Abstract
Free full text

Investigating SARS-CoV-2 persistent contamination in different indoor environments
Associated Data
Abstract
Environmental contamination caused by COVID-19 patients could be a medium of transmission. Previous reports of SARS-CoV-2 in environmental surfaces were about short-term contamination. This study investigated SARS-CoV-2 RNA existence in room-temperature and low-temperature environments long after exposure (>28 days). A department store, where a COVID-19 outbreak was occurred in January 2020 (the epicenter of 43 COVID-19 patients), and a patient's apartment were included as room-temperature environments after being blocked for 57 days and 48 days, respectively. Seven cold storages and imported frozen foods inside were included as low-temperature environments (under −18 °C). Twenty food markets with potential contamination of imported frozen foods were also included to study the consecutive contamination. Information about temperature, relative humidity, and the number of days of environmental samples since the last exposure was collected and analyzed. In sum, 11,808 swab samples were collected before disinfection, of which 35 samples were positive. Persistent contamination of SARS-CoV-2 RNA was identified in the apartment (6/19), the department store (3/50), food packages in cold storages (23/1360), environmental surfaces of cold storages (2/345), and a package in the food market (1/10,034). Two positive samples were isolated from the bathroom of the apartment (66.7 %, 2/3), and doorknobs were proved with contamination in the apartment (40 %, 2/5) and cold storage (33.3 %, 1/3). The epidemiology information and environmental contamination results of an imported frozen food related COVID-19 case (138th COVID-19 patient in Tianjin) were analyzed. Based on the Ct values, the number of copies of two target genes was calculated by standard curves and linear regressions. In conclusion, SARS-CoV-2 RNA can be detected in room-temperature environments at least 57 days after the last exposure, much longer than previous reports. Based on the results of this study and previous studies, infectious SARS-CoV-2 could exist for at least 60 days on the surface of cold-chain food packages. Doorknobs and toilets (bathrooms) were important positions in COVID-19 control. High-risk populations of cold-chain-related logistic operations, such as porters, require strict prevention and high-level personal protection.
1. Introduction
Coronavirus disease 2019 (COVID-19), which is caused by severe acute respiratory syndrome coronavirus 2 (SARS-CoV-2), spreads rapidly around the world (Chen et al., 2020). By July 1, 2021, more than 180 million COVID-19 cases and more than 3.9 million deaths were reported (WHO, 2021).
Several studies reported the presence of SARS-CoV-2 genetic materials on surfaces of COVID-19 hospital wards, indicating environmental contamination (Biryukov et al., 2020; Li et al., 2020; Razzini et al., 2020; Ryu et al., 2020; Zhou et al., 2020a, 2020b). These results proved that both clinical and asymptomatic COVID-19 patients might contaminate their surroundings (Wei et al., 2020). However, other than the hospital environment, most of the COVID-19 cases were infected in the community (Ebrahim et al., 2020). In COVID-19 wards or other hospital areas, the healthcare workers were well trained and with adequate personal protection equipment (Cameli et al., 2020). The medical environments were also under the high frequency of disinfection and with advanced ventilation. These could minimize the possibility of infection and transmission (Aghalari et al., 2021; Morawska et al., 2020). While in non-medical environments, both equipment and sanitation were deficient. Though there were several studies about COVID-19 community transmission (Cai et al., 2020; Xie et al., 2020), the results of non-medical environment samples were mainly about wastewater and air transmission (Scott et al., 2021; Sodiq et al., 2021). Environmental contaminations, such as inanimate surfaces, were mentioned in several studies (Abrahao et al., 2021; Carraturo et al., 2020; Dargahi et al., 2021; Wiktorczyk-Kapischke et al., 2021). However, the persistent contamination (>28 days) was not fully elucidated, especially in low-temperature environments.
Reverse transcriptase-polymerase chain reaction (RT-PCR) testing was the most common and powerful tool for SARS-CoV-2 detection in clinical and environmental samples. As the culture of viable and infectious SARS-CoV-2 should be applied in biological safety protection third-level laboratory (BSL-3) or above, the RT-PCR result was the most important contamination index in many countries. However, there was no data about the persistent existence of SARS-CoV-2 RNA in different environments.
In Tianjin, China, 43 COVID-19 patients were infected in the Baodi department store, which was the main epicenter in the city (Jiang et al., 2020). These cases were about 29.3 % (43/147) of all cases identified domestically. After the outbreak, the store was blocked and unoccupied for 57 days. Another study site in this study was a patient's apartment. A COVID-19 patient was living in this apartment, and the whole family traveled to Inner Mongolia, China (stayed for 48 days) before this patient being diagnosed. Then he was confirmed as a COVID-19 patient and died in Inner Mongolia, which means the apartment was blocked and unoccupied for 48 days since the last presence of the patient.
In Nov 2020, an imported frozen food related porter was diagnosed as a 138th COVID-19 patient in Tianjin, China (Group et al., 2020). Before this case, there was no case reported in Tianjin for more than 143 days (from June 17, 2020, to November 8, 2020) (Group and Zhang, 2020). This case and a series of cases that occurred successively were associated with imported frozen food contaminated in a foreign country and sealed in a refrigerated container. The container was transported to Tianjin Port, and then the contaminated cargos were transferred to the cold storage where this porter worked. From the food sealed in the container in Canada to the infection that happened, the entire time length was more than 60 days. As an international port city, many frozen foods from endemic countries were stored in several cold storages in Tianjin.
These sites above accidently offered us opportunities to study the persistent existence of SARS-CoV-2 (RNA level or viable virus level) in room-temperature and low-temperature environments long after exposure (>28 days). At the same time, the distribution and main influencing factors of SARS-CoV-2 persistent contamination in different environments were analyzed. Contamination risk of international frozen foods transportation and disinfection experience of frozen food in China was introduced in this study for global pandemic mitigation efforts.
2. Materials and methods
2.1. Description of sampled environments
This study was conducted from March 11 to December 16, 2020, in Tianjin City. Based on indoor air temperature, study sites in this article could be classified into two categories: room-temperature environments and low-temperature environments (under −18 °C). The Baodi department store and a patient's apartment were included in this study as room-temperature environments. Seven cold storages and the frozen foods inside were included as low-temperature environments. Twenty food markets with potential contamination of imported frozen foods were also included to study the consecutive contamination. The COVID-19 patients mentioned in this study were diagnosed according to the Chinese management guidelines for COVID-19 (Version 7.0), as described in previous studies (Tsang et al., 2020; Zhou et al., 2020b). As mentioned in the introduction section, the department store and the patient's apartment were evacuated after the last presence of diagnosed patients for 57 days (January 27 - March 23, 2020) and 48 days (January 24 - March 11, 2020), respectively. The refrigerated containers were sealed and shipped from foreign countries. After arriving at Tianjin Port, the frozen foods in containers were moved to cold storage. From the eight batches of frozen food loading into containers in the exporting countries to sampling in Tianjin, the time intervals were 45 days at least to 179 days at most.
2.2. Environmental sampling
In this study, a total of 11,808 samples were collected from environments with potential persistent contamination of SARS-CoV-2 before disinfection. Environmental samples were collected from inanimate surfaces in these indoor areas by swabbing each item using sterile swabs premoistened with a viral transport medium (Youkang MT0301; Beijing, China) and deposited into 3.5 mL of the viral transport medium. The standard sampling area was 10 × 10 cm2 (swab applied in horizontal followed by vertical and diagonal sweeps). Small and irregular objects, such as doorknobs and bracelets, were swabbed the whole surface.
In the kitchen, sitting room, bedroom, and bathroom of the patient's apartment, 19 frequently touched surfaces were sampled (Fig. 1 ). According to our previous epidemiology investigation (Jiang et al., 2020), COVID-19 patients mainly appeared on the department store's first floor. Fifty samples were collected from environmental surfaces of three floors and toilets outside the main building, based on the track of patients (Fig. 2 ).
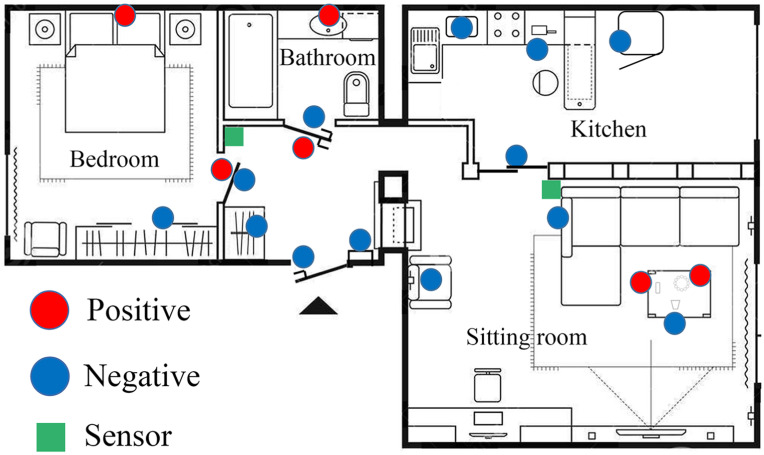
Environmental sampling sites and RT-PCR results in patient's apartment.
An illustration shows the patient's apartment, which was blocked and sampled 48 days after exposure. The circles in the figure show the RT-PCR results of environmental samples collected before disinfection. The red circles indicate positivity for both two SARS-CoV-2 target genes (= positive). The blue circles indicate negativity. (For interpretation of the references to color in this figure legend, the reader is referred to the Web version of this article.)

Environmental sampling sites, RT-PCR results, and patients’ exposure range in Baodi Department Store.
An illustration shows the structure and distribution of positive/negative environmental samples in the Baodi department. The circles in the figure show the RT-PCR results of environmental samples collected before disinfection. The red circles indicate positivity for both two SARS-CoV-2 target genes (= positive). The blue circles indicate negativity. The yellow background indicates patients' exposure during COVID transmission. (For interpretation of the references to color in this figure legend, the reader is referred to the Web version of this article.)
