Abstract
Free full text

The potential of JAK/STAT pathway inhibition by ruxolitinib in the treatment of COVID-19
Graphical abstract
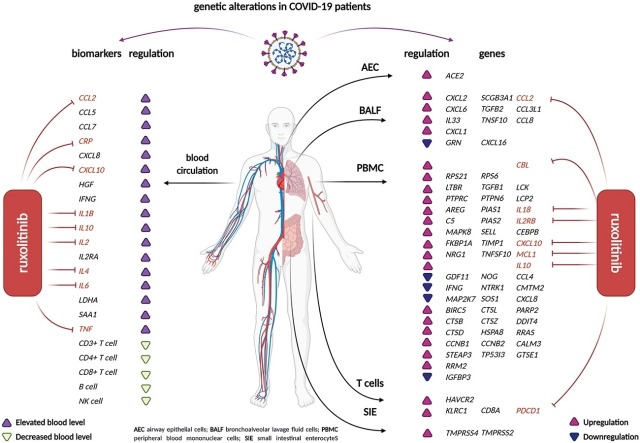
Abstract
Ruxolitinib is the first approved JAK1 and JAK2 inhibitor, and is known to interfere with the JAK / STAT signaling pathway, one of the critical cellular signaling pathways involved in the inflammatory response. This review presents an overview of SARS-CoV-2 and the COVID-19 pandemic, and then focuses on the potential efficacy of ruxolitinib in this infection. The potential targets of ruxolitinib were determined by using genetic alterations that have been reported in COVID-19 patients. The potential effectiveness of ruxolitinib is suggested by evaluating the interactions of these potential targets with ruxolitinib or JAK/STAT pathway.
1. Introduction
In the final days of 2019, a pneumonia of unknown etiology with fever, breathing difficulties, and invasive lung lesions was reported in Wuhan China by the WHO. On January 7, 2020, Chinese scientists identified the etiologic agent as a new type of coronavirus, with the genome sequence available five days later [1]. WHO changed the status of the disease to a pandemic on March 11, 2020, because of the rapid increase in cases and worldwide spread [1]. As of June 17, 2020, the total number of Coronavirus disease 19 (COVID-19) cases worldwide was approximately 8 million and the total number of deaths was approximately 450,000, a death total ratio of 5.48 percent [2]. At present, there is no protective vaccine or approved treatments available.
2. Overview of the SARS-CoV-2
The virus, which is the cause of the COVID-19 was named as Severe Acute Respiratory Syndrome-related Coronavirus (SARS-CoV-2) by Coronaviridae Study Group of the International Committee on Taxonomy of Viruses (Fig. 1 a). SARS-CoV-2 is a betacoronavirus, in the Coronaviridae family along with two other species that infect humans, SARS-CoV, and MERS-CoV [3].
The genomic structure of the virus is a positive-sense, single-stranded RNA which is approximately 30 kb (29,903 nucleotides). The viral RNA is packaged by nucleocapsid proteins and this structure is surrounded by a bilayer lipid corona structure which includes membrane, envelope, and spike proteins (Fig. 1b). The transcriptome contains the open reading frame (ORF) 1ab, S, ORF3a, E, M, ORF6, ORF7a, ORF7b, ORF8, N, and ORF10 genes, respectively. ORF1ab is cleavaged to nonstructural proteins (nsp). Among them, nsp12 has RNA-dependent RNA polymerase activity which performs replication and transcription of the viral genome using it as a template. The functions of other ORFs, which encode accessory proteins, are not yet clearly described [4]. The S gene encodes the Spike glycoprotein that binds to the human angiotensin-converting enzyme 2 (ACE2) receptor to infect the host cells [5]. While Envelope and Membrane proteins encoded by E and M genes, associate with the bilayer lipid envelope structure on the outer surface of the virus, N codes the Nucleocapsid protein that directly interacts with the viral genome [6].
The S protein of virion binds to the ACE2 receptor of the cell that will be infected by the virus (Fig. 1c). In the process following the binding, it is suggested that proteases especially TMPRSS2, on the surface of the host cell can strengthen binding and trigger receptor-mediated endocytosis by causing conformational changes in the S glycoprotein [5]. The early endosome carrying the virion matures towards the late endosome during vesicular traffic process and the gradual increase in the endosomal lumen acidity causes the release of the viral genome to the cytoplasm [7]. Firstly, ORF1ab is translated using the viral RNA, and its cleavage forms the RNA-dependent RNA polymerase which is involved in both replication and transcription of structural proteins. Using these transcripts, cytoplasmic ribosomes translate the nucleocapsid protein, and ER-bound ribosomes translate the spike, envelope, and membrane proteins into the ER lumen. Nucleocapsid packed viral RNA is encapsulated within the vesicle which carries spike, envelope, and membrane proteins on its membrane in the Endoplasmic Reticulum Golgi Intermediate Compartment (ERGIC). Finally, a complete virion is released to the extracellular region by exocytosis [8].
