Abstract
Free full text

Low temperature modulates natural peel degreening in lemon fruit independently of endogenous ethylene
Abstract
Peel degreening is an important aspect of fruit ripening in many citrus fruit, and previous studies have shown that it can be advanced by ethylene treatment or by low-temperature storage. However, the important regulators and pathways involved in natural peel degreening remain largely unknown. To determine how natural peel degreening is regulated in lemon fruit (Citrus limon), we studied transcriptome and physiochemical changes in the flavedo in response to ethylene treatment and low temperatures. Treatment with ethylene induced rapid peel degreening, which was strongly inhibited by the ethylene antagonist, 1-methylcyclopropene (1-MCP). Compared with 25 ºC, moderately low storage temperatures of 5–20 °C also triggered peel degreening. Surprisingly, repeated 1-MCP treatments failed to inhibit the peel degreening induced by low temperature. Transcriptome analysis revealed that low temperature and ethylene independently regulated genes associated with chlorophyll degradation, carotenoid metabolism, photosystem proteins, phytohormone biosynthesis and signalling, and transcription factors. Peel degreening of fruit on trees occurred in association with drops in ambient temperature, and it coincided with the differential expression of low temperature-regulated genes. In contrast, genes that were uniquely regulated by ethylene showed no significant expression changes during on-tree peel degreening. Based on these findings, we hypothesize that low temperature plays a prominent role in regulating natural peel degreening independently of ethylene in citrus fruit.
Introduction
Fruit ripening is a multifaceted process comprising various physiochemical and structural changes such as softening, degradation of starch to sugars, development of colour, and production of aroma volatiles (Cherian et al., 2014; Seymour and Granell, 2014). In citrus fruit, colour development, commonly known as peel degreening, is a critical part of ripening and is characterized by a colour change in the peel from green to yellow/red/orange (Iglesias et al., 2007). Peel degreening is an important aspect for marketability of citrus fruit (Porat, 2008), and hence there is wide interest in determining the fundamental regulatory mechanisms involved.
There are two main pathways that have been linked to citrus peel degreening. The first is chlorophyll degradation, which initially involves dephytilation of chlorophyll molecules by chlorophyllase (CLH) and pheophytinase (PPH), followed by removal of the central Mg atom by Mg-dechelatase to form pheophorbide. Pheophorbide is then converted to red chlorophyll catabolites (RCC) by pheophorbide a oxidase (PaO), and RCC is reduced to colourless compounds by RCC reductase (RCCR) (Hörtensteiner, 2006; Shimoda et al., 2016; Yin et al., 2016). The second pathway is carotenoid biosynthesis, which starts with the condensation of two geranylgeranyl pyrophosphate (GGPP) molecules by phytoene synthase (PSY) to form phytoene. Phytoene desaturase (PDS) and ζ-carotene desaturase (ZDS) successively convert phytoene to lycopene, which is then converted to α-carotene or β-carotene by lycopene ε-cyclase (LCYe) or lycopene β-cyclase (LCYb), respectively. α-carotene is later converted to lutein via sequential hydroxylation by ε-ring hydroxylase and β-ring hydroxylase (CHYb), whilst β-carotene is converted to zeaxanthin via β-cryptoxanthin by CHYb (Cunningham et al., 1996; Ohmiya et al., 2019). Genes encoding various enzymes for the main steps of chlorophyll degradation and carotenoid metabolism have been isolated and functionally characterized (Rodrigo et al., 2013).
The phytohormone ethylene has been routinely used for commercial degreening in citrus fruit (Purvis and Barmore, 1981; Porat, 2008; Mayuoni et al., 2011). Exogenous ethylene application has been shown to transcriptionally modulate both chlorophyll degradation and carotenoid metabolism (Rodrigo and Zacarias, 2007; Shemer et al., 2008; Yin et al., 2016). Transcription factors (TFs) that may be involved in ethylene-induced peel degreening have also been identified and characterized (Yin et al., 2016). Nevertheless, it remains unclear whether ethylene plays a role during natural degreening since citrus fruit are non-climacteric and the amounts of ethylene produced are minute (Eaks, 1970; Sawamura, 1981; Katz et al., 2004).
Temperature has a large impact on a wide range of plant growth and developmental processes, including fruit ripening and maturation. Low temperature is thought to slow most cell metabolic activities, and hence it is the major postharvest technology used to delay fruit ripening and senescence (McGlasson et al., 1979; Hardenburg et al., 1986). However, promotion of fruit ripening by low temperature has been described in various fruit species, including kiwifruit (Kim et al., 1999; Mworia et al., 2012; Asiche et al., 2017; Mitalo et al., 2018b), European pears (El-Sharkawy et al., 2003; Nham et al., 2017), and apples (Tacken et al., 2010). Recently, transcriptome studies have suggested that low temperature-specific genes might have regulatory roles during fruit ripening in kiwifruit (Asiche et al., 2018; Mitalo et al., 2018a, 2019a, 2019b) and European pears (Mitalo et al., 2019c).
Low temperature has also been linked to the promotion of fruit ripening (especially peel degreening) in several citrus fruit species. Typically, the seasonal temperature drops as most citrus fruit mature on the tree. Previous reports have correspondingly demonstrated that cold periods below 13 °C are required to stimulate on-tree fruit colour development (Manera et al., 2012, 2013; Rodrigo et al., 2013; Conesa et al., 2019). During storage, low/intermediate temperatures (6–15 °C) have also been shown to promote citrus peel degreening (Matsumoto et al., 2009; Van Wyk et al., 2009; Zhu et al., 2011; Carmona et al., 2012b; Tao et al., 2012). Natural peel degreening in citrus fruit has to date been attributed to ethylene signalling, on the assumption that the basal System I ethylene levels produced by mature fruit are physiologically active (Goldschmidt et al., 1993; Carmona et al., 2012a). However, it remains unclear whether the colour changes in the peel during on-tree maturation and low-temperature storage are caused by basal ethylene, low temperature, and/or a synergistic effect of ethylene and low temperature, or because of another unknown mechanism.
In this study, we performed combined physiological and transcriptome analysis to examine the involvement of ethylene in low temperature-triggered degreening in citrus. Using lemon fruit, we found that peel degreening occurred faster at moderately low storage temperatures (5–20 °C; hereafter referred to simply as low temperature) compared to 25 °C. RNA-seq unveiled a distinct set of genes that were differentially regulated by low temperature independently of ethylene. Furthermore, repeated treatments of fruit with 1-methylcyclopropene (1-MCP), a well-known ethylene antagonist (Sisler and Serek, 1997; Watkins, 2006), failed to inhibit the low temperature-triggered degreening responses. Our results suggest that low temperature might modulate citrus peel degreening independently of basal endogenous ethylene.
Materials and methods
Plant material and treatments
Lemon fruit (Citrus limon L. cv. ‘Allen Eureka’) grown under standard cultural practices were collected in 2018 from a commercial orchard in Takamatsu, Kagawa, Japan. To examine on-tree degreening, sampling took place at seven time-points during fruit development on 3 Sept., 27 Sept., 12 Oct., 30 Oct., 14 Nov., 29 Nov., and 13 Dec., corresponding to 171, 196, 211, 230, 246, 261, and 276 d after full bloom (DAFB), respectively. To characterize postharvest effects of ethylene, fruit at 196 DAFB were divided into four groups containing 20 replicates each. The first group was used as the untreated control, the second group was treated with 2 µl l–1 1-MCP for 12 h, the third group was continuously treated with 100 µl l–1 ethylene, while the fourth group was initially treated with 2 µl l–1 1-MCP for 12 h followed by continuous treatment with 100 µl l–1 ethylene. 1-MCP was released by dissolving SmartFresh™ powder (AgroFresh, PA, USA) in water. All treatments were carried out at 25 °C for up to 8 d. For postharvest storage tests, fruit at 196 DAFB were divided into five groups of 40 replicates each, and stored at either 5, 10, 15, 20, or 25 °C for up to 42 d. In addition, three separate groups of 40 fruit each were stored at either 5, 15, or 25 °C with 1-MCP treatments for 12 h repeated twice a week. Samples of fruit peel (flavedo) were collected, frozen in liquid nitrogen, and stored at –80 °C for future analysis. At each sampling time, the flavedo was collected from each of three replicate fruits.
Determination of the citrus colour index (CCI)
The L, a, and b Hunter lab parameters (Jiménez-Cuesta et al., 1981) were measured at four even, equatorial sites on the fruit surface using a CR-200B chromameter (Konica Minolta). CCI values are presented as the results of 1000a/Lb transformation (Ríos et al., 2010), and are expressed as the mean value of five fruits.
Determination of chlorophyll and carotenoid content
Chlorophylls were extracted and quantified from three replicate fruits per treatment according to the procedure described by Rodrigo et al. (2003), with slight modifications. Chlorophylls were extracted in 80% acetone and appropriate dilutions were used to quantify absorbance at 646.8 nm and 663.2 nm. The content was calculated from these measurements using the Lichtenthaler and Wellburn equations (Wellburn, 1994). Extraction and quantification of carotenoids were conducted using three replicate fruits per treatment according to the procedures described by Kato et al. (2004) and Matsumoto et al. (2007), with slight modifications. Briefly, carotenoids were successively extracted with 40% methanol and diethyl ether/methanol (containing 0.1% butylated hydroxytoluene). After saponification with methanolic potassium hydroxide, the organic layer of the extracts was vacuum-dried and analysed by HPLC. The HPLC analysis was carried out on an Extrema LC-4000 system (Jasco, Tokyo, Japan) equipped with a photodiode-array detector and autosampler. Samples were analysed on a Develosil C30-UG column (3 µm, 150×4.6 mm, Nomura Chemicals, Aichi, Japan) set at 20 °C and 0.5 ml min–1 flow rate. The UV-Vis spectra were obtained between 250 nm and 550 nm, and chromatograms were processed at 450 nm. The quantification of carotenoids was based on curves generated using authentic standards.
Phytohormone measurements
Phytohormone extraction and analysis were performed according to the method described by Gupta et al. (2017), using deuterium-labelled internal standards for indole-3-acetic acid (IAA), abscisic acid (ABA), jasmonic acid (JA), gibberellins (GAs), trans-zeatin (tZ), N6-isopentenyladenine (iP), and salicylic acid (SA), and 13C-labelled jasmonoyl-L-isoleucine (JA-Ile). Eluted fractions were analysed on a 1260–6410 Triple Quad LC/MS system equipped with a ZOR-BAX Eclipse XDB-C18 column (Agilent Technologies). Liquid chromatography conditions are described in Supplementary Table S1 at JXB online, and the multiple-reaction-monitoring mode of the tandem quadrupole MS and precursor-product ion transitions for each compound are listed in Supplementary Table S2.
RNA-seq and differential gene expression analysis
Total RNA was extracted from the flavedo of three replicate fruits from the control and ethylene groups after 4 d of treatment, as well as from fruit after 28 d of storage at 5, 15, or 25 °C. Illumina paired-end libraries were constructed using a NEBNext® Ultra™ RNA Library Prep Kit for Illumina (New England Biolabs), before being sequenced using an Illumina HiSeq 2500 platform (Hokkaido System Co. Ltd., Japan). Trimming was done to obtain ≥10 million paired reads per sample, and the reads were mapped to the reference Citrus clementina Genome v1.0 (Wu et al., 2014). Gene expression levels were calculated using the reads per kilobase per million (RPKM) method and differentially expressed genes (DEGs) were identified using false-discovery rates (FDR) analysis (Robinson et al., 2010). DEG selection was based on two criteria, namely genes with RPKM≥3.0 and FDR≤0.001, and fold-change≥3.0 in the mean RPKM for ethylene versus control, 5 °C versus 25 °C, and/or 15 °C versus 25 °C. For co-expression analysis, the weighted gene co-expression network analysis (WGCNA) method (Zhang and Horvath, 2005) was used to generate modules of highly correlated genes based on the RNA-seq expression data. Gene modules were identified by implementing the WGCNA package in R (Langfelder and Horvath, 2008). The soft-thresholding power and tree-cut parameters used for the WGCNA analysis were 12 and 0.15, respectively. Significantly enriched gene ontology (GO) terms were established using the agriGO (v.2.0) web-based toolkit (Tian et al., 2017), using hypergeometric tests followed by a Bonferroni correction to calculate a P-value for each term. We used P<0.05 as the cut-off for a significantly enriched GO term.
