected with the powdery mildew fungus. HortScience, 16, 781–783. [Google Scholar]
- Stark‐Urnau, M. and Kast, W.K. (1999) Development of ontogenic resistance to powdery mildew in fruit of differentially susceptible grapevines (cvs. Trollinger and Lemberger). Mitt. Klosterneuburg. 49, 186–189. [Google Scholar]
- Staudt, G. (1997) Evaluation of resistance to grapevine powdery mildew (Uncinula necator Schw. Burr., anamorph Oidium tuckeri Berk.) in accessions of Vitis species. Vitis, 36, 151–154. [Google Scholar]
- Stein, U. , Heintz, C. and Blaich, R. (1985) The in vitro examination of grapevines regarding resistance to powdery and downy mildew. Z. Pflanzenk. Pflanzen. 92, 355–369. [Google Scholar]
- Stummer, B.E. and Scott, E.S. (2003) Detection of novel genotypes in progeny from a controlled cross between isolates of Uncinula necator belonging to distinct phenetic groups. Australas. Plant Pathol. 32, 213–218. [Google Scholar]
- Stummer, B.E. , Zanker, T. , Scott, E.S. and Whisson, D.L. (2000) Genetic diversity in populations of Uncinula necator: comparison of RFLP‐ and PCR‐based approaches. Mycol. Res. 104, 44–52. [Google Scholar]
- Thomashow, M.F. (1999) Plant cold acclimation: freezing tolerance genes and regulatory 699 mechanisms. Annu. Rev. Plant Physiol. Plant Mol. Biol. 50, 571–599. [Abstract] [Google Scholar]
- Wakefield, L. , Gadoury, D.M. , Seem, R.C. , Milgroom, M.G. , Sun, Q. and Cadle‐Davidson, L. (2011) Differential gene expression during conidiation in the grape powdery mildew fungus, Erysiphe necator . Phytopathology, 101 (in press). [Abstract] [Google Scholar]
- Wan, J. , Zhang, X.C. , Neece, D. , Ramonell, K.M. , Clough, S. , Kim, S.Y. , Stacey, M.G. and Stacey, G. (2008) A LysM receptor‐like kinase plays a critical role in chitin signaling and fungal resistance in Arabidopsis. Plant Cell, 20, 471–481. [Abstract] [Google Scholar]
- Wan, Y.Z. , Schwaninger, H. , He, P.C. and Wang, Y.J. (2007) Comparison of resistance to powdery mildew and downy mildew in Chinese wild grapes. Vitis, 46, 132–136. [Google Scholar]
- Wang, Y. and Hua, J. (2009) A moderate decrease in temperature induces COR15a expression through the CBF signaling cascade and enhances freezing tolerance. Plant J. 60, 340–349. [Abstract] [Google Scholar]
- Wei, Y.D. , Zhang, Z.G. , Andersen, C.H. , Schmelzer, E. , Gregersen, P.L. , Collinge, D.B. , Smedegaard‐Petersen, V. and Thordal‐Christensen, H. (1998) An epidermis/papilla specific oxalate oxidase‐like protein in the defense response of barley attacked by the powdery mildew fungus. Plant Mol. Biol. 36, 101–112. [Abstract] [Google Scholar]
- Welter, L.J. , Göktürk‐Baydar, N. , Akkurt, M. , Maul, E. , Eibach, R. , Töpfer, R. and Zyprian, E.M. (2007) Genetic mapping and localization of quantitative trait loci affecting fungal disease resistance and leaf morphology in grapevine (Vitis vinifera L). Mol. Breed. 20, 359–374. [Google Scholar]
- Weltzien, H.C. (1978) Geographical distribution of powdery mildews In: The Powdery Mildews (Spencer D.M., ed.), pp. 39–49. London: Academic Press. [Google Scholar]
- Wiese, J. , Kranz, T. and Schubert, S. (2004) Induction of pathogen resistance in barley by abiotic stress. Plant Biol. 6, 529–536. [Abstract] [Google Scholar]
- Wilcox, W.F. and Wolf, T.K. (2008) Disease management In: Wine Grape Production Guide for Eastern North America, NRAES‐145 (Wolf T.K., ed.), pp. 216–240. Ithaca, NY: Natural Resource, Agriculture, and Engineering Service. [Google Scholar]
- Willocquet, L. , Colombet, D. , Rougier, M. , Fargues, J. and Clerjeau, M. (1996) Effect of radiation, especially UV‐B, on spore germination and mycelial growth of the grape powdery mildew. Eur. J. Plant Pathol. 102, 441–449. [Google Scholar]
- Winterhagen, P. , Howard, S.F. , Qui, W. and Kovács, L.G. (2008) Transcriptional upregulation of grapevine MLO genes in response to powdery mildew infection. Am. J. Enol. Vitic. 59, 159–168. [Google Scholar]
- Ypema, H.L. and Gubler, W.D. (1997) Long term effect of temperature and triadimefon on proliferation of Uncinula necator: implications for fungicide resistance and disease risk assessment. Plant Dis. 81, 1187–1192. [Abstract] [Google Scholar]
- Ypema, H.L. and Gubler, W.D. (2000) The distribution of early season grapevine shoots infected by Uncinula necator from year to year: a case study in two California vineyards. Am. J. Enol. Vitic. 51, 1–6. [Google Scholar]
Articles from Molecular Plant Pathology are provided here courtesy of Wiley
Full text links
Read article at publisher's site: https://doi.org/10.1111/j.1364-3703.2011.00728.x
Read article for free, from open access legal sources, via Unpaywall:
https://europepmc.org/articles/pmc6638670?pdf=render
Citations & impact
Impact metrics
Article citations
The Transcriptional Landscape of Berry Skin in Red and White PIWI ("Pilzwiderstandsfähig") Grapevines Possessing QTLs for Partial Resistance to Downy and Powdery Mildews.
Plants (Basel), 13(18):2574, 13 Sep 2024
Cited by: 0 articles | PMID: 39339549 | PMCID: PMC11434962
Applicability of metabolomics to improve sustainable grapevine production.
Front Mol Biosci, 11:1395677, 06 Sep 2024
Cited by: 0 articles | PMID: 39310375 | PMCID: PMC11413592
Review Free full text in Europe PMC
A New Approach for CRISPR/Cas9 Editing and Selection of Pathogen-Resistant Plant Cells of Wine Grape cv. 'Merlot'.
Int J Mol Sci, 25(18):10011, 17 Sep 2024
Cited by: 0 articles | PMID: 39337500 | PMCID: PMC11432302
A Sustainable Strategy for Marker-Assisted Selection (MAS) Applied in Grapevine (Vitis spp.) Breeding for Resistance to Downy (Plasmopara Viticola) and Powdery (Erysiphe Necator) Mildews.
Plants (Basel), 13(14):2001, 22 Jul 2024
Cited by: 0 articles | PMID: 39065527 | PMCID: PMC11280485
What are the 100 most cited fungal genera?
Stud Mycol, 108:1-411, 15 Jul 2024
Cited by: 1 article | PMID: 39100921 | PMCID: PMC11293126
Go to all (76) article citations
Similar Articles
To arrive at the top five similar articles we use a word-weighted algorithm to compare words from the Title and Abstract of each citation.
Identification and utilization of a new Erysiphe necator isolate NAFU1 to quickly evaluate powdery mildew resistance in wild Chinese grapevine species using detached leaves.
Plant Physiol Biochem, 98:12-24, 10 Nov 2015
Cited by: 10 articles | PMID: 26590705
The Perfect Stage of Powdery Mildew of Grapevine Caused by Erysiphe necator Found in Peru.
Plant Dis, 96(5):768, 01 May 2012
Cited by: 0 articles | PMID: 30727546
Strategies for RUN1 Deployment Using RUN2 and REN2 to Manage Grapevine Powdery Mildew Informed by Studies of Race Specificity.
Phytopathology, 105(8):1104-1113, 10 Aug 2015
Cited by: 25 articles | PMID: 26039639
Powdery Mildew Resistance Genes in Vines: An Opportunity to Achieve a More Sustainable Viticulture.
Pathogens, 11(6):703, 18 Jun 2022
Cited by: 6 articles | PMID: 35745557 | PMCID: PMC9230758
Review Free full text in Europe PMC