Abstract
Free full text

Post-acute sequelae of COVID-19: Evidence of mood & cognitive impairment
Abstract
Acute health consequences associated with coronavirus disease 2019 (COVID-19) infection have been thoroughly characterized; however, long-term impacts are not yet understood. Post-acute sequelae of COVID-19 (PASC), also known as Long COVID syndrome, is the persistence of COVID-19 symptoms long after viral infection. In addition to physical symptoms, those with PASC experience changes in mental health, but few studies have empirically examined these effects. The current study investigated mood and cognitive functioning in individuals who have recovered from COVID-19 infection. We recruited 100 male and female adults (M = 30 years old) with no history of mood or cognitive impairment prior to the COVID-19 pandemic (Jan. 2020). Half of the subjects were healthy controls (i.e., no prior COVID-19 infection) and half had received a past COVID-19 diagnosis (ascertained by PCR or antibody test) but were no longer infectious. Participants completed self-reported measures of stress, depression, and anhedonia, as well as the Attention Network Test (ANT), a behavioural measure of attentional alerting, orienting and executive functioning. Relative to controls, depression and anhedonia were significantly higher in the past-COVID group. Selective impairment in attention was observed in the past-COVID group, marked by deficits in executive functioning while alerting and orienting abilities remained intact. Effects were most pronounced among individuals diagnosed 1–4 months prior to assessment. There were no group differences in pandemic-related experiences with respect to social interaction, social distancing, or isolation. The past-COVID group scored significantly higher on perceived stress; however, this did not moderate any effects observed on mood or cognition. These findings implicate a protracted reaction to the virus, possibly via prolonged inflammation, contributing to sustained mood dysregulation and cognitive impairment. Future research should examine the neural and physiological underpinnings of PASC, particularly mechanisms that promote psychiatric sequelae 1–4 months following diagnosis.
1. Introduction
The devastating health consequences of acute COVID-19 infection have been thoroughly characterized, but there is growing concern that those who survive the infection may experience long-term effects. Indeed, many survivors develop chronic and debilitating symptoms long after viral recovery, despite mild illness severity at onset (Carfi et al., 2020), pointing to residual effects that span multiple organ systems within the peripheral and central nervous system (CNS). Scientific and medical communities have identified an urgent need to investigate this syndrome, known as post-acute sequelae of COVID-19 (PASC; National Institutes of Health, 2021), in order to develop effective therapies and to prevent further escalation of adverse health outcomes.
Currently, efforts toward elucidating PASC focus predominantly on lingering respiratory complications following infection, which largely include chronic pulmonary diffusion abnormalities (Cortinovis et al., 2021; Shah et al., 2021). Mental health consequences, however, have also been identified among large proportions of COVID-19 survivors. Not only are these incidences observed in non-hospitalized individuals, but they are significantly higher relative to matched cohorts with post-viral influenza or any respiratory tract infection (RTI; Taquet et al., 2021a). This might implicate a COVID-19-specific origin, although chronic psychiatric disturbances have been reported with other betacoronaviruses in the past (Rogers et al., 2020). Of relevance, a retrospective study found that more than a quarter (n = 30/90) of Severe Acute Respiratory Syndrome (SARS) survivors presented with a psychiatric disorder at 30 months post-illness, with 15% (n = 14/90) showing depression (Mak et al., 2009). Of those with post-SARS depression, 71% (n = 10/14) had comorbid post-traumatic stress disorder (PTSD), confounding any direct effects of SARS on depressed mood.
There is little consensus surrounding the precise onset or duration of PASC; however, neurological and psychiatric morbidities have been recorded up to six months following COVID-19 infection, including first-time psychiatric diagnoses (Taquet et al., 2021a, 2021b). In addition to neurodegenerative and cerebrovascular disorders, symptoms of mood disorders have emerged in large proportions of survivors (Taquet et al., 2021b). Early COVID-19 studies reported increased depressive symptoms shortly after infection (e.g., 1 month; Mazza et al., 2020; Paz et al., 2020), but acute post-viral symptoms and few control variables in these studies hinder the ability to draw conclusions related to PASC. More recently, Perlis et al. (2021) found that over half (n = 2046/3904) of individuals with prior COVID-19 illness met criteria for major depressive disorder (MDD) on average 4 months following initial symptoms. Notably, the study did not exclude participants on the basis of prior psychiatric illness, which is a limitation given bidirectional associations between COVID-19 and psychopathology. Specifically, those with prior psychiatric diagnoses are at higher risk for COVID-19 infection (Taquet et al., 2021b) and show more severe psychopathology following infection (Lamontagne et al., 2020; Mazza et al., 2020). Accordingly, studies must carefully control for preexisting psychiatric or neurological disturbances, as well as any pandemic-related mood dysregulation (e.g., isolation, worry).
Anhedonia (i.e., a loss of interest or pleasure) is a core symptom of MDD that has emerged in clinical descriptions of PASC, but has yet to be studied systematically. Elevated anhedonic symptoms have been observed 2 weeks post-COVID-19 (El Sayed et al., 2021), but these conclusions were drawn without a comparator (i.e., a non-COVID-19 group). Nevertheless, these findings are salient in the context of COVID-19 infection, given protracted proinflammatory responses associated with this virus (Mould and Janssen, 2021). Indeed, prevailing hypotheses surrounding psychiatric sequela of COVID-19 point to sustained neuroinflammatory processes that disrupt the blood-brain-barrier, damaging neurons and glia (Alonso-Lana et al., 2020). Preclinical (Rossetti et al., 2016) and clinical (Eisenberger et al., 2010) literature strongly support a link between widespread systemic inflammation and reward processing dysregulation, necessitating further investigations into COVID-19 and anhedonia.
Relatedly, individuals with prior COVID-19 illness commonly report a diminished ability to concentrate (“brain fog”) that persists for weeks to months (Nalbandian et al., 2021). In the acute phase (4–6 weeks after symptom onset), the vast majority of hospitalized or inpatient COVID-19 survivors show cognitive impairment as assessed by clinical screenings (e.g., Brief Memory & Executive Test [BMET] or Montreal Cognitive Assessment [MoCA]; Hosp et al., 2021; Jaywant et al., 2021). Directly relevant to the current study, neuropsychological tests reveal deficits predominantly in executive function, with specific impairment in orientation, processing speed, set-shifting and divided attention (Jaywant et al., 2021). Importantly, preexisting cognitive deficits are rarely ruled out, making these findings difficult to interpret. Some have hypothesized that executive function disturbances post-COVID-19 infection could be a consequence of frontal lobe pathology (Ardila and Lahiri, 2020). Of note, severe executive dysfunction within 1 month of symptom onset is also accompanied by predominant frontoparietal hypometabolism (Hosp et al., 2021), implicating a precise neurological underpinning within the central executive network (CEN). In these studies, the interval between symptom onset and evaluation is short; thus, it remains unclear whether cognitive deficits are consequences of residual (i.e., acute) COVID-19 responses (e.g., hyperinflammation) or if they reflect collateral post-viral effects that are not yet understood.
Mounting evidence points to post-viral effects of COVID-19 on mood and cognitive abilities, but whether or not dysregulation progresses over time remains inconclusive. In the current study, we examined the long-term effects of SARS-CoV-2 infection on mood and cognition, with a particular emphasis on two prominent symptoms of MDD: anhedonia and cognitive dysfunction. Contrary to other studies, we implemented rigorous pre-screening to exclude individuals who had a history of psychiatric or neurological disorders prior to the COVID-19 pandemic. This decision was based on literature showing bidirectional associations between SARS-CoV-2 and psychopathology (Taquet et al., 2021b), including cognitive impairment (Batty et al., 2020). We recruited from the general population, with no restrictions on COVID-19 severity or maximum time elapsed since diagnosis. Critically, we assessed and controlled for group differences in pandemic-related experiences, given extensive literature showing elevated stress during the COVID-19 pandemic (Kujawa et al., 2020). Stress has profound effects on both mood (Pizzagalli, 2014) and cognitive functioning (McEwen and Sapolsky, 1995), but few studies have accounted for its role in PASC. To assess cognitive abilities, we administered the Attention Network Test (ANT), which is a computer-based behavioural task of executive functioning, orientation and alerting. To our knowledge, our study is the first to implement a behavioural task to investigate cognitive dysfunction in individuals who recovered from COVID-19.
We subdivided our COVID-19 cohort into three groups: acute COVID-19, PASC and post-PASC. Prior studies have defined acute COVID-19 as the interval up to 1 month following symptom onset (Nalbandian et al., 2021). We adapted this definition by excluding the 2-week period immediately following diagnosis, given that SARS-CoV-2 has been found to replicate up to this time frame (i.e., the host is infectious for up to two weeks; Centers for Disease Control and Prevention, 2021). This modification helps eliminate disruptions in mood or cognition as a result of ongoing infection (e.g., fever, nausea), although we recognize that some individuals may show these symptoms beyond viral infection. We defined PASC as the interval beyond 1 month (see Nalbandian et al., 2021) and up to 4 months post-diagnosis based on literature highlighting psychiatric sequela within this 3-month interval (Hosp et al., 2021; Perlis et al., 2021). Finally, we defined post-PASC as the interval beyond 4 months of COVID-19 diagnosis. Based on recent literature, we hypothesized that prior COVID-19 infection would increase symptoms of MDD, particularly anhedonia and cognitive dysfunction, relative to controls. In particular, we expected to see impairments in the acute COVID-19 phase that would persist into the PASC phase. This would support ongoing investigations showing sustained mental health disruptions beyond viral recovery.