Reverse-transcriptase quantitative PCR (RT-qPCR)
Total RNA was extracted from the flavedo of fruit at the time of sampling from the tree (0 d, on-tree fruit), after 4 d for the ethylene, 1-MCP+ethylene, and control groups, and after 28 d storage at either 5, 10, 15, 20, or 25 °C. To remove genomic DNA contamination from the extracted RNA, treatment with DNase I (Nippon Gene, Tokyo, Japan) was carried out, followed by clean-up with FavorPrep after using a Tri-Reagent RNA Clean-up Kit (Favorgen Biotech. Co., Ping-Tung, Taiwan). For all treatments, 2.4 µg of clean RNA was reverse-transcribed to cDNA using a TaKaRa RNA PCR™ kit. Gene-specific primers were designed using the Primer3 software (v.0.4.0, http://bioinfo.ut.ee/primer3-0.4.0/;Supplementary Table S3). The gene expression of three biological replicates was examined using a MYiQ Single-Color Reverse Transcriptase-Quantitative PCR Detection System (Bio-Rad) using TB Green™ Premix ExTaq™ II (Takara). AcActin (Ciclev10025866m.g) was used as the housekeeping gene after examining its constitutive expression pattern in the RNA-seq results. Relative expression values were calculated using fruit at 196 DAFB (0 d).
Statistical analysis
Data were subjected to statistical analysis using R v.3.4.0 (www.r-project.org). Differences in CCI, pigment and phytohormone contents, and gene expression were determined using ANOVA followed by Tukey’s post hoc test.
Results
Ethylene-induced degreening
In response to ethylene treatment, a colour change was initiated in the peel from green to yellow after 2 d, and a full yellow colour developed after 8 d (Fig. 1A). This colour change was indicated by a rapid increase in CCI from –14.2 at harvest (0 d) to –1.8 after 8 d. However, it was notable that fruit pre-treated with 1-MCP followed by continuous ethylene treatment retained their greenish colour and showed no significant changes in CCI throughout the experimental period.
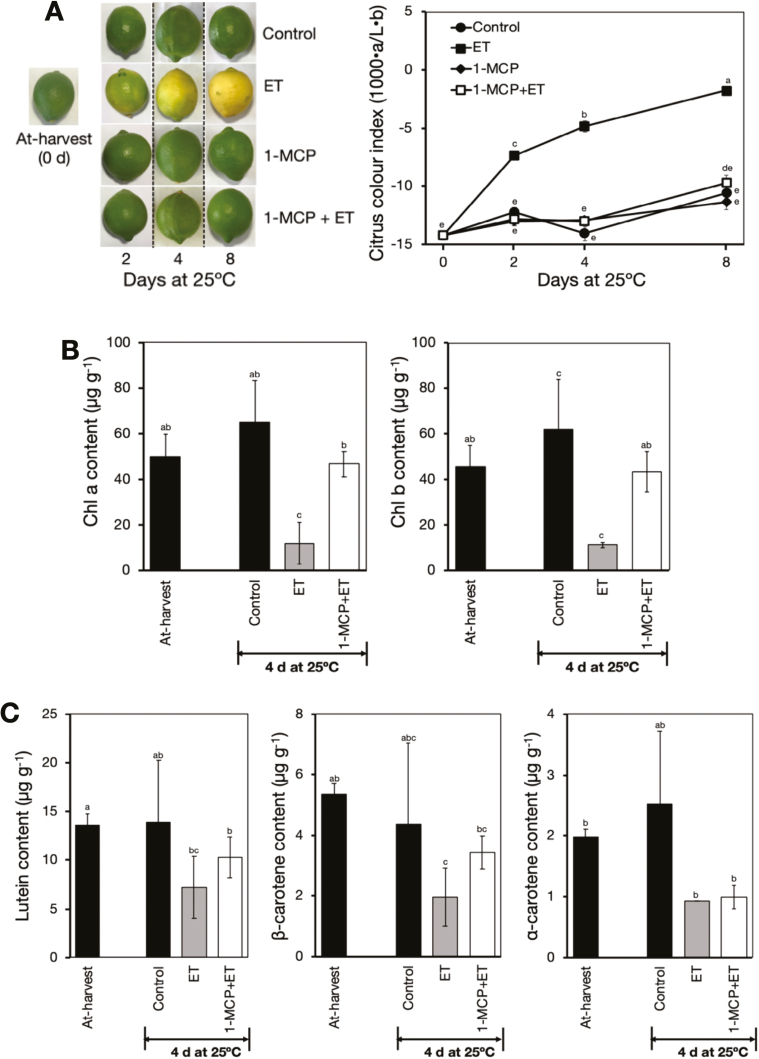
Ethylene-induced peel degreening in detached lemon fruit. (A) Appearance and colour index of fruit in response to treatment with ethylene and/or 1-methylcyclopropene (1-MCP). Fruit were either untreated (control), continuously treated with 100 µl l–1 ethylene (ET), treated with 2 µl l–1 1-MCP for 12 h (1-MCP), or pre-treated with 2 µl l–1 1-MCP for 12 h followed by continuous treatment with 100 µl l–1 ethylene (1-MCP+ET). An increase in the colour index indicates the fruit changing from green to yellow. (B) Changes in peel chlorophyll contents in response to ethylene and/or 1-MCP treatments. (C) Changes in peel carotenoid contents in response to ethylene and/or 1-MCP treatments. Data are means (±SE) of five replicate fruits. Different letters indicate significant differences between means as determined using ANOVA followed by Tukey’s test (P<0.05).
To further determine the role of ethylene in citrus peel degreening, we examined changes in the major colour pigments, namely chlorophylls and carotenoids. Both chlorophyll a and b in the peel decreased drastically upon ethylene treatment (from ~50 µg g–1 at 0 d to only 11 µg g–1 at 4 d), and pre-treatment with 1-MCP completely abolished this effect (Fig. 1B). Lutein, β-carotene, and α-carotene were the major carotenoids in the peel of the fruit (Supplementary Fig. S1), and these results were in close agreement with those of Agócs et al. (2007). Interestingly, all the three identified carotenoids displayed a substantial decrease after 4 d of ethylene treatment, and the ethylene-induced reduction was also inhibited by pre-treatment with 1-MCP (Fig. 1C). Taken together, these results demonstrated that ethylene played a key role in regulating peel degreening, and that 1-MCP treatment effectively rendered the fruit insensitive to ethylene.
Peel degreening at different storage temperatures and the effects of 1-MCP
Changes in peel colour in detached fruit were also investigated during storage at different temperatures. The colour of the fruit at 5–20 °C gradually changed from green to yellow, with a concomitant increase in CCI to about –2.3 after 28–42 d (Fig. 2A). Peel degreening was most pronounced at 15 °C, followed by 10 °C and 20 °C, whereas at 5 °C the fruit retained an appreciable greenish colour even after 42 d. In contrast, fruit at 25 °C retained their greenish colour and there were minimal changes in CCI across the storage period. These results indicated that moderately low storage temperatures promoted peel degreening in lemon fruit.
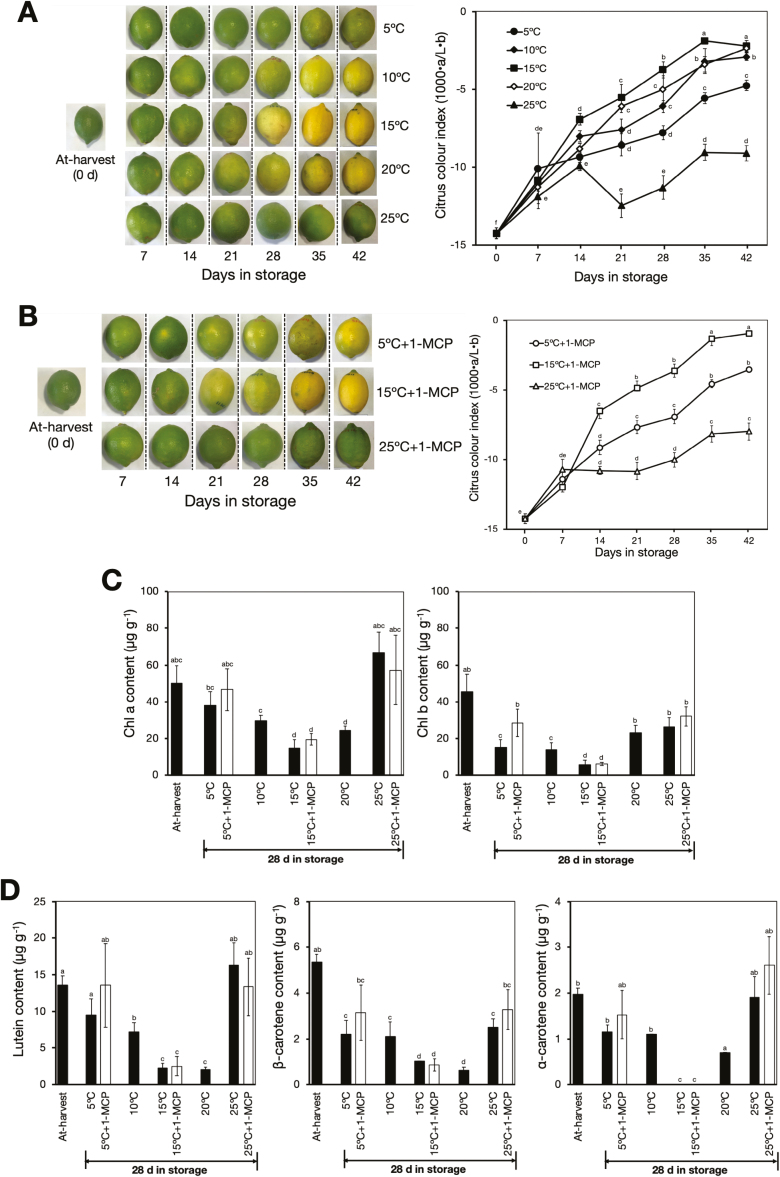
Promotion of peel degreening by low temperature in detached lemon fruit. (A) Appearance and colour index of fruit during storage at 5–25 °C in an ethylene-free environment. An increase in the colour index indicates the fruit changing from green to yellow. (B) Effects of 1-MCP treatment on peel colour changes during storage at 5, 15, or 25 °C. Treatments with 1-MCP (2 µl l–1) were carried out twice a week to keep fruit insensitive to ethylene. (C) Peel chlorophyll contents at different storage temperatures with or without 1-MCP treatment. (D) Peel carotenoid contents during storage at different temperatures with or without 1-MCP treatment. Data are means (±SE) of five replicate fruits. Different letters indicate significant differences between means as determined using ANOVA followed by Tukey’s test (P<0.05)
To determine whether the basal levels of System I ethylene present in the mature fruit played a role in the degreening that we observed at low temperature, we treated fruit at 5, 15, and 25 °C twice a week with 1-MCP. Surprisingly, comparable degreening was observed in fruit at 5 °C and 15 °C but not at 25 °C, notwithstanding the repeated 1-MCP treatments (Fig. 2B). This suggested that low temperature might have promoted the colour changes in the fruit peel independently of ethylene.
The promotion of degreening by low temperature was further investigated through determination of the chlorophyll and carotenoid contents of the peel. We found that there were substantial decreases in chlorophyll a and b contents after 28 d of storage at moderately low temperatures (5–20 °C), whereas no significant changes were observed at 25 °C (Fig. 2C). The chlorophyll content also decreased in fruit at 5 °C and 15 °C despite repeated 1-MCP treatments. Similar to the ethylene experiment, we observed a substantial decrease in the peel contents of lutein, β-carotene, and α-carotene at 5–20 °C, while there were no observable changes at 25 °C (Fig. 2D). However, it was notable that repeated 1-MCP treatments did not inhibit the reduction in the contents of the carotenoids at 5 °C and 15 °C. Taken together, these results indicated that, relative to 25 °C, low storage temperatures modulated peel degreening in lemon fruit, possibly in an ethylene-independent manner.
Differential expression analysis in the flavedo
Overview of the changes in the transcriptome.
To gain deeper insights into the mechanisms of low-temperature promotion of peel degreening, we conducted a comprehensive transcriptome analysis to compare the temperature-induced responses with those activated by ethylene. The ethylene-induced responses were examined by comparing flavedo samples from controls with those of fruit subjected to 4 d of ethylene treatment. For the responses triggered by low temperature, samples obtained after 28 d of storage at either 5 °C or 15 °C were compared with those at 25 °C.
RNA-seq analysis identified 3105 DEGs (q-value<0.001) that were regulated by either ethylene or low temperature (Fig. 3). Of these, ethylene accounted for 2329, compared with the 5 °C and 15 °C treatments that accounted for 1634 and 597, respectively (Fig. 3A). In all treatments, the number of down-regulated DEGs was higher than that of up-regulated ones. Ethylene treatment exclusively up- and down-regulated 592 and 700 genes, respectively (Fig. 3B). A combined total of 337 and 439 genes were exclusively up- and down-regulated, respectively, by the 5 °C and 15 °C treatments. The remaining DEGs (420 up-regulated and 617 down-regulated) were jointly influenced by ethylene, 5 °C and/or 15 °C. Subsequent WGCNA of the total 3105 DEGs identified eight modules (clusters of highly interconnected genes; Fig. 3C), and heatmapping indicated that the largest of these modules comprised genes that were down-regulated by ethylene or low temperature. The second largest was the module comprising genes exclusively up-regulated by ethylene. The remaining modules included genes that were differentially regulated by both ethylene and low temperature.