3. Overview of the COVID-19
3.1. Symptoms
SARS-CoV-2 is transmitted from human to human with droplets and from the mucosal surfaces of the nose, mouth, and eyes [9]. It is thought that the majority of the SARS-CoV-2 infected individuals are asymptomatic depending on their general health conditions and age. Fever, dry cough, fatigue or weakness, and dyspnea are the most common (>50%); myalgia, chest oppression or pain, diarrhea, loss of or poor appetite, shortness of breath, expectoration, anorexia are common (<50% and >10%); headache, chest pain, sore throat, vomiting, loss of smell and taste are the less common (<10%) symptoms of the diagnosed cases [[10], [11], [12], [13], [14], [15], [16], [17], [18], [19], [20]].
3.2. Diagnosis
In addition to general symptoms and laboratory findings, chest computed tomography (CT), rapid antibody-based methods, and molecular tests including Real-Time Reverse Transcriptase–PCR are utilized for diagnosis of COVID-19 [10]. SARS-CoV-2 was isolated from different clinical samples including upper and lower respiratory tract passages, blood, and stool. However the infectious nature of the live virus is not exactly defined, with the exception of the respiratory tract samples [21]. Based on Real-Time Reverse Transcriptase–PCR test results, the infectivity rate decreases in virus from bronchoalveolar lavage, sputum, throat, nasal and pharyngeal swabs, respectively [22]. Similarly, the infectivity rate appears to be higher in the early and progressive stages of the disease, compared to the recovery stage. The high viral load and infectious properties of the respiratory samples are thus suggestive evidence of respiratory transmission [23].
3.3. Risk factors
Advanced age (≥ 65 years) is defined as the most common risk factor. Comorbidities - hypertension, cardiovascular diseases, diabetes, chronic obstructive pulmonary diseases, malignancies, chronic kidney or hepatic diseases, asthma, or infectious diseases such as tuberculosis, and hepatitis - have been identified as other risk groups [10,11,13,17,19,24]. Although smoking is the main risk factor for various diseases especially lung cancer, it is not classified as a risk factor of COVID-19 as yet [25]. Various genetic factors may also affect the prognosis of COVID-19; for example, the phenotypes of HLA-B *46:01 and HLA-B*15:03 affect the severity of infection by causing low and high binding affinity of SARS-CoV-2 to cells, respectively [26].
3.4. Complications
Complications triggered by COVID-19 are the main factors affecting disease severity and death. The most common complication of the COVID-19 is acute respiratory distress syndrome (ARDS). It is characterized by the appearance of ground-glass opacities in the lungs and results in serious respiratory failure and secondary complications, including multiple organ failure related to insufficient oxygenation levels [20,24,27]. Cytokine release syndrome or cytokine storm (See “4- Cytokine storm and COVID-19″ section), hemophagocytic lymphohistiocytosis, and septic shock are frequently seen as complications from hyperactivation of the immune system [[28], [29], [30], [31], [32]]. Development of the autoimmune diseases including neurodegenerative disorders like Guillain Barre Syndrome, hematologic disorders like autoimmune hemolytic anemia is reported during COVID-19 treatment [33,34]. Acute cardiac, kidney, and liver injury are reported as common complications [20,24,27]. Although meningitis and encephalitis are also reported as less common complications of COVID-19, other bacterial or viral co-infections are quite frequent and they may result in deaths [18,35].
3.5. Current therapies
No treatment or drug has yet been approved, although different therapeutic approaches are currently being tested against the symptoms of COVID-19. Current treatment applications are separated into two subgroups: the first group of the treatment strategies includes antiviral drugs and immune-based therapies to overcome viral infection; the second group comprises antithrombotics, ventilation or oxygen therapies, used for secondary complications.