For refrigerated containers and cold storages, environmental surfaces with the potential of contamination, such as panel, floor, doorknob, the internal surface of refrigerator and freezer, were sampled. All the food packages in seven cold storages, such as beef, pork, and seafood, were sampled. According to the fact that some frozen foods were imported before June, and without strict screening and disinfection, samples of the frozen foods related surfaces in twenty food markets were also collected in this study.
After surface swabs were collected, all samples were transferred to the BSL-2 laboratory under cool conditions (2–8 °C) within 2 h for further processing.
2.3. Laboratory analysis
The method used in this study was one-step real-time reverse transcription-polymerase chain reactions (RT-PCR). Samples collected were under RT-PCR testing for SARS-CoV-2 after preprocessing. According to the manufacturer's instructions, viral RNA was extracted from environmental swab samples using a GeneRotex Nucleic Acid Extraction System (Tianlong, Xian, China). The automatic RNA extraction was performed with a 200 μL transport medium, then about 70 μL RNA extraction was obtained for further testing.
RT-PCR was performed using a commercial kit approved by the China Food and Drug Administration specific for SARS-CoV-2 detection (BioGerm Medical Biotechnology Co, Ltd, Shanghai, China) on the CFX96 Touch RealTime PCR Detection System (Bio-Rad, Hercules, CA) following the manufacturer's instructions. The tests were aimed at confirming or excluding the presence of the SARS-CoV-2 RNA on particulate matter. The target genes in this study were open reading frame-1 antibodies (ORF1ab) and nucleocapsid protein (N), as previously described (Lai et al., 2020). The sequence of primers and probes used in this study were listed in Supplementary Table 1.
Each reaction contained 20 μl of Ready-to-use Mastermixes and 5 μl of RNA sample (final volume 25 μl). The reaction was run with initial conditions of 50 °C for 10 min (one cycle), 95 °C for 5 min (one cycle), followed by 40 cycles of 95 °C for 10 s, and 55 °C for 40 s, as instructed by the kit manufacture. A cycle threshold (Ct) value of >38 denoted negative findings for SARS-CoV-2, whereas a Ct value of ≤38 denoted positive findings, as described in a previous study (Dang et al., 2020).
2.4. Disinfection, cleaning, and resampling
The disinfection was performed by spraying surfaces of objects and ground using 1000 mg/L chlorine-containing disinfectants with common sprayers. Electronic products were wiped using sanitary wipes containing 75 % alcohol. The cleaning process was performed 30 min after disinfection by wiping surfaces of objects and ground with water and detergent soaking wipes and mobs.
In the patient's apartment and department store, samples were collected for three rounds: before disinfection, after disinfection, and after cleaning procedure. Additionally, samples were collected at the same sites 30 min after the disinfection. Then cleaning procedure was performed, and samples were collected again after 30 min.
2.5. Temperature distribution and period of environments with potential contamination
In this study, information about the temperature and humidity of all the environmental samples was collected. Also, the period (number of days) between the last exposure and sample collection of each site was calculated. Environmental samples were categorized by locations or food classifications. The number of days after the last exposure was estimated by epidemiology information, the dates of department store and apartment were sealed, and the dates of refrigerated containers set sail to China from different countries. Information on temperature and relative humidity in the apartment was recorded by two household temperature/humidity sensors (Aqara, Shenzhen) placed in the sitting room and bedroom (Fig. 1). The temperature and humidity information of cold storages, anterooms, and refrigerated containers was collected from cold-chain surveillance systems. Unfortunately, no air quality information about the department store was recorded from January 27 to March 23, 2020. As the whole street was blocked during the epidemic for 75 days (January 27 - April 10, 2020), indoor information of another building located next to the department store was collected for analysis. This building also has three floors and was without heating and ventilation during the epidemic. As high temperature could inactivate SARS-CoV-2 (Biryukov et al., 2020), the indoor peak air temperature and average air temperature each day were evaluated in this study. Relative humidity information of the study sites above was also collected.
2.6. Preparation of the quantification curve
The linearized plasmid of two target genes with the concentration of 5 × 105 copies/mL was obtained from the RT-PCR kit manufacture. Linear regression analyses were performed with six serial dilutions (5 × 105, 5 × 104, 5 × 103, 5 × 102, 50, 5 copies/mL). The RT-PCR assays were conducted on three consecutive days, and the serial dilutions of ORF1ab and N genes were performed in technical octuplicates for each day, respectively.
2.7. Statistical analysis
To summarize the information about the samples obtained, we used descriptive statistical techniques, as appropriate. Standard curve and linear regression were performed to estimate the SARS-CoV-2 RNA copy numbers of two target genes using SPSS 22.0 (IBM Corp, Armonk, NY, USA), as a previous study described (Queiroz et al., 2021). The independent-samples t-test was performed to compare the number of viral copies of samples collected from different environments using SPSS 22.0.
2.8. Ethics statement
The Institutional Review Board of Tianjin CDC approved the design and protocol of this study, which was conducted following the amended Helsinki Declaration.
3. Results
3.1. Patient's apartment
Before the disinfection procedure, SARS-CoV-2 RNA was detected in six of 19 (31.58 %) environmental samples inside the patient's apartment using real-time RT-PCR. Four samples were obtained from the bedroom, and two (head of the bed and a doorknob) were positive. In the sitting room, six samples were collected, and two (a TV remote control and a bracelet, both on a side table) were contaminated with SARS-CoV-2 RNA. Three environmental samples were obtained in the bathroom, and two (a water tap and a doorknob) were positive. No viral RNA was detected in the kitchen of the patient's apartment.
RT-PCR results of three samples were still partially positive after the disinfection procedure. No positive result was reported from samples collected after cleaning. Details of the RT-PCR results in the patient's apartment are listed in Table 1 . The distribution of all the samples in the apartment is presented in Fig. 1.
Table 1
RT-PCR results of environmental samples in patient's apartment and department store (before and after disinfection).
Sample Sites | Location (Positive/Total) | Results of two target gene | ||||
---|---|---|---|---|---|---|
Before disinfection | After disinfection | |||||
N gene | ORF1ab | N gene | ORF1ab | |||
Patient's apartment (6/19) | Sitting Room (2/8) | Entrance door knob (inner) | ||||
Video door phone | ||||||
Shoe cabinet | ||||||
Remote control | + | + | + | + | ||
Bracelets | + | + | ||||
Cup | ||||||
Sofa | ||||||
Chair | ||||||
Kitchen (0/4) | Knife | |||||
Chopping board | ||||||
Kitchen sliding door knob | ||||||
Refrigerator inside | ||||||
Bedroom (2/4) | Bedroom door knob (inner) | + | + | |||
Bedroom door knob (outer) | ||||||
Bed headboard | + | + | + | |||
Closet | ||||||
Bathroom (2/3) | Bathroom door knob (inner) | |||||
Bathroom door knob (outer) | + | + | ||||
Bathroom water tap | + | + | ||||
Department store (3/50) | First floor, Shoes area 1 (2/8) | Surface of shoe box | + | + | + | + |
Water cup | + | + | + | |||
First floor, Shoes area 2 (1/6) | Desk | + | + | + | ||
First floor, Clothe area (0/12) | ||||||
First floor, Office (0/6) | ||||||
Second floor, Child area (0/5) | ||||||
Third floor, Office (0/6) | ||||||
Toilets outside the building (0/7) |
3.2. Baodi department store
Before the disinfection procedure, three of 50 (6.0 %) environmental samples inside the department store were proved positive for both of the two target genes by RT-PCR. These three samples were collected from a shoebox, a desk and a water cup in the first-floor footwear zone which was the central area of exposure by COVID-19 patients during the outbreak.
Samples collected from other areas of the department store, such as office zone, shopping zones, and toilets, were all negative in RT-PCR testing. After the disinfection procedure, the RT-PCR results of the two samples were still partially positive. No positive result was reported from samples collected after cleaning. Details of the real-time RT-PCR results of the department store are listed in Table 1. The distribution of all the sample locations in the department store is visualized in Fig. 2.
3.3. Cold-chain-related samples
A total of 11,739 swab samples were collected, including 6676 cold-chain-related food packages and 5063 environmental samples. Of 1360 samples collected from food packages in seven cold storages, 23 (1.69 %) samples were positive. Of 345 samples collected from environmental surfaces of seven cold storages, two (0.58 %) samples were positive. In twenty food markets, 10,034 samples of food packages and environmental surfaces were collected, one (0.01 %) package of Hairbut (Hippoglossus) from Pakistan was positive. Details are presented in Table 2 .
Table 2
RT-PCR results of cold-chain related food and environmental surface samples.
Classification | Exported Country | Food package | Environmental surface | ||||
---|---|---|---|---|---|---|---|
Positive | Total | Positive | Total | ||||
Cold-chain (25/1705) | Cold storage 1 | Pig's head | Canada | 1 | 45 | 0 | 50 |
Pig's knuckle | Germany | 1 | 56 | 1a | 48 | ||
Cold storage 2 | Albatrossia pectoralis | Russia | 7 | 123 | 1b | 73 | |
Cold storage 3 | Hairtail (Trichiurus haumela) | India | 1 | 190 | 0 | 15 | |
Cold storage 4 | Pork | Argentina | 6 | 200 | 0 | 46 | |
Cold storage 5 | Pig's knuckle | Denmark | 1 | 167 | 0 | 33 | |
Cold storage 6 | Hairtail (Trichiurus haumela) | India | 1 | 511 | 0 | 50 | |
Cold storage 7 | Beef | Argentina | 5 | 68 | 0 | 30 | |
Markets (1/10,034) | 1c | 5316 | 0 | 4718 | |||
In sum | 24 | 6676 | 2 | 5063 |
3.4. Temperature distribution and period of environments with potential contamination
After investigating the imported frozen food associated with SARS-CoV-2, the main process of frozen food international transportation was elucidated. Except for several steps processed outside the cold storage or container, foods and packages were in cold-chain (under −18 °C) most of the time. The main process of frozen food international logistics is visualized in Fig. 3 .
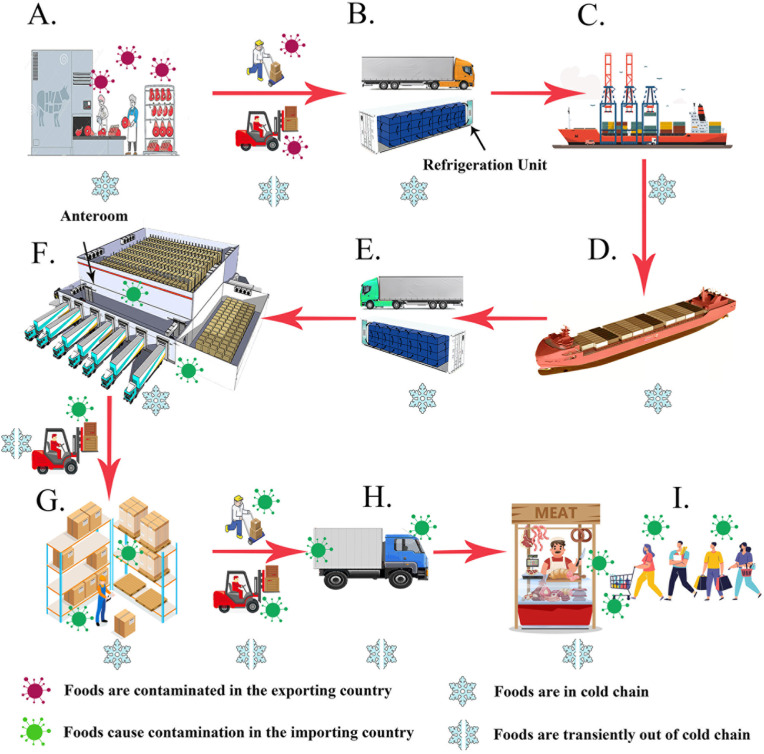
A typical route of frozen food international transportation.
An illustration shows the main process of frozen food international transportation. A. Meat plants in the exporter country; B. Foods are transported to containers in the refrigerated trucks; C. Containers transferred to the container ship; D. The ship arrived in the importing country; E. The container is unloaded to a refrigerated truck. F. Truck arrives at cold storage and has food unloaded in the anteroom; F. Foods are stored in the cold storage; G. Foods are distributed by small refrigerated vehicles. H. The packages are opened, and the meat is distributed by retailers.
As the local trade association demanded, all refrigerated containers in Tianjin set the operating temperature as −20 °C. When the temperature went up to −18 °C, the compressor would start to refrigerate and cool down to −20 °C. Before being stored in cold storages, the frozen foods were firstly carried from containers to the anterooms, which are located outside the cold storage and the ambient temperature was about 0–15 °C. As the anterooms are half-open, the air temperature in anterooms was also affected by the free air temperature outdoors (Fig. 3).
Since high environment temperature tends to cause virus inactivation (Biryukov et al., 2020; Harmooshi et al., 2020), the peak ambient temperatures (every three days) of each study site are presented in Fig. 4 . The average ambient temperature of the department store and the apartment is also presented. The number of total samples and positive samples of each classification is shown in Fig. 4. Relative humidity in cold storages and containers was kept at 85%–95 % to prevent the surface of the meat from drying out. Relative humidity in the department store and the patient's apartment was between 20% and 40 % during the whole period.
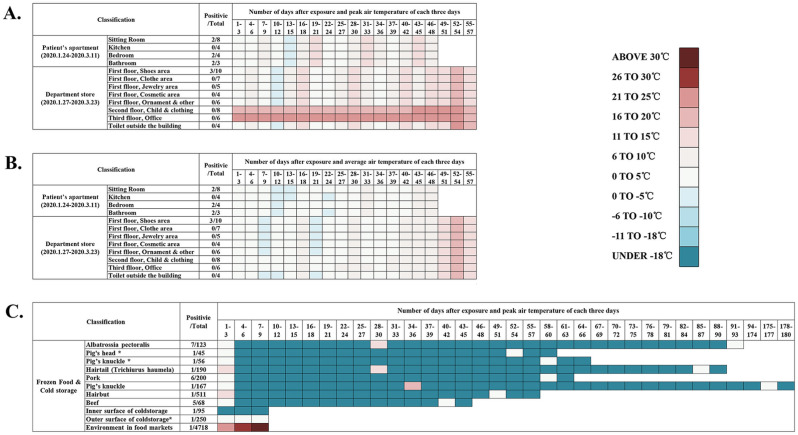
Temperature distribution and period (number of days) of environments with potential contamination by COVID-2019 patients before sample collection.
An illustration shows temperature and period since contamination of all the environmental samples in this study. Environmental samples were categorized by locations or food classifications. The color blocks in the figure show the maximum ambient temperature of each environment every three days. Information about Day 94–174 was shown in one block. The number of total samples and positive samples of each classification was shown in the figure. A. Number of days since last exposure and peak air temperature of each three days of the department store and the apartment. B. Number of days since last exposure and average air temperature of each three days of the department store and the apartment. C. Number of days since last exposure and average air temperature of cold-chain-related food packages and environments. (For interpretation of the references to color in this figure legend, the reader is referred to the Web version of this article.)
3.5. Estimation of viral copy number
Standard curves that could be used to estimate the SARS-CoV-2 RNA copy number was established by plotting the ORF1ab and N gene Ct values of serial dilutions of synthetic RNA on a log/linear scale, followed by linear regression analysis (Fig. 5 ). The results showed that the assay is linear across at least six logs and highly reproducible, enabling Ct values to convert to viral copy numbers. In this study, there were five batches of RT-PCR kits produced by the manufacture, and eight samples were not included in the copy number estimation for not used the same amplification system that establishing the standard curve. The quantification results (copies/mL & copies/cm2) of the 27 samples are shown in Table 3 and Fig. 6 . For samples collected from room-temperature environments, the number of copies of two target genes varied from 2.72 to 61.06 copies/cm2 (ORF1ab) and 1.33–97.06 copies/cm2 (N). For samples collected from low-temperature environments, the number of copies of two target genes was varied from 3.23 to 15786.69 copies/cm2 (ORF1ab) and 1.89–15850.74 copies/cm2 (N). The median number of virus copies estimation of samples collected from the department store, the patient's apartment and food packages in different cold storages were also labeled in Table 3 and Fig. 6.

Quantification curve of ORF1ab and N gene.
An illustration shows the linear regression analyses of 6 serial dilutions (ranging from 5 to 5 × 105 copies per reaction) using linearized plasmid with ORF1ab or N gene region, respectively, tested by RT-PCR for SARS-CoV-2. Ct means cycle threshold. A. Quantification curve and linear regression formulation of ORF1ab by RT-PCR. B. Quantification curve and linear regression formulation of ORF1ab by RT-PCR.
Table 3
Ct values and corresponding SARS-CoV-2 target genes copy number estimation.
Sample Sites | Location (Positive/Total) | Ct value | Copies/mL (Copies/cm2) | Median Copies/mL (Median Copies/cm2) | ||||||||
---|---|---|---|---|---|---|---|---|---|---|---|---|
ORF1ab | N | ORF1ab | N | ORF1ab | N | |||||||
Department store | First floor | Surface of shoe box | 36.55 | 37.91 | 199.90 | (7.00) | 40.99 | (1.44) | 81.95 | (2.87) | 40.99 | (1.44) |
Water cup | 37.98 | 37.80 | 77.73 | (2.72) | 44.91 | (1.57) | ||||||
Desk | 37.90 | 38.00 | 81.95 | (2.87) | 38.03 | (1.33) | ||||||
Patient's apartment | Sitting Room | Remote control | 34.68 | 34.34 | 687.45 | (24.06) | 797.02 | (27.90) | 757.04 | (26.50) | 835.18 | (29.23) |
Bracelets | 34.48 | 32.84 | 784.54 | (27.46) | 2773.23 | (97.06) | ||||||
Bedroom | Bedroom door knob (inner) | 34.59 | 34.23 | 729.55 | (25.54) | 873.33 | (30.57) | |||||
Bed headboard | 33.76 | 34.35 | 1262.28 | (44.18) | 790.42 | (27.66) | ||||||
Bathroom | Bathroom door knob (outer) | 33.27 | 35.89 | 1744.68 | (61.06) | 219.74 | (7.69) | |||||
Bathroom water tap | 35.16 | 33.60 | 500.66 | (17.52) | 1474.41 | (51.60) | ||||||
Cold-chain | Food package | Pig's head | 34.46 | 32.71 | 794.97 | (27.83) | 3089.70 | (108.14) | 872.16 | (30.53) | 1460.64 | (51.12) |
Pig's knuckle | 24.86 | 26.71 | 451,048.20 | (15,786.69) | 452,878.18 | (15,850.74) | ||||||
Hairtail (Trichiurus haumela) | 36.00 | 35.60 | 287.47 | (10.06) | 279.64 | (9.79) | ||||||
Pork 1 | 34.61 | 35.37 | 719.98 | (25.20) | 338.56 | (11.85) | ||||||
Pork 2 | 34.65 | 35.32 | 701.21 | (24.54) | 352.92 | (12.35) | ||||||
Pork 3 | 37.68 | 33.28 | 94.77 | (3.32) | 1923.71 | (67.33) | ||||||
Pork 4 | 31.85 | 30.02 | 4457.17 | (156.00) | 28,909.17 | (1011.82) | ||||||
Pork 5 | 33.02 | 31.53 | 2057.93 | (72.03) | 8239.66 | (288.39) | ||||||
Pork 6 | 35.53 | 31.56 | 392.11 | (13.72) | 8036.72 | (281.28) | ||||||
Pig's knuckle | 37.72 | 37.58 | 92.30 | (3.23) | 53.92 | (1.89) | ||||||
Hairtail (Trichiurus haumela) | 33.70 | 34.80 | 1313.31 | (45.97) | 543.76 | (19.03) | ||||||
Beef 1 | 33.02 | 35.27 | 2057.93 | (72.03) | 367.90 | (12.88) | ||||||
Beef 2 | 31.09 | 32.69 | 7363.33 | (257.72) | 3141.50 | (109.95) | ||||||
Beef 3 | 32.49 | 34.88 | 2920.57 | (102.22) | 508.77 | (17.81) | ||||||
Beef 4 | 34.35 | 34.07 | 854.88 | (29.92) | 997.57 | (34.92) | ||||||
Beef 5 | 34.29 | 32.94 | 889.44 | (31.13) | 2552.02 | (89.32) | ||||||
Environmental surface | Floor under a shelf | 37.38 | 36.65 | 115.54 | (4.04) | 116.82 | (4.09) | 115.54 | (4.04) | 116.82 | (4.09) | |
Markets | Food package | Hairbut (Hippoglossus) | 35.50 | 34.10 | 399.96 | (14.00) | 973.00 | (34.06) | 399.96 | (14.00) | 973.00 | (34.06) |
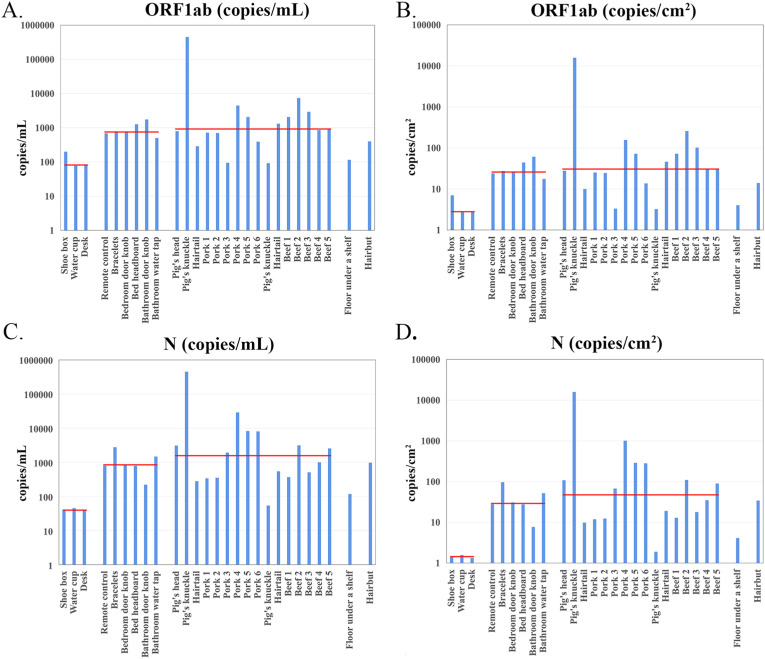
Distributions of the number of copies of two target genes (ORF1ab and N) of 27 positive samples from different study sites.
The number of copies of the samples was quantified using quantification curves. The X-axis shows sample names and the Y-axis shows different ranges of the number of copies (copies/mL or copies/cm2). Red lines in the illustration indicate the median value of samples collected from the department store, the patient's apartment and food packages in cold storage, respectively. A. Histogram of target gene ORF1ab (copies/mL); B. Histogram of target gene ORF1ab (copies/cm2); C. Histogram of target gene N (copies/mL); D. Histogram of target gene N (copies/cm2). (For interpretation of the references to color in this figure legend, the reader is referred to the Web version of this article.)
Of the 17 samples collected from low-temperature environments and ten samples collected from room-temperature environments, there were no significant differences between the mean virus number of two groups for both ORF1ab and N gene (P = 0.316; P = 0.284).
4. Discussions
It has been confirmed that the main transmission routes of COVID-19 are via respiratory droplets and close contact (Chen et al., 2020). In our previous study of the Baodi department store (Jiang et al., 2020) and many other reports (Domingo et al., 2020; Lane et al., 2021; Noorimotlagh et al., 2021), the aerosol transmission of COVID-19 was strongly supported. At the same time, evidence of indirect transmission via environmental contamination was also reported (Abrahao et al., 2021; Cai et al., 2020; Marques and Domingo, 2021). In several studies about the existence of SARS-CoV-2 in non-medical environments (Abrahao et al., 2021; Luo et al., 2020), samples were collected within three days after exposure. To our knowledge, no research was previously reported to evaluate the persistent contamination by SARS-CoV-2 RNA in both room-temperature and low-temperature inanimate surfaces.
In sum, 11,808 swab samples were collected before disinfection in this study. After RT-PCR testing, 35 (0.30 %) swab samples were positive. For room-temperature environments, persistent contamination of SARS-CoV-2 RNA was identified in the apartment (6/19) and the department store (3/50) (Table 1). For cold-chain-related samples, positive samples were collected from food packages in cold storages (23/1360), environmental surfaces of cold storages (2/345), and a package in the food market (1/10,034) (Table 2).
As the apartment was blocked since the patient's whole family left Tianjin (January 24, 2020), the six SARS-CoV-2 RNA positive results of samples collected in the patient's apartment (March 11, 2020) suggest that SARS-CoV-2 RNA were detectable 48 days after the last exposures. The positivity ratio of samples from the patient's apartment was 31.6% (6/19), which is lower than those samples obtained from inhabited environments immediately after contamination, such as COVID-19 quarantine rooms in Qingdao, China (47.8 %) (Hu et al., 2020), COVID-19 ICUs in Chengdu, China (39.3 %) (Wei et al., 2020) and Milan Italy (41.75 %) (Razzini et al., 2020). These pieces of evidence indicate that the RNA contamination may decline over time after the last exposure.
In the department store, all the entrances were blocked during the epidemic. The ventilating and air-conditioning (HVAC) system were also shut down for 57 days before sample collection (January 27 - March 23, 2020). Of the 50 samples collected, there were still three (6.0 %) positive results. A shoebox, a desk and a water cup were still contaminated by SARS-CoV-2 RNA since the last exposure. These environmental contaminations were probably caused by direct touching of patients or the deposition of droplets, which could be a potential transmission pathway in public environments.
Significant contaminations of common household objects by SARS-CoV-2 were reported in the previous studies (Cai et al., 2020; Ong et al., 2020; Santarpia et al., 2020). Two recent studies from Guangzhou, China (Xie et al., 2020) and Minas Gerais, Brazil (Abrahao et al., 2021) tested public environmental surfaces for SARS-CoV-2 RNA and identified several positive results. Doorknobs have been frequently reported with SARS-CoV-2 RNA contamination, both in community and hospital wards (Wei et al., 2020; Xie et al., 2020; Zhang et al., 2020). In this study, doorknobs were also proved with contamination in the patient's apartment (40 %, 2/5) and cold storage (33.3 %, 1/3) (Fig. 1, Fig. 2, Table 1, Table 2). A previous study about the influenza A virus showed that contact of 5 s could transfer 31.6 % of the viral load to the hands (Yen et al., 2015). So contamination of frequent touch surfaces, such as doorknobs in public settings, is a potential source of viral transmission during the pandemic (Bean et al., 1982; Yen et al., 2015).
The stability of viruses in the environment is essential in risk analysis. Live viral culture could confirm the viability and infectiousness of a sample. However, the most common method of SARS-CoV-2 detection in China and many other countries was RT-PCR testing of several fragments in the virus genome. A typical complete genome of SARS-CoV-2 was a 29,903 bp single-strand RNA (Accession Number: NC_045512.2). For the diagnostic detection of SARS-CoV-2, the N gene and ORF1ab were the most common targets (Lai et al., 2020). In the RT-PCR protocol of this study, the target sequence lengths of N and ORF1ab are 99 bp (nt28881-28979) and 119 bp (nt13342-13460), respectively. When SARS-CoV-2 was inactivated, these two fragments were still more likely to keep intact over a period and led to positive results of RT-PCR.
Water and relative humidity are important factors of virus survival (Harmooshi et al., 2020). The viable period of Human coronavirus 229 E (HCoV) in tap water was 10 days at 23 °C and more than 100 days at 4 °C, which was proved by virus culture in a previous study (Gundy et al., 2008). The water cup was the most frequently contaminated site (2/2, 100 %) in another study conducted in China (Liu et al., 2021). In this study, the water cup was also identified with contamination in room-temperature environments (1/2, 50 %), which is understandable because the patient's saliva has a high viral load and water in the cup may also play an important part in virus persistence.
In indoor concealed environments, relative humidity in the department store and the patient's apartment was between 20 and 40 % during the blocked period, a normal indoor relative humidity in the winter of North China. The relative humidity data were obtained from the sitting room and hallway of the apartment (Fig. 1), no information on the kitchen and bathroom were available. Generally speaking, enclosed bathrooms and toilets were locations with high humidity (Zhang et al., 2019). In this study, two positive samples were isolated from the apartment's bathroom (66.7 %, 2/3). In a previous study, toilets played a special role in the COVID-19 transmission, and 23.8 % (10/42) of samples from toilets were RT-PCR positive (Luo et al., 2020). In another study, a traveler with an N95 mask was infected in the toilet of an evacuation flight (Bae et al., 2020). As small and enclosed spaces where mask taking off and face washes could be performed, coupled with special relative humidity and ventilation conditions, toilets and bathrooms were important positions in COVID-19 control and prevention.
The temperature was considered the most influential factor of virus survival in indoor environments (Aboubakr et al., 2020; Auler et al., 2020; Biryukov et al., 2020; Deyab, 2020). The warmer temperature could shorten virus half-life (Aboubakr et al., 2020; Biryukov et al., 2020). When the ambient temperature was above 25 °C, the lifespan of the SARS-CoV-2 will decrease dramatically (Doremalen et al., 2020; Harmooshi et al., 2020). In the apartment and department store, the SARS-CoV-2 RNA stayed positive for 48 days and 57 days, respectively. This time length exceeded the most extended period of SARS-CoV-2 RNA environmental contamination ever reported (28 days, in a medical environment) (Zhou et al., 2020b). Of all the study sites with positive results identified, peak indoor air temperature was below 20 °C during the study period (Fig. 4). Low air temperature in the apartment and department store may delay the degradation of virus RNA. These results indicate that the SARS-CoV-2, in RNA level, could exist with the integrity of both ORF1ab and N gene for at least 57 days in environments temperature under 20 °C.
Besides, ventilation may also play an important role in virus contamination. Most of the ventilation system of non-medical environments was without high-efficiency particulate air (HEPA) filter or all fresh air-conditioning. At the first stage of environmental contamination from COVID-19 patients, the ventilation system could accelerate the spread of SARS-CoV-2 in the indoor building (Azuma et al., 2020; Lu et al., 2020). However, the ventilation system of the patient's apartment and department store was shut down during isolation which could be another essential factor for the long-term persistence of virus RNA. The absence of ventilation may keep the enclosed environment with more stable humidity and temperature for post contamination.
In a previous study, potentially contaminated object surfaces had been disinfected several times before sampling and still had positive RT-PCR results identified (Xie et al., 2020). In this study, RT-PCR positive samples were also identified from environments after disinfection and virus inactivation. It is understandable that two target genes account for only a small portion of the SARS-CoV-2 whole-genome and have a chance to remain intact even after the RNA structure was destroyed. However, after a thorough cleaning with detergent and water, all samples were proved negative. This result was consistent with previous reports that the SARS-CoV-2 RNA in environmental samples would be reduced to undetectable levels after implementing rigorous sanitization procedures (Liu et al., 2020b).
On Nov 8, 2020, a porter in cold storage was confirmed as the 138th COVID-19 patient in Tianjin (Group et al., 2020). After that, nine follow-up cases were reported. In this cold storage, a package of pig's knuckle which was shipped from Germany, a package of pig's head which was shipped from Canada, and a doorknob of the cold storage were proved with positive RT-PCR results. Several reasons could confirm imported frozen foods infected this patient: First, this porter had not left Tianjin City for at least 60 days before infection. Moreover, there was no local COVID-19 infection in Tianjin for 143 days (June 17, 2020, to Nov 8, 2020) (Group et al., 2020). Second, in September 2020, in Qingdao, China, viable SARS-CoV-2 were isolated from the imported frozen food package's surface by virus culture and proved with a European origin by the phylogeny of whole-genome sequences (Liu et al., 2020a). Furthermore, the frozen food contamination also caused porters infected in Qingdao (Liu et al., 2020a) and Shanghai (Fang et al., 2020). Third, in our previous study, the whole genome sequence of the 138th case in Tianjin was proved close to Canadian strains in phylogenetic analysis (Group et al., 2020). Moreover, whole-genome sequences of imported frozen food related COVID-19 cases in Beijing belonged to Branch 1 of L-lineage from Europe in the phylogenetic tree (Tan et al., 2020). Based on the evidence above, the foods were shipped from a foreign country and have been sealed in cold-chain (under −18 °C) for more than 60 days since their last exposure, were confirmed as the source of the 138th case in Tianjin in Nov 2020 (Bai et al., 2021; Group et al., 2020).
Many studies had investigated the viral load of clinical samples from COVID-19 patients (Cheng et al., 2020; Gottlieb et al., 2021). However, the number of virus copies of environmental surface samples was seldom reported. In a previous study, the viral RNA concentrations of an environmental sample related to a COVID-19 patient diagnosed and under medical care for 23 days were 38 copies/cm2 (Feng et al., 2021), which is close to the results of samples collected from the apartment and cold storages in this study (Table 3). In a meta-analysis study, the mean duration of COVID-19 patients’ viral shedding (95 % CI) was 17.0 (15.5–18.6) days. Considering this patient was in the later phase of clinical treatment (23rd day) and the high-frequency disinfection applied in the COVID-19 ward, 38 copies/cm2 could not represent a typical COVID-19 patient viral shedding level.
Based on RT-qPCR, viral load of sputum and throat swab samples collected from COVID-19 patient's upper respiratory tract were detected, varying from negative to 1.34 × 1011 copies/mL (Cheng et al., 2020; Pan et al., 2020). Besides direct touching, coughing, sneezing, and speaking could cause environmental contamination by generating droplets or aerosolizing the respiratory fluid. In a previous study conducted in the laboratory, on a surface with high viral loads contamination, viable SARS-CoV-2 was isolated for up to 28 days at 20 °C from common surfaces (Riddell et al., 2020). Environmental surfaces pose an infection risk if contaminated with a high concentration of SARS-CoV-2 (Paton et al., 2021), especially in low-temperature environments. In this study, the environmental sample related to the 138th COVID-19 case was detected with the highest number of virus copies of all the samples analyzed. The number of virus copies of both ORF1ab (4.51 × 105 copies/mL, 1.58 × 104 copies/cm2) and N gene (4.53 × 105 copies/mL, 1.59 × 104 copies/cm2) was significantly higher than other samples (Fig. 6). The results above indicate that a high viral load environmental contamination may be a necessary condition of secondary infection.
Before symptom onset and receiving medical treatment in the quarantine ward, most COVID-19 patients were still working or living in non-medical environments. Several studies reported viral load peaks during the prodromal phase of illness or at the time of symptom onset (He et al., 2020; Wölfel et al., 2020; Zou et al., 2020). So the contamination in the patient's house, working place, or public areas may have a high concentration of virus copies, emphasizing the importance of environmental disinfection.
It is believed that SARS-CoV-2 decayed more rapidly when the temperature was increased (Biryukov et al., 2020; Riddell et al., 2020). However, no significant differences were identified from low-temperature environments and room-temperature environments samples in the number of copies of both ORF1ab (P = 0.316) and N gene (P = 0.284). As the original contamination level of different study sites in this study was unclear, the RNA degradation rate in different temperatures requires further study.
There were few domestic COVID-19 patients in the community during the study period in China (WHO, 2021), so it is possible to exclude influences from local patients and trace the transmission pathways of imported frozen foods. In this study, 26 RT-PCR positive samples were detected from cold-chain-related surfaces, which indicate that imported frozen foods from COVID-19 pandemic countries shall remain under strict surveillance and disinfection. However, most disinfectants would soon freeze, and disinfection was challenging to perform in temperature under −18 °C.
COVID-19 outbreaks among meat processing workers were reported in North Rhine Westfalia, German (Gunther et al., 2020) and 30 states in the USA (Herstein et al., 2021; Waltenburg et al., 2021). Modern meat processing plants have several environmental conditions, including low temperature, low air exchange rates, and constant air recirculation. The workers engage in heave labor and work with close distance. All these factors promote the efficient aerosol and contact transmission of SARS-CoV-2 particles (Gunther et al., 2020; Middleton et al., 2020).
In low-temperature environments (under −18 °C), the infectious viral particle could survive longer than in room-temperature environments. In the post-pandemic of COVID-19, imported frozen foods played a special role in China. Not only in Tianjin but also in Qingdao (Liu et al., 2020a), Shanghai (Fang et al., 2020) and Beijing (Pang et al., 2020; Tan et al., 2020), imported frozen foods were confirmed as sources of the COVID-19 outbreaks. These results offered evidence of surface to human transmission. By July 1, 2021, there were more than 180 million COVID-19 cases worldwide (WHO, 2021). Due to the increasing number of cases, there would be a consequently increased potential for contamination in the global cold-chain foods industry. The contamination risk in the main process of cold-chain foods transportation and sales are shown in Fig. 3. Furthermore, not only the frozen foods, other cold-chains-related cargos of global logistics operations, such as mRNA COVID-19 vaccine, also require safety protocol for SARS-CoV-2 contamination control and prevention. High-risk populations, such as porters and meat processing plant workers who work in the cold-chain industry, require strict prevention and management.
Since December 2020, the imported frozen foods were under strict supervision in China, and three main disinfection measures were practiced in early 2021. In customs of many cities, the total disinfection of a container was practiced with a hydrogen peroxide disinfector (Fig. 7 A). After that, the foods were processed with a sanitation tunnel machine in the anteroom of cold storage (Fig. 7B). When the food case was opened in the retail section, the inner part of the case would be disinfected with alcohol-based disinfectants (Fig. 7C). Personal protection equipment of imported frozen food related workers in cold storages including goggles, protective suits, N95 mask, and two layers of gloves. As the information on the contamination of each imported frozen food container in the export country was not available, the effectiveness of the disinfection process could not be accurately evaluated in this study. Besides, appropriate ventilation-associated building engineering controls are recommended in meat processing plants and cold storages, including sufficient and effective ventilation, possibly enhanced by particle filtration and air disinfection, avoiding air recirculation, and avoiding overcrowding (Aghalari et al., 2021; Morawska et al., 2020).
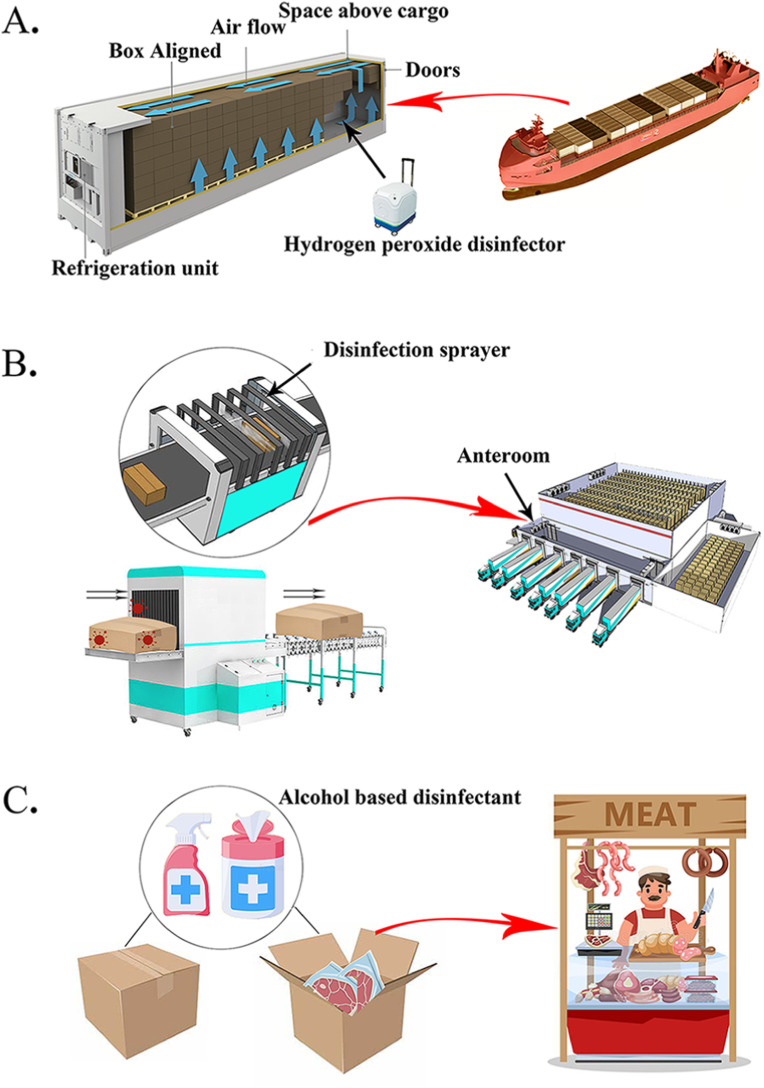
Main disinfection measures of imported frozen foods.
An illustration shows three main disinfection measures of imported frozen food. A. The whole container is disinfected with frozen foods inside of it. A hydrogen peroxide disinfector is used for virus inactivation. In brief, 35 % food-grade hydrogen peroxide solution is vaporized by the disinfector and being released in the container. Then vaporized hydrogen peroxide concentration is maintained at 50–60 ppm in the sealed container for 1 h. B. Before being stocked in the cold storage, a sanitation tunnel machine in the anteroom is used to inactivate surface contamination of frozen food with 200 ppm chlorine dioxide solution. C. The food case is opened by a retailer, and small packages are processed by sprayer and wipes with alcohol-based disinfectant.
This study is the first report on the SARS-CoV-2 persistent contamination in room-temperature and low-temperature environments. Systematical analysis of the contamination in frozen foods, the risk points of contamination during the entire import process of frozen foods, and introduction of the practical experience of China in conducting the disinfection of imported frozen foods are included in this study.
We are aware of the limitations of this study. Although 11,808 samples from different environments were included in this study, the amount only represents a low volume of the whole space of the non-medical environment with potential contamination in Tianjin. Secondly, viral culture, which could test the viability, was not performed in this study. However, a SARS-CoV-2 viral culture result of frozen food in China was offered to support our analysis and conclusions (Liu et al., 2020a). Despite the limitations, we believe that the findings reported here could guide the prevention and control of SARS-CoV-2 environmental contamination.
5. Conclusions
SARS-CoV-2 RNA can be detected by RT-PCR 57 days after the last exposure in room-temperature environments, much longer than previous reports. Doorknobs and toilets (bathrooms) were important positions in COVID-19 control. Infectious SARS-CoV-2 can exist for at least 60 days on the surface of cold-chain food packages (under −18 °C). High-risk populations of cold-chain-related logistic operations, such as porters, require strict prevention and high-level personal protection. Even after disinfection, SARS-CoV-2 RNA can still be partially detected in the environment. Cleaning with water and detergent is an effective way to eliminate the persistent existence of RNA fragments on environmental surfaces.
Funding sources
This research was supported by the Key Technologies R & D program of Tianjin (20ZXGBSY00100); Technologies Program of Tianjin Health Commission (2020XKC02).
Declaration of competing interest
The authors declare that they have no known competing financial interests or personal relationships that could have appeared to influence the work reported in this paper.
Footnotes
Appendix ASupplementary data to this article can be found online at https://doi.org/10.1016/j.envres.2021.111763.
Appendix A. Supplementary data
The following is the Supplementary data to this article:
References
- Aboubakr H.A., Sharafeldin T.A., Goyal S.M. Stability of SARS-CoV-2 and other coronaviruses in the environment and on common touch surfaces and the influence of climatic conditions: a review. Transbound. Emerg. Dis. 2020;68:296–312. [Europe PMC free article] [Abstract] [Google Scholar]
- Abrahao J.S., Sacchetto L., Rezende I.M., Rodrigues R.A.L., Crispim A.P.C., Moura C., Mendonca D.C., Reis E., Souza F., Oliveira G.F.G., Domingos I., de Miranda Boratto P.V., Silva P.H.B., Queiroz V.F., Machado T.B., Andrade L.A.F., Lourenco K.L., Silva T., Oliveira G.P., de Souza Alves V., Alves P.A., Kroon E.G., de Souza Trindade G., Drumond B.P. Detection of SARS-CoV-2 RNA on public surfaces in a densely populated urban area of Brazil: a potential tool for monitoring the circulation of infected patients. Sci. Total Environ. 2021;766 [Europe PMC free article] [Abstract] [Google Scholar]
- Aghalari Z., Dahms H.-U., Sosa-Hernandez J.E., Oyervides-Muñoz M.A., Parra-Saldívar R. Evaluation of SARS-COV-2 transmission through indoor air in hospitals and prevention methods: a systematic review. Environ. Res. 2021;195 [Europe PMC free article] [Abstract] [Google Scholar]
- Auler A.C., Cassaro F.A.M., da Silva V.O., Pires L.F. Evidence that high temperatures and intermediate relative humidity might favor the spread of COVID-19 in tropical climate: a case study for the most affected Brazilian cities. Sci. Total Environ. 2020;729 [Europe PMC free article] [Abstract] [Google Scholar]
- Azuma K., Yanagi U., Kagi N., Kim H., Hayashi M. Environmental factors involved in SARS-CoV-2 transmission: effect and role of indoor environmental quality in the strategy for COVID-19 infection control. Environ. Health Prev. Med. 2020;25:66. [Europe PMC free article] [Abstract] [Google Scholar]
- Bae S., Shin H., Koo H., Lee S., Yang J., Yon D. Asymptomatic transmission of SARS-CoV-2 on evacuation flight. Emerg. Infect. Dis. 2020;26:2705–2708. [Europe PMC free article] [Abstract] [Google Scholar]
- Bai L., Wang Y., Wang Y., Wu Y., Liu Z. Controlling COVID-19 transmission due to contaminated imported frozen food and food packaging. China CDC Weekly. 2021;3:30–33. [Europe PMC free article] [Abstract] [Google Scholar]
- Bean B., Moore B.M., Sterner B., Peterson L.R., Gerding D.N., Balfour H.H. Survival of influenza viruses on environmental surfaces. J. Infect. Dis. 1982;146:47–51. [Abstract] [Google Scholar]
- Biryukov J., Boydston J.A., Dunning R.A., Yeager J.J., Wood S., Reese A.L., Ferris A., Miller D., Weaver W., Zeitouni N.E., Phillips A., Freeburger D., Hooper I., Ratnesar-Shumate S., Yolitz J., Krause M., Williams G., Dawson D.G., Herzog A., Dabisch P., Wahl V., Hevey M.C., Altamura L.A. Increasing temperature and relative humidity accelerates inactivation of SARS-CoV-2 on surfaces. mSphere. 2020;5 e00441–20. [Europe PMC free article] [Abstract] [Google Scholar]
- Cai J., Sun W., Huang J., Gamber M., Wu J., He G. Indirect virus transmission in cluster of COVID-19 cases, Wenzhou, China, 2020. Emerg. Infect. Dis. 2020;26:1343–1345. [Europe PMC free article] [Abstract] [Google Scholar]
- Cameli M., Pastore M.C., Henein M., Aboumarie H.S., Mandoli G.E., D'Ascenzi F., Cameli P., Franchi F., Mondillo S., Valente S. Safe performance of echocardiography during the COVID-19 pandemic: a practical guide. Rev. Cardiovasc. Med. 2020;21:217–223. [Abstract] [Google Scholar]
- Carraturo F., Del Giudice C., Morelli M., Cerullo V., Libralato G., Galdiero E., Guida M. Persistence of SARS-CoV-2 in the environment and COVID-19 transmission risk from environmental matrices and surfaces. Environ. Pollut. 2020;265 [Europe PMC free article] [Abstract] [Google Scholar]
- Chen N., Zhou M., Dong X., Qu J., Gong F., Han Y., Qiu Y., Wang J., Liu Y., Wei Y., Xia J., Yu T., Zhang X., Zhang L. Epidemiological and clinical characteristics of 99 cases of 2019 novel coronavirus pneumonia in Wuhan, China: a descriptive study. Lancet. 2020;395:507–513. [Europe PMC free article] [Abstract] [Google Scholar]
- Cheng V.C., Wong S.C., Chan V.W., So S.Y., Chen J.H., Yip C.C., Chan K.H., Chu H., Chung T.W., Sridhar S., To K.K., Chan J.F., Hung I.F., Ho P.L., Yuen K.Y. Air and environmental sampling for SARS-CoV-2 around hospitalized patients with coronavirus disease 2019 (COVID-19). Infect. Control Hosp. Epidemiology. 2020;41:1258–1265. [Europe PMC free article] [Abstract] [Google Scholar]
- Dang Y., Liu N., Tan C., Feng Y., Yuan X., Fan D., Peng Y., Jin R., Guo Y., Lou J. Comparison of qualitative and quantitative analyses of COVID-19 clinical samples. Clin. Chim. Acta. 2020;510:613–616. [Europe PMC free article] [Abstract] [Google Scholar]
- Dargahi A., Jeddi F., Vosoughi M., Karami C., Hadisi A., Ahamad Mokhtari S., Ghobadi H., Alighadri M., Haghighi S.B., Sadeghi H. Investigation of SARS CoV-2 virus in environmental surface. Environ. Res. 2021;195 [Europe PMC free article] [Abstract] [Google Scholar]
- Deyab M.A. Coronaviruses widespread on nonliving surfaces: important questions and promising answers. Z. Naturforsch. C. J. Biosci. 2020;75:363–367. [Abstract] [Google Scholar]
- Domingo J.L., Marquès M., Rovira J. Influence of airborne transmission of SARS-CoV-2 on COVID-19 pandemic. A review. Environ. Res. 2020;188 [Europe PMC free article] [Abstract] [Google Scholar]
- Doremalen N.V., Bushmaker T., Morris D.H., Holbrook M.G., Munster V.J. Aerosol and surface stability of SARS-CoV-2 as compared with SARS-CoV-1. N. Engl. J. Med. 2020;382:1564–1567. [Europe PMC free article] [Abstract] [Google Scholar]
- Ebrahim S.H., Ahmed Q.A., Gozzer E., Schlagenhauf P., Memish Z.A. Covid-19 and community mitigation strategies in a pandemic. BMJ. 2020;368 [Abstract] [Google Scholar]
- Fang F., Song Y., Hao L., Nie K., Sun X. A case of COVID-19 detected in a cargo worker at Pudong Airport — Shanghai Municipality, China, November 8, 2020. China CDC Weekly. 2020;2:910–911. [Europe PMC free article] [Abstract] [Google Scholar]
- Feng B., Xu K., Gu S., Zheng S., Zou Q., Xu Y., Yu L., Lou F., Yu F., Jin T., Li Y., Sheng J., Yen H.L., Zhong Z., Wei J., Chen Y. Multi-route transmission potential of SARS-CoV-2 in healthcare facilities. J. Hazard Mater. 2021;402 [Europe PMC free article] [Abstract] [Google Scholar]
- Gottlieb R.L., Nirula A., Chen P., Boscia J., Skovronsky D.M. Effect of Bamlanivimab as monotherapy or in combination with Etesevimab on viral load in patients with mild to moderate COVID-19: a randomized clinical trial. J. Am. Med. Assoc. 2021;325:632–644. [Europe PMC free article] [Abstract] [Google Scholar]
- Group L.T., Song Y., Zhao X., Li X., Xu W. A case of COVID-19 — Tianjin municipality, China, November 7, 2020. China CDC Weekly. 2020;3:207–210. [Europe PMC free article] [Abstract] [Google Scholar]
- Group L.T., Zhang Y. The source of infection of the 137th confirmed case of COVID-19 — Tianjin municipality, China, June 17, 2020. China CDC Weekly. 2020;2:507–510. [Europe PMC free article] [Abstract] [Google Scholar]
- Gundy P.M., Gerba C.P., Pepper I.L. Survival of coronaviruses in water and wastewater. Food. Environ. Virol. 2008;1:10–14. [Google Scholar]
- Gunther T., Czech-Sioli M., Indenbirken D., Robitaille A., Tenhaken P., Exner M., Ottinger M., Fischer N., Grundhoff A., Brinkmann M.M. SARS-CoV-2 outbreak investigation in a German meat processing plant. EMBO Mol. Med. 2020;12 [Europe PMC free article] [Abstract] [Google Scholar]
- Harmooshi N.N., Shirbandi K., Rahim F. Environmental concern regarding the effect of humidity and temperature on SARS-COV-2 (COVID-19) survival: fact or fiction. Environ. Sci. Pollut. Res. 2020;27:36027–36036. [Europe PMC free article] [Abstract] [Google Scholar]
- He X., Lau E., Wu P., Deng X., Wang J., Hao X., Lau Y., Wong Y., Guan Y., Tan X. Temporal dynamics in viral shedding and transmissibility of COVID-19. Nat. Med. 2020;26:672–675. [Abstract] [Google Scholar]
- Herstein J.J., Degarege A., Stover D., Austin C., Schwedhelm M.M., Lawler J.V., Lowe J.J., Ramos A.K., Donahue M. Characteristics of SARS-CoV-2 transmission among meat processing workers in Nebraska, USA, and effectiveness of risk mitigation measures. Emerg. Infect. Dis. 2021;27:1032–1038. [Europe PMC free article] [Abstract] [Google Scholar]
- Hu X., Xing Y., Ni W., Zhang F., Lu S., Wang Z., Gao R., Jiang F. Environmental contamination by SARS-CoV-2 of an imported case during incubation period. Sci. Total Environ. 2020;742 [Europe PMC free article] [Abstract] [Google Scholar]
- Jiang G., Wang C., Song L., Wang X., Zhou Y., Fei C., Liu H. Aerosol transmission, an indispensable route of COVID-19 spread: case study of a department-store cluster. Front. Environ. Sci. Eng. 2020;15:46. [Europe PMC free article] [Abstract] [Google Scholar]
- Lai C., Wang C.Y., Ko W.C., Hsueh P.R. In vitro diagnostics of coronavirus disease 2019: Technologies and application. J. Microbiol. Immunol. Infect. 2020;54:164–174. [Europe PMC free article] [Abstract] [Google Scholar]
- Lane M.A., Brownsword E.A., Ahmed B., Ingersoll J.M., Jesse W., Marie A., Matthew K., Uyeki T.M., Lindsley W.G., Kraft C.S. 2021. Clin. Infect. Dis., ciaa1880. [Europe PMC free article] [Abstract] [Google Scholar]
- Li Y.H., Fan Y.Z., Jiang L., Wang H.B. Aerosol and environmental surface monitoring for SARS-CoV-2 RNA in a designated hospital for severe COVID-19 patients. Epidemiol. Infect. 2020;148:e154. [Europe PMC free article] [Abstract] [Google Scholar]
- Liu J., Liu J., He Z., Yang Z., Yuan J., Wu H., Zhu P., Fu X., Lin Y., Zhang Y., Zhao Z., He S., Ma X. Duration of SARS-CoV-2 positive in quarantine room environments: a perspective analysis. Int. J. Infect. Dis. 2021;105:68–74. [Europe PMC free article] [Abstract] [Google Scholar]
- Liu P., Yang M., Zhao X., Guo Y., Wang L., Zhang J., Lei W., Han W., Jiang F., Liu W.J., Gao G.F., Wu G. Cold-chain transportation in the frozen food industry may have caused a recurrence of COVID-19 cases in destination: successful isolation of SARS-CoV-2 virus from the imported frozen cod package surface. Biosaf Health. 2020;2:199–201. [Europe PMC free article] [Abstract] [Google Scholar]
- Liu Y., Ning Z., Chen Y., Guo M., Lan K. Aerodynamic analysis of SARS-CoV-2 in two Wuhan hospitals. Nature. 2020;582:557–561. [Abstract] [Google Scholar]
- Lu J., Gu J., Li K., Xu C., Yang Z. COVID-19 outbreak associated with air conditioning in restaurant, Guangzhou, China, 2020. Emerg. Infect. Dis. 2020;26:1628–1631. [Europe PMC free article] [Abstract] [Google Scholar]
- Luo L., Liu D., Zhang H., Li Z., Zhen R., Zhang X., Xie H., Song W., Liu J., Huang Q., Liu J., Yang X., Chen Z., Mao C. Air and surface contamination in non-health care settings among 641 environmental specimens of 39 COVID-19 cases. PLoS Neglected Trop. Dis. 2020;14 [Europe PMC free article] [Abstract] [Google Scholar]
- Marques M., Domingo J.L. Contamination of inert surfaces by SARS-CoV-2: persistence, stability and infectivity. A review. Environ. Res. 2021;193 [Europe PMC free article] [Abstract] [Google Scholar]
- Middleton J., Reintjes R., Lopes H. Meat plants—a new front line in the covid-19 pandemic. BMJ. 2020;370:m2716. [Abstract] [Google Scholar]
- Morawska L., Tang J.W., Bahnfleth W., Bluyssen P.M., Boerstra A., Buonanno G., Cao J., Dancer S., Floto A., Franchimon F., Haworth C., Hogeling J., Isaxon C., Jimenez J.L., Kurnitski J., Li Y., Loomans M., Marks G., Marr L.C., Mazzarella L., Melikov A.K., Miller S., Milton D.K., Nazaroff W., Nielsen P.V., Noakes C., Peccia J., Querol X., Sekhar C., Seppanen O., Tanabe S.I., Tellier R., Tham K.W., Wargocki P., Wierzbicka A., Yao M. How can airborne transmission of COVID-19 indoors be minimised? Environ. Int. 2020;142 [Europe PMC free article] [Abstract] [Google Scholar]
- Noorimotlagh Z., Jaafarzadeh N., Martínez S., Mirzaee S.A. A systematic review of possible airborne transmission of the COVID-19 virus (SARS-CoV-2) in the indoor air environment. Environ. Res. 2021;193 [Europe PMC free article] [Abstract] [Google Scholar]
- Ong S., Tan Y.K., Chia P.Y., Lee T.H., Ng O.T., Wong M., Marimuthu K. Air, surface environmental, and personal protective equipment contamination by severe acute respiratory syndrome coronavirus 2 (SARS-CoV-2) from a symptomatic patient. J. Am. Med. Assoc. 2020;323:1610–1612. [Europe PMC free article] [Abstract] [Google Scholar]
- Pan Y., Zhang D., Yang P., Poon L., Wang Q. Viral load of SARS-CoV-2 in clinical samples. Lancet Infect. Dis. 2020;20:411–412. [Europe PMC free article] [Abstract] [Google Scholar]
- Pang X., Ren L., Wu S., Ma W., Wang J. Cold-chain food contamination as the possible origin of COVID-19 resurgence in Beijing. Natl. Sci. Rev. 2020;7:1861–1864. [Europe PMC free article] [Abstract] [Google Scholar]
- Paton S., Spencer A., Garratt I., Thompson K.A., Dinesh I., Aranega-Bou P., Stevenson D., Clark S., Dunning J., Bennett A., Pottage T. Persistence of severe acute respiratory syndrome coronavirus 2 (SARS-CoV-2) virus and viral RNA in relation to surface type and contamination concentration. Appl. Environ. Microbiol. 2021;87 [Europe PMC free article] [Abstract] [Google Scholar]
- Queiroz J., Rampazzo R.C.P., Filho E., Oliveira G.S., Oliveira S.D.C., Souza L.F.B., Pereira S.D.S., Rodrigues M.M.S., Maia A.C.S., da Silva C.C., Mendonca A., Lugtenburg C.A.B., Aguiar F.A.A., Rodrigues R.S.S., Santos C.H.N., Guimaraes A., Maximo F.R., Santos A.O.D., Krieger M.A., Salcedo J.M.V., Dall'Acqua D.S.V. Development of a quantitative one-step multiplex RT-qPCR assay for the detection of SARS-CoV-2 in a biological matrix. Int. J. Infect. Dis. 2021;104:373–378. [Europe PMC free article] [Abstract] [Google Scholar]
- Razzini K., Castrica M., Menchetti L., Maggi L., Negroni L., Orfeo N.V., Pizzoccheri A., Stocco M., Muttini S., Balzaretti C.M. SARS-CoV-2 RNA detection in the air and on surfaces in the COVID-19 ward of a hospital in Milan. Italy. Sci. Total Environ. 2020;742 [Europe PMC free article] [Abstract] [Google Scholar]
- Riddell S., Goldie S., Hill A., Eagles D., Drew T.W. The effect of temperature on persistence of SARS-CoV-2 on common surfaces. Virol. J. 2020;17:145. [Europe PMC free article] [Abstract] [Google Scholar]
- Ryu B.H., Cho Y., Cho O.H., Hong S.I., Kim S., Lee S. Environmental contamination of SARS-CoV-2 during the COVID-19 outbreak in South Korea. Am. J. Infect. Contr. 2020;48:875–879. [Europe PMC free article] [Abstract] [Google Scholar]
- Santarpia J.L., Rivera D.N., Herrera V.L., Morwitzer M.J., Lowe J.J. Aerosol and surface contamination of SARS-CoV-2 observed in quarantine and isolation care. Sci. Rep. 2020;10:12732. [Europe PMC free article] [Abstract] [Google Scholar]
- Scott L.C., Aubee A., Babahaji L., Vigil K., Tims S., Aw T.G. Targeted wastewater surveillance of SARS-CoV-2 on a university campus for COVID-19 outbreak detection and mitigation. Environ. Res. 2021;200 [Europe PMC free article] [Abstract] [Google Scholar]
- Sodiq A., Khan M.A., Naas M., Amhamed A. Addressing COVID-19 contagion through the HVAC systems by reviewing indoor airborne nature of infectious microbes: will an innovative air recirculation concept provide a practical solution? Environ. Res. 2021;199 [Europe PMC free article] [Abstract] [Google Scholar]
- Tan W., Niu P., Zhao X., Pan Y., Wu G. Reemergent cases of COVID-19 — xinfadi wholesales market, beijing municipality, China, June 11, 2020. China CDC Weekly. 2020;2:502–504. [Europe PMC free article] [Abstract] [Google Scholar]
- Tsang T.K., Wu P., Lin Y., Lau E.H.Y., Leung G.M., Cowling B.J. Effect of changing case definitions for COVID-19 on the epidemic curve and transmission parameters in mainland China: a modelling study. Lancet Public Health. 2020;5:e289–e296. [Europe PMC free article] [Abstract] [Google Scholar]
- Waltenburg M.A., Rose C.E., Victoroff T., Butterfield M., Dillaha J.A., Heinzerling A., Chuey M., Fierro M., Jervis R.H., Fedak K.M., Leapley A., Gabel J.A., Feldpausch A., Dunne E.M., Austin C., Pedati C.S., Ahmed F.S., Tubach S., Rhea C., Tonzel J., Krueger A., Crum D.A., Vostok J., Moore M.J., Kempher H., Scheftel J., Turabelidze G., Stover D., Donahue M., Thomas D., Edge K., Gutierrez B., Berl E., McLafferty M., Kline K.E., Martz N., Rajotte J.C., Julian E., Diedhiou A., Radcliffe R., Clayton J.L., Ortbahn D., Cummins J., Barbeau B., Carpenter S., Pringle J.C., Murphy J., Darby B., Graff N.R., Dostal T.K.H., Pray I.W., Tillman C., Rose D.A., Honein M.A., Cdc C., Emergency Response T. Coronavirus disease among workers in food processing, food manufacturing, and agriculture workplaces. Emerg. Infect. Dis. 2021;27:243–249. [Europe PMC free article] [Abstract] [Google Scholar]
- Wei L., Lin J., Duan X., Huang W., Zong Z. Asymptomatic COVID-19 patients can contaminate their surroundings: an environment sampling study. mSphere. 2020;5 e00442–20. [Europe PMC free article] [Abstract] [Google Scholar]
- Who . Vol. 2021. 2021. https://covid19.who.int/ (WHO Coronavirus (COVID-19) Dashboard). [Google Scholar]
- Wiktorczyk-Kapischke N., Grudlewska-Buda K., Walecka-Zacharska E., Kwiecinska-Pirog J., Radtke L., Gospodarek-Komkowska E., Skowron K. SARS-CoV-2 in the environment-Non-droplet spreading routes. Sci. Total Environ. 2021;770 [Europe PMC free article] [Abstract] [Google Scholar]
- Wölfel R., Corman V., Guggemos W., Seilmaier M., Zange S., Müller M., Niemeyer D., Jones T., Vollmar P., Rothe C., Hoelscher M., Bleicker T., Brünink S., Schneider J., Ehmann R., Zwirglmaier K., Drosten C., Wendtner C. Virological assessment of hospitalized patients with COVID-2019. Nature. 2020;581:465–469. [Abstract] [Google Scholar]
- Xie C., Zhao H., Li K., Zhang Z., Lu X., Peng H., Wang D., Chen J., Zhang X., Wu D., Gu Y., Yuan J., Zhang L., Lu J. The evidence of indirect transmission of SARS-CoV-2 reported in Guangzhou, China. BMC Publ. Health. 2020;20:1202. [Europe PMC free article] [Abstract] [Google Scholar]
- Yen Lee, Angela Kwok, Jan Gralton, Mary-Louise McLaws. Face touching: a frequent habit that has implications for hand hygiene. Am. J. Infect. Contr. 2015;43:112–114. [Europe PMC free article] [Abstract] [Google Scholar]
- Zhang S., Wang C., Lin M., Deng Q., Ye Y., Li Z., Qiu L., Wang Z. Analysis of the virus contamination and disinfection effect in isolation ward of patients with COVID-19. Front Public Health. 2020;8:486. [Europe PMC free article] [Abstract] [Google Scholar]
- Zhang Z., Ishida S., Tagashira S., Fukuda A. Danger-pose detection system using commodity wi-fi for bathroom monitoring. Sensors. 2019;19:884. [Europe PMC free article] [Abstract] [Google Scholar]
- Zhou J., Otter J.A., Price J.R., Cimpeanu C., Garcia D.M., Kinross J., Boshier P.R., Mason S., Bolt F., Holmes A.H., Barclay W.S. Investigating SARS-CoV-2 surface and air contamination in an acute healthcare setting during the peak of the COVID-19 pandemic in London. Clin. Infect. Dis. 2020 [Europe PMC free article] [Abstract] [Google Scholar]
- Zhou Y., Zeng Y., Chen C. Presence of SARS-CoV-2 RNA in isolation ward environment 28 days after exposure. Int. J. Infect. Dis. 2020;97:258–259. [Europe PMC free article] [Abstract] [Google Scholar]
- Zou L., Ruan F., Huang M., Liang L., Huang H., Hong Z., Yu J., Kang M., Song Y., Xia J. SARS-CoV-2 viral load in upper respiratory specimens of infected patients. N. Engl. J. Med. 2020;382:1177–1179. [Europe PMC free article] [Abstract] [Google Scholar]
Citations & impact
Impact metrics
Citations of article over time
Smart citations by scite.ai
Explore citation contexts and check if this article has been
supported or disputed.
https://scite.ai/reports/10.1016/j.envres.2021.111763
Article citations
Genetic tracing of market wildlife and viruses at the epicenter of the COVID-19 pandemic.
Cell, 187(19):5468-5482.e11, 01 Sep 2024
Cited by: 4 articles | PMID: 39303692
Persistence of two coronaviruses and efficacy of steam vapor disinfection on two types of carpet.
Virol J, 21(1):207, 02 Sep 2024
Cited by: 0 articles | PMID: 39223556 | PMCID: PMC11367742
Combined prevention and treatment measures are essential to control nosocomial infections during the COVID-19 pandemic.
World J Virol, 13(2):91286, 01 Jun 2024
Cited by: 0 articles | PMID: 38984081
Exploring indoor and outdoor dust as a potential tool for detection and monitoring of COVID-19 transmission.
iScience, 27(3):109043, 26 Jan 2024
Cited by: 1 article | PMID: 38375225 | PMCID: PMC10875567
Indoor Inactivation of SARS-CoV-2 Virus by Liquid Hyperoxygen.
Pathogens, 13(3):244, 11 Mar 2024
Cited by: 0 articles | PMID: 38535587 | PMCID: PMC10974922
Go to all (25) article citations
Data
Data behind the article
This data has been text mined from the article, or deposited into data resources.
BioStudies: supplemental material and supporting data
RefSeq - NCBI Reference Sequence Database
- (1 citation) RefSeq - NC_045512.2
Similar Articles
To arrive at the top five similar articles we use a word-weighted algorithm to compare words from the Title and Abstract of each citation.
SARS-CoV-2 RNA contamination on surfaces of a COVID-19 ward in a hospital of Northern Italy: what risk of transmission?
Eur Rev Med Pharmacol Sci, 24(17):9202-9207, 01 Sep 2020
Cited by: 14 articles | PMID: 32965015
Environmental Contamination of SARS-CoV-2 in a Non-Healthcare Setting.
Int J Environ Res Public Health, 18(1):E117, 26 Dec 2020
Cited by: 17 articles | PMID: 33375308 | PMCID: PMC7797951
The distribution of SARS-CoV-2 contamination on the environmental surfaces during incubation period of COVID-19 patients.
Ecotoxicol Environ Saf, 208:111438, 30 Sep 2020
Cited by: 15 articles | PMID: 33039873 | PMCID: PMC7526608
Stability and transmissibility of SARS-CoV-2 in the environment.
J Med Virol, 95(1):e28103, 07 Sep 2022
Cited by: 29 articles | PMID: 36039831 | PMCID: PMC9537778
Review Free full text in Europe PMC