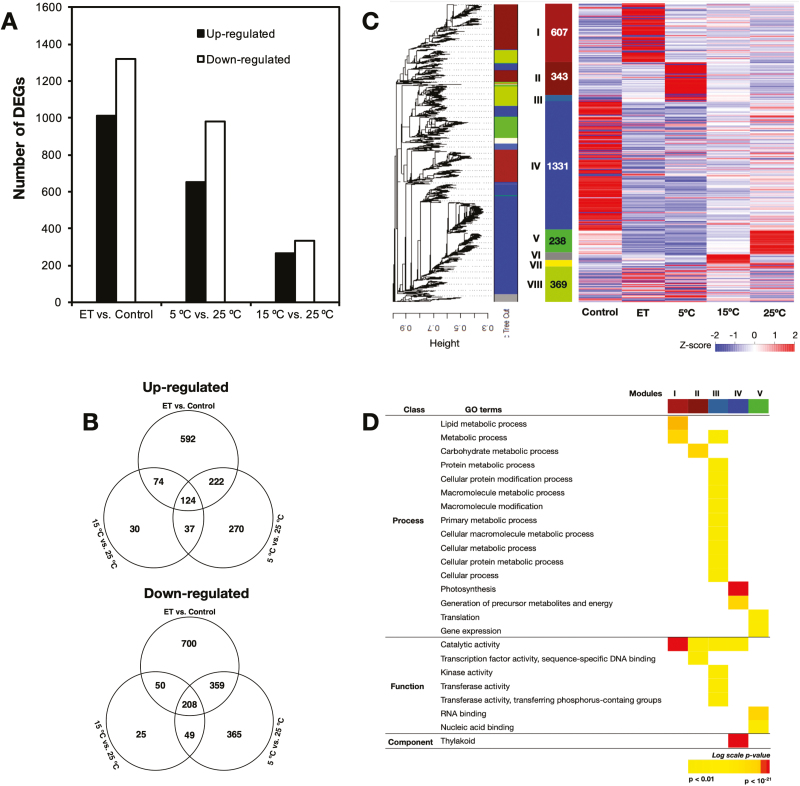
Global transcriptome changes induced by ethylene treatment and low temperature treatments in the flavedo of detached lemon fruit. (A) The numbers of differentially expressed genes (DEGs) in response to ethylene (ET), 5 °C, and 15 °C. (B) Venn diagrams showing the up- and down-regulated DEGs in response to ethylene, 5 °C, and 15 °C. (C) Weighted gene co-expression network analysis (WGCNA) and subsequent heatmap visualization of the 3105 DEGs from RNA-seq data. Each end branch in the tree represents one of the DEGs and each major branch represents one of the modules. The panel with total gene numbers shows the eight different modules obtained after implementing the tree cut-off (0.15) to merge close modules. The panel at the right shows a heatmap visualization of the expression measures of DEGs in each module in response to ethylene and the different storage temperatures. (D) Selected GO terms enriched among the WGCNA co-expressed DEGs in response to ethylene, 5 °C, and 15 °C.
Based on the WGCNA results, we performed GO classification to further examine the molecular changes involved. Dominant GO terms in Module I (comprising genes specifically up-regulated by ethylene) were ‘metabolic process’ and catalytic activity’ (Fig. 3D). There was also significant enrichment of DEGs associated with ‘metabolic process’, ‘macromolecule modification’, ‘cellular process’, ‘photosynthesis’, ‘thylakoid’, ‘metabolite precursors’, ‘translation’, ‘gene expression’ and ‘catalytic’ activity in Modules II, III, IV, and V, indicating that they were enriched by both ethylene and low temperature. Other GO terms that were enriched in response to ethylene or low temperature were related to ‘transcription factor activity’, ‘transferase activity’, ‘RNA binding’, and ‘nucleic acid binding’. No enriched GO terms were detected for DEGs in the Modules VI, VII, and VIII. It was notable that significant enrichment of DEGs related to ‘photosynthesis’ and ‘catalytic’ activity was observed in response to ethylene, 5 °C, and 15 °C (Supplementary Fig. S2). Overall, the DEGs identified could be pooled into three distinct groups. The first group comprised ethylene-specific genes, the second group included low temperature-specific genes, and the third group consisted of genes regulated by either ethylene or low temperature. Detailed information about the DEGs showing specific and shared responses to ethylene, 5 °C, and/or 15 °C is given in Supplementary Tables S4–S10.
Transcripts associated with chlorophyll metabolism.
One of the main processes contributing to peel degreening is chlorophyll degradation (Hörtensteiner, 2006). We therefore examined the expression patterns of chlorophyll metabolism genes in the RNA-seq data, and found that only three of the 10 identified DEGs were up-regulated by ethylene or low temperature (Fig. 4A, Supplementary Table S11). Of these genes, two (ClCLH1 and ClPPH) have been previously associated with chlorophyll degradation in citrus fruit (Jacob-Wilk et al., 1999; Yin et al., 2016). RT-qPCR analysis confirmed that ClCLH1 was up-regulated only in response to ethylene treatment, while expression of ClPPH increased in response to ethylene or low temperature (Fig. 4B). Interestingly, however, repeated 1-MCP treatments failed to suppress the increased expression levels of ClPPH at 5 °C and 15 °C despite a single treatment inhibiting ethylene-induced changes.
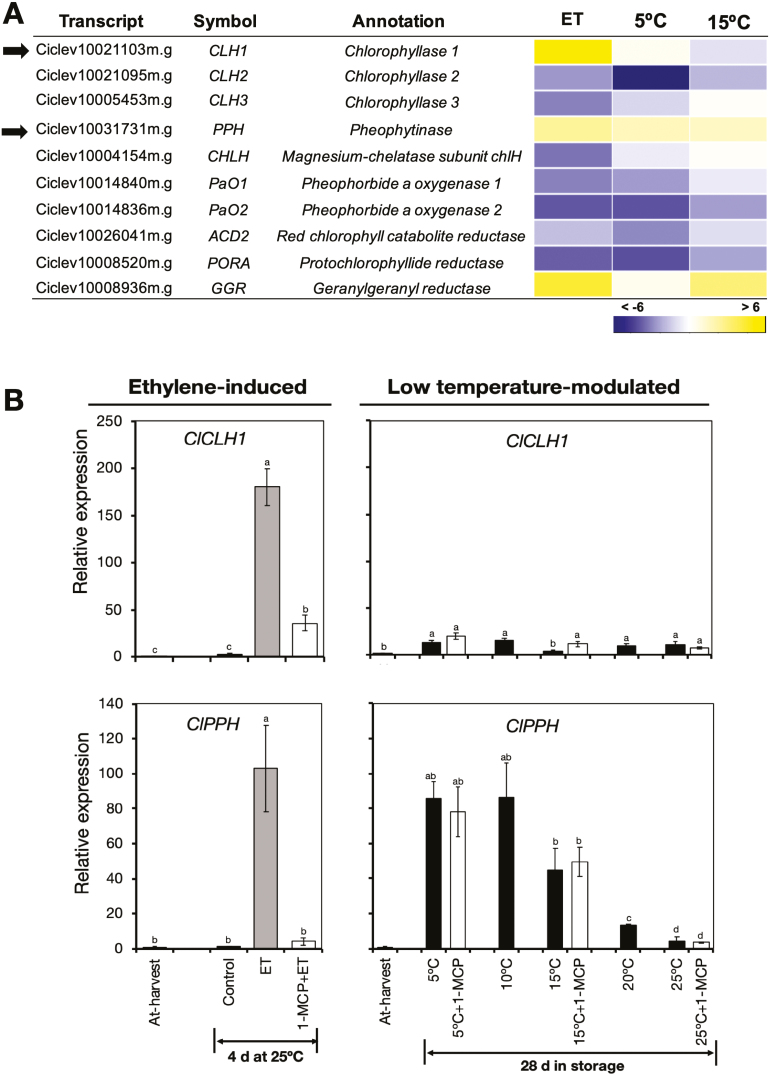
Expression of genes related to chlorophyll metabolism in the flavedo of detached lemon fruit in response to ethylene or low temperatures. (A) Heatmap showing identified differentially expressed genes associated with chlorophyll metabolism in fruit exposed to ethylene, 5 °C, or 15 °C. The data are expressed as the log2 value of fold-change for ethylene (ET) versus control, 5 °C versus 25 °C, and 15 °C versus 25 °C. (B) RT-qPCR analysis of the expression of chlorophyllase 1 (ClCLH1) and pheophytinase (ClPPH), indicated by the arrows in (A), which are known to be involved in chlorophyll degradation in many plant species. Expression is relative to the value at harvest and the housekeeping gene was AcActin. Data are means (±SE) of three replicate fruits. Different letters indicate significant differences between means as determined using ANOVA followed by Tukey’s test (P<0.05).
Transcripts associated with carotenoid metabolism.
The other process that leads to peel degreening is carotenoid metabolism, and the RNA-seq data identified 13 DEGs associated with this (Fig. 5A, Supplementary Table S11). Of these genes, ClPSY1, ClLCYb2a, and ClCHYb1 displayed high RPKM values and unique expression patterns, and we selected them for further analysis by RT-qPCR. This revealed that ClPSY1 and ClLCYb2a were up-regulated during both ethylene-induced and low temperature-modulated degreening, while ClCHYb1 was up-regulated exclusively by low temperature (Fig. 5B). The expression of all three genes increased in the peel of fruit at 5 °C and 15 °C despite repeated 1-MCP treatments.
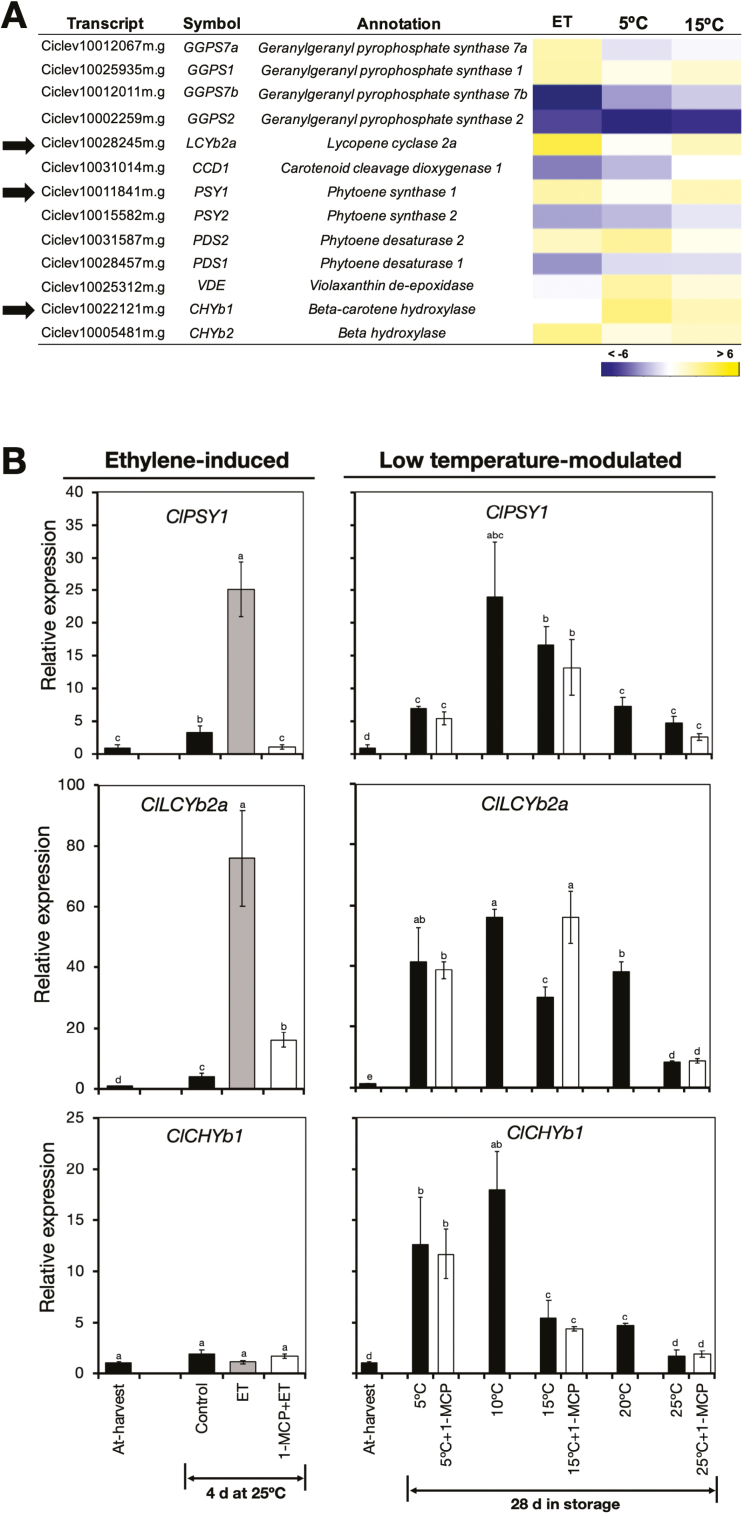
Expression of genes related to carotenoid metabolism in the flavedo of detached lemon fruit in response to ethylene or low temperatures. (A) Heatmap of identified differentially expressed genes associated with carotenoid metabolism in fruit exposed to ethylene, 5 °C, or 15 °C. The data are expressed as the log2 value of fold-change for ethylene (ET) versus control, 5 °C versus 25 °C, and 15 °C versus 25 °C. (B) RT-qPCR analysis of the expression of phytoene synthase 1 (ClPSY1), lycopene cyclase 2a (ClLCYb2a), and β-carotene hydroxylase 1 (ClCHYb1), indicated by the arrows in (A). Expression is relative to the value at harvest and the housekeeping gene was AcActin. Data are means (±SE) of three replicate fruits. Different letters indicate significant differences between means as determined using ANOVA followed by Tukey’s test (P<0.05).
Transcripts encoding photosystem proteins.
Genes encoding photosystem proteins also featured prominently among the identified DEGs, and most of them were down-regulated by both ethylene treatment and low temperature (Fig. 6A, Supplementary Table S11); however, ethylene appeared to have the greater influence on their down-regulation. Since most of the genes in this category showed a similar expression pattern, we selected only one, light harvesting complex 2 (ClLHCB2), for validation and further analysis by RT-qPCR, and the results confirmed that both ethylene treatment and low temperature caused a reduction in expression levels (Fig. 6B). Nevertheless, repeated 1-MCP treatments did not suppress the decrease in expression induced at 5 °C and 15 °C, suggesting that the influence of low temperature on ClLHCB2 expression was independent of ethylene.
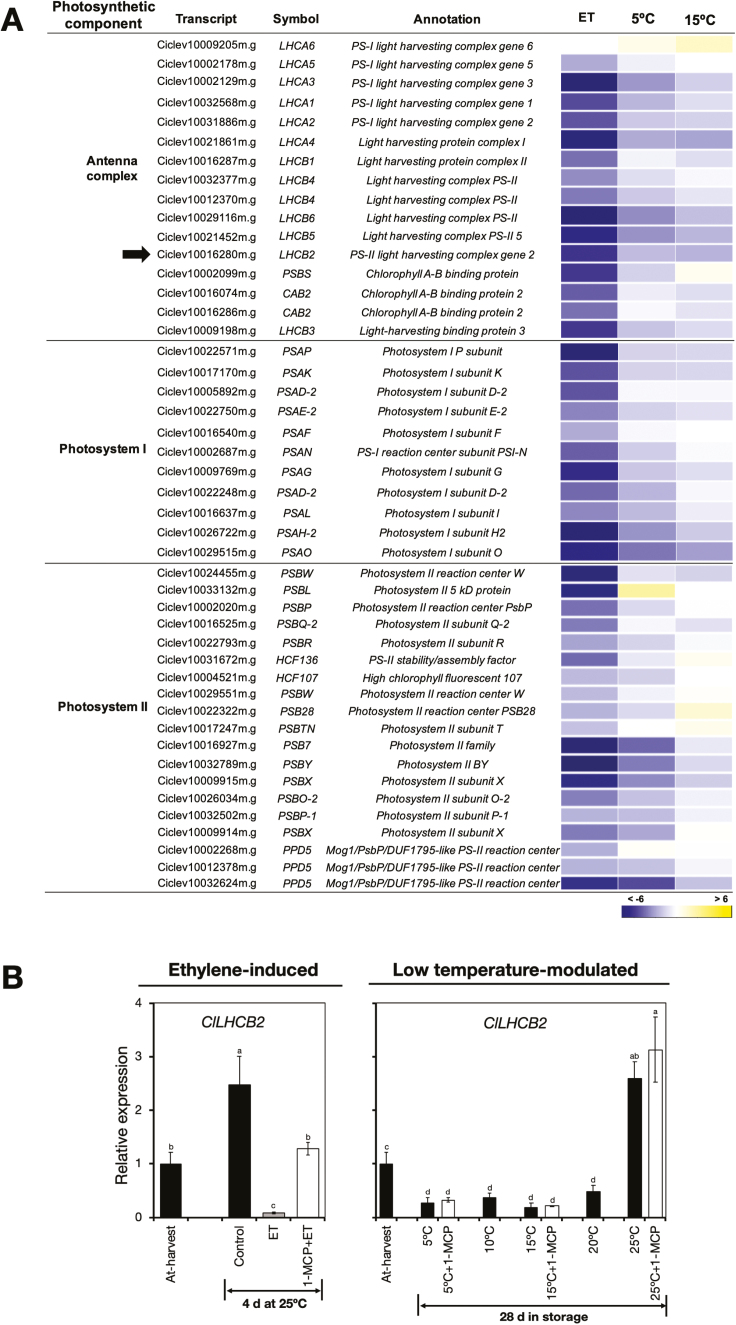
Changes in the expression of genes encoding photosystem proteins in the flavedo of detached lemon fruit in response to ethylene or low temperatures. (A) Heatmap of identified differentially expressed genes encoding photosystem proteins in fruit exposed to ethylene, 5 °C, or 15 °C. The data are expressed as the log2 value of fold-change for ethylene (ET) versus control, 5 °C versus 25 °C, and 15 °C versus 25 °C. (B) RT-qPCR analysis of the expression of light harvesting complex 2 (ClLHCB2), indicated by the arrow in (A). Expression is relative to the value at harvest and the housekeeping gene was AcActin. Data are means (±SE) of three replicate fruits. Different letters indicate significant differences between means as determined using ANOVA followed by Tukey’s test (P<0.05).
Phytohormone levels and associated transcripts.
Another prominent category among the identified DEGs included genes that were associated with the biosynthesis and signalling of phytohormones, especially ethylene, JA, ABA, auxin, and GA (Fig. 7A). Most of the ethylene-related genes were up-regulated by ethylene treatment, while low temperature only showed a slight effect on their expression, especially at 5 °C. Genes that were associated with JA and ABA were mostly up-regulated by both ethylene treatment and low temperature. Auxin-related genes showed varied expression patterns, although the general trend was towards down-regulation by both ethylene treatment and low temperature. We also identified three GA-associated DEGs, of which one, ClGA20ox2 that is associated with GA biosynthesis, was down-regulated by both ethylene treatment and low temperature, especially at 5 °C. In contrast, ClGA2ox4 and ClGA2ox8, which are associated with GA degradation, were up-regulated by ethylene treatment and by low temperature. To further examine the roles of ethylene and low temperature in the regulation of phytohormone-related genes, we selected 9-cis-epoxycarotenoid dioxygenase (ClNCED5), which is associated with ABA biosynthesis, for additional analysis by RT-qPCR. The results confirmed that it was up-regulated both after 4 d of ethylene exposure and 28 d of storage at low temperatures (5–20 °C; Fig. 7B). There was also a significant increase in ClNCED5 expression in fruit that were repeatedly treated with 1-MCP at 5 °C and 15 °C. The transcript levels of ClNCED5 were notably higher in fruit stored at low temperature than in fruit treated with ethylene.
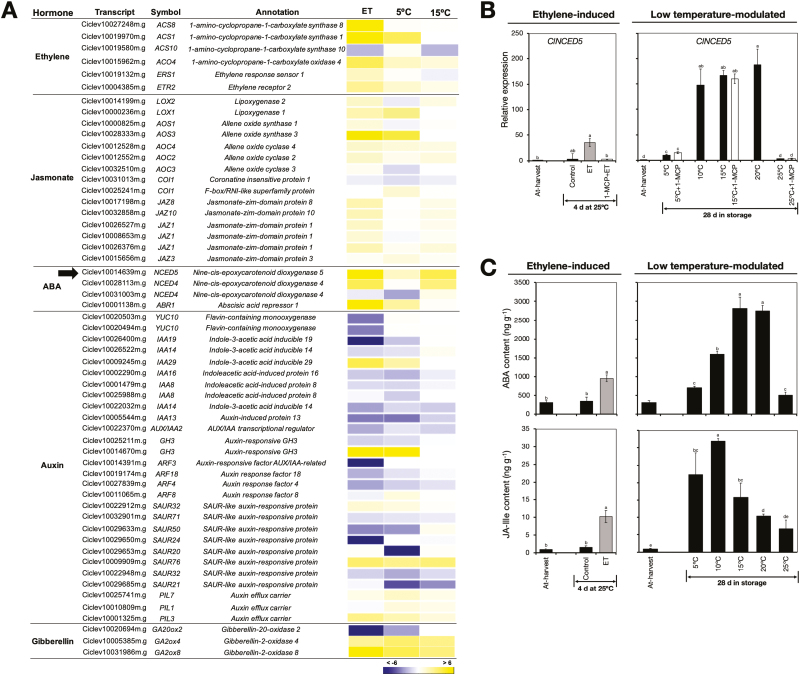
Levels of phytohormones and the expression of associated genes in the flavedo of detached lemon fruit. (A) Heatmap showing differentially expressed genes encoding proteins associated with phytohormone biosynthesis and signalling in fruit exposed to ethylene or low temperatures. The data are expressed as the log2 value of fold-change for ethylene (ET) versus control, 5 °C versus 25 °C, and 15 °C versus 25 °C. (B) RT-qPCR analysis of the ABA biosynthetic gene 9-cis-epoxycarotenoid dioxygenase 5 (ClNCED5), indicated by the arrow in (A). Expression is relative to the value at harvest and the housekeeping gene was AcActin. (C) Levels of ABA and JA-Ile in lemon fruit treated with ethylene or after 28 d storage at the indicated temperatures. Data are means (±SE) of three replicate fruits. Different letters indicate significant differences between means as determined using ANOVA followed by Tukey’s test (P<0.05).
The results described above motivated us to determine the phytohormone content in the flavedo of fruit exposed to ethylene and different storage temperatures. The results indicated that both caused a significant increase in ABA and JA-Ile levels (Fig. 7C) In particular, levels were substantially higher in fruit stored at low temperatures than in fruit treated with ethylene. Unfortunately, we were not able to detect other hormones because of their extremely low endogenous levels and severe ion suppression effects during the LC/MS analysis.
Transcripts encoding transcription factors.
A total of 128 DEGs in the RNA-seq data were found to encode a wide range of putative TF families, including AP2/ERF, bHLH, MYB, NAC, GRAS, zinc finger, homeobox, WRKY, MADS, and TCP (Fig. 8A, Supplementary Table S11). This underscored the relevance of TF activity in the peel degreening process of lemon fruit. We pooled the identified genes into three distinct groups, namely those that were influenced by ethylene only, such as ClERF114, those that were influenced by low temperature only, such as ClERF3, and those that were influenced by both ethylene and low temperature, such as ClbHLH25. RT-qPCR analysis confirmed that ClERF114 was exclusively up-regulated by ethylene treatment as its expression was maintained at minimal levels during storage (Fig. 8B). In contrast, ClERF3 was exclusively up-regulated by low temperature since marginal expression levels were observed in ethylene-treated fruit (Fig. 8C). Finally, ClbHLH25 expression increased both upon ethylene treatment and after storage at temperatures lower than 25 °C (Fig. 8D). It was also notable that repeated 1-MCP treatments failed to abolish the up-regulation of ClERF3 and ClbHLH25 at 5 °C and 15 °C (Fig. 8C, ,DD).
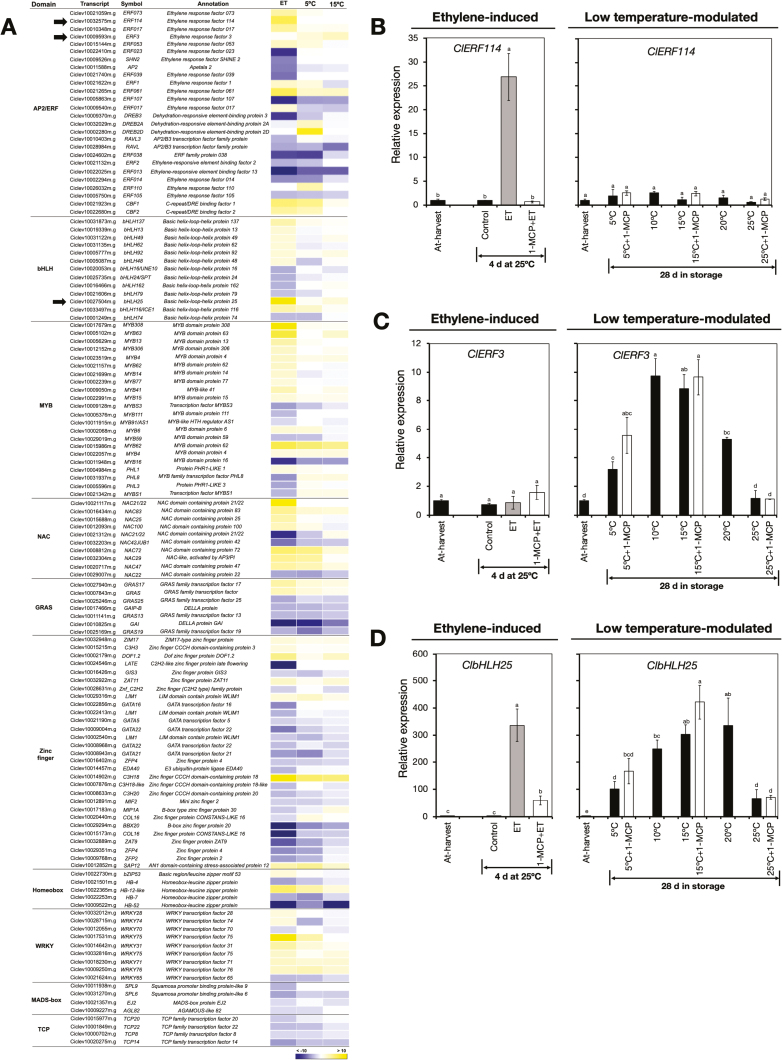
Changes in expression of genes encoding transcription factors in the flavedo of detached lemon fruit. (A) Heatmap showing identified differentially expressed genes encoding various transcription factors in fruit exposed to ethylene or low temperatures. The data are expressed as the log2 value of fold-change for ethylene (ET) versus control, 5 °C versus 25 °C, and 15 °C versus 25 °C. (B–D) RT-qPCR analysis of the relative expression of (B) ClERF114, (C) ClERF3, and (D) ClbHLH25, as indicated by the arrows in (A). Expression is relative to the value at harvest and the housekeeping gene was AcActin. Data are means (±SE) of three replicate fruits. Different letters indicate significant differences between means as determined using ANOVA followed by Tukey’s test (P<0.05).
On-tree peel degreening and expression analysis of associated genes
The roles of ethylene and low temperature in natural peel degreening were investigated during on-tree maturation of lemon fruit. Fruit were harvested at seven progressive stages ranging from 171–276 DAFB between early September and mid-December. Peel colour progressively changed from green on 3 September to full yellow on 13 December, which was indicated by a concomitant increase in CCI from –16.3 to –1.1 (Fig. 9A). During this period, the mean minimum temperature in the orchard decreased gradually from 22.5 °C to 3.7 °C. The increase in CCI was initially slow between 3 September to 12 October (from –16.3 to –14.2) when the minimum temperatures were above 13 °C; however, CCI increased rapidly between 12 October and 13 December (from –14.2 to –1.1) when the minimum temperatures were below 13 °C. The observed loss of green colour during on-tree maturation corresponded closely with a gradual decrease in the chlorophyll a and b contents in the peel (Fig. 9B). Degreening was also accompanied by a gradual decline in the peel contents of lutein, β-carotene, and α-carotene (Fig. 9C).
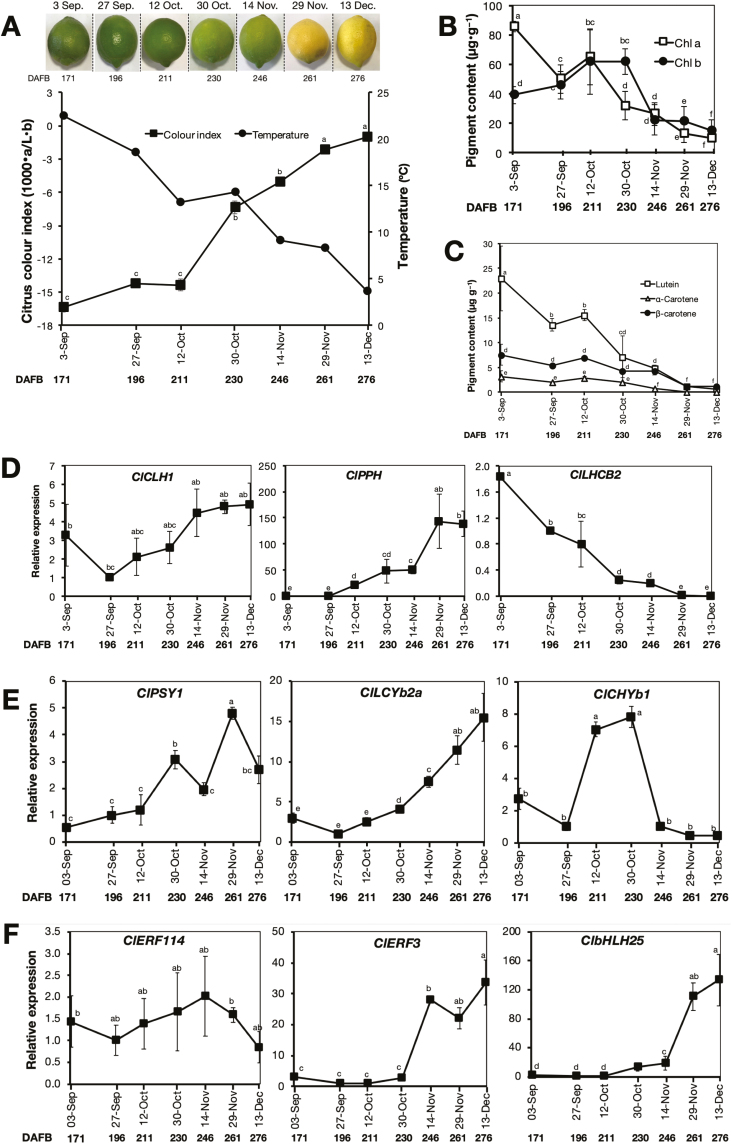
Changes in peel colour and gene expression analysis in lemon fruit during on-tree maturation. (A) Appearance and citrus colour index of representative fruit at different developmental stages together with data for minimum ambient temperatures. An increase in the colour index indicates the fruit changing from green to yellow. The temperature data were accessed from the website of Japan Meteorological Agency (http://www.data.jma.go.jp/obd/stats/etrn/view/daily_s1.php?prec_no=72&block_no=47891&year=2014&month=12&day=&view=p1). (B) Chlorophyll a and b contents at different developmental stages. (C) Levels of lutein, α-carotene, and β-carotene at different developmental stages. (D–F) RT-qPCR analysis of the expression of selected genes associated with chlorophyll metabolism and photosystem proteins (D), carotenoid metabolism (E), and transcription factors (F) at different developmental stages. Expression is relative to the value at harvest and the housekeeping gene was AcActin. Data are means (±SE) of five replicate fruits. Different letters indicate significant differences between means as determined using ANOVA followed by Tukey’s test (P<0.05).
We then examined the expression patterns of selected genes induced by ethylene and/or low temperature as identified from the RNA-seq data. On-tree peel degreening coincided with up-regulation of ClPPH and down-regulation of ClLHCB2 (Fig. 9D), both of which we had established to be influenced by low temperature (Figs 4B, ,6B).6B). However, the ethylene-specific ClCLH1 did not show any significant changes in expression. On-tree degreening was also accompanied by up-regulation of all three of the carotenoid metabolism genes that we analysed, namely ClPSY1, ClLCYb2a, and ClCHYb1 (Fig. 9E), which we had established to be up-regulated by low temperature (Fig. 5B). Among the genes encoding TFs, the ethylene-specific ClERF114 did not show any significant expression changes, whereas both ClERF3 and ClbHLH25 were up-regulated from 30 October onwards, when the minimum temperatures were below 13 °C (Fig. 9F). Taken together, these results demonstrated strong similarities between on-tree and low temperature-modulated degreening, and further highlighted dissimilarities with ethylene-induced changes.
Discussion
A close association between low temperature and peel colouration has been described in several citrus species (Carmona et al., 2012b; Manera et al., 2012, 2013). In agreement with these findings, our results showed that moderately low storage temperatures (5–20 °C) promoted peel degreening in lemon fruit, and induced substantial changes in chlorophyll and carotenoid contents (Fig. 2). To date, the molecular mechanisms involved have not been determined, but because of the known involvement of ethylene in citrus degreening (Purvis and Barmore, 1981; Shemer et al., 2008; Yin et al., 2016), it has been suggested that it might play a role in the process, as trace levels of physiologically active System I ethylene are thought to be bound in tissues (Goldschmidt et al., 1993; Carmona et al., 2012b). In this current study, we present conclusive data demonstrating that low temperature can modulate natural peel degreening in lemon fruit independently of the ethylene signal.
Ethylene-induced degreening is completely inhibited by pre-treatment with 1-MCP (Fig. 1; Jomori et al., 2003; McCollum and Maul, 2007; Li et al., 2016) and 1-MCP treatment has been shown to inhibit the ripening process in fruit that have a strong requirement for ethylene to ripen (Watkins, 2006). In higher plants, ethylene receptors act as negative regulators (Hua and Meyerowitz, 1998), and their binding by ethylene subjects them to degradation via the ubiquitin-proteasome pathway (Kevany et al., 2007). 1-MCP is assumed to irreversibly bind to and phosphorylate the receptors (Kamiyoshihara et al., 2012) with a higher affinity than ethylene (Jiang et al., 1999), resulting in relatively stable complexes that suppress signalling even in the presence of ethylene. If endogenous ethylene is physiologically active during storage, then its action should be suppressed by the application of antagonists such as 1-MCP. In this study, however, it was surprising that peel degreening elicited by low temperature was not abolished by repeated 1-MCP treatments (Fig. 2), indicating that it most likely occurred in an ethylene-independent manner.
This conclusion was supported by the transcriptomic data that we obtained. Genes that were differentially regulated during lemon fruit peel degreening could be categorized into three distinct groups, first those regulated specifically by ethylene, second those regulated exclusively by low temperature, and third those regulated by ethylene or low temperature (Fig. 3). Ethylene-specific genes such as ClCLH1 and ClERF114 did not show any significant changes in expression during low temperature-modulated degreening (Figs 4B, ,8B),8B), indicating that ethylene signalling was non-functional in the stored fruit. In addition, our identification of low temperature-specific genes, including ClCHYb1 and ClERF3 (Figs 5B, ,8C),8C), strongly supports the concept that low temperature-modulated degreening involves a mechanism that operates outside the sphere of influence of ethylene. Although the third set of genes were differentially regulated by ethylene or low temperature, efforts to suppress the low-temperature regulation of key members (ClPPH, ClLHCB2, ClPSY1, ClLCYb2a, ClNCED5, and ClbHLH25) using repeated 1-MCP treatments were unsuccessful (Figs 4B, ,5B,5B, ,6B,6B, ,7B,7B, ,8D).8D). Thus, taken together with our physiological and metabolic data, it was evident that ethylene-independent regulation of peel degreening by low temperature was occurring in lemon fruit.
As chlorophyll degradation is the most obvious change leading to peel degreening (Fig. 1B, ,2C),2C), we examined the changes in expression of related genes that were induced by ethylene and low temperature. We found that ethylene treatment did indeed lead to increased expression levels of ClCLH1 and ClPPH (Fig. 4B), in agreement with previous findings in various citrus fruit species (Jacob-Wilk et al., 1999; Shemer et al., 2008; Yin et al., 2016). During storage, however, there were minimal changes in the expression of ClCLH1, whereas ClPPH was up-regulated at all the tested temperatures from 5–20 °C, in agreement with the reduction in chlorophyll content. ClPPH encodes an enzyme with similar dephytilation activity as CLH (Schelbert et al., 2009), thus providing a mechanism by which the loss of chlorophyll triggered by low temperature is regulated.
The carotenoid content of the peel decreased upon degreening in response to both ethylene and low temperature (Figs 1C, ,2D).2D). Such decreases are not uncommon as previous studies have also found similar results in lemon fruit during maturation, especially for lutein (Kato, 2012; Conesa et al., 2019). Nevertheless, the yellowish appearance of degreened fruit (Figs 1A, ,2A,2A, ,B)B) could be attributed to the small but significant levels of lutein, β-carotene, and α-carotene that were present (Fig. 1C, ,2D),2D), the appearance of which might have been enhanced by the loss of chlorophyll. The observation that the expression of carotenoid metabolism genes such as ClPSY1, ClLCYb2a, and ClCHYb1 were stimulated by ethylene or low temperature (Fig. 5) agreed with previous reports (Rodrigo and Zacarias, 2007; Matsumoto et al., 2009); however, this is the first study to demonstrate that carotenoid metabolism is modulated by low temperature independently of ethylene.
The degradation of chlorophylls caused by exposure to ethylene or low temperature could also be facilitated by changes in photosystem proteins. The disruption of pigment-protein complexes is thought to be a crucial step in the chlorophyll degradation pathway (Barry, 2009). Thus, the stay-green gene SGR, which encodes a Mg dechelatase (Shimoda et al., 2016), has been shown to aid the dis-aggregation of photosystem proteins, and in particular the light-harvesting chlorophyll a/b-binding (CAB) complex (Jiang et al., 2011; Sakuraba et al., 2012). Because photosystem proteins bind pigments, a large drop in their transcripts caused by ethylene or low temperature (Fig. 6) would possibly favour the accumulation of free chlorophylls that can easily be accessed by degradatory enzymes. Peng et al. (2013) have also reported that the transcript levels of CitCAB1 and CitCAB2 drastically decrease during ethylene-induced and natural peel degreening in ‘Ponkan’ mandarins. However, our results suggest that the decrease in the expression of photosystem-encoding genes during natural peel degreening could be stimulated by low temperature independently of ethylene.
Besides ethylene, various phytohormones including ABA, GA, and JA have been implicated in the colour changes that occur in the peel during the maturation of citrus fruit. Peel degreening has been shown to be accompanied by an increase in ABA content (Goldschmidt et al., 1973), as well as in the expression of ABA biosynthetic and signalling elements (Kato et al., 2006; Rodrigo et al., 2006). In addition, exogenous ABA accelerates fruit ripening and enhances fruit colour development (Wang et al., 2016), while ABA-deficient citrus mutants show a delay in the rate of peel degreening (Rodrigo et al., 2003). In our study, ABA levels increased in fruit treated with ethylene and in fruit stored at low temperatures (Fig. 7C), and this was accompanied by an increase in the expression of ABA biosynthetic and signalling genes (Fig. 7A, ,B).B). These findings, together with previous reports, suggest that ABA has a positive regulatory role in both ethylene-induced and low temperature-modulated peel degreening in lemon fruit. GA, on the other hand, is known to retard colour changes in the peel, and this effect is seen when it is applied to green citrus fruit (Alós et al., 2006; Rodrigo and Zacarias, 2007; Ríos et al., 2010). It was therefore logical that the transcript levels of the GA biosynthetic gene ClGA20ox2 decreased, whereas those of the degradatory genes ClGA2ox4 and GA2ox8 increased during peel degreening caused by ethylene or low temperature (Fig. 7A). JAs have largely been studied to date in the context of plant adaptive responses to various biotic and abiotic stresses (Zhang et al., 2020). However, they have also been shown to promote fruit ripening in citrus (Zhang et al., 2014), strawberry (Concha et al., 2013), and tomato (Liu et al., 2012). This is consistent with our findings, as degreening in lemon fruit was accompanied by an increase in the levels of JA-Ile (Fig. 7C), which is the active conjugate of JA. In addition, the expression of a large number of JA biosynthetic and signalling-related genes were independently upregulated by ethylene treatment or low temperature (Fig. 7A).
Developmentally regulated plant processes such as peel degreening are typically influenced by TFs. Various TFs in Arabidopsis such as NACs (AtNAC046 and AtORE1), bHLHs (AtPIF4 and AtPIF5), and ethylene insensitive 3 (AtEIN3) have been shown to significantly enhance leaf senescence by promoting the activity of genes related to chlorophyll degradation (Song et al., 2014; Qiu et al., 2015; Zhang et al., 2015; Oda-Yamamizo et al., 2016). In broccoli (Brassica oleracea), the MYB, bHLH, and bZIP gene families are associated with chlorophyll metabolism while NACs and ERFs regulate carotenoid biosynthesis (Luo et al., 2019). CitERF6 and CitERF13 have also been associated with chlorophyll degradation during ethylene-induced and natural peel degreening in citrus (Yin et al., 2016; Li et al., 2019). In our present work, the expression patterns of genes encoding a wide range of TF families suggested that the ethylene-induced and low temperature-modulated peel degreening pathways were distinct in lemon fruit (Fig. 8). Thus, ethylene-induced degreening is most likely to be regulated by ethylene-specific TFs such as ClERF114 (Fig. 8B) and shared TFs such as ClbHLH25 (Fig. 8D), whereas low temperature-modulated degreening could be regulated by specific TFs such as ClERF3 (Fig. 8C), as well as shared ones such as ClbHLH25 (Fig. 8D).
In our study, low temperature also appeared to play a prominent role in natural peel degreening during on-tree maturation of the lemon fruit. Peel degreening and the associated reduction in the content of chlorophylls and carotenoids coincided with the gradual decline in minimum ambient temperatures to below 13 °C (Fig. 9A–C). Previous studies have also demonstrated that peel degreening in most citrus fruit progresses as the ambient temperature decreases (Manera et al., 2012, 2013; Rodrigo et al., 2013; Conesa et al., 2019). It is intriguing that ClCLH1 and ClERF114, which we identified as exhibiting an ethylene-specific pattern of expression (Figs 4B, ,8B),8B), did not show any significant changes in expression during on-tree degreening (Fig. 9D, ,F).F). Previous studies have also demonstrated that ClCLH1 homologues in other citrus fruit exhibit a dramatic induction in response to ethylene treatment and yet lack a measurable increase in expression during natural degreening (Jacob-Wilk et al., 1999; Yin et al., 2016). We suggest that this discrepancy could be due to a lack of a functional ethylene signalling during on-tree maturation, given that ClCLH1 was only up-regulated in the presence of ethylene (Fig. 4B). In contrast, genes that responded to low temperatures during storage, namely ClPPH, ClLHCB2, ClPSY1, ClLCYb2a, ClCHYb1, ClERF3, and ClbHLH25, also exhibited similar expression patterns during on-tree maturation (Fig. 9D–F), indicating that they were also involved in the on-tree peel degreening processes. These similarities between low temperature-induced and on-tree gene expression patterns, coupled with their dissimilarities to ethylene-induced changes, provide clear evidence to suggest that on-tree peel degreening responses are modulated by low temperature independently of ethylene in lemon fruit.
It is also important to note that many genes were differentially expressed in fruit at 5 °C and yet the rate of peel degreening was significantly slower than in fruit at 10–20 °C. This was probably due to low activity of the enzymes associated with degreening at 5 °C, as low temperature is known to generally decrease enzyme activity in plants (Jin et al., 2009; Yun et al., 2012).
The regulation of fruit ripening by low temperature is not unique to citrus fruit. Previous studies have also demonstrated its role, either independently or in concert with ethylene, in the regulation of fruit ripening in various species such as kiwifruit (Mworia et al., 2012; Asiche et al., 2017, 2018; Mitalo et al., 2018b, 2019a, 2019b), pears (El-Sharkawy et al., 2003; Mitalo et al., 2019c), and apples (Tacken et al., 2010). From an ecological perspective, the primary purpose of ripening is to make fruit attractive to seed-dispersing organisms. To ensure their future survival, most temperate fruit species are faced with the challenge of dispersing their seeds in time before the onset of harsh winter conditions. Therefore, temperature drops associated with autumn might provide an alternative stimulus for inducing ripening in fruits such as citrus that lack a functional ethylene signalling pathway during maturation.
Based on the results of this study, we present a simplified model that summarizes the distinct ethylene-dependent and low temperature-modulated regulatory mechanisms of peel degreening in citrus fruit (Fig. 10). According to this model, both ethylene and low temperature cause changes in the levels of unique TFs (such as the ethylene-specific ClERF114- and the low temperature-specific ClERF3) as well as in shared TFs such as ClbHLH25. These then trigger the expression of various genes associated with chlorophyll degradation, carotenoid metabolism, photosystem disassembly, and phytohormones, resulting in peel degreening. In future studies, we aim to identify the direct and indirect targets of the low temperature-regulated genes that we have identified here, and thus to determine the molecular bases for the modulation of peel degreening and fruit ripening in general.
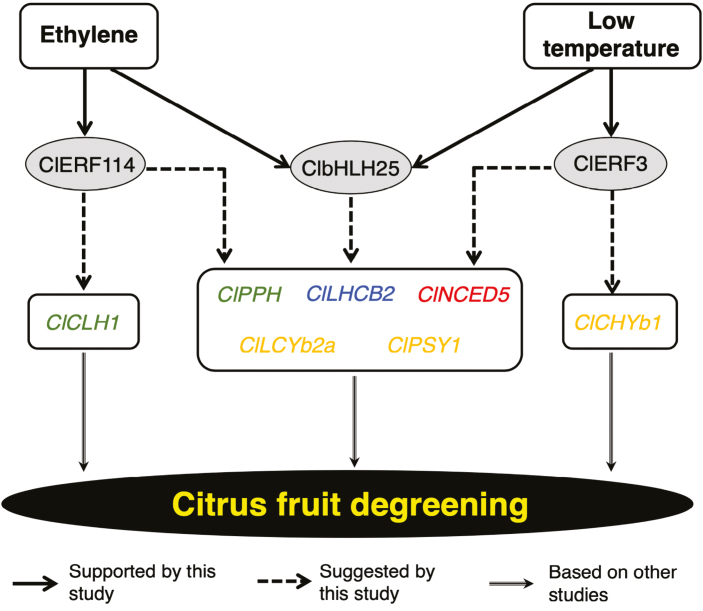
Proposed model for the distinct ethylene-dependent and low temperature-modulated peel degreening in lemon fruit. Uniquely ethylene-regulated transcription factors (TFs) are represented by ClERF114 while low temperature-specific TFs are represented by ClERF3. An additional set of TFs (represented by ClbHLH25) are shared between the ethylene and low-temperature regulatory pathways. Exposure to ethylene or low temperature induces changes in the levels of these unique and shared TFs, triggering the expression of various genes associated with chlorophyll degradation (green), carotenoid metabolism (orange), photosystem proteins (blues), and phytohormones (red).
Supplementary data
Fig. S1. Chromatogram showing the carotenoids identified in the peel of lemon.
Fig. S2. Selected GO terms enriched among DEGs responding to ethylene, and storage at 5 °C and 15 °C.
Table S1. Liquid chromatography conditions for phytohormone analysis.
Table S2. Parameters used for LC-ESI-MS/MS analysis of phytohormones.
Table S3. Primer sequences used for RT-qPCR.
Table S4. DEGs exclusively responding to ethylene.
Table S5. DEGs responding to ethylene or to low temperature at 5 °C.
Table S6. DEGs responding to ethylene or to low temperature at 15 °C.
Table S7. DEGs responding to either ethylene, or to low temperatures at 5 °C or 15 °C.
Table S8. DEGs exclusively responding to low temperature at 5 °C.
Table S9. DEGs responding to low temperature at either 5 °C or 15 °C.
Table S10. DEGs exclusively responding to low temperature at 15 °C.
Table S11. Selected DEGs associated with peel degreening.
eraa206_suppl_Supplementary-Figures-S1-S2
eraa206_suppl_Supplementary-Tables-S1-S2
eraa206_suppl_Supplementary-Tables-S3-S11
Acknowledgements
This study was supported in part by a Grant-in-Aid for Scientific Research (grant nos 24380023, 16H04873, and 20H02977) from the Japan Society for the Promotion of Science, and by the Joint Usage/Research Centre, Institute of Plant Science and Resources, Okayama University.
Data deposition
The RNA-seq data generated in this study have been deposited in the DDBJ Sequence Read Archive (accession numbers: DRR216064–DRR216079).
References
- Agócs A, Nagy V, Szabó Z, Márk L, Ohmacht R, Deli J. 2007. Comparative study on the carotenoid composition of the peel and the pulp of different citrus species. Innovative Food Science and Emerging Technologies 8, 390–394. [Google Scholar]
- Alós E, Cercós M, Rodrigo MJ, Zacarías L, Talón M. 2006. Regulation of color break in citrus fruits. Changes in pigment profiling and gene expression induced by gibberellins and nitrate, two ripening retardants. Journal of Agricultural and Food Chemistry 54, 4888–4895. [Abstract] [Google Scholar]
- Asiche WO, Mitalo OW, Kasahara Y, Tosa Y, Mworia EG, Owino WO, Ushijima K, Nakano R, Yano K, Kubo Y. 2018. Comparative transcriptome analysis reveals distinct ethylene-independent regulation of ripening in response to low temperature in kiwifruit. BMC Plant Biology 18, 47. [Europe PMC free article] [Abstract] [Google Scholar]
- Asiche WO, Mitalo OW, Kasahara Y, Tosa Y, Mworia EG, Ushijima K, Nakano R, Kubo Y. 2017. Effect of storage temperature on fruit ripening in three kiwifruit cultivars. The Horticulture Journal 86, 403–410. [Google Scholar]
- Barry CS. 2009. The stay-green revolution: recent progress in deciphering the mechanisms of chlorophyll degradation in higher plants. Plant Science 176, 325–333. [Google Scholar]
- Carmona L, Rodrigo MJ, Zacarías L. 2012a Exploring the involvement of ethylene in the regulation of color changes in citrus fruit. Acta Horticulturae 934, 879–885. [Google Scholar]
- Carmona L, Zacarías L, Rodrigo MJ. 2012b Stimulation of colouration and carotenoid biosynthesis during postharvest storage of ‘Navelina’ orange fruit at 12 °C. Postharvest Biology and Technology 74, 108–117. [Google Scholar]
- Cherian S, Figueroa CR, Nair H. 2014. ‘Movers and shakers’ in the regulation of fruit ripening: a cross-dissection of climacteric versus non-climacteric fruit. Journal of Experimental Botany 65, 4705–4722. [Abstract] [Google Scholar]
- Concha CM, Figueroa NE, Poblete LA, Oñate FA, Schwab W, Figueroa CR. 2013. Methyl jasmonate treatment induces changes in fruit ripening by modifying the expression of several ripening genes in Fragaria chiloensis fruit. Plant Physiology and Biochemistry 70, 433–444. [Abstract] [Google Scholar]
- Conesa A, Manera FC, Brotons JM, Fernandez-Zapata JC, Simón I, Simón-Grao S, Alfosea-Simón M, Nicolása JJM, Valverde JM, García-Sanchez F. 2019. Changes in the content of chlorophylls and carotenoids in the rind of ‘Fino 49’ lemons during maturation and their relationship with parameters from the CIELAB color space. Scientia Horticulturae 243, 252–260. [Google Scholar]
- Cunningham FX Jr, Pogson B, Sun Z, McDonald KA, DellaPenna D, Gantt E. 1996. Functional analysis of the beta and epsilon lycopene cyclase enzymes of Arabidopsis reveals a mechanism for control of cyclic carotenoid formation. The Plant Cell 8, 1613–1626. [Abstract] [Google Scholar]
- Eaks IL. 1970. Respiratory response, ethylene production, and response to ethylene of citrus fruit during ontogeny. Plant Physiology 45, 334–338. [Abstract] [Google Scholar]
- El-Sharkawy I, Jones B, Li ZG, Lelievre JM, Pech JC, Latche A. 2003. Isolation and characterization of four ethylene perception elements and their expression during ripening in pears (Pyrus communis L) with/without cold requirement. Journal of Experimental Botany 54, 1615–1625. [Abstract] [Google Scholar]
- Goldschmidt EE, Goren R, Even-Chen Z, Bittner S. 1973. Increase in free and bound abscisic acid during natural and ethylene-induced senescence of citrus fruit peel. Plant Physiology 51, 879–882. [Abstract] [Google Scholar]
- Goldschmidt EE, Huberman M, Goren R. 1993. Probing the role of endogenous ethylene in the degreening of citrus fruit with ethylene antagonists. Plant Growth Regulation 12, 325–329. [Google Scholar]
- Gupta A, Hisano H, Hojo Y, Matsuura T, Ikeda Y, Mori IC, Snthil-Kumar M. 2017. Global profiling of phytohormone dynamics during combined drought and pathogen stress in Arabidopsis thaliana reveals ABA and JA as major regulators. Scientific Reports 7, 4017. [Europe PMC free article] [Abstract] [Google Scholar]
- Hardenburg RE, Watada AE, Wang CY. 1986. The commercial storage of fruits, vegetables, and florist and nursery stocks. Agriculture Handbook, 66. Washington, DC: USDA. [Google Scholar]
- Hörtensteiner S. 2006. Chlorophyll degradation during senescence. Annual Review of Plant Biology 57, 55–77. [Abstract] [Google Scholar]
- Hua J, Meyerowitz EM. 1998. Ethylene responses are negatively regulated by a receptor gene family in Arabidopsis thaliana. Cell 94, 261–271. [Abstract] [Google Scholar]
- Iglesias DJ, Cercós M, Colmenero-Flores JM, et al. . 2007. Physiology of citrus fruiting. Brazilian Journal of Plant Physiology 19, 333–362. [Google Scholar]
- Jacob-Wilk D, Holland D, Goldschmidt EE, Riov J, Eyal Y. 1999. Chlorophyll breakdown by chlorophyllase: isolation and functional expression of the Chlase1 gene from ethylene-treated Citrus fruit and its regulation during development. The Plant Journal 20, 653–661. [Abstract] [Google Scholar]
- Jiang H, Chen Y, Li M, Xu X, Wu G. 2011. Overexpression of SGR results in oxidative stress and lesion-mimic cell death in rice seedlings. Journal of Integrative Plant Biology 53, 375–387. [Abstract] [Google Scholar]
- Jiang Y, Joyce DC, Macnish AJ. 1999. Extension of the shelf life of banana fruit by 1-methylcyclopropene in combination with polyethylene bags. Postharvest Biology and Technology 16, 187–193. [Google Scholar]
- Jiménez-Cuesta M, Cuquerella J, Martinez-Jávega JM. 1981. Determination of a color index for citrus fruit degreening. Proceedings of the International Society of Citriculture 2, 750–753. [Google Scholar]
- Jin WW, Xu CJ, Li X, Zhang B, Wang P, Allan AC, Chen KS. 2009. Expression of ROP/RAC GTPase genes in postharvest loquat fruit in association with senescence and cold regulated lignification. Postharvest Biology and Technology 54, 9–14. [Google Scholar]
- Jomori MLL, Kluge RA, Jacomino AP. 2003. Cold storage of ‘Tahiti’ lime treated with 1-methylcyclopropene. Scientia Agricola 60, 785–788. [Google Scholar]
- Kamiyoshihara Y, Tieman DM, Huber DJ, Klee HJ. 2012. Ligand-induced alterations in the phosphorylation state of ethylene receptors in tomato fruit. Plant Physiology 160, 488–497. [Abstract] [Google Scholar]
- Kato M. 2012. Mechanism of carotenoid accumulation in citrus fruit. Journal of the Japanese Society for Horticultural Science 81, 219–233. [Google Scholar]
- Kato M, Ikoma Y, Matsumoto H, Sugiura M, Hyodo H, Yano M. 2004. Accumulation of carotenoids and expression of carotenoid biosynthetic genes during maturation in citrus fruit. Plant Physiology 134, 824–837. [Abstract] [Google Scholar]
- Kato M, Matsumoto H, Ikoma Y, Okuda H, Yano M. 2006. The role of carotenoid cleavage dioxygenases in the regulation of carotenoid profiles during maturation in citrus fruit. Journal of Experimental Botany 57, 2153–2164. [Abstract] [Google Scholar]
- Katz E, Lagunes PM, Riov J, Weiss D, Goldschmidt EE. 2004. Molecular and physiological evidence suggests the existence of a system II-like pathway of ethylene production in non-climacteric Citrus fruit. Planta 219, 243–252. [Abstract] [Google Scholar]
- Kevany BM, Tieman DM, Taylor MG, Cin VD, Klee HJ. 2007. Ethylene receptor degradation controls the timing of ripening in tomato fruit. The Plant Journal 51, 458–467. [Abstract] [Google Scholar]
- Kim HO, Hewett EW, Lallu N. 1999. The role of ethylene in kiwifruit softening. Acta Horticulturae 498, 255–262. [Google Scholar]
- Langfelder P, Horvath S. 2008. WGCNA: an R package for weighted correlation network analysis. BMC Bioinformatics 9, 559. [Europe PMC free article] [Abstract] [Google Scholar]
- Li L, Lichter A, Chalupowicz D, Gamrasni D, Goldberg T, Nerya O, Ben-Arie R, Porat R. 2016. Effects of the ethylene-action inhibitor 1-methylcyclopropene on postharvest quality of non-climacteric fruit crops. Postharvest Biology and Technology 111, 322–329. [Google Scholar]
- Li SJ, Xie XL, Liu SC, Chen KS, Yin XR. 2019. Auto- and mutual-regulation between two CitERFs contribute to ethylene-induced citrus fruit degreening. Food Chemistry 299, 125163. [Abstract] [Google Scholar]
- Liu L, Wei J, Zhang M, Zhang L, Li C, Wang Q. 2012. Ethylene independent induction of lycopene biosynthesis in tomato fruits by jasmonates. Journal of Experimental Botany 63, 5751–5761. [Europe PMC free article] [Abstract] [Google Scholar]
- Luo F, Cai JH, Kong XM, Zhou Q, Zhou X, Zhao YB, Ji SJ. 2019. Transcriptome profiling reveals the roles of pigment mechanisms in postharvest broccoli yellowing. Horticulture Research 6, 74. [Europe PMC free article] [Abstract] [Google Scholar]
- Manera FJ, Brotons JM, Conesa A, Porras I. 2012. Influence of temperature on the beginning of degreening in lemon peel. Scientia Horticulturae 145, 34–38. [Google Scholar]
- Manera FJ, Brotons JM, Conesa A, Porras I. 2013. Relation between temperature and the beginning of peel color change in grapefruit (Citrus paradisi Macf.). Scientia Horticulturae 160, 292–299. [Google Scholar]
- Matsumoto H, Ikoma Y, Kato M, Kuniga T, Nakajima N, Yoshida T. 2007. Quantification of carotenoids in citrus fruit by LC-MS and comparison of patterns of seasonal changes for carotenoids among citrus varieties. Journal of Agricultural and Food Chemistry 55, 2356–2368. [Abstract] [Google Scholar]
- Matsumoto H, Ikoma Y, Kato M, Nakajima N, Hasegawa Y. 2009. Effect of postharvest temperature and ethylene on carotenoid accumulation in the flavedo and juice sacs of Satsuma mandarin (Citrus unshiu Marc.) fruit. Journal of Agricultural and Food Chemistry 57, 4724–4732. [Abstract] [Google Scholar]
- Mayuoni L, Tietel Z, Patil BS, Porat R. 2011. Does ethylene degreening affect internal quality of citrus fruit? Postharvest Biology and Technology 62, 50–58. [Google Scholar]
- McCollum G, Maul P. 2007. 1-Methylcyclopropene inhibits degreening but stimulates respiration and ethylene biosynthesis in grapefruit. HortScience 42, 120–124. [Google Scholar]
- McGlasson WB, Scott KJ, Mendoza DB Jr. 1979. The refrigerated storage of tropical and subtropical products. International Journal of Refrigeration 2, 199–206. [Google Scholar]
- Mitalo OW, Asiche WO, Kasahara Y, Tosa Y, Owino WO, Mworia EG, Ushijima K, Nakano R, Kubo Y. 2018a Characterization of ripening-related genes involved in ethylene-independent low temperature-modulated ripening in ‘Rainbow Red’ kiwifruit during storage and on-vine. The Horticulture Journal 87, 421–429. [Google Scholar]
- Mitalo OW, Asiche WO, Kasahara Y, Tosa Y, Tokiwa S, Ushijima K, Nakano R, Kubo Y. 2019a Comparative analysis of fruit ripening and associated genes in two kiwifruit cultivars (‘Sanuki Gold’ and ‘Hayward’) at various storage temperatures. Postharvest Biology and Technology 147, 20–28. [Google Scholar]
- Mitalo OW, Tokiwa S, Kasahara Y, et al. . 2018. b Determination of optimum temperature for long-term storage and analysis of ripening-related genes in ‘Rainbow Red’ kiwifruit. Acta Horticulturae 1218, 517–5 24. [Google Scholar]
- Mitalo OW, Tokiwa S, Kondo Y, et al. . 2019. b Low temperature storage stimulates fruit softening and sugar accumulation without ethylene and aroma volatile production in kiwifruit. Frontiers in Plant Science 10, 888. [Europe PMC free article] [Abstract] [Google Scholar]
- Mitalo OW, Tosa Y, Tokiwa S, et al. . 2019. c ‘Passe Crassane’ pear fruit (Pyrus communis L.) ripening: revisiting the role of low temperature via integrated physiological and transcriptome analysis. Postharvest Biology and Technology 158, 110949. [Google Scholar]
- Mworia EG, Yoshikawa T, Salikon N, Oda C, Asiche WO, Yokotani N, Abe D, Ushijima K, Nakano R, Kubo Y. 2012. Low-temperature-modulated fruit ripening is independent of ethylene in ‘Sanuki Gold’ kiwifruit. Journal of Experimental Botany 63, 963–971. [Europe PMC free article] [Abstract] [Google Scholar]