Remdesivir (GS-5734, Gilead Sciences) is an RNA-dependent RNA polymerase inhibitor, used against RNA viruses such as Ebolaviruses, although it has not yet been approved for any indication [36,37]. Chloroquine (or hydroxychloroquine) is an approved antimalarial drug that increases the pH of lysosomes and inhibits autophagy by suppressing lysosome-autophagosome fusion [38]. This autophagy inhibitor is a part of the current COVID-19 treatment protocol because it inhibits the endocytic pathway which allows virus entry into the cell and activation after binding to the ACE2 receptor [39]. Nevertheless, current indicated that chloroquine has no beneficial value in seriously ill patinets. HIV protease inhibitors have been approved for use in treatment of HIV that function to inhibit proteolysis of viral proteins necessary to complete the HIV life cycle [40]. It is predicted that protease inhibition performed with agents such as Lopinavir/Ritonavir (Kaletra, Abbott Laboratories) may also be effective against SARS-CoV-2 [41].
The use of plasma (known as convalescent plasma therapy) or immune globulins from recovered individuals is being tested in clinical trials to help activate the immune system against SARS-CoV-2 in patients. Also, interferons (interferon alfa and interferon beta) are being tested for the same purpose [42]. Numerous clinical studies aimed to induce adaptive immunity are currently underway by different research teams [43,44].
It has been reported that the infection-related increase of coagulation parameters especially the D-dimer (normal range <0.5 μg/ml) is directly proportional to the severity of the disease. Coagulation abnormalities cause disseminated intravascular coagulation and triggers venous thromboembolism and pulmonary embolism which are among the main causes of COVID-19 related death. Antithrombotic and anticoagulant drugs including heparin, warfarin, direct-acting oral anticoagulants are used to protect against the development of coagulation and thromboembolism complications during the treatment process [45].
3.6. Genetic alterations in COVID-19
Various genetic alterations have been reported that could potentially be used as therapeutic targets during COVID-19 infection (Fig. 2 ). These variations especially include inflammation and immune response regulation [10,11,[13], [14], [15], [16], [17],19,24,27,29,31,32,[46], [47], [48], [49], [50], [51], [52], [53]]. Furthermore, increased expression of ACE2 and TMPRSS2 may contribute to complications in the heart, lungs, and different organs of the nervous system [47,54].
4. Cytokine storm and COVID-19
As a consequence of SARS-CoV-2 infection, a cytokine storm syndrome is triggered by dysregulated immune responses; the cytokine storm is characterized by a high inflammatory response, including elevated levels of cytokines and immune cells that infiltrate and destroy organs and cause lung lesions, respiratory dysfunction, multiple organ damage, and death [28]. Cytokines are a group of immunoregulatory cell-cell communication molecules including different subtypes named chemokine (chemotaxis cytokine), interleukin (leukocyte related cytokine), lymphokine (lymphocytes-related cytokine), monokine (monocytes-related cytokine) and interferons. Although originally thought to be secreted by specific immune cells, it is now recognized that nonimmune cells, fibroblasts or endothelial cellsrespond to inflammation or injury, as well as monocytes, macrophages, B- and T-lymphocytes. These cytokines are both cause and effect of the immune response and include both pro- and anti-inflammatory molecules [55].
4.1. The JAK/ STAT pathway
Cytokines regulate different cellular and immune processes and their activation is controlled by the JAK/STAT signalling pathway [56]. The Janus kinases (JAKs) and the signal transducers and activators of transcriptions (STATs) form one of the main regulatory cell signaling pathways (Fig. 3 ). The JAK non-receptor tyrosine kinase family includes Jak1, Jak2, Jak3, and Tyrosine kinase 2 (Tyk2) proteins. Their unique structure consists of seven JAK homology domains (JH1-7); at the carboxy-terminal, are two kinase domains (JH1 and JH2). This family is named for the mythological Janus god because of the two headed tandem kinase domains. The JH1 domain is a catalytic component and a second kinase domain is a pseudo-kinase JH2 that has an autoregulatory suppressor function. JH3 is a Src homology (SH2) domain and the activated SH2 generates a binding site for STAT transcription factors. At the amino terminal end is a receptor-interacting FERM domain comprising JH4-7 (Band 4.1, ezrin, radixin, moesin) [57]. The JAK non-receptor tyrosine kinases receive numerous different extracellular signals (growth factor, cytokine, and hormone) from host receptors and transfer these responses to the nucleus via the intracellular STATs. When extracellular signals are received by the specific JAK-associated receptor, a conformational change occurs that causes autophosphorylation on the tyrosines of the JAKs, and subsequent dimerization of the STATs. Dimerized STATs are directed into the nucleus and trigger transcription of the immune regulatory, apoptotic, cell cycle, and differentiation related genes. The STAT protein family includes STAT1, STAT2, STAT3, STAT4, STAT5a, STAT5b, and STAT6, and all contain an N-terminal, coiled-coil domain involved in protein-protein interactions, DNA-binding domain for sequence-specific DNA binding andnuclear localization, a linker region, an SH2 domain involved in dimerization and protein association, and a transactivation domain (TAD) that carries conserved tyrosine residues that are phosphorylation sites for host kinases [58]. Depending on the physiological signal, the JAK/STAT pathway regulates critical cellular homeostasis processes including immune response, proliferation, differentiation, migration, and apoptosis[59].
The IL6/JAK/STAT3 signaling pathway represents a specific branch of the JAK/STAT pathway that includes IL6, an essential pleiotropic cytokine produced by B cells T cells, dendritic cells, and macrophages to generate an immune response or inflammation. Binding of IL6 to its specific receptor (IL6 receptor-subunit alpha IL6R) triggers a heterohexameric complex with IL6 receptor subunit-β (gp130, IL6ST) and activates the IL6/JAK/STAT3 pathway, that includes activation of inflammation-related downstream targets [58].
IL6 is is one of the pivotal inflammatory cytokines upregulated in influenza, vaccinia, hepatitis B and C, Crimean-Congo hemorrhagic fever, and human immunodeficiency virus infections in humans [60]. In the context of COVID-19 cytokine storm, IL6 is likewise one of the most highly expressed cytokines; elevated serum levels of IL6 are considered one of the main indicators of poor prognosis in SARS-CoV-2 infection. The local inflammatory response, generated in part through IL6, also spreads throughout the body and contributes to cytokine release and acute respiratory distress syndromes, as well as organ damage. Different therapeutic strategies to overcome hyper-inflammation include the use of JAK/STAT pathway inhibitors and particularly anti-IL6 inhibitors [28].
5. Overview of the ruxolitinib and effect mechanisms
The first approved JAK inhibitor was ruxolitinib, followed by other JAK inhibitors including baricitinib, upadacitinib, tofacitinib, peficitinib, and fedratinib [[61], [62], [63]] that are under clinical investigation for the treatment of the cytokine storm. Among these, baricitinib (LY3009104, INCB028050, Olumiant, Eli Lilly) was the second JAK1 and JAK2 inhibitor, approved in 2018 for treatment of rheumatoid arthritis. In addition to its anti-inflammatory effects, baricitinib also inhibits virus endocytosis, indicating a dual specificity inhibitor [64]. And although clinical studies are underway, there is a caveat - baricitinib may increase patient vulnerability to co-infection, virus reactivation, lymphocytopenia, and neutropenia, thus indicating that it may not be an ideal treatment option [65]. A similar situation is encountered with interleukin inhibitors. Tocilizumab, an approved IL6 receptor antagonist, has been shown to reduce cytokine release syndrome symptoms in severe patients COVID-19 [66], but may also contribute to an increased risk of death by increasing immunosuppression in severely ill patients [30].
5.1. Ruxolitinib
Ruxolitinib (INCB018424; Jakavi; Incyte Corporation) is a potent JAK1 and JAK2 inhibitor that blocks JAK kinase activity and prevents STAT activation and nuclear translocation. Ruxolitinib was approved by the FDA in November 2011, December 2014, and May 2019 for the treatments of Myelofibrosis, Polycythemia Vera, and Acute Graft-Versus-Host Disease, respectively to reduce the high level of cytokine release associated with these disorders. Therefore, ruxolitinib has begun to take its placein the treatment of autoimmune diseases such as rheumatoid arthritis, psoriasis, and lupus erythematosus, as well as other allergic and inflammatory diseases [67]. Ruxolitinib also inhibits IL6/JAK/STAT3 pathway, thus reducing circulating IL6 levels [68,69].
5.2. Ruxolitinib and viral infections
The potential of ruxolitinib in the treatment of different inflammatory conditions is also being investigated.
5.2.1. Immunosuppression
Ruxolitinib is used in both acute and chronic graft versus host disease from allogeneic hematopoietic stem cell transplantation. [70]. Hemophagocytic lymphohistiocytosis, a rare secondary disease triggered by viral infection or autoimmune disease, in which a hyper-activated immune response may causesevere complications; ruxolitinib has been shown to suppress cytokine levels and the JAK/STAT pathway in Epstein-Barr Virus (EBV)-associated hemophagocytic lymphohistiocytosis [71].
5.2.2. Antiviral efficacy
The anti-viral properties of ruxolitinib may have activity against Human Immunodeficiency Virus (HIV) and EBV infections. Ruxolitinib has been shown to inhibit HIV-1 replication in lymphocytes and macrophages and to suppress HIV-1 reactivation [72, as well as to inhibit production of inflammatory cytokines such as IL1β, IL2, IL5, IL6, IL7, IL13, IL15, and IFNG [[73], [74], [75]]. Similarly, the anti-viral potential of ruxolitinib is also indicated in EBV infection where ruxolitinib inhibits EBV-infected PBMC proliferation and reduces elevated inflammatory cytokines by inhibition of STAT3 [76,77].
5.2.3. Opportunistic infections
Because the JAK/STAT pathway is a primary signal pathway, suppression of this pathway can also result in the emergence of opportunistic infections. The development of Polyomavirus (JC-Virus and BK-Virus) related fatal encephalopathy and meningitis has been reported during ruxolitinib treatment [78,79]. Because the JAK/STAT pathway inhibits Zika Virus (ZIKV) and Hepatitis C Virus (HCV), members of the Flaviviridae family, it is suggested that ruxolitinib may actually increase viral replication [80,81]. Hepatitis B Virus (HBV) reactivation has also been reported due to ruxolitinib treatment [82]. Infections of different Herpesvirus family members which include Varicella-Zoster Virus (VZV), EBV, and Cytomegalovirus (CMV), have also been reported. Development of gastric ulcer and meningoencephalitis due to EBV and VZV infections has been reported in patients with myelofibrosis and polycythemia vera treated with ruxolitinib, respectively [83,84]. Ruxolitinib has also been associated with reactivation of CMV, VZV, and EBV during myelofibrosis, graft versus host disease, and myelodysplastic syndrome treatments [[85], [86], [87], [88]]. Reactivation causes secondary diseases that include lymphoproliferative disorders [89].
6. Potential interactions between ruxolitinib and COVID-19
Since ruxolitinib is well-toleratedand used in the elderly population at present, it is a powerful candidate to overcome the hyperimmune syndrome that arises in COVID-19 patients [68]. A number of clinical trials assessing the efficacy of ruxolitinib in COVID-19 related symptoms are ongoing (Table 1 ).
Table 1
Clinical trials including ruxolitinib.
Trial ID | Name | Sponsor | Dose | Design | Patients & Medical condition | Time frame | Status |
---|---|---|---|---|---|---|---|
EUCTR2020-001662-11-DE | RUXCOVID | Novartis Pharma AG | Once daily 5 mg |
|
|
| R |
EUCTR2020-001459-42-DK | Ruxolitinib Treatment in Patients with Severe COVID-19 Infection. A Danish Safety and Efficacy Study. | Zealand University Hospital-Denmark | Once daily 10 to 40 mg |
|
|
| O |
ChiCTR2000029580 | Severe novel coronavirus pneumonia (COVID-19) patients treated with ruxolitinib in combination with mesenchymal stem cells: a prospective, single blind, randomized controlled clinical trial | Department of Hematology, Tongji Hospital, Tongji Medical College, Huazhong University of Science and Technology- China |
|
|
| R | |
2020-001777-71 | Ruxolitinib therapy to avoid Ventilation and improve outcome for deteriorating COVID-19 patients – RAVEN | Guy’s and St Thomas' NHS Foundation Trust- UK Novartis Pharmaceuticals UK Ltd- free of charge drug supply |
|
|
| O | |
NCT04348071 | Safety and Efficacy of Ruxolitinib for COVID-19 | University of Colorado, Denver- USA | Twice daily 10 mg |
|
|
| NYR |
NCT04354714 | Ruxolitinib to Combat COVID-19 | Washington University School of Medicine- USA Collobrator Incyte Corporation | Twice daily 5 mg and 10 mg |
|
|
| W |
NCT04377620 | Assessment of Efficacy and Safety of Ruxolitinib in Participants With COVID-19-Associated ARDS Who Require Mechanical Ventilation (RUXCOVID-DEVENT) | Incyte Corporation | Twice daily 5 mg and 15 mg |
|
|
| R |
NCT04366232 | Efficacy of Intravenous Anakinra and Ruxolitinib During COVID-19 Inflammation (JAKINCOV) | Centre Hospitalier Intercommunal de Toulon La Seyne sur Mer- France | Twice daily 5 mg |
|
|
| NYR |
NCT04338958 | Ruxolitinib in Covid-19 Patients With Defined HyperinFlammation (RuxCoFlam) | University of Jena- Germany | Twice daily 10 mg or 20 mg |
|
|
| R |
NCT04334044 | Treatment of SARS caused by COVID-19 with Ruxolitinib | Grupo Cooperativo de Hemopatías Malignas -Mexico | Twice daily 5 mg |
|
|
| R |
NCT04331665 | Study of the Efficacy and Safety of Ruxolitinib to Treat COVID-19 Pneumonia | University Health Network, Toronto- USA | Twice daily 10 mg (for 14 days) 5 mg, (for 2 days) Once daily 5 mg (for 1 day) |
|
|
| NYR |
NCT04374149 | Therapeutic Plasma Exchange Alone or in Combination With Ruxolitinib in COVID-19 Associated CRS | Prisma Health-Upstate | Plasma excahange and Once daily 5 mg (for14 days) |
|
|
| NYR |
NCT04359290 | Ruxolitinib for Treatment of Covid-19 Induced Lung Injury ARDS (RuXoCoil) | Philipps University Marburg Medical Center- Germany | Twice daily 10 mg to 15 mg |
|
|
| NYR |
NCT04355793 | Expanded Access Program of Ruxolitinib for the Emergency Treatment of Cytokine Storm From COVID-19 Infection | Incyte Corporation | Twice daily 5 mg starting dose |
|
| A | |
NCT04361903 | COVID-19: Ruxolitinib for the Treatment of cytokinE Storm resPiratory dIstREss Syndrome. RESPIRE Study | Azienda USL Toscana Nord Ovest- Italy | Twice daily at least 20 mg (for the first 48 hours) |
|
|
| NYR |
NCT04414098 | Ruxolitinib in the Treatment of Covid-19 | Marcelo Iastrebner- Argentina Collaborator: Novartis | Twice daily 5 mg |
|
|
| NYR |
NCT04337359 | Ruxolitinib Managed Access Program (MAP) for Patients Diagnosed With Severe/Very Severe COVID-19 Illness | Novartis Pharmaceuticals | Once daily 5 mg | A | |||
NCT04424056 | An Open Randomized Therapeutic Trial Using ANAKINRA, TOCILIZUMAB Alone or in Association With RUXOLITINIB in Severe Stage 2b and 3 of COVID19-associated Disease (INFLAMMACOV) | Assistance Publique Hopitaux De Marseille- France | Different combinations with anakinra, tocilizumab |
|
|
| NYR |
NCT04348695 | Study of Ruxolitinib Plus Simvastatin in the Prevention and Treatment of Respiratory Failure of COVID-19. (Ruxo-Sim-20) | Hospital Universitario Madrid Sanchinarro-Madrid- Spain | Twice daily 5 mg (7 days) Twice daily 10 mg (following 7 days) combination with simvastatin |
|
|
| R |
*Collaborator: Apices Soluciones S.L. | |||||||
NCT04403243 | COLchicine Versus Ruxolitinib and Secukinumab In Open Prospective Randomized Trial (COLORIT) | Lomonosov Moscow State University Medical Research and Educational Center- Russia | Twice daily 5 mg |
|
|
| R |
A available; NYR not yet recruiting; O ongoing; R recruiting; W withdrawn.
To determine the potential molecular efficacy of ruxolitinibon genetic alterations,molecular pathways that include altered genes were determined by the KEGG Pathway Database and the STRING Database Version 11 (Fig. 4 ). Ruxolitinib reduced the expression of inflammatory biomarkers at both the gene and protein levels in different cells (Table 2 ).
Table 2
Genetic alterations are directly targeted by ruxolitinib in COVID-19.