2. Methods
2.1. Participants
Fifty participants with a prior COVID-19 diagnosis and 50 controls were recruited from Canada and the United States through advertisements on Craigslist (50%), social media (32%), and Reddit (18%). Sample sizes in the COVID-19 subgroups were as follows: acute COVID-19 (n = 15), PASC (n = 17) and post-PASC (n = 15). Inclusion criteria included right-handedness (Chapman and Chapman, 1987), age between 18 and 60 years, normal (or corrected-to-normal) vision, fluency in English and, for the COVID-19 group, a past (> 14 days) COVID-19 diagnosis as confirmed by an antibody or polymerase chain reaction (PCR) test. Based on an eligibility survey we developed, participants were excluded if they reported experiencing mood irregularities (e.g., depression, mania), cognitive deficits (e.g., ADHD, learning disability) or psychosis at any time prior to the COVID-19 pandemic (January 2020). Participants were also excluded if they reported lifetime history of chronic or serious medical, neurological or hormonal disturbances (e.g., seizures, concussion); current or past alcohol/drug abuse (drug use in the past 3 weeks); or any psychological disorder (e.g., obsessive compulsive disorder, eating disorders).
The eligibility survey was completed by 426 individuals. Based on the eligibility criteria listed above, only 24% (N = 100) were deemed eligible to proceed to the study. Internet Protocol (IP) addresses were recorded and participants were excluded if their IP address was previously registered. When eligibility surveys lacked detail or omitted critical information, a follow-up phone interview was conducted. Eligible participants were sent the study link by email and paid $30 in their respective currency (CAD or USD) upon completion. This study was approved by the Queen's University Internal Review Board (Study Number: GPSYC-1027-20).
2.2. Clinical measures
To assess current mood states, participants completed a series of inventories, including the Beck Depression Inventory-II (BDI-II; Beck et al., 1996), the Snaith-Hamilton Pleasure Scale (SHAPS; Snaith et al., 1995) and the Mood and Anxiety Symptom Questionnaire (MASQ; Watson et al., 1995). The MASQ assessed anxiety-specific symptoms (anxious arousal) and depression-specific symptoms (anhedonic depression, i.e., loss of interest and positive affect). The positive affect subscale was reverse scored, such that higher values indicate high positive affect. To assess current (self-reported) cognitive abilities, participants completed the Attentional Control Scale (ACS; Derryberry and Reed, 2002), which assessed attention focusing and shifting. Participants also completed the Perceived Stress Scale (PSS; Cohen et al., 1983) and the Pandemic Stress Index (PSI; Harkness, 2020), both of which assessed the degree to which current situations (e.g., lockdown, isolation) were appraised by the individual as being stressful and disruptive to daily life. The administration of these measures took approximately 30 min.
The COVID-19 group completed an additional survey related to their experience with the virus. These items included the date of their diagnosis (as determined by a positive PCR or antibody test), a checklist of COVID-19 symptoms that they experienced, and whether they sought medical attention (including hospitalization). They also completed a Likert sliding scale denoting the severity of their illness from mild (0) to severe (100). As a reliability check, participants completed this scale once during the eligibility survey (time 1) and again during the study survey (time 2). A difference score was calculated (rating at time 2 minus rating at time 1) to quantify the discrepancy between the two time points. The average of the two ratings was used to calculate the final severity score for each participant (the average difference score between the two ratings was 8).
2.3. Task
Participants completed the Attention Network Test (ANT; Fan et al., 2002) (see Fig. 1), which is a behavioural task used to assess three attentional networks: alerting, orienting and executive control (conflict). During the computer task, participants were shown an array of five arrows which were preceded by one of four cue types (no cue, center cue, double cue or spatial cue) used to predict target presentation and/or location (i.e., above or below a fixation cross). Participants were instructed to use two keys on their keyboard to indicate the direction (left or right) of the central arrow (the target), which was surrounded by congruent («<<<), incongruent (»<») or neutral (– –<– –) flankers. They were also instructed to respond as quickly and accurately as possible.
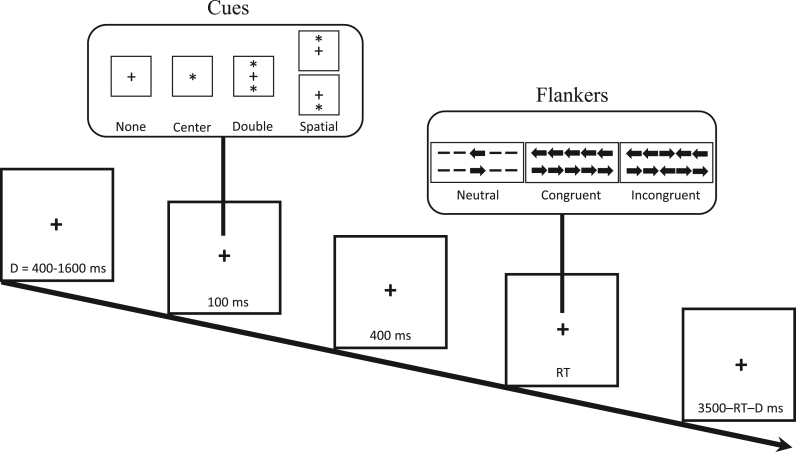
Attention Network Test (ANT). For each trial of this computer task (288 trials total), a fixation cross is presented for a random duration (D = 400–1600 ms) followed by one of four cue types (100 ms): no cue, center cue, double cue or spatial cue. These cues are used to predict target presentation and/or location. Then, a fixation cross is presented (400 ms) followed by presentation of an array of five arrows (target and flankers). Using two keys on their keyboard, participants are instructed to indicate the direction (left or right) of the central arrow, which is surrounded by neutral, congruent or incongruent flankers. They are instructed to respond as quickly and accurately as possible. Each trial ends with a fixation cross (3500 ms – reaction time [RT] – D). The task takes approximately 30 min to complete.
The task consisted of 288 pseudo-randomized trials divided into three blocks (96 trials each) with a break between each block. For each trial, a fixation cross was presented (400–1600 ms) followed by a cue (100 ms) and then a fixation cross (400 ms). The array of arrows (target and flankers) were then presented until the participant responded. The trial ended with a fixation cross (3500 ms - RT - fixation cross duration). The task took approximately 30 min to complete.
2.4. Procedure
All data collection in the current study occurred between January and March 2021. Eligible participants were contacted by email with detailed instructions for completing the study, a web link to access the study and a random ID. The web link directed participants to a web-based application (Research Electronic Data Capture; REDCap; Vanderbilt University), which included a letter of information (LOI) and consent form. If an individual wished to proceed to the study, they were required to provide electronic informed consent. As a means to protect privacy, participants were instructed to enter their ID into the online database following the informed consent procedure.
The study consisted of two components, both of which were conducted online. In the first half, which was administered in REDCap, participants completed a demographics survey and self-report questionnaires (BDI, SHAPS, MASQ, ACS, PSS, PSI). In the second half, they were directed to download an online experimentation software, Inquisit Player 6.4.1 (Millisecond Software), in order to complete the ANT. In the initial instructions, participants were advised to complete the task using a laptop or desktop computer as the software was not compatible with mobile devices. All participants completed a practice test comprised of 24 trials prior to task onset. Upon task completion, participants were shown a debriefing page and were later contacted by study staff to arrange electronic payment for their participation.
2.5. Data analysis
Within the COVID-19 group, three participants did not indicate the date of their diagnosis, resulting in a final sample size of 47 for the COVID-19 subgroups. For the ANT, 8 participants in the COVID-19 group and 6 participants in the control group did not complete the task due to technical difficulties. Two participants in the COVID-19 group were removed from ANT analyses because their RT scores were greater than 3 SDs above the mean. Final sample sizes were as follows: Control [n = 50 for self-reports, n = 44 for ANT], acute COVID-19 [n = 15 for self-reports, n = 13 for ANT], PASC [n = 17 for self-reports, n = 14 for ANT], and post-PASC [n = 15 for self-reports, n = 10 for ANT].
Differences in demographic characteristics between the COVID-19 and control groups were analysed. Chi-square (χ2) tests were used to assess categorical variables (country, gender, income bracket, race/ethnicity), whereas independent samples t tests were used to assess continuous variables (age, education).
Two analyses were conducted for each self-report measure. Independent samples t tests were performed to assess differences between the COVID-19 and control group and one-way analysis of variance (ANOVAs) were performed to examine differences between the three COVID-19 subgroups and the control group. Pairwise comparisons were reported for significant interactions using Bonferroni multiple-significance-test corrections. Pearson correlations were used to examine relationships between self-report measures in each group.
For the ANT, incorrect trials or trials with RTs less than 100 ms or greater than 1700 ms were removed from RT analyses. Furthermore, trials with RTs falling outside the mean ± 2 SDs were excluded. Overall, 1% of trials were excluded from the final analyses. Accuracy and RTs were analysed using mixed-model ANOVAs. First, a general 2 (groups) x 4 (cues) x 3 (condition) ANOVA was conducted with group (control, COVID-19) as the between-subjects factor and cues (none, center, double, spatial) and condition (congruent, incongruent, neutral) as within-subjects factors. Second, a 4 (groups) x 4 (cues) x 3 (condition) ANOVA was conducted with subgroups (control, acute COVID-19, PASC, post-PASC) as the between-subjects factor. Based on emerging literature showing cognitive impairments following COVID-19 infection (e.g., Ardila and Lahiri, 2020; Jaywant et al., 2021; Puchner et al., 2021), we developed an a priori hypothesis that prior COVID-19 diagnosis would impair performance within each of the attention networks. Accordingly, one-tailed t tests and ANOVAs were used for all ANT analyses.