- Nham NT, Macnish AJ, Zakharov F, Mitcham EJ. 2017. ‘Bartlett’ pear fruit (Pyrus communis L.) ripening regulation by low temperatures involves genes associated with jasmonic acid, cold response, and transcription factors. Plant Science 260, 8–18. [Abstract] [Google Scholar]
- Oda-Yamamizo C, Mitsuda N, Sakamoto S, Ogawa D, Ohme-Takagi M, Ohmiya A. 2016. The NAC transcription factor ANAC046 is a positive regulator of chlorophyll degradation and senescence in Arabidopsis leaves. Scientific Reports 6, 23609. [Europe PMC free article] [Abstract] [Google Scholar]
- Ohmiya A, Kato M, Shimada T, Nashima K, Kishimoto S, Nagata M. 2019. Molecular basis of carotenoid accumulation in horticultural crops. The Horticulture Journal 88, 135–149. [Google Scholar]
- Peng G, Xie XL, Jiang Q, Song S, Xu CJ. 2013. Chlorophyll a/b binding protein plays a key role in natural and ethylene-induced degreening of Ponkan (Citrus reticulata Blanco). Scientia Horticulturae 160, 37–43. [Google Scholar]
- Porat R. 2008. Degreening of citrus fruit. Tree and Forestry Science and Biotechnology 2, 71–76. [Google Scholar]
- Purvis AC, Barmore CR. 1981. Involvement of ethylene in chlorophyll degradation in peel of citrus fruits. Plant Physiology 68, 854–856. [Abstract] [Google Scholar]
- Qiu K, Li Z, Yang Z, et al. . 2015. EIN3 and ORE1 accelerate degreening during ethylene-mediated leaf senescence by directly activating chlorophyll catabolic genes in Arabidopsis. PLoS Genetics 11, e1005399. [Europe PMC free article] [Abstract] [Google Scholar]
- Ríos G, Naranjo MA, Rodrigo MJ, Alós E, Zacarías L, Cercós M, Talón M. 2010. Identification of a GCC transcription factor responding to fruit colour change events in citrus through the transcriptomic analyses of two mutants. BMC Plant Biology 10, 276. [Europe PMC free article] [Abstract] [Google Scholar]
- Robinson MD, McCarthy DJ, Smyth GK. 2010. edgeR: a Bioconductor package for differential expression analysis of digital gene expression data. Bioinformatics 26, 139–140. [Europe PMC free article] [Abstract] [Google Scholar]
- Rodrigo MJ, Alquézar B, Alós E, Lado J, Zacarías L. 2013. Biochemical bases and molecular regulation of pigmentation in the peel of Citrus fruit. Scientia Horticulturae 163, 46–62. [Google Scholar]
- Rodrigo MJ, Alquezar B, Zacarías L. 2006. Cloning and characterization of two 9-cis-epoxycarotenoid dioxygenase genes, differentially regulated during fruit maturation and under stress conditions, from orange (Citrus sinensis L. Osbeck). Journal of Experimental Botany 57, 633–643. [Abstract] [Google Scholar]
- Rodrigo MJ, Marcos JF, Alférez F, Mallent MD, Zacarías L. 2003. Characterization of Pinalate, a novel Citrus sinensis mutant with a fruit-specific alteration that results in yellow pigmentation and decreased ABA content. Journal of Experimental Botany 54, 727–738. [Abstract] [Google Scholar]
- Rodrigo MJ, Zacarias L. 2007. Effect of postharvest ethylene treatment on carotenoid accumulation and the expression of carotenoid biosynthetic genes in the flavedo of orange (Citrus sinensis L. Osbeck) fruit. Postharvest Biology and Technology 43, 14–22. [Google Scholar]
- Sakuraba Y, Schelbert S, Park SY, Han SH, Lee BD, Andrès CB, Kessler F, Hörtensteiner S, Paek NC. 2012. STAY-GREEN and chlorophyll catabolic enzymes interact at light-harvesting complex II for chlorophyll detoxification during leaf senescence in Arabidopsis. The Plant Cell 24, 507–518. [Abstract] [Google Scholar]
- Sawamura M. 1981. Levels of endogenous ethylene in attached citrus fruits. Agricultural and Biological Chemistry 45, 2935–2937. [Google Scholar]
- Schelbert S, Aubry S, Burla B, Agne B, Kessler F, Krupinska K, Hörtensteiner S. 2009. Pheophytin pheophorbide hydrolase (pheophytinase) is involved in chlorophyll breakdown during leaf senescence in Arabidopsis. The Plant Cell 21, 767–785. [Abstract] [Google Scholar]
- Seymour GB, Granell A. 2014. Fruit development and ripening. Journal of Experimental Botany 65, 4489–4490. [Europe PMC free article] [Abstract] [Google Scholar]
- Shemer TA, Harpaz-Saad S, Belausov E, Lovat N, Krokhin O, Spicer V, Standing KG, Goldschmidt EE, Eyal Y. 2008. Citrus chlorophyllase dynamics at ethylene-induced fruit color-break: a study of chlorophyllase expression, posttranslational processing kinetics and in situ intracellular localization. Plant Physiology 148, 108–118. [Abstract] [Google Scholar]
- Shimoda Y, Ito H, Tanaka A. 2016. Arabidopsis STAY-GREEN, Mendel’s green cotyledon gene, encodes magnesium-dechelatase. The Plant Cell 28, 2147–2160. [Abstract] [Google Scholar]
- Sisler EC, Serek M. 1997. Inhibitors of ethylene responses in plants at the receptor level: recent developments. Physiologia Plantarum 100, 577–582. [Google Scholar]
- Song Y, Yang C, Gao S, Zhang W, Li L, Kuai B. 2014. Age-triggered and dark-induced leaf senescence require the bHLH transcription factors PIF3, 4, and 5. Molecular Plant 7, 1776–1787. [Europe PMC free article] [Abstract] [Google Scholar]
- Tacken E, Ireland H, Gunaseelan K, et al. . 2010. The role of ethylene and cold temperature in the regulation of the apple POLYGALACTURONASE1 gene and fruit softening. Plant Physiology 153, 294–305. [Abstract] [Google Scholar]
- Tao N, Wang C, Xu J, Cheng Y. 2012. Carotenoid accumulation in postharvest “Cara Cara” navel orange (Citrus sinensis Osbeck) fruits stored at different temperatures was transcriptionally regulated in a tissue-dependent manner. Plant Cell Reports 31, 1667–1676. [Abstract] [Google Scholar]
- Tian T, Liu Y, Yan H, You Q, Yi X, Du Z, Xu W, Su Z. 2017. agriGO v2.0: a GO analysis toolkit for the agricultural community, 2017 update. Nucleic Acids Research 45, W122–W129. [Europe PMC free article] [Abstract] [Google Scholar]
- Van Wyk AA, Huysamer M, Barry GH. 2009. Extended low-temperature shipping adversely affects rind colour of ‘Palmer Navel’ sweet orange [Citrus sinensis (L.) Osb.] due to carotenoid degradation but can partially be mitigated by optimising post-shipping holding temperature. Postharvest Biology and Technology 53, 109–116. [Google Scholar]
- Wang X, Yin W, Wu J, Chai L, Yi H. 2016. Effects of exogenous abscisic acid on the expression of citrus fruit ripening-related genes and fruit ripening. Scientia Horticulturae 201, 175–183. [Google Scholar]
- Watkins CB. 2006. The use of 1-methylcyclopropene (1-MCP) on fruits and vegetables. Biotechnology Advances 24, 389–409. [Abstract] [Google Scholar]
- Wellburn AR. 1994. The spectral determination of chlorophylls a and b, as well as total carotenoids, using various solvents with spectrophotometers of different resolution. Journal of Plant Physiology 144, 307–313. [Google Scholar]
- Wu GA, Prochnik S, Jenkins J, et al. . 2014. Sequencing of diverse mandarin, pummelo and orange genomes reveals complex history of admixture during citrus domestication. Nature Biotechnology 32, 656–662. [Europe PMC free article] [Abstract] [Google Scholar]
- Yin XR, Xie XL, Xia XJ, Yu JQ, Ferguson IB, Giovannoni JJ, Chen KS. 2016. Involvement of an ethylene response factor in chlorophyll degradation during citrus fruit degreening. The Plant Journal 86, 403–412. [Abstract] [Google Scholar]
- Yun Z, Jin S, Ding Y, Wang Z, Gao H, Pan Z, Xu J, Cheng Y, Deng X. 2012. Comparative transcriptomics and proteomics analysis of citrus fruit, to improve understanding of the effect of low temperature on maintaining fruit quality during lengthy post-harvest storage. Journal of Experimental Botany 63, 2873–2893. [Europe PMC free article] [Abstract] [Google Scholar]
- Zhang B, Horvath S. 2005. A general framework for weighted gene co-expression network analysis. Statistical Applications in Genetics and Molecular Biology 4, 10.2202/1544-6115.1128. [Abstract] [Google Scholar]
- Zhang Y, Bouwmeester HJ, Kappers IF. 2020. Combined transcriptome and metabolome analysis identifies defence responses in spider mite-infested pepper (Capsicum annuum). Journal of Experimental Botany 71, 330–343. [Europe PMC free article] [Abstract] [Google Scholar]
- Zhang Y, Liu Z, Chen Y, He JX, Bi Y. 2015. PHYTOCHROME-INTERACTING FACTOR 5 (PIF5) positively regulates dark-induced senescence and chlorophyll degradation in Arabidopsis. Plant Science 237, 57–68. [Abstract] [Google Scholar]
- Zhang YJ, Wang XJ, Wu JX, Chen SY, Chen H, Chai LJ, Yi HL. 2014. Comparative transcriptome analyses between a spontaneous late-ripening sweet orange mutant and its wild type suggest the functions of ABA, sucrose and JA during citrus fruit ripening. PLoS ONE 9, e116056. [Europe PMC free article] [Abstract] [Google Scholar]
- Zhu A, Li W, Ye J, Sun X, Ding Y, Cheng Y, Deng X. 2011. Microarray expression profiling of postharvest Ponkan mandarin (Citrus reticulata) fruit under cold storage reveals regulatory gene candidates and implications on soluble sugars metabolism. Journal of Integrative Plant Biology 53, 358–374. [Abstract] [Google Scholar]
Articles from Journal of Experimental Botany are provided here courtesy of Oxford University Press
Full text links
Read article at publisher's site: https://doi.org/10.1093/jxb/eraa206
Read article for free, from open access legal sources, via Unpaywall:
https://academic.oup.com/jxb/article-pdf/71/16/4778/33573305/eraa206.pdf
Citations & impact
Impact metrics
Citations of article over time
Alternative metrics
Smart citations by scite.ai
Explore citation contexts and check if this article has been
supported or disputed.
https://scite.ai/reports/10.1093/jxb/eraa206
Article citations
Comparative analysis of the PAL gene family in nine citruses provides new insights into the stress resistance mechanism of Citrus species.
BMC Genomics, 25(1):1020, 31 Oct 2024
Cited by: 0 articles | PMID: 39482587 | PMCID: PMC11526608
The underlying molecular mechanisms of hormonal regulation of fruit color in fruit-bearing plants.
Plant Mol Biol, 114(5):104, 24 Sep 2024
Cited by: 0 articles | PMID: 39316226
Review
Postharvest Quality of Citrus medica L. (cv Liscia-Diamante) Fruit Stored at Different Temperatures: Volatile Profile and Antimicrobial Activity of Essential Oils.
Foods, 13(11):1596, 21 May 2024
Cited by: 0 articles | PMID: 38890825 | PMCID: PMC11171597
Transcriptomic analysis in tomato fruit reveals divergences in genes involved in cold stress response and fruit ripening.
Front Plant Sci, 14:1227349, 28 Jul 2023
Cited by: 2 articles | PMID: 37575935 | PMCID: PMC10416649
Ethylene Response Factor MaERF012 Modulates Fruit Ripening by Regulating Chlorophyll Degradation and Softening in Banana.
Foods, 11(23):3882, 01 Dec 2022
Cited by: 1 article | PMID: 36496689 | PMCID: PMC9738063
Go to all (9) article citations
Data
Data behind the article
This data has been text mined from the article, or deposited into data resources.
BioStudies: supplemental material and supporting data
Similar Articles
To arrive at the top five similar articles we use a word-weighted algorithm to compare words from the Title and Abstract of each citation.
Examining the Role of Low Temperature in Satsuma Mandarin Fruit Peel Degreening via Comparative Physiological and Transcriptomic Analysis.
Front Plant Sci, 13:918226, 13 Jul 2022
Cited by: 3 articles | PMID: 35909736 | PMCID: PMC9328020
Altered sensitivity to ethylene in 'Tardivo', a late-ripening mutant of Clementine mandarin.
Physiol Plant, 151(4):507-521, 29 Dec 2013
Cited by: 5 articles | PMID: 24372483
Involvement of an ethylene response factor in chlorophyll degradation during citrus fruit degreening.
Plant J, 86(5):403-412, 01 Jun 2016
Cited by: 64 articles | PMID: 27037684
Effects of 1-methylcyclopropene (1-MCP) on the expression of genes involved in the chlorophyll degradation pathway of apple fruit during storage.
Food Chem, 308:125707, 19 Oct 2019
Cited by: 13 articles | PMID: 31669943
Funding
Funders who supported this work.
Grant-in-Aid for Scientific Research (3)
Grant ID: 24380023
Grant ID: 20H02977
Grant ID: 16H04873