Abstract
Free full text

SV40 and human mesothelioma
Abstract
Simian virus 40 (SV40) is a DNA tumor virus capable of infecting and transforming human mesothelial (HM) cells in vitro. Hamsters injected intracardially to expose most tissue types to SV40 preferentially develop mesotheliomas. In humans, asbestos is the main cause of mesothelioma, and asbestos and SV40 are co-carcinogens in transforming HM cells in tissue culture and in causing mesothelioma in hamsters. Laser microdissection experiments conducted in the laboratory of Adi Gazdar demonstrated that SV40 was present specifically in the malignant mesothelioma cells and not in nearby stromal cells. Further experiments demonstrated that SV40 remains episomal in HM cells and astrocytes because of the production of a long antisense RNA that represses viral capsid protein production. Thus, the potent SV40 oncoprotein, T-antigen (Tag), is expressed, but because the capsid proteins are not produced, the cells are not lysed and, instead, become transformed. Together this evidence suggests that SV40 may contribute to the development of mesotheliomas in humans. However, epidemiological evidence to support this hypothesis is lacking. This chapter also summarizes the introduction of SV40, a monkey virus, into the human population as an unrecognized contaminant of early poliovaccines. In addition to mesotheliomas, SV40 now is linked with brain cancers, osteosarcomas, and lymphomas in humans. Explanations are provided for the apparent geographic variations in SV40 prevalence and for controversies about the role of SV40 in human cancer.
Introduction
A review that used current technology to verify the diagnosis of all lung/pleural cancer deaths recorded over 100 years at Massachusetts General Hospital in Boston identified the first case of mesothelioma in 1947, with a steep increase of this diagnosis in the 1960s and thereafter (1). The authors suggested that mesothelioma was possibly a “new” cancer caused by the massive exposure to asbestos during and after World War II. This theory has been proven incorrect, at least in part, as mesothelioma occurs “spontaneously” in carriers of germline BAP1 heterozygous mutations regardless of asbestos exposure (1-4). Asbestos likely may increase the risk of mesothelioma in these individuals (5). However, the increase of mesothelioma in the 1960s coincided with human exposure to simian virus 40 (SV40) to which the human population was exposed massively between 1955–1963 when poliovaccines were contaminated with viable, infectious SV40 (6-8).
Here the authors summarize these issues. Dr. Gazdar, our co-author, had just started to write this article when he unexpectedly became ill and died; the coauthors completed the work he had started.
Human exposure to SV40
SV40 is a DNA tumor virus that is endemic in rhesus monkeys. Because poliovaccines were initially prepared by growing the poliovirus in primary rhesus monkey kidney cells in tissue culture, many lots of poliovaccines in the US were contaminated with SV40 from 1954 until 1963 (8,9). Moreover the poliovaccines that were prepared in the former Soviet Union and in countries under its influence, were contaminated with infectious SV40 at least until 1978, with the exception of former Jugoslavia that produced their own SV40-free poliovaccines (10). In Italy, one of the poliovirus stocks used to prepare poliovaccines remained contaminated until 1991 when the contaminant was discovered and the contaminated poliovaccine stock replaced (10). In China, until the recent past, poliovaccines were still prepared using rhesus monkey kidney cells, and thus were likely contaminated, until the very recent past (10). Both the attenuated (Sabin) oral, live poliovaccines (OPV) and the inactivated (Salk) poliovaccines (IPV) contained live SV40. There was no inactivation step in production of OPVs, and the process used to inactivate polioviruses in IPVs was insufficient to inactivate all contaminating SV40. However, not all batches of poliovaccines were contaminated and the amount of contamination varied greatly (9,11-13). Notably, these production processes meant that the level of contaminating SV40 was much higher in OPVs than IPVs and that all lots of OPV would have been contaminated as the vaccine seed stocks contained SV40. IPV lots would have been contaminated when the primary cultures of rhesus monkey kidney cells used for vaccine production were naturally infected with SV40. In addition, the route of administration was presumably more natural for OPV (oral) than for IPV (intramuscular). These factors make it likely that contaminated OPV exposures initiated the majority of human infections (13). Finally, researchers always assumed that SV40 infection of monkey cells would also produce vacuolization which allowed detection of the infection. However, recent work by researchers at the Food and Drug Administration revealed that SV40 can infect monkey cells without producing vacuoles. The implication of these studies is that many more vaccines may have been contaminated than current estimates (14).
SV40 causes cancer
DNA tumor viruses are usually not oncogenic in their natural host (for example, SV40 does not appear to cause cancer in rhesus monkeys) but they often become oncogenic when they cross species (15). Tests in hamsters revealed that SV40 produced sarcomas when injected subcutaneously, ependymomas and other types of brain tumors when injected intracranially, leukemias, lymphomas and bone tumors when injected intravenously, and mesotheliomas, osteosarcomas and lymphomas when injected intracardially. These are the same tumor types in which DNA sequences, mRNAs, and proteins corresponding to SV40 have been identified by multiple research teams [reviewed in (7,11,16)] (13,17). The occurrence of mesotheliomas in 60% of hamsters injected intracardially (18) was startling as these tumors had previously been linked only to asbestos exposure. The prevalence of mesotheliomas observed upon intracardiac injection suggested that the virus reached the pleura and pericardium directly upon the injection tract. Moreover, the pericardial inflammation caused by the needle puncture may have favored malignant growth. Furthermore, intrapleural injection of SV40 caused mesotheliomas in 100% of injected hamsters (19).
SV40 is one of the most potent human carcinogens used in research laboratories
SV40 infection per se causes malignant transformation of human cells (11,12). Dr. Hilary Koprowski provided probably the most compelling evidence for SV40 carcinogenesis in humans when his team took punch biopsies from terminally-ill patients, “volunteers”, established their fibroblasts in tissue culture, and infected the cells with SV40. The cells were re-injected back into the donors and formed tumor nodules that eventually regressed, either for lack of a blood supply or because of an immune response (20). These types of experiments are no longer allowed and presently we must rely on indirect evidence to identify human carcinogens, such as tridimensional foci formation, growth in soft agar, and tumor growth in mice (21). In contrast, Koprowski’s experiments provided unequivocal direct evidence that SV40 was a human carcinogen.
The potent tumor-inducing capacity of SV40 is linked largely to its major oncoprotein, the SV40 large tumor antigen (Tag) that binds and inactivates several cellular tumor suppressors, including pRb and p53, thus simultaneously impairing two cellular pathways that regulate G1/S transition and G2/M checkpoints of the cell cycle (22,23); [reviewed in (7,24)]. Intriguingly, SV40 Tag requires TP53 to transform human cells. On one hand, the Tag-p53 complex inactivates the tumor suppressor activity of TP53, while, on the other hand, this complex binds and activates the IGF receptor and induces cell growth. This mechanism is required for SV40 transformation as it provides a critical survival signal to circumvent apoptosis during the early phases of SV40 infection (25) and supports the early phases of tumor growth (26). Accordingly, down-regulation of TP53 reduces the transforming activity of SV40 in human mesothelial (HM) cells (25). SV40 Tag also binds and modulates p300 activity (27). Moreover, at least in mesothelial cells and astrocytes, SV40 infection induces telomerase activity, a process that requires both the large Tag and the small t-antigen (tag) of SV40 (28). This does not happen following transfection where SV40 is often under the control of a heterologous promoter. Among several other activities, SV40 Tag in human cells induces Notch 1 (29) and Met (30) activity, promoting cell growth. Of note, Met activity causes the secretion of the hepatocyte growth factor (HGF), which has a paracrine growth-stimulating effect on nearby cells, including cells not infected with SV40 (30). As a consequence of these activities, within a few days following SV40 infection, non-permissive human cells develop marked aneuploidy, which is ultimately responsible for the maintenance of the malignant phenotype (15,31-33).
Note that the widespread belief that integration of the SV40 DNA into the host genome and expression of the SV40 Tag are required to maintain the transformed phenotype is based largely on transfection experiments in which Tag expression is under the control of a heterologous promoter. It does not apply to natural infections in which Tag is under the control of its own promoter and whose activity is enhanced by the production of the small t antigen (tag), a viral protein that binds and inactivates PP2A, thus modulating SV40 replication (34). Indeed, there are multiple lines of evidence indicating that once SV40 infection has caused extensive aneuploidy the transformed cells no longer require the presence or expression of SV40 to maintain the transformed phenotype or for tumor growth (33,35-37). There is supporting evidence that once cells are transformed, there may be an immune selection in vivo against cells expressing SV40 tumor antigens that favors the growth of subclones in which either viral proteins are not expressed or the SV40 DNA has been lost altogether (38).
SV40 detection in human cancers
Following a number of sporadic reports of SV40 in human cancers [reviewed in (7)] (17), DJ Bergsagel in the laboratory of Robert Garcea reported that 90% of human ependymomas and choroid plexus tumors contained SV40 DNA sequences and that some of the tumor cells expressed the SV40 Tag (39). A few years later, John Lednicky in Janet Butel’s laboratory isolated infectious SV40 from one of those cancer specimens confirming that, at least in that specific biopsy, the “SV40-like sequences” detected by the polymerase chain reaction (PCR) and immunohistochemistry (IHC) corresponded to infectious SV40 (40).
The finding that SV40 could cause mesothelioma in hamsters (18,19) and the coincidental exponential increase in the incidence of mesothelioma in the years that followed the administration of SV40-contaminated vaccines led to tests of the hypothesis that SV40 might have contributed, together with asbestos, to the increased incidence of mesothelioma in recent decades. The results revealed that most mesotheliomas and some osteosarcomas contained SV40 DNA sequences (41,42) [reviewed in (17,22)]. These findings, together with those involving brain tumors (39), suggested that SV40 might play a causative role in certain types of human cancers and might have contributed to the massive increase in the incidence of mesothelioma in recent decades (43-45).
Mesotheliomas are rare tumors whose incidence increased exponentially in the Western world after 1960 (data in other parts of the world are not available), an increase that has been linked to the large use of asbestos during and after World War II (46-48). Asbestos causes chronic inflammation and the release of mutagenic oxygen radicals (49-51), as well as the activation of various cellular pathways that promote cell growth (52-55). Kroczynska et al. demonstrated that SV40 and asbestos are co-carcinogens in causing mesothelioma in hamsters and malignant transformation of HM cells in tissue culture (56). This study strongly suggested that SV40 infection and asbestos exposure together could have contributed to the development of human mesothelioma. A molecular epidemiological study conducted by Cristaudo et al., supported these findings (57).
Is the detection of SV40 in human cancer reliable and is it linked to the administration of SV40-contaminated poliovaccines?
Numerous studies, including blinded multi-laboratory studies designed to address the specificity of these findings (58) verified the presence of SV40 DNA sequences in brain tumors, mesotheliomas, lymphomas, and osteosarcomas, the same tumor types that were induced by SV40 when injected into hamsters [reviewed in (6,7,15,44,59,60)] (8,17). Some studies, however, did not find SV40 DNA in these same tumor types (61-63). The authors of these negative papers argued that the positive results were caused by laboratory contamination with DNA sequences from SV40-containing plasmids widely used in many laboratories. In-depth discussions of these issues can be found in references (7,8,11,12,43,62,64-66).
It is possible that some studies reported accidental contamination, especially those studies in which controls were minimal, such as when detection or absence of SV40 DNA was measured only by ethidium bromide staining without performing hybridization with specific probes and/or confirming the results using DNA sequencing. It is also clear that some studies that reported negative results used technical approaches that were flawed and/or inadequate to detect SV40 [see: (8,67,68)]. In some cases, the authors themselves recognized the flaws and, by correcting the technical approach, were able to detect SV40 DNA (69) and SV40 Tag (70) in some mesothelioma biopsies.
Molecular analysis of SV40 in human cancers
Sequence analysis of the regulatory region of SV40 DNA in human brain tumors showed that the enhancer region contained single 72-basepair elements (simple or archetypal structure), in contrast to laboratory strains of SV40 which contain rearranged, complex regulatory regions (40,71). Importantly, a study of commercial oral poliovaccines from 1955 detected the presence of SV40 and found that the contaminating SV40 contained simple regulatory regions with single 72-basepair elements (9). A study of a meningioma brain tumor from a laboratory scientist produced strong evidence of a known exposure to SV40 linked to subsequent cancer development 10–15 years later (72). Viral strain-specific DNA sequences in the meningioma were identical to those of the laboratory exposure source.
Epidemiology
It appears that the frequency of detection of SV40 in human cancers is influenced by geographic variations corresponding to the very different risks of exposure to SV40-contaminated poliovaccines in various countries (10,13,73,74). For example, De Rienzo et al. did not detect SV40 in 9/9 Turkish mesothelioma biopsies and did detect SV40 in 4/11 US mesothelioma biopsies, each sample tested in triplicate, i.e., 3 separate biopsies, producing consistent results (75). Similarly, Emri et al. (74) detected SV40 in 2/2 Italian mesothelioma biopsies and in 0/29 Turkish mesotheliomas. In contrast to the US and Italy, Turkey did not use SV40-contaminated poliovaccines, as vaccination in that country started only in the mid-1970s when most vaccines were SV40-free (10).
Epidemiologists argued in favor of and against the role of SV40 in causing mesothelioma and other human cancers (76). Viruses are rarely complete carcinogens, and cancer is usually a rare consequence of viral infection, with co-factors playing very important roles. For example, less than 1 in 1,000 women infected with human papilloma virus (HPV) develop carcinoma in situ and about 1 in 100 women with carcinoma in situ may develop invasive cervical carcinoma. Accordingly, geographical differences and related cofactors may influence the biological significance of SV40 infection, as observed with other viruses (59). For example, about 90% of nasopharyngeal carcinomas in China and of Burkitt’s lymphomas in Africa are causally linked to Epstein-Barr virus (EBV) infection, but the same tumor types in the US and Europe are rarely linked to EBV infection.
To further complicate epidemiology, humans could presumably be infected with SV40 by transmission via aerosol or gastrointestinal exposure by individuals who had received contaminated poliovaccines (13,77,78). Accordingly, SV40 DNA has been detected in individuals born after 1963 (11,17). This evidence further complicated the interpretation of the epidemiological studies that tried to determine whether SV40-contaminated poliovaccines were linked to the increased incidence of mesothelioma and of other cancers by comparing pre- and post-1963 cohorts [reviewed in: (13,59)]. Given these limitations, the Institute of Medicine (IOM), now renamed National Academy of Medicine, reviewed the issue of whether exposure to SV40-contaminated poliovaccines had contributed to an increase of mesothelioma and of other cancers, and concluded that all epidemiological studies were “flawed” because it was impossible to reliably identify those individuals who had received SV40-contaminated poliovaccines from those who had not (79). Therefore, a combination of epidemiology and molecular studies is a better approach than using only epidemiology to address the possible role of SV40 in human cancer (80).
Because of variability among the design of many published studies, the Butel laboratory performed a meta-analysis of reports of SV40 association with human cancers [summarized in (81)]. Well-controlled studies were included in the analysis that met stringent criteria: only patients with primary malignancies were tested, analyses were carried out on primary cancer specimens only (not cell lines), a control group of subjects was analyzed in parallel, and the same laboratory techniques were used on both case and control samples. For mesotheliomas, analysis of 996 subjects (528 cancer patients and 468 controls) from 15 studies yielded an odds ratio (OR) of SV40 association of 15.1. Meta-analysis of brain cancer, bone cancer, and non-Hodgkin lymphoma reports gave ORs of 3.8, 24.5, and 5.4, respectively.
Model of SV40 infections and associated cancers in humans
A model has been described recently that predicts patterns of SV40 infections and associated virus-positive cancers in humans (13). Key features of the model are that human infections by SV40 reflect primarily past exposure to contaminated oral poliovaccines (not IPVs), that viral transmission occurs among humans by a fecal/urine-oral route under poor sanitation and living conditions, and that viral infections persist today in selected populations in only certain geographic areas. Predictions of the model are that SV40 prevalence rates will vary widely and will be generally very low in countries and populations that were not exposed to SV40-contaminated OPV and/or enjoy good sanitation and living conditions. Of course, persons from high-prevalence areas might carry SV40 persistent infections into low-prevalence countries and transmit infections in regions there. This model is compatible with historical usage of contaminated poliovaccines and can explain inconsistencies in the published literature. For example, some studies analyzed samples from countries that were not sites of common usage of SV40-contaminated OPV and/or considered IPVs as the exposure source. The model also demonstrates the importance of selection of appropriate study populations and emphasizes the differences in expected patterns of findings between SV40 and those of a cancer virus distributed worldwide, such as HPV.
Laser microdissection and methylation studies
As the issue of SV40 presence in human tumors was becoming controversial, Adi Gazdar was quite skeptical about the positive findings. Gazdar had exceptional credibility in the thoracic cancer world as he had developed most of what was known about the molecular biology of lung cancer, and he also had strong credibility in virology having played a key role in the discovery of HTLV-1 (82) and later of HTLV-3 (subsequently renamed HIV). However, sadly, although Gazdar provided the precious cells that were used to culture and isolate HIV (as HIV would not grow in other available cells), his critical contribution was not acknowledged in the resulting paper. Gazdar proposed at multiple mesothelioma meetings that SV40 was a passenger virus that did not play any role in causing mesothelioma or other human malignancies, and he set up an experiment to disprove the relevance of SV40 detection in human cancer biopsies (Figure 1). The results surprised Gazdar who eventually became a strong supporter of research aimed at establishing the role of SV40 infection in human cancer (Figure 2). Gazdar and collaborators found SV40 DNA in microdissected mesothelioma cells from 57 of 118 mesothelioma biopsies and in only 1 of 75 stromal cells microdissected from nearby tissue and in 0 of 50 microdissected lung cancer specimens (Figure 1). Gazdar, who had blinded his postdoctoral fellow about whether specimens were mesotheliomas or lung adenocarcinomas, noted that it would have been impossible to produce a similar laboratory artifact (83). In subsequent experiments, Gazdar and collaborators found that mesothelioma biopsies containing SV40 DNA sequences had significantly increased methylation of different genes, especially of RASSF1A (P=0.007), and that the tumor suppressor RASSF1A became more highly methylated, and thus inactivated, in tissue culture following SV40 infection of primary HM cells (84). Gazdar and collaborators obtained similar results for lymphoid malignancies and they concluded that SV40 was a human pathogen that had a causative role in certain human malignancies (85). In parallel experiments, Ramael et al. detected SV40 DNA by in situ PCR specifically in tumor cells (86). These experiments supported results of IHC analyses in human mesothelioma biopsies that demonstrated SV40 Tag expression only in tumor cells and not in nearby stromal cells (41). Moreover, Cacciotti et al., found that SV40 induced high levels of vascular endothelial growth factor (VEGF) in mesothelioma and thus supported tumor growth (87).
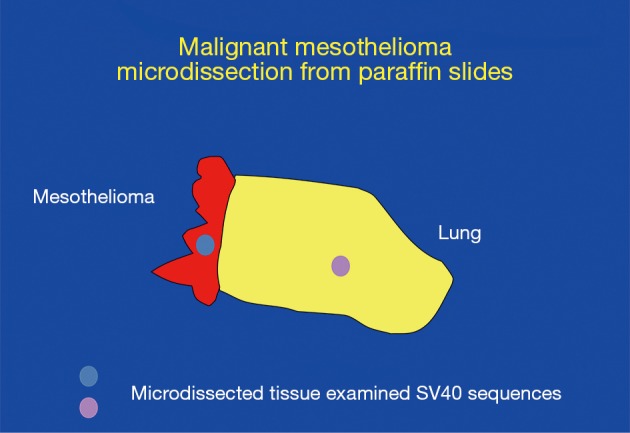
Laser microdissection experiments conducted in Dr. Adi Gazdar laboratory on pleura/lung biopsies. Mesothelioma tissue and lung tissue were present on the same slide, and were microdissected and analyzed in parallel. SV40 DNA was specifically detected in mesothelioma microdissected tissue (red) and not in nearby lung tissue (yellow) (83).
Serology
Serological studies have addressed the issue of whether SV40 causes human infections and whether such infections are linked to cancer development by testing for anti-SV40 antibodies in human sera [reviewed in (66)]. Thirty SV40 seroprevalence studies reported from 1961 to 2019 were summarized recently by Butel and colleagues (88). Overall, these studies support the existence of SV40 human infections, as well as a link between the administration of contaminated poliovaccines and detection of SV40 antibodies in human sera. Reported studies involved countries in Europe (Czech Republic, England, Finland, Germany, Hungary, Italy, Poland, Sweden), Asia (Kazakhstan), and Latin America (Colombia, Nicaragua), as well as the US [references cited in (88)]. The serological assays included neutralization tests which are highly specific, but labor-intensive and time-consuming to perform. More often, versions of an enzyme-linked immunosorbent assay (ELISA) were used. It was argued by some, however, that serological assays were too non-specific as they could detect antibodies against human polyomaviruses BKV and JCV and thus would over-estimate the prevalence of SV40 antibodies in the human population (89). Concerns of possible cross-reactivity increased when three new human polyomaviruses were discovered [KIV, WUV, and Merkel cell PyV (MCV)], all of which could have been a source of cross-reactivity in previous testing. Robert Garcea and collaborators then developed what was regarded as a more reliable serological assay that distinguished among different polyomaviruses, and they concluded that “only” 2% of tested subjects contained anti-SV40 antibodies, a finding that on one hand suggested that previous studies had over-estimated the prevalence of anti-SV40 antibodies in human sera and on the other hand confirmed human infections with SV40 (90). It should be noted that 2% of the population is a huge number, meaning that millions of people may have been/may be infected with SV40, a monkey virus that was not naturally present in humans, a virus that is a potent carcinogen in human cells in tissue culture, and a virus that causes cancer in hamsters. The presence of SV40 antibodies is found also among individuals both younger and older than possible vaccine recipients of contaminated OPV, suggesting that SV40 has crossed the monkey-human barrier and has established a permanent foothold in the human population (88,90).
Recently, a new ELISA approach was developed that is based on two synthetic peptides that mimic specific epitopes on SV40 viral capsid proteins (i.e., VP mimotopes) (91). That assay detected SV40 antibodies in Italian patients with mesotheliomas (92), as well as in healthy donors and patients with brain tumors, osteosarcomas, and non-Hodgkin lymphomas (88). The seroprevalence of SV40 antibodies was higher in the cancer patient groups than in the healthy individuals. More recently, another new ELISA was described that is able to detect antibodies against the SV40 oncoprotein, large Tag. It uses two synthetic peptides that mimic epitopes on Tag (93). SV40 Tag antibodies were reportedly detected in osteosarcoma patients, as well as in healthy subjects [see (88)]. This assay for Tag antibodies, when positive, provides evidence that SV40 had undergone replication in those infected individuals, as Tag is produced in cells during the viral replication cycle but is not a component of mature virus particles.
SV40 transformation of human cells is cell type specific
Non-permissive cells are those cells that do not support efficient viral replication. For example, hamster cells are non-permissive to SV40 replication because the SV40 Tag does not interact well with the host DNA polymerase-alpha primase (94). Either the virus becomes integrated into the host cell DNA in such a way that both Tag and tag are expressed and transforms those cells—at a rate of about 1 per 107 infected cells—or the viral sequences are lost during cell division and there is no pathological consequence due to the infection (95). In contrast, green monkey kidney cells, such as CV-1 cells, are permissive to SV40 replication. In those cells, SV40 replicates rapidly and within a few weeks all infected cells are lysed and the virus released into the cell culture medium. Because the infected cells are lysed, there is no malignant transformation. Human cells, instead, are called semi-permissive for SV40, a term intended to indicate that SV40 can replicate although less efficiently than in green monkey kidney cells. At any given time, only a variable fraction of cells supports viral replication while the remaining cells appear unaffected [reviewed in (7)]. Rarely, SV40 may become integrated in the human host cell genome and, if SV40 Tag and tag are produced, those cells can then give rise to transformed foci. Some of such foci may grow for a few passages until they enter so-called “crisis”, a period during which morphologically-transformed primary cells stop growing and become growth arrested. Occasionally, some cells escape crisis and become immortal because telomerase becomes activated and cell lines can be established, at a proposed rate of about 1 per 107 or 108 infected cells. Nearly all experiments in human cells were conducted using human fibroblasts, cells that are easy to obtain [reviewed in (41)]. The hypothesis that fibroblasts are not transformed because they are lysed was supported by transfection experiments in which SV40 genomes mutated in their origin of replication became integrated into the host cell genome and easily produced transformed cell lines. These experiments mimicked the results in hamster cells in which the virus cannot replicate and thus cannot lyse the cells (7). Therefore, almost all studies in human cells were conducted by transfection and, since transfection requires integration into the host cell genome, it was erroneously assumed that, similar to what was observed in hamster cells, unless SV40 became integrated into the human DNA it could not transform human cells.
HM cells and astrocytes are unusually susceptible to SV40-mediated transformation
As SV40 was found mainly in mesotheliomas and brain tumors and not in human fibrosarcoma, which are the tumors derived from fibroblasts, Carbone and colleagues decided to infect primary HM cells and human astrocytes with SV40 in tissue culture. They used fibroblasts as controls in parallel experiments. They found that the susceptibility of human cells to SV40 was cell-type dependent. About 1-5 per 1,000 infected HM cells (15,32) and about 1-3 per 10 human astrocytes (96) infected with SV40 gave rise to morphologically-transformed foci that developed into colonies capable of growing in soft agar (a widely used in vitro measurement of malignant transformation). Moreover, nearly 90% of the transformed foci could be established as cell lines. Those cells were immortal, likely related to the activation of telomerase within 72 hours after infection (28) as they never went through a crisis period (15,32,96). These results are exceptional as no other cell type has been reported to become transformed at such high frequency following SV40 infection. In contrast, none of the over 108 infected human fibroblasts, in multiple experiments, ever gave rise to colonies capable of growing in soft agar and/or of growing into cell lines. Not only did fibroblasts not give rise to transformed cell lines in parallel experiments, but the literature indicates that less than 5% of SV40-induced transformed foci of human fibroblasts develop into continuous cell lines [reviewed in (60)].
Unexpectedly, SV40 DNA was found to be present in an episomal (non-integrated) state in transformed HM cells and astrocytes (96,97). Common beliefs were that episomal SV40 should replicate and lyse the cells, yet HM and astrocyte SV40 transformed cell lines continued to contain episomal SV40. It took several years to determine the mechanism (97). Briefly, although the viral DNA was episomal only the Tag and tag proteins were expressed. Capsid proteins were not expressed so viral particles were not formed. However, in a small fraction of cells (estimated at less than 1%), SV40 replicated, produced virus, and lysed those cells, so the tissue culture fluid from those cell lines contained infectious SV40 even after 50 passages in culture. The mechanism that prevented capsid protein formation and thus viral assembly in over 99% of the cells was related to the production of a long antisense SV40 RNA that led to the formation of double-stranded mRNA comprising the mRNA for the capsid proteins that was degraded. SV40 is a very small virus which has a circular DNA genome of 5.2 kB. In permissive cells, two separate mRNAs are produced from the Ori region of replication, one going clockwise that is spliced to produce the large and the small t antigens, and an mRNA going in the opposite, counter-clockwise direction—which is spliced to produce the different SV40 capsid proteins. We found that in mesothelial cells and in astrocytes, but not in fibroblasts, the sense mRNA does not detach from the SV40 DNA template and continues to run along the circular genome, resulting in the formation of an antisense RNA that pairs with the mRNA coding for the capsid proteins, forming double-stranded mRNA that is degraded (Figure 3). This mechanism sometimes fails in less than 1% of the cells for unknown reasons, resulting in viral particle formation and lysis of that subpopulation of cells. Accordingly, SV40 mRNA and capsid proteins are detectable only during the first few days following SV40 infection of HM cells and astrocytes and then become undetectable as cells become transformed (97).
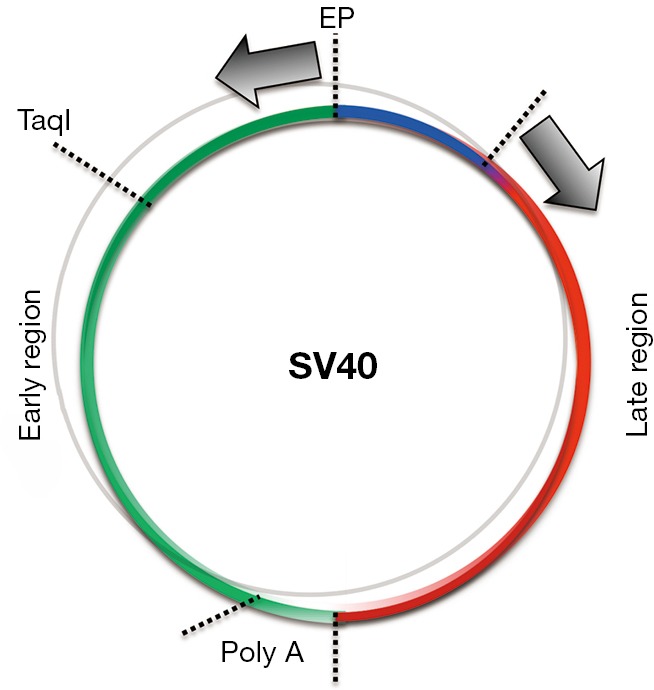
Two separate mRNAs (arrows) are produced from the Ori region of replication, one going counter-clockwise in the so called early region that is spliced to produce the large (Tag) and the small t antigen (tag), and an mRNA going in the opposite, clockwise direction, known as late region, which is spliced to produce the different SV40 VP capsid proteins. In mesothelial cells and in astrocytes, but not in fibroblasts, the late region mRNA—which codes for Tag and tag, does not detach from the SV40 DNA template when it reaches the Poly A site, and instead continues to run along the circular genome, resulting in the formation of an antisense RNA that pairs with the mRNA coding for the capsid proteins, forming double-stranded mRNA that is degraded (97).
Conclusions
SV40 is a monkey virus that has now crossed species and infects members of the human population at frequencies that vary in different parts of the world. Based on what we have learned about the biology of SV40, this virus is a human carcinogen and certain cell types, such as mesothelial cells and astrocytes and probably additional cell types not yet identified, are much more susceptible than others to SV40 malignant transformation. Experimental data indicate that some cells can inhibit SV40 replication through cell-specific mechanisms and survive infection. The unfortunate consequence is that surviving cells can accumulate SV40-induced genetic damage and are prone to malignant transformation. No cancer epidemic followed the administration of SV40-contaminated poliovaccines. This might have been because of lack of public awareness, low frequencies of certain SV40 neoplasias, and/or immune system recognition and elimination of SV40-transformed cells. Further, there is experimental evidence that SV40 may be lost over time by subpopulations of cells that become independent of SV40 for tumor growth. Thus, SV40 may function, all or in part, as an exogenous agent that increases the basal level of spontaneous mutations and lowers the threshold for tumor development. This mechanism is known as indirect carcinogenesis or as “hit and run” carcinogenesis (21). Such instances could favor SV40 co-carcinogenesis upon asbestos exposure for the genesis of some mesotheliomas. Increasing awareness of the variable geographic distributions and prevalence rates of current SV40 human infections can inform the design of future targeted studies.
Acknowledgments
Funding: M Carbone reports grants from the National Institutes of Health, National Cancer Institute, the US Department of Defense, and the UH Foundation through donations to support research on “Pathogenesis of Malignant Mesothelioma” from Honeywell International Inc, Riviera United‐4‐a Cure, and the Maurice and Joanna Sullivan Family Foundation. M Carbone has a patent issued for BAP1. M Carbone has a patent issued for “Using Anti‐HMGB1 Monoclonal Antibody or other HMGB1 Antibodies as a Novel Mesothelioma Therapeutic Strategy,” and a patent issued for “HMGB1 As a Biomarker for Asbestos Exposure and Mesothelioma Early Detection”. M Carbone is a board‐certified pathologist who provides consultation for mesothelioma expertise and diagnosis.
Notes
Ethical Statement: The authors are accountable for all aspects of the work in ensuring that questions related to the accuracy or integrity of any part of the work are appropriately investigated and resolved.
Footnotes
Conflicts of Interest: MC serves as the unpaid Guest Editor of the focused issue “Mesothelioma: What We know and What We Do Not Know in 2020)”. TLCR. Vol 9, Supplement 1 (February 2020). AG serves as the former unpaid editorial board member of TLCR. The other author has no conflicts of interest to declare.
References
Articles from Translational Lung Cancer Research are provided here courtesy of AME Publications
Full text links
Read article at publisher's site: https://doi.org/10.21037/tlcr.2020.02.03
Read article for free, from open access legal sources, via Unpaywall:
https://tlcr.amegroups.com/article/viewFile/35999/24147
Citations & impact
Impact metrics
Article citations
Diagnosis of Pleural Mesothelioma: Is Everything Solved at the Present Time?
Curr Oncol, 31(9):4968-4983, 27 Aug 2024
Cited by: 0 articles | PMID: 39329996 | PMCID: PMC11430569
Review Free full text in Europe PMC
Malignant Epithelioid Mesothelioma of the Tunica Vaginalis Testis Presenting as Hydrocele in a Kidney Transplant Recipient.
Case Rep Urol, 2024:9227764, 10 Jan 2024
Cited by: 0 articles | PMID: 38239857 | PMCID: PMC10794071
Phosphorylation of Human Polyomavirus Large and Small T Antigens: An Ignored Research Field.
Viruses, 15(11):2235, 09 Nov 2023
Cited by: 0 articles | PMID: 38005912 | PMCID: PMC10674619
Review Free full text in Europe PMC
Preventive and therapeutic opportunities: targeting BAP1 and/or HMGB1 pathways to diminish the burden of mesothelioma.
J Transl Med, 21(1):749, 25 Oct 2023
Cited by: 2 articles | PMID: 37880686 | PMCID: PMC10599047
Review Free full text in Europe PMC
The Role of DNA Viruses in Human Cancer.
Cancer Inform, 22:11769351231154186, 13 Jun 2023
Cited by: 6 articles | PMID: 37363356 | PMCID: PMC10286548
Review Free full text in Europe PMC
Go to all (16) article citations
Data
Data behind the article
This data has been text mined from the article, or deposited into data resources.
BioStudies: supplemental material and supporting data
Similar Articles
To arrive at the top five similar articles we use a word-weighted algorithm to compare words from the Title and Abstract of each citation.
Simian virus 40 and human tumors: It is time to study mechanisms.
J Cell Biochem, 76(2):189-193, 01 Dec 1999
Cited by: 33 articles | PMID: 10618636
Review
The pathogenesis of mesothelioma.
Semin Oncol, 29(1):2-17, 01 Feb 2002
Cited by: 209 articles | PMID: 11836664
Review
New molecular and epidemiological issues in mesothelioma: role of SV40.
J Cell Physiol, 180(2):167-172, 01 Aug 1999
Cited by: 46 articles | PMID: 10395286
Review