Genes | Regulation in COVID-19 | Reference | Effects of ruxolitinib | Reference |
---|---|---|---|---|
CBL | Downregulated after treatment in severe cases (PBMC) | [14] | Inhibits CBL mutant Pluripotent Stem Cells | [90] |
CXCL10 | Upregulated in COVID-19 patients PBMC (compared to normal PBMC) and elevated blood level in severe patients | [32,49,52] | Downregulates the expression level in macrophages | [91] |
IL10 | Upregulated in COVID-19 patients PBMC (compared to normal PBMC)and elevated blood level in severe patients | [10,11,49,50,53,14,15,16,18,19,24,27,31] | Decreases secretion in macrophages | [92] |
IL18 | Upregulated COVID-19 patients PBMC (compared to normal PBMC) | [49] | Downregulates IL18 expression levels in lymphoblasts | [69] |
IL2 | Elevated blood level in severe patients | [31] | Reduces IL2 levels in T cells | [73] |
IL2RB | Downregulated after treatment in severe cases (PBMC) | [14] | Inhibits JAK/STAT pathway activating IL2RB mutant Ba/F3 cells | [93] |
MCL1 | Downregulated after treatment in severe cases (PBMC) | [14] | Downregulates expression level in lymphoblasts | [94] |
PDCD1 | Increased levels on T cells | [50] | Decreases PDCD1 levels in T cells | [95] |
CCL2 | Upregulated COVID-19 patients BALF (compared to normal BALF) Elevated blood level in severe patients | [32,49] | Reduces secreted protein levels in bone marrow mesenchymal stromal cells | [96] |
CRP | Elevated blood level in severe patients | [10,12,13,18,29] | Reduces CRP level | [97] |
IL1B | Elevated blood level in severe patients | [11,15,27] | Downregulates expression in T cells | [98] |
IL4 | Elevated blood level in severe patients | [27] | Reduces IL4 levels in patients | [99] |
IL6 | Elevated blood level in severe patients | [10,11,29,31,32,50,53,66,100,[13], [14], [15], [16], [17],19,24,27] | Downregulates IL6 expression level in lymphoblast | [69] |
TNF | Elevated blood level in severe patients | [11,15,32] | Reduces the expression in mast cells | [101] |
PBMC peripheral blood mononuclear cells; BALF bronchoalveolar lavage fluid cells.
7. Conclusion
It is clear that ruxolitinib has an important potential in overcoming complications caused by immune hyperactivation related to the JAK/STAT signaling pathway. Since the JAK/STAT pathway is associated with the induction of multiple molecular immune pathways, inhibition of this pathway may result in the inhibition of several cellular responses Considered together, ruxolitinib has potential in the treatment of COVID-19 infection; however, adverse effects such as opportunistic infections as a result of immune suppression must also be considered.
Declaration of Competing Interest
The authors declare that they have no known competing financial interests or personal relationships that could have appeared to influence the work reported in this paper.
Acknowledgment
Graphical abstract and Figure 1-3 were created by using BioRender.
Biographies
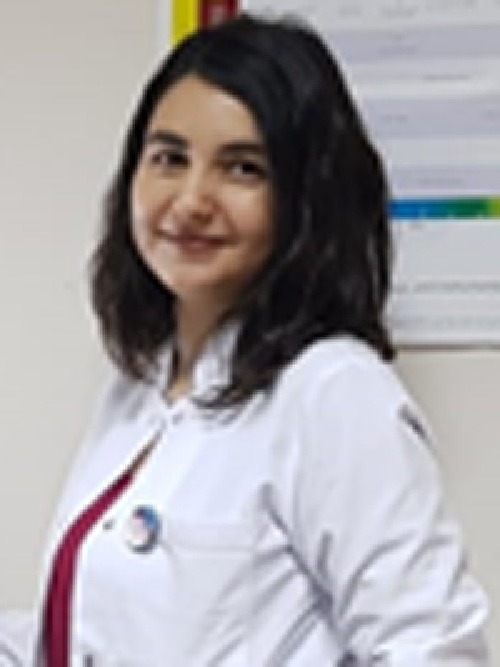
Bakiye Goker Bagca is a PhD student in Medical Biology Department at Ege University and she is a research assistant in this department. Her master thesis includes JAK/STAT pathway regulation and the effects of ruxolitinib in leukemic and healthy cells. She is taking roles in research projects including IL6 signaling regulation.
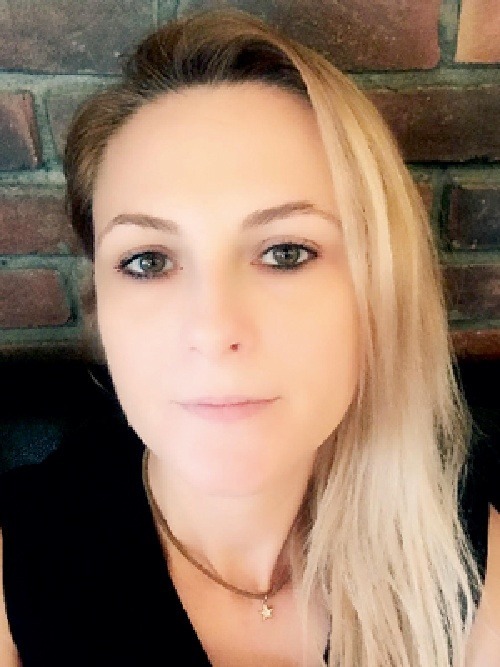
Cigir Biray Avci, PhD, is an Associate Professor in Medical Biology Department Ege University Medical School. She has numerous research and review papers including IL6 signaling, JAK/STAT signaling, and cross-talks about the other cellular signaling pathways. She is supervising various research projects about genetic and epigenetic regulation of molecular signaling.
References
Full text links
Read article at publisher's site: https://doi.org/10.1016/j.cytogfr.2020.06.013
Read article for free, from open access legal sources, via Unpaywall:
https://europepmc.org/articles/pmc7305753?pdf=render
Citations & impact
Impact metrics
Citations of article over time
Alternative metrics
Smart citations by scite.ai
Explore citation contexts and check if this article has been
supported or disputed.
https://scite.ai/reports/10.1016/j.cytogfr.2020.06.013
Article citations
Mechanisms of Tripterygium wilfordii Hook F on treating rheumatoid arthritis explored by network pharmacology analysis and molecular docking.
Open Med (Wars), 19(1):20240967, 30 May 2024
Cited by: 0 articles | PMID: 38841174
Adverse drug reactions associated with COVID-19 management.
Naunyn Schmiedebergs Arch Pharmacol, 397(10):7353-7376, 14 May 2024
Cited by: 0 articles | PMID: 38743117
Review
The oncogenic mechanisms of the Janus kinase-signal transducer and activator of transcription pathway in digestive tract tumors.
Cell Commun Signal, 22(1):68, 25 Jan 2024
Cited by: 1 article | PMID: 38273295 | PMCID: PMC10809652
Review Free full text in Europe PMC
Exogenous Klotho Extends Survival in COVID-19 Model Mice.
Pathogens, 12(12):1404, 29 Nov 2023
Cited by: 0 articles | PMID: 38133288 | PMCID: PMC10746004
Ganoderic acid A attenuated hepatic impairment by down-regulating the intracellular JAK2-STAT3 signaling pathway in induced mushroom poisoning.
Am J Transl Res, 16(1):295-303, 15 Jan 2024
Cited by: 1 article | PMID: 38322557 | PMCID: PMC10839386
Go to all (61) article citations
Data
Data behind the article
This data has been text mined from the article, or deposited into data resources.
BioStudies: supplemental material and supporting data
Clinical Trials (Showing 12 of 12)
- (1 citation) ClinicalTrials.gov - NCT04348071
- (1 citation) ClinicalTrials.gov - NCT04366232
- (1 citation) ClinicalTrials.gov - NCT04359290
- (1 citation) ClinicalTrials.gov - NCT04331665
- (1 citation) ClinicalTrials.gov - NCT04355793
- (1 citation) ClinicalTrials.gov - NCT04374149
- (1 citation) ClinicalTrials.gov - NCT04348695
- (1 citation) ClinicalTrials.gov - NCT04354714
- (1 citation) ClinicalTrials.gov - NCT04334044
- (1 citation) ClinicalTrials.gov - NCT04377620
- (1 citation) ClinicalTrials.gov - NCT04361903
- (1 citation) ClinicalTrials.gov - NCT04338958
Show less
European Clinical Trials
- (1 citation) EU Clinical Trials Register - 2020-001777-71
Similar Articles
To arrive at the top five similar articles we use a word-weighted algorithm to compare words from the Title and Abstract of each citation.
Inhibition of cytokine signaling by ruxolitinib and implications for COVID-19 treatment.
Clin Immunol, 218:108517, 23 Jun 2020
Cited by: 56 articles | PMID: 32585295 | PMCID: PMC7308779
Review Free full text in Europe PMC
Baricitinib, a drug with potential effect to prevent SARS-COV-2 from entering target cells and control cytokine storm induced by COVID-19.
Int Immunopharmacol, 86:106749, 01 Jul 2020
Cited by: 92 articles | PMID: 32645632 | PMCID: PMC7328558
Review Free full text in Europe PMC
The Janus kinase 1/2 inhibitor ruxolitinib in COVID-19 with severe systemic hyperinflammation.
Leukemia, 34(7):1805-1815, 09 Jun 2020
Cited by: 148 articles | PMID: 32518419 | PMCID: PMC7282206
Potential Treatments for COVID-19 Related Cytokine Storm - Beyond Corticosteroids.
Front Immunol, 11:1445, 16 Jun 2020
Cited by: 22 articles | PMID: 32612616 | PMCID: PMC7308586