Scores for each attention network were calculated using RTs: The alerting network (RTNo Cue− RTDouble Cue) examines changes in RT following a warning signal; the orienting network (RTCentral Cue− RTSpatial Cue) examines changes in RT following spatial cues that precede a target's location; and the executive network (RTIncongruent− RTCongruent) examines changes in RT under conditions of increased conflict (interference effect). For the alerting and orienting networks, higher scores indicated greater efficiency; for the executive network, higher scores indicated less efficiency.
For each analysis, a multiple regression model was used to investigate whether stress (as indexed by the PSS) moderated the relationship between prior COVID-19 diagnosis and mood or cognitive impairments. Whenever necessary, Greenhouse Geisser corrections were used for violations of sphericity in mixed-model ANOVAs. Finally, a Bonferroni correction (0.05 /# of tests) was used for multiple comparisons.
3. Results
3.1. Demographic characteristics & COVID-19 experience
Demographic and COVID-19 data for the full sample are summarized in Table 1. The COVID-19 and control groups did not differ with respect to age [t(98) = -0.93, p = 0.35], gender [χ2(1) = 1.95, p = 0.16], income [χ2(5) = 8.59, p = 0.13], education [t(98) = -0.98, p = 0.33] or race/ethnicity [χ2(4) = 0.56, p = 0.97]. Relative to the control group, there were significantly more people from the USA and fewer people from Canada in the COVID-19 group [χ2(1) = 22.50, p < 0.001]. Notably, participants residing in the USA did not differ from those in Canada on demographic characteristics (ps > .28), COVID-19 or pandemic stress experiences (ps > .29), clinical self-reports (ps > .10) or ANT measures (ps > .81).
Table 1
Demographic information and COVID-19 experience.
Characteristic | COVID n = 50 | Control n = 50 |
---|---|---|
Age, Mean (SD) | 30.80 (7.79) | 29.14 (9.87) |
Country, No. (%) | ||
Canada | 11 (22) | 35 (70)![]() |
USA | 39 (78)![]() | 15 (30) |
Gender, No. (%) | ||
Female | 29 (58) | 35 (70) |
Male | 21 (42) | 14 (28) |
Non-binary | 0 | 1 (2) |
Income, No. (%) | ||
< $50,000 | 24 (48) | 17 (34) |
$50,000-$100,000 | 10 (20) | 22 (44) |
> $100,000 | 16 (32) | 11 (22) |
Education, Mean (SD) (# years) | 16.12 (2.95) | 15.54 (2.93) |
Race/ethnicity, No. (%) | ||
White | 26 (52) | 26 (52) |
Black | 10 (20) | 8 (16) |
Hispanic | 8 (16) | 8 (16) |
Asian | 4 (8) | 5 (10) |
Indigenous | 2 (4) | 3 (6) |
Acute COVID-19 severity, Mean (SD) (/100) | 54.71 (23.05) | N/A |
Days post-diagnosis, Mean (SD) | 123.63 (94.71) | N/A |
Symptoms, No. (%) | N/A | |
Asymptomatic | 2 (4) | |
Cough | 33 (66) | |
Fever | 23 (46) | |
Fatigue | 39 (78) | |
Myalgia | 28 (56) | |
Dyspnea | 18 (36) | |
Headache | 34 (68) | |
Diarrhea | 22 (44) | |
Nausea/vomiting | 17 (34) | |
Sore throat | 23 (46) | |
Nasal congestion | 26 (52) | |
Loss of taste/smell | 31 (62) |
Asterisks denote between-group statistical significance, () p < 0.05.
Within the COVID-19 group, 4% were asymptomatic, 52.1% received medical attention for their symptoms and 2% were hospitalized. The average number of COVID-related symptoms was 6.3 (SD = 2.8), which on average persisted for 17.5 days (SD = 20.8) after first symptom onset. Within the COVID-19 group, the age range was 20–53 years old; within the control group, the age range was 19–60 years old. On average, participants rated the severity of their acute COVID-19 illness as moderate (M = 54.71/100), ranging from 2/100 to 95/100. The average severity rating was 56.64 in the eligibility survey and 51.46 in the study survey. Severity scores did not differ between the three COVID-19 subgroups, F(2,40) = 0.03, p = 0.98. Moreover, the average number of days post-diagnosis (i.e., # days from COVID-19 diagnosis to study completion) was 123.63 (~4 months) with a range of 14–312 days. COVID-19 severity did not differ by gender [t(48) = -1.46, p = 0.15] or ethnicity [F(4,49) = 0.52, p = 0.72]. Severity rating was significantly higher in individuals who experienced fever, dyspnea, nausea/vomiting or coughing relative to those who did not experience these symptoms, ts(48)>-2.20, ps < 0.03. Finally, severity ratings were significantly higher among those who received medical attention (M = 67.52, SD = 14.55) relative to those who did not (M = 42.57, SD = 23.68), t(48) = -4.35, p < 0.001.
3.2. Pandemic-related stress
As shown in Fig. 2A, individuals with a prior COVID-19 diagnosis showed significantly higher PSS scores relative to controls [t(98) = -3.45, p = 0.001], implicating a higher perception of life stress in this group. For the PSI (Fig. 2B–F), the COVID-19 group reported a greater effect of the pandemic on day-to-day life [t(98) = 1.96, p < 0.05], but there were no group differences with respect to number of life changes during the pandemic [t(98) = -1.62, p = 0.11], number of days social distancing [t(95) = -0.52, p = 0.61], number of days socially interacting with others [t(98) = 0.63, p = 0.53], and number of days in isolation [t(96) = -1.55, p = 0.13].
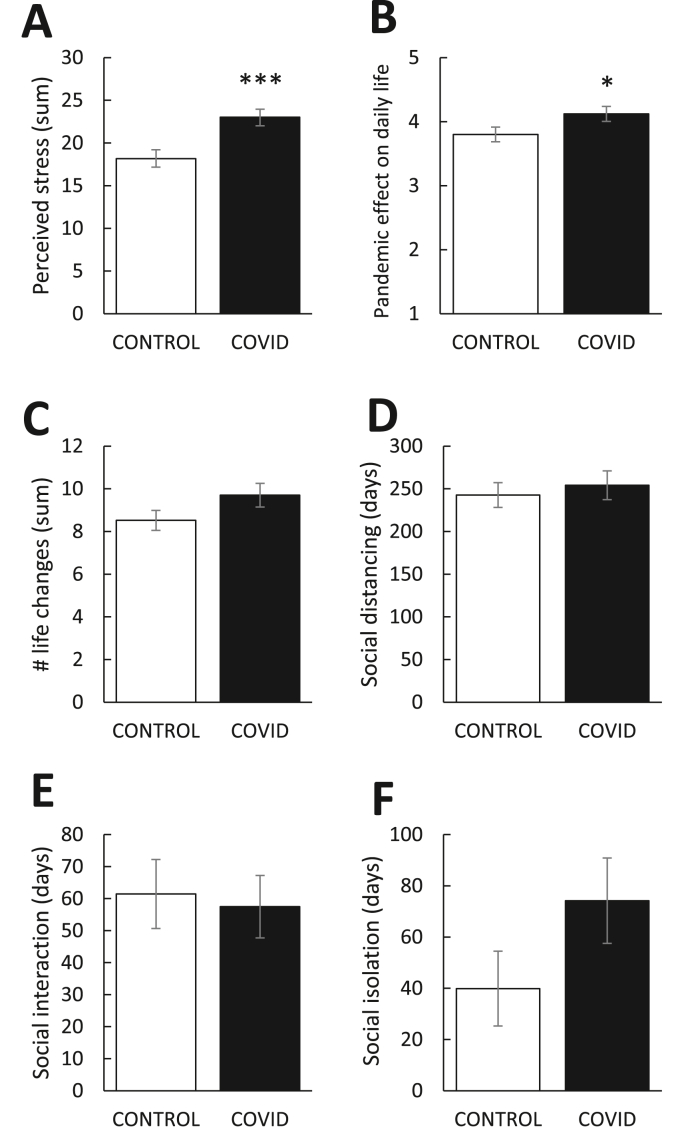
Mean (±SEM) pandemic-related experiences as indexed by the (A) Perceived Stress Scale and (B–F) Pandemic Stress Index. Relative to controls (n = 50), individuals with prior COVID-19 diagnosis (n = 47) (A) showed significantly higher perceived stress and (B) reported greater disruption in their day-to-day life as a direct result of the pandemic (scale 1 to 5). There were no group differences in self-reported (C) number of major life changes following the pandemic or number of days (D) social distancing, (E) interacting with others or (F) socially isolating (i.e., quarantine). Asterisks denote statistical significance, () p < 0.05, (
) p < 0.001.
3.3. Self-report measures of mood & attention
3.3.1. Depression & anxiety
As shown in Fig. 3, individuals with a prior COVID-19 diagnosis showed significantly higher BDI-II [t(98) = -2.60, p < 0.01] and MASQ Anxious Arousal [t(98) = 4.45, p < 0.001] scores relative to controls, suggesting these individuals have higher depression and anxiety. BDI-II scores did not differ among the COVID-19 subgroups, although there was a trend toward significance [F(3,93) = 2.35, p = 0.08]. MASQ Anxious Arousal scores were significantly different between the COVID-19 subgroups [F(3,93) = 9.48, p < 0.001], such that higher scores were observed in the acute and PASC groups relative to controls, ps < 0.001. Anxious arousal scores in the acute and PASC groups were significantly higher than the post-PASC group, ps < 0.01, but the two groups did not differ from each other, p = 0.30.
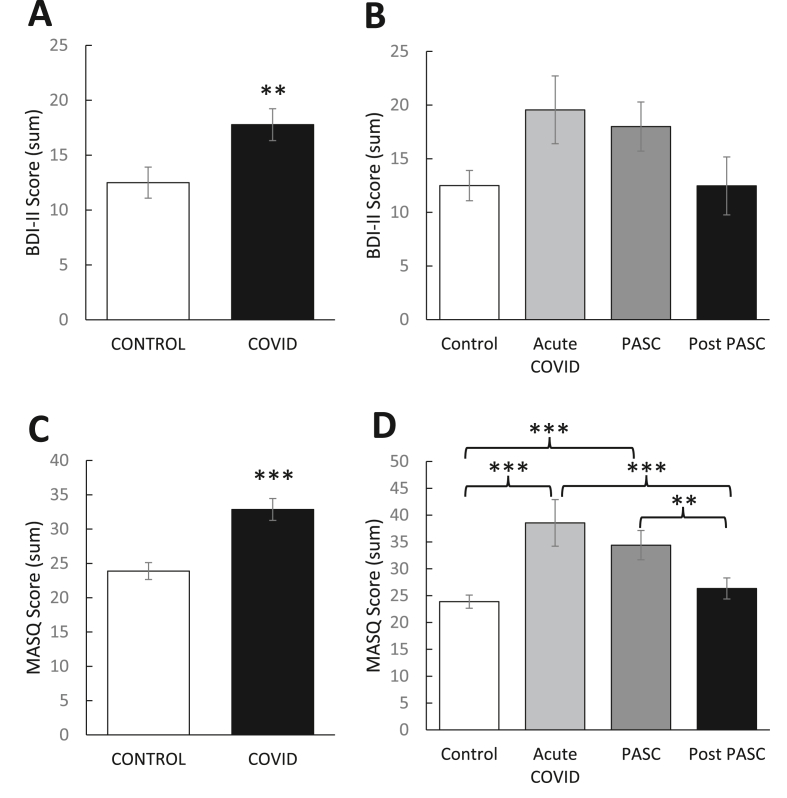
Mean (±SEM) self-reported (A, B) depression and (C, D) anxiety as indexed by the Beck Depression Inventory-II (BDI-II) and Anxious Arousal subscale of the Mood and Anxiety Symptom Questionnaire (MASQ), respectively. Relative to controls (n = 50), individuals with prior COVID-19 diagnosis (n = 47) showed significantly higher (A) BDI-II and (C) MASQ scores. (B, D) This effect was most pronounced in the acute (14–30 days post diagnosis) and PASC (1–4 months post diagnosis) phases. (B) BDI-II scores were not significantly different among the three post-diagnosis phases. (D) MASQ scores were significantly lower in the post PASC (4+ months post diagnosis) phase relative to the acute and PASC phases. Asterisks denote statistical significance, () p < 0.05, (
) p < 0.01, (
) p < 0.001. PASC = Post-Acute Sequelae of COVID-19.
3.3.2. Anhedonia
Fig. 4A shows significantly higher SHAPS scores in individuals with a prior COVID-19 diagnosis relative to controls [t(98) = -2.98, p = 0.004], suggesting these individuals report reduced capacity to experience pleasure. As shown in Fig. 4B, SHAPS scores were significantly different between the COVID-19 subgroups [F(3,93) = 4.51, p = 0.005], such that scores in the PASC (but not acute, p = 0.08) group were significantly higher than the control group, p < 0.001. Scores in the post-PASC group were significantly lower than the PASC group, p < 0.05. Analysis using the traditional binary scoring (≤ 2 = normal; ≥ 3 = abnormal; Snaith et al., 1995) showed a greater number of abnormal SHAPS scores in the COVID-19 group relative to the control group [χ2(1,N = 100) = 5.84, p = 0.02]. Binomial statistics also revealed that significantly more people in the control group had scores ≤2 compared to the COVID-19 group, p = 0.007. In the COVID-19 group, there were a significantly greater number of abnormal scores (no = 22; 44%) than would be expected (nE = 16.3; 33%) and there were a significantly lower number of normal scores (no = 27; 54%) than would be expected (nE = 32.7; 65%), ps < 0.01. The opposite effect was observed in the control group, with a significantly greater number of normal scores (no = 39; 78%) than would be expected (nE = 33.3; 67%) and a significantly lower number of abnormal scores (no = 11; 22%) than would be expected (nE = 16.7; 33%), ps < 0.01.
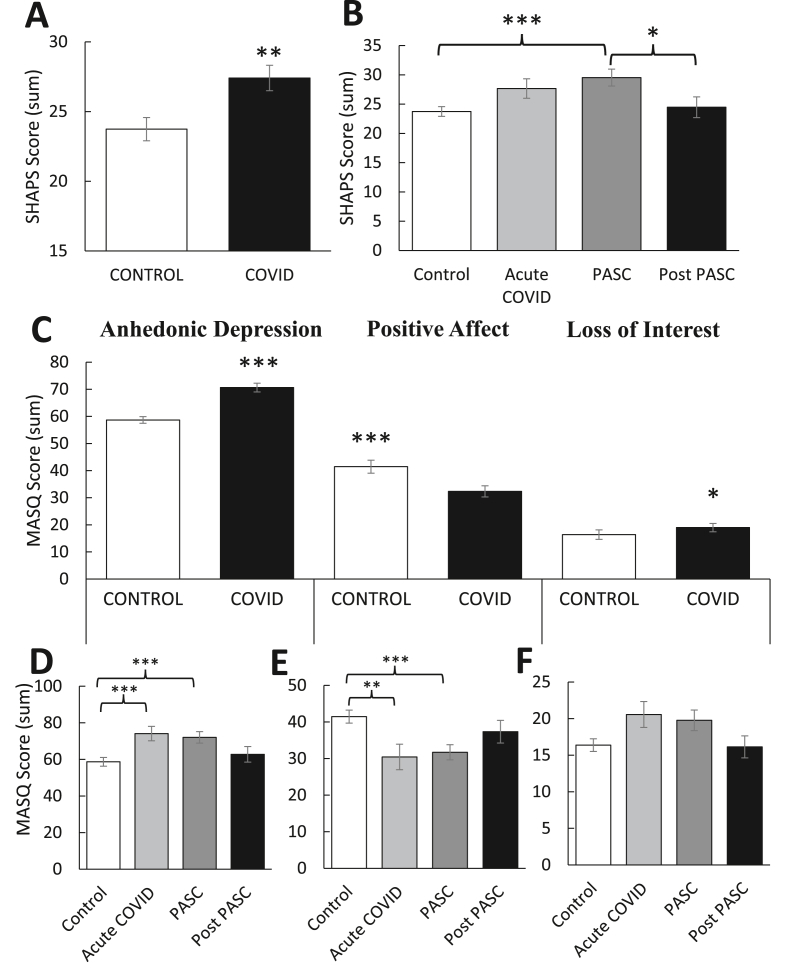
Mean (±SEM) self-reported anhedonia as indexed by the (A–B) Snaith–Hamilton Pleasure Scale (SHAPS) and (C–F) Anhedonic Depression, Positive Affect and Loss of Interest subscales of the Mood and Anxiety Symptom Questionnaire (MASQ). Relative to controls (n = 50), individuals with prior COVID-19 diagnosis (n = 47) showed (A) higher SHAPS scores (denoting reduced pleasure capacity), which (B) were greatest in the PASC (1–4 months post diagnosis) phase. They also showed (C) higher Anhedonic Depression and Loss of Interest, but lower Positive Affect scores, each of which were most pronounced in the acute (14–30 days post diagnosis) and PASC phases. Asterisks denote statistical significance, () p < 0.05, (
) p < 0.01, (
) p < 0.001. PASC = Post-Acute Sequelae of COVID-19.
As shown in Fig. 4C, individuals with a prior COVID-19 diagnosis reported higher MASQ Anhedonic Depression scores [t(98) = 3.78, p < 0.001] relative to controls, specifically showing lower Positive Affect [t(98) = -3.89, p < 0.001] and higher Loss of Interest [t(98) = 2.16, p < 0.05]. Fig. 4D shows significant differences between the COVID-19 subgroups for MASQ Anhedonic Depression [F(3,93) = 4.58, p = 0.005], such that scores in the acute and PASC groups were significantly higher than scores in the control group, ps < 0.008, but the two groups did not differ from each other, p = 0.75. For Positive Affect (Fig. 4E), there were significant differences between the COVID-19 subgroups [F(3,93) = 4.43, p = 0.006], such that scores in the acute and PASC groups were significantly lower than scores in the control group, ps < 0.01, but the two groups did not differ from each other, p = 0.79. As shown in Fig. 4F, there were no significant differences in Loss of Interest between the COVID-19 subgroups, but the effect approached significance [F(3,93) = 2.44, p = 0.07).
3.3.3. Self-reported attention
Individuals with a prior COVID-19 diagnosis did not differ from controls on total ACS scores [t(98) = 0.25, p = 0.80], nor did they differ on either ACS subscale: attention focusing [t(98) = 0.02, p = 0.98]; attention shifting [t(98) = 0.58, p = 0.56], suggesting prior COVID-19 infection does not alter self-reported attentional control (data not shown).
3.4. Attention Network Test (ANT)
3.4.1. Accuracy
As expected, there was a significant main effect of Condition [F(2,166) = 9.72, p < 0.001], such that accuracy was significantly lower for incongruent compared to congruent or neutral trials, ps < 0.001. There was also a significant main effect of Group [F(3,77) = 2.07, p < 0.05], such that accuracy in the control group (M = 96.0%, SE = 2.3) was significantly higher than both the acute COVID-19 (M = 83.2%, SE = 5.9) and PASC (M = 88.1%, SE = 4.1) groups, ps < .05. No additional main effects (ps > 0.07) or interactions (ps > 0.28) emerged for accuracy (data not shown).
3.4.2. Reaction time
A significant main effect of Group emerged [F(3,77) = 3.35, p < 0.01], such that RTs in the PASC group were significantly slower than the control group, p = 0.002. RTs among the other COVID-19 subgroups did not differ from each other. A main effect of Condition also emerged [F(2,140) = 114.22, p < 0.001], such that RT was significantly higher for incongruent compared to congruent or neutral trials, ps < 0.001. Finally, there was a significant main effect of Cue [F(3,210) = 40.90, p < 0.001], such that RTs for the no cue and center cue conditions were significantly higher than the double or spatial cue conditions, ps < 0.001. RT for the no cue condition was significantly higher than all other cue conditions, ps < 0.001. No additional main effects emerged, ps > 0.08.
Critically, a 2-way Condition × Group interaction emerged [F(6,140) = 1.79, p = 0.04], such that RTs in the PASC group were significantly higher than the control group for congruent (p = 0.02), incongruent (p = 0.001) and neutral (p = 0.008) trials. No other pairwise differences emerged among the COVID-19 subgroups, all ps > 0.08. No additional 2-way interactions emerged, ps > 0.05.
As shown in Table 2, a 3-way Group X Condition × Cue interaction emerged [F(18,420) = 1.47, p = 0.04]. To follow-up, three separate Cue X Group ANOVAs were conducted for each flanker condition with a Bonferroni adjustment (α = .017). Analyses revealed a significant interaction within the incongruent condition [F(9,213) = 2.01, p = 0.005], such that RTs in the PASC group were significantly higher than the control group for no cue (p = 0.005), center cue (p < 0.001), double cue (p = 0.005) or spatial cue (p < 0.001) conditions. There was also a significant interaction within the neutral condition [F(9,213) = 3.11, p = 0.001], such that RTs in the PASC group were significantly higher than the control group for all cue conditions, ps < .003. There was no interaction within the congruent condition [F(9,216) = 1.46, p = 0.08]. No other pairwise differences emerged among the COVID-19 subgroups, all ps > 0.05.
Table 2
Mean (±SEM) reaction times for correct responses in the Attention Network Task (ANT).
Group | No Cue | Center Cue | Double Cue | Spatial Cue | |
---|---|---|---|---|---|
Congruent | |||||
Control | 519 (+13) | 476 (+12) | 460 (+10) | 456 (+12) | |
Acute COVID | 574 (+21) | 500 (+31) | 486 (+35) | 493 (+33) | |
PASC | 593 (+39)![]() ![]() | 523 (+29) | 524 (+37)![]() | 516 (+30)![]() | |
Post PASC | 531 (+25) | 481 (+26) | 471 (+24) | 457 (+21) | |
Incongruent | |||||
Control | 570 (+13) | 564 (+14) | 555 (+13) | 536 (+14) | |
Acute COVID | 628 (+21) | 615 (+17) | 582 (+19) | 597 (+18) | |
PASC | 650 (+32)![]() ![]() | 714 (+78)![]() ![]() ![]() | 634 (+41)![]() ![]() | 676 (+77)![]() ![]() | |
Post PASC | 599 (+29) | 582 (+31) | 577 (+27) | 559 (+39) | |
Neutral | |||||
Control | 510 (+11) | 454 (+10) | 445 (+10) | 428 (+9) | |
Acute COVID | 565 (+32) | 521 (+18)![]() | 440 (+16) | 457 (+16) | |
PASC | 586 (+36)![]() ![]() | 520 (+31)![]() ![]() | 496 (+28)![]() | 486 (+23)![]() ![]() | |
Post PASC | 513 (+19) | 458 (+20) | 457 (+29) | 454 (+25) |
Bolded cells indicate the value is significantly different from the control group. Asterisks denote statistical significance, () p < 0.05, (
) p < 0.01, (
) p < 0.001.
3.4.3. Attention networks
As shown in Fig. 5A, individuals with a prior COVID-19 diagnosis had higher RTs for conflict compared to controls [t(82) = -1.85, p = 0.03], implicating reduced efficiency within the executive network. The effect size was medium [Cohen's d = 0.40]. There were no group differences for either the alerting or orienting networks, ps > 0.23. As shown in Fig. 5B–D, there was a significant difference between the COVID-19 subgroups [F(3,77) = 2.17, p < 0.05], such that the PASC group were characterized by significantly higher conflict RTs than the control group, p = 0.01. The effect size was medium [η2 = 0.09]. No other differences emerged, all ps > 0.17.
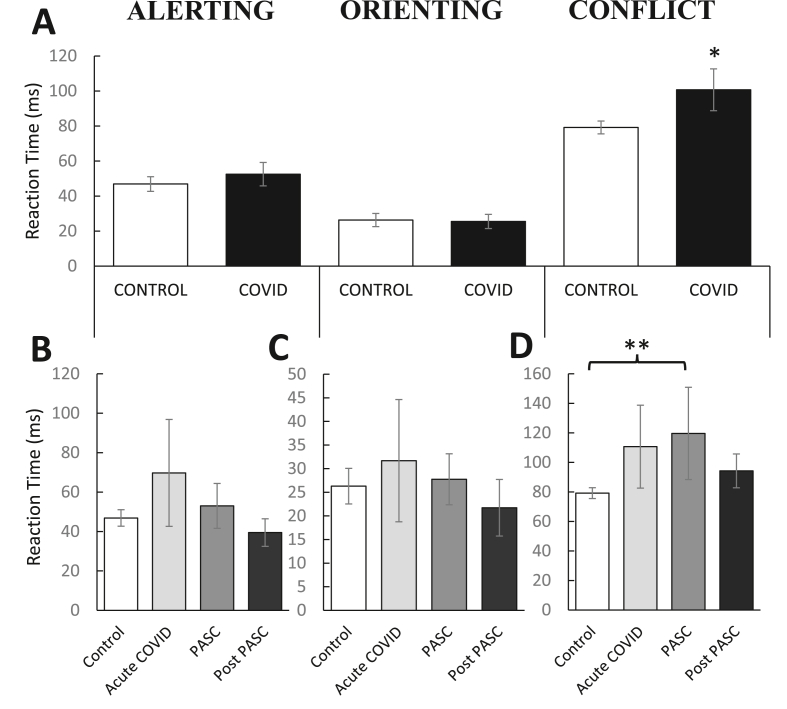
Mean (±SEM) reaction time (RT) for the three attentional networks in the Attention Network Test (ANT): Alerting (RTNo Cue− RTDouble Cue), Orienting (RTCentral Cue− RTSpatial Cue) and Conflict (RTIncongruent− RTCongruent). (A) Prior COVID-19 diagnosis was associated with selective attentional impairments; specifically, deficits in executive control but not alerting or orienting abilities. Relative to controls (n = 44), past COVID-19 infection (n = 37) did not alter (B) alerting or (C) orienting abilities at any point following diagnosis; however, (D) executive control was exclusively impaired in the PASC phase (1–4 months post diagnosis). This effect did not persist into the post PASC phase (4+ months post diagnosis). Asterisks denote statistical significance, () p < 0.05, (
) p < 0.01. PASC = Post-Acute Sequelae of COVID-19.
3.5. Complementary analyses
Independent multiple regression models revealed that PSS scores did not moderate the effect of COVID-19 on BDI-II, MASQ or SHAPS scores [Bs < −0.26, SEs>0.17, βs<−0.43, ps > 0.28], suggesting the effects on mood are independent of perceived life stress. Similarly, PSS scores did not moderate the effect observed within the conflict network [B = −1.71, SE = 1.92, β = −0.50, p = 0.38], suggesting the effect of COVID-19 on executive control did not depend on one's perceptions of current life stress. Together, the variables accounted for only 6% of the variance in executive control, R2 = 0.06, F(3,77) = 1.50, p = 0.22.
Table 3 summarizes bivariate Pearson correlations for self-report measures of mood in each group. As expected, in both the COVID-19 and control groups, there were significant positive relationships between BDI-II scores and anxious arousal [rs(48)>0.32, ps < 0.03], anhedonic depression [rs(48)>0.70, ps < 0.001] and SHAPS [rs(48)>0.50, ps < 0.001] scores. Both groups also showed a significant positive relationship between anhedonic depression and SHAPS scores [rs(48)>0.48, ps < 0.001]. The control group had a significant positive relationship between SHAPS and anxious arousal [r(48) = 0.39, p = 0.006] scores, but this was not found in the COVID-19 group, p = 0.57.
Table 3
Bivariate correlations between mood, age and acute COVID-19 severity.
COVID | BDI-II | Anxious Arousal | Anhedonic Depression | Positive Affect | Loss of Interest | SHAPS | COVID Severity |
---|---|---|---|---|---|---|---|
Age | -.23 | -.02 | -.19 | .20 | -.12 | .02 | .39![]() ![]() |
BDI-II | – | – | – | – | – | – | .12 |
Anxious Arousal | .32![]() | – | – | – | – | – | .27 |
Anhedonic Depression | .70![]() ![]() ![]() | .31![]() | – | – | – | – | -.01 |
Positive Affect | -.62![]() ![]() ![]() | -.11 | -.94![]() ![]() ![]() | – | – | – | .08 |
Loss of Interest | .60![]() ![]() ![]() | .56![]() ![]() ![]() | .76![]() ![]() ![]() | -.49![]() ![]() ![]() | – | – | .11 |
SHAPS | .50![]() ![]() ![]() | .08 | .48![]() ![]() ![]() | -.54![]() ![]() ![]() | .21 | – | .04 |
CONTROL | BDI-II | Anxious Arousal | Anhedonic Depression | Positive Affect | Loss of Interest | SHAPS | COVID Severity |
---|---|---|---|---|---|---|---|
Age | .01 | -.01 | -.06 | .04 | -.08 | -.01 | N/A |
BDI-II | – | – | – | – | – | – | N/A |
Anxious Arousal | .49![]() ![]() ![]() | – | – | – | – | – | N/A |
Anhedonic Depression | .75![]() ![]() ![]() | .24 | – | – | – | – | N/A |
Positive Affect | -.59![]() ![]() ![]() | -.07 | -.95![]() ![]() ![]() | – | – | – | N/A |
Loss of Interest | .83![]() ![]() ![]() | .54![]() ![]() ![]() | .77![]() ![]() ![]() | -.54![]() ![]() ![]() | – | – | N/A |
SHAPS | .52![]() ![]() ![]() | .39![]() ![]() | .57![]() ![]() ![]() | -.51![]() ![]() ![]() | .50![]() ![]() ![]() | – | N/A |
Asterisks denote statistical significance, () p < 0.05, (
) p < 0.01, (
) p < 0.001. BDI-II = Beck Depression Inventory-II; SHAPS = Snaith–Hamilton Pleasure Scale.
Interestingly, no significant correlations emerged between COVID-19 severity and any self-report measures of mood [all ps > 0.06], suggesting COVID-related illness severity was unrelated to elevated depression or anxiety symptoms. As expected, age was significantly positively correlated with COVID-19 severity [r(48) = 0.39, p = 0.006], but age was not correlated with any of the self-report measures of mood, all ps > 0.49.
Within the control group, ANT conflict scores significantly correlated with scores on the BDI-II [r(48) = 0.41, p = 0.005], MASQ Anxious Arousal [r(48) = 0.31, p = 0.04] and ACS Attention Shifting [r(48) = -0.38, p = 0.04], but not Attention Focusing [r(48) = -0.23, p = 0.25]. These correlations were not significant in the COVID-19 group [all ps > 0.06]; however, Fisher's r-to-z transformations showed that the coefficients were not significantly different from those in the control group [zs < 0.80, ps > 0.42], with the exception of the conflict /BDI-II relationship [z = 2.17, p = 0.03].
4. Discussion
The overarching goal of the current study was to examine long-term mental health sequelae of COVID-19 infection, with a particular focus on depressive symptoms. Three major findings emerged. First, disruptions in mood and cognition were most prominent in the PASC phase, implicating COVID-19-induced pathology that persists beyond acute viral infection. Second, an anhedonic phenotype was observed following COVID-19 infection, marked by reductions in pleasure capacity and positive affect, as well as a loss of interest in previously rewarding activities. Finally, the PASC phase was uniquely associated with a selective impairment in executive functioning, but not attentional orienting or alerting, highlighting specificity of cognitive dysfunction post-COVID infection. Critically, none of these effects were moderated by appraisal of current life stress, nor were they a product of psychiatric impairment that predated the COVID-19 pandemic. Contrary to prior literature in this domain (e.g., Jaywant et al., 2021), our findings were not related to severity of illness or hospitalization; in fact, the overwhelming majority of our sample (98%) was non-hospitalized and effects did not vary by acute illness severity. Of note, our sample was comprised predominantly of adults in their mid-20s and 30s, suggesting PASC might affect a broader demographic than those with the highest risk for poor COVID-19-related health outcomes. Collectively, these factors underscore alarming post-acute consequences of COVID-19 infection that warrant further investigation.
In accordance with Perlis et al. (2021), the current study observed depressive symptoms emerging within 4 months of COVID-19 diagnosis. In this context, the PASC phase might be of relevance to susceptibility factors that contribute to psychiatric sequelae of COVID-19. Very few studies have elucidated the pathophysiology of PASC, but some evidence supports a role for sustained inflammatory responses that contribute to poor mental health outcomes. Indeed, decades of research highlights low-grade inflammation as a strong predictor of long-term depressive symptoms (Zalli et al., 2016), with over half of depressed individuals showing elevated inflammatory markers (Osimo et al., 2019). With respect to COVID-19, significant depression within 2 months of hospital discharge is associated with lymphopenia and elevated plasma C-reactive protein (CRP; Mandal et al., 2021), both of which are markers of increased systemic inflammation. Notably, these biomarkers are not detected in all COVID-19 patients with post-acute depression (Mandal et al., 2021) and there are no changes in CRP levels or lymphocyte counts at 10 weeks post-symptom onset (e.g., Townsend et al., 2020). Interestingly, systemic immune inflammation (indexed by lymphocyte, neutrophil and platelet counts) during acute COVID-19 infection predicts depressive severity one (Mazza et al., 2020) and three (Mazza et al., 2021) months after hospitalization. These findings implicate an incubation period between acute hyperinflammatory reactions and later depression onset. In support of this notion, proinflammatory cytokine blockade during acute COVID-19 infection protects against depression onset at 3-months post-hospitalization (Benedetti et al., 2021). Importantly, the current study does not support prior findings of psychiatric impairment beyond 4 months of COVID-19 diagnosis (e.g., Taquet et al., 2021a). In fact, mood and cognitive dysregulation were resolved within the post-PASC phase (i.e., indistinguishable from controls), further highlighting the specificity of PASC. It remains unclear whether biomarkers, like protracted inflammation, underlie these temporal effects. Future research should assess physiological mechanisms longitudinally to elucidate this phenomenon.
The current study is the first to report profound anhedonic depression after COVID-19 infection, particularly in the acute and PASC phases. Some have postulated that neurological sequelae of COVID-19 could reflect direct actions of SARS-CoV-2 on the brain (Boldrini et al., 2021; Saleki et al., 2020). Indeed, SARS-CoV-2 mRNA has been detected in cerebrospinal fluid (CSF; Moriguchi and Mastorakis, 2020), suggesting the virus could either damage (Welcome et al., 2021) or permeate (Rhea et al., 2021) the blood-brain barrier (BBB). To this extent, some have attributed neuropsychiatric manifestations of COVID-19 to disruptions in the BBB that result in atrophy to neural and glial cells (Alonso-Lana et al., 2020). Of relevance, molecular adaptations of the BBB in mice are associated with morphological changes within the nucleus accumbens (NAc), contributing to sustained anhedonic behaviour (Dudek et al., 2020; Menard et al., 2017). Relatedly, diminished BBB integrity promotes infiltration of peripheral cytokines, namely interleukin (IL)-6, into the CNS (Russo et al., 2018). This is particularly salient in the context of COVID-19-induced hypercytokinemia (i.e., “cytokine storm syndrome”), which is characterized by IL-6 hypersecretion (Del Valle et al., 2020). Extensive animal research underscores a role of IL-6 in reward processing dysregulation (e.g., De La Garza et al., 2005; Rossetti et al., 2016), although the underlying mechanisms of these effects remain unclear. Among psychiatrically healthy adults, pharmacological or stress-induced IL-6 release is linked to decreases in ventral striatal responses to reward (Eisenberger et al., 2010; Treadway et al., 2017). Interestingly, elevated plasma IL-6 is also associated with volumetric decreases in the striatum (Ironside et al., 2020), pointing to its role in long-term morphological alterations to mesolimbic reward circuitry. Collectively, these findings suggest that heightened inflammatory responses from COVID-19 infection could contribute to sustained deficits in reward processing. Further research is needed to shed light on the neuroinvasive potential of SARS-CoV-2 and whether BBB disruptions precede the anhedonic phenotype we observed in COVID-19 survivors.
Distinct cognitive deficits were also observed in the months following COVID-19 infection, corroborating reports of brain fog in COVID-19 survivors (NIH, 2021). Impairment in executive functioning was observed in the PASC phase; however, orienting and alerting abilities remained intact throughout COVID-19 recovery. Our findings support recent literature from Mazza et al. (2021) showing similar executive function impairments three months following COVID-19 hospitalization, which were associated with elevated inflammatory markers during infection. In contrast to the current study, however, these impairments were accompanied by a broad range of cognitive deficits (e.g., psychomotor slowing and verbal fluency impairment; Mazza et al., 2021). This suggests that cognitive deficits in PASC are complex and nuanced, perhaps influenced by factors like acute illness severity (i.e., hospitalization) or the neuropsychological assay used to assess cognition. In support of the latter, the current study did not find impairments in self-reported attentional control, although subjective attentional shifting was associated with task-based executive functioning. Interestingly, executive function deficits in the ANT are seen in unmedicated individuals with MDD, such that conflict processing is slower relative to healthy controls (Sommerfeldt et al., 2016). Similar effects are reported in other tasks that probe executive functioning (e.g., flanker or Stroop; Epp et al., 2012), suggesting overlapping mechanisms might be involved in PASC-induced deficits. Indeed, atypical electrophysiological responses are observed in unmedicated MDD patients during conflict processing (Holmes and Pizzagalli, 2008). Specifically, reduced activity in the dorsal anterior cingulate cortex (dACC) is associated with the largest interference effects (i.e., slower RTs and lower accuracy for incongruent trials; Holmes and Pizzagalli, 2008). Accordingly, isolated frontocingulate dysfunction among COVID-19 survivors may underlie deficits in executive function while other cognitive processes remain unscathed. Some postulate that frontal circuitry is preferentially targeted by SARS-CoV-2, contributing to executive dysfunction (Toniolo et al., 2021). Direct links between frontal lobe abnormalities and cognitive impairment post-COVID-19 are lacking, but there is some evidence of frontal hypometabolism in severe cases (Hosp et al., 2021; Matschke et al., 2020). Research should continue to probe specific elements of cognitive processing, as well as their neural underpinnings, to characterize the breadth of cognitive deficits in PASC.
Findings from the current study should be interpreted in the context of important limitations. Firstly, COVID-19 has been associated with lingering physical ailments (e.g., fatigue, headache) in some individuals, which may be most salient within the acute phase. In the current study, participants reported experiencing symptoms for 17.5 days after first symptom onset, with considerable variability in this time period. Although unlikely, it is possible that psychiatric impairments observed within 14–31 days of diagnosis may be secondary to ongoing post-viral symptoms. This may be uniquely relevant to the Anxious Arousal subscale of the MASQ, which assesses somatic tension and physiological arousal (e.g., lightheadedness, nausea, shortness of breath). In this case, physical symptoms of anxiety may not be dissociable from those of acute COVID-19 infection, confounding the interpretation of these results. Notably, these effects persisted into the PASC phase; however, we cannot rule out the possibility of ongoing physical symptoms at any time post-diagnosis. Given the selective cognitive impairments observed in the COVID-19 group (i.e., normal alerting and orienting abilities), it is less likely that post-acute physical symptoms, such as fatigue, confounded results from the ANT. It is also possible that participants within the control group either had COVID-19-like symptoms and did not receive testing or contracted COVID-19 but were asymptomatic. Future studies could exclude participants if they had experienced any COVID-19 symptoms prior to completing the study.
Contrary to prior literature, our effects were not linked to any particular symptom that was experienced during acute COVID-19 illness. Neurological manifestations have been associated with a loss of olfactory or gustatory function during infection, which is thought to reflect neuroinvasive properties of the virus (Baig, 2020). Specifically, SARS-CoV-2 binds to angiotensin-converting enzyme (ACE)-2 receptors in the olfactory epithelium, invading cells that surround olfactory neurons (Baig and Sanders, 2020). Others have found associations between post-acute depression severity and the presence of headache during infection (Perlis et al., 2021). Although the majority of our sample experienced taste or smell alterations (62%) and headache (68%), these individuals did not show greater psychiatric impairment relative to those who did not experience these symptoms. The current findings suggest that acute COVID-19 symptoms may not reliably predict psychiatric sequelae, necessitating further investigation into relationships between the acute illness experience and PASC.
Based on the purely behavioural nature of the study, precise mechanisms underlying the link between COVID-19 and depression remain unknown. Nevertheless, these findings offer preliminary insights into clinical manifestations of PASC, which appear to parallel the pathophysiology of depression. Future research in this domain could be guided by evidence from prior betacoronaviruses that are genetically similar to SARS-CoV-2. For instance, SARS, which invades the CNS (Xu et al., 2005), produced lasting depressive symptoms in some survivors for several years (for a comprehensive review, see Rogers et al., 2020). Unlike prior coronavirus outbreaks, the COVID-19 pandemic elicited severe disruptions to daily life, which was evident in our control group. In particular, we observed a six-to seven-fold increase in depression scores (as indexed by the BDI-II) relative to what is typically reported in a healthy control group (e.g., Dillon and Pizzagalli, 2013); this occurred despite the absence of lifetime depressive symptoms prior to the COVID-19 pandemic. Thus, effect sizes in the current study may have been greater in the absence of pandemic-related influences and results should be interpreted with consideration of this caveat.
5. Conclusion
Efforts toward elucidating psychiatric sequelae of COVID-19 are becoming increasingly urgent, given the potential for profound public health ramifications. The current study adds to mounting evidence of mood and cognitive impairments following COVID-19 infection, particularly within four months of diagnosis. This study is among the first to shed light on post-acute deficits in reward processing and executive function in COVID-19 survivors, necessitating further investigation into neurobiological underpinnings of PASC. To this end, researchers should continue to focus on COVID-19-specific mechanisms, such as protracted inflammation, that might contribute to prolonged psychiatric consequences. Accordingly, this research will help guide the development of targeted therapies to treat post-acute mental health impairments after recovery from COVID-19.
Declarations of competing interest
SJL, MFW, and MCO declare no competing interests. Over the past 3 years, DAP has received consulting fees from BlackThorn Therapeutics, Boehringer Ingelheim, Compass Pathway, Concert Pharmaceuticals, Engrail Pharmaceuticals, Otsuka Pharmaceuticals, and Takeda Pharmaceuticals; one honorarium from Alkermes, and research funding from the National Institute of Mental Health (NIMH), Dana Foundation, Brain and Behavior Research Foundation, Millennium Pharmaceuticals. In addition, he has received stock options from BlackThorn Therapeutics. No funding from these entities was used to support the current work.
Acknowledgments
This work was supported by a Discovery Grant from the Natural Sciences and Engineering Research Council (NSERC) of Canada to MCO (203707), and by an NSERC Alexander Graham Bell Graduate Scholarship and a Michael Smith Foreign Study Supplement to SJL. DAP was partially supported by R37 MH068376 and R01 MH095809 from the NIMH. The authors thank Dr. Daryl Wilson and Geoffrey Harrison for study design assistance and statistical advice.
References
- Alonso-Lana S., Marquié M., Ruiz A., Boada M. Cognitive and neuropsychiatric manifestations of COVID-19 and effects on elderly individuals with dementia. Front. Aging Neurosci. 2020;12:588872. [Europe PMC free article] [Abstract] [Google Scholar]
- Ardila A., Lahiri D. Executive dysfunction in COVID-19 patients. Diabetes & Metabolic Syndrome. 2020;14(5):1377–1378. [Europe PMC free article] [Abstract] [Google Scholar]
- Baig A.M. Neurological manifestations in COVID-19 caused by SARS-CoV-2. CNS Neurosci. Ther. 2020;26(5):499–501. [Europe PMC free article] [Abstract] [Google Scholar]
- Baig A.M., Sanders E.C. Potential neuroinvasive pathways of SARS-CoV-2: deciphering the spectrum of neurological deficit seen in coronavirus disease-2019 (COVID-19) J. Med. Virol. 2020;92(10):1845–1857. [Europe PMC free article] [Abstract] [Google Scholar]
- Batty G.D., Deary I.J., Luciano M., Altschul D.M., Kivimäki M., Gale C.R. Psychosocial factors and hospitalisations for COVID-19: prospective cohort study based on a community sample. Brain Behav. Immun. 2020;89:569–578. [Europe PMC free article] [Abstract] [Google Scholar]
- Beck A.T., Steer R.A., Brown G.K. The Psychological Corporation, Harcourt Brace; Toronto: 1996. [Google Scholar]
- Benedetti F., Mazza M., Cavalli G., Ciceri F., Dagna L., Rovere-Querini P. Can cytokine blocking prevent depression in COVID-19 survivors? J. Neuroimmune Pharmacol. 2021;16(1):1–3. [Europe PMC free article] [Abstract] [Google Scholar]
- Boldrini M., Canoll P.D., Klein R.S. How COVID-19 affects the brain. JAMA psychiatry. 2021 10.1001/jamapsychiatry.2021.0500. Published online March 26, 2021. [Europe PMC free article] [Abstract] [CrossRef] [Google Scholar]
- Carfì A., Bernabei R., Landi F. Persistent symptoms in patients after acute COVID-19. J. Am. Med. Assoc. 2020;324(6):603–605. [Europe PMC free article] [Abstract] [Google Scholar]
- Centers for Disease Control and Prevention Discontinuation of isolation for persons with COVID-19 not in healthcare settings. 2021. https://www.cdc.gov/coronavirus/2019-ncov/hcp/duration-isolation.html Accessed.
- Chapman L.J., Chapman J.P. The measurement of handedness. Brain Cognit. 1987;6(2):175–183. [Abstract] [Google Scholar]
- Cohen S., Kamarck T., Mermelstein R. A global measure of perceived stress. J. Health Soc. Behav. 1983;24:385–396. [Abstract] [Google Scholar]
- Cortinovis M., Perico N., Remuzzi G. Long-term follow-up of recovered patients with COVID-19. Lancet. 2021;397(10270):173–175. [Europe PMC free article] [Abstract] [Google Scholar] Retracted
- De La Garza R., II Endotoxin-or pro-inflammatory cytokine-induced sickness behavior as an animal model of depression: focus on anhedonia. Neurosci. Biobehav. Rev. 2005;29(4–5):761–770. [Abstract] [Google Scholar]
- Del Valle D.M., Kim-Schulze S., Huang H.H., Beckmann N.D., Nirenberg S., Wang B. An inflammatory cytokine signature predicts COVID-19 severity and survival. Nat. Med. 2020;26(10):1636–1643. [Europe PMC free article] [Abstract] [Google Scholar]
- Derryberry D., Reed M.A. Anxiety-related attentional biases and their regulation by attentional control. J. Abnorm. Psychol. 2002;111(2):225–236. [Abstract] [Google Scholar]
- Dillon D.G., Pizzagalli D.A. Evidence of successful modulation of brain activation and subjective experience during reappraisal of negative emotion in unmedicated depression. Psychiatr. Res. Neuroimaging. 2013;212(2):99–107. [Europe PMC free article] [Abstract] [Google Scholar]
- Dudek K.A., Dion-Albert L., Lebel M., LeClair K., Labrecque S., Tuck E. Molecular adaptations of the blood–brain barrier promote stress resilience vs. depression. Proc. Natl. Acad. Sci. Unit. States Am. 2020;117(6):3326–3336. [Europe PMC free article] [Abstract] [Google Scholar]
- Eisenberger N.I., Berkman E.T., Inagaki T.K., Rameson L.T., Mashal N.M., Irwin M.R. Inflammation-induced anhedonia: endotoxin reduces ventral striatum responses to reward. Biol. Psychiatr. 2010;68(8):748–754. [Europe PMC free article] [Abstract] [Google Scholar]
- El Sayed S., Shokry D., Gomaa S.M. Post-COVID-19 fatigue and anhedonia: a cross-sectional study and their correlation to post-recovery period. Neuropsychopharmacology Reports. 2021;41(1):50–55. [Europe PMC free article] [Abstract] [Google Scholar]
- Epp A.M., Dobson K.S., Dozois D.J., Frewen P.A. A systematic meta-analysis of the Stroop task in depression. Clin. Psychol. Rev. 2012;32(4):316–328. [Abstract] [Google Scholar]
- Fan J., McCandliss B.D., Sommer T., Raz A., Posner M.I. Testing the efficiency and independence of attentional networks. J. Cognit. Neurosci. 2002;14(3):340–347. [Abstract] [Google Scholar]
- Harkness A., Behar-Zusman V., Safren S.A. Understanding the impact of COVID-19 on Latino sexual minority men in a US HIV hot spot. AIDS Behav. 2020;24(7):2017–2023. [Europe PMC free article] [Abstract] [Google Scholar]
- Holmes A.J., Pizzagalli D.A. Response conflict and frontocingulate dysfunction in unmedicated participants with major depression. Neuropsychologia. 2008;46(12):2904–2913. [Europe PMC free article] [Abstract] [Google Scholar]
- Hosp J.A., Dressing A., Blazhenets G., Bormann T., Rau A., Schwabenland M., Meyer P.T. Cognitive impairment and altered cerebral glucose metabolism in the subacute stage of COVID-19. Brain. 2021 10.1093/brain/awab009. Published April 03, 2021. [Europe PMC free article] [Abstract] [CrossRef] [Google Scholar]
- Ironside M., Admon R., Maddox S.A., Mehta M., Douglas S., Olson D.P., Pizzagalli D.A. Inflammation and depressive phenotypes: evidence from medical records from over 12 000 patients and brain morphology. Psychol. Med. 2020;50(16):2790–2798. [Europe PMC free article] [Abstract] [Google Scholar]
- Jaywant A., Vanderlind W.M., Alexopoulos G.S., Fridman C.B., Perlis R.H., Gunning F.M. Frequency and profile of objective cognitive deficits in hospitalized patients recovering from COVID-19. Neuropsychopharmacology. 2021 10.1038/s41386-021-00978-8. Published online February 15, 2021. [Europe PMC free article] [Abstract] [CrossRef] [Google Scholar]
- Kujawa A., Green H., Compas B.E., Dickey L., Pegg S. Exposure to COVID-19 pandemic stress: associations with depression and anxiety in emerging adults in the United States. Depress. Anxiety. 2020;37(12):1280–1288. [Abstract] [Google Scholar]
- Lamontagne S.J., Pizzagalli D.A., Olmstead M.C. Does inflammation link stress to poor COVID-19 outcome? Stress Health. 2020 10.1002/smi.3017. Published online December 14, 2020. [Abstract] [CrossRef] [Google Scholar]
- Mak I.W.C., Chu C.M., Pan P.C., Yiu M.G.C., Chan V.L. Long-term psychiatric morbidities among SARS survivors. Gen. Hosp. Psychiatr. 2009;31(4):318–326. [Europe PMC free article] [Abstract] [Google Scholar]
- Mandal S., Barnett J., Brill S.E., Brown J.S., Denneny E.K., Hare S.S. ‘Long-COVID’: a cross-sectional study of persisting symptoms, biomarker and imaging abnormalities following hospitalisation for COVID-19. Thorax. 2021;76(4):396–398. [Europe PMC free article] [Abstract] [Google Scholar]
- Matschke J., Lütgehetmann M., Hagel C., Sperhake J.P., Schröder A.S., Edler C. Neuropathology of patients with COVID-19 in Germany: a post-mortem case series. Lancet Neurol. 2020;19(11):919–929. [Europe PMC free article] [Abstract] [Google Scholar]
- Mazza M.G., De Lorenzo R., Conte C., Poletti S., Vai B., Bollettini I. Anxiety and depression in COVID-19 survivors: role of inflammatory and clinical predictors. Brain Behav. Immun. 2020;89:594–600. [Europe PMC free article] [Abstract] [Google Scholar]
- McEwen B.S., Sapolsky R.M. Stress and cognitive function. Curr. Opin. Neurobiol. 1995;5(2):205–216. [Abstract] [Google Scholar]
- Menard C., Pfau M.L., Hodes G.E., Kana V., Wang V.X., Bouchard S. Social stress induces neurovascular pathology promoting depression. Nat. Neurosci. 2017;20(12):1752–1760. [Europe PMC free article] [Abstract] [Google Scholar]
- Moriguchi T., Harii N., Goto J., Harada D., Sugawara H., Takamino J. A first case of meningitis/encephalitis associated with SARS-Coronavirus-2. Int. J. Infect. Dis. 2020;94:55–58. [Europe PMC free article] [Abstract] [Google Scholar]
- Mould K.J., Janssen W.J. Signs of self-sustained inflammatory circuits in severe COVID pneumonia. Nature. 2021;590:553–554. [Abstract] [Google Scholar]
- Nalbandian A., Sehgal K., Gupta A., Madhavan M.V., McGroder C., Stevens J.S. Post-acute COVID-19 syndrome. Nat. Med. 2021;27:601–615. [Europe PMC free article] [Abstract] [Google Scholar]
- NIH (2021). Accessed Apr 28 2021. https://www.nih.gov/about-nih/who-we-are/nih-director/statements/nih-launches-new-initiative-study-long-covid.
- Osimo E.F., Baxter L.J., Lewis G., Jones P.B., Khandaker G.M. Prevalence of low-grade inflammation in depression: a systematic review and meta-analysis of CRP levels. Psychol. Med. 2019;49(12):1958–1970. [Europe PMC free article] [Abstract] [Google Scholar]
- Paz C., Mascialino G., Adana-Díaz L., Rodríguez-Lorenzana A., Simbaña-Rivera K., Gómez-Barreno L. Anxiety and depression in patients with confirmed and suspected COVID-19 in Ecuador. Psychiatr. Clin. Neurosci. 2020;74(10):554–555. [Europe PMC free article] [Abstract] [Google Scholar]
- Pizzagalli D.A. Depression, stress, and anhedonia: toward a synthesis and integrated model. Annu. Rev. Clin. Psychol. 2014;10:393–423. [Europe PMC free article] [Abstract] [Google Scholar]
- Puchner B., Sahanic S., Kirchmair R., Pizzini A., Sonnweber B., Wöll E., Mühlbacher A., Garimorth K., Dareb B., Ehling R., Wenter J., Schneider S., Brenneis C., Weiss G., Tancevski I., Sonnweber T., Löffler-Ragg J. Beneficial effects of multi-disciplinary rehabilitation in post-acute COVID-19 – an observational cohort study. Eur. J. Phys. Rehabil. Med. 2021;15:2021. 10.23736/s1973-9087.21.06549-7. Published online January. [Abstract] [CrossRef] [Google Scholar]
- Rhea E.M., Logsdon A.F., Hansen K.M., Williams L.M., Reed M.J., Baumann K.K. The S1 protein of SARS-CoV-2 crosses the blood–brain barrier in mice. Nat. Neurosci. 2021;24(3):368–378. [Europe PMC free article] [Abstract] [Google Scholar]
- Rogers J.P., Chesney E., Oliver D., Pollak T.A., McGuire P., Fusar-Poli P. Psychiatric and neuropsychiatric presentations associated with severe coronavirus infections: a systematic review and meta-analysis with comparison to the COVID-19 pandemic. The Lancet Psychiatry. 2020;7(7):611–627. [Europe PMC free article] [Abstract] [Google Scholar]
- Rossetti A.C., Papp M., Gruca P., Paladini M.S., Racagni G., Riva M.A., Molteni R. Stress-induced anhedonia is associated with the activation of the inflammatory system in the rat brain: restorative effect of pharmacological intervention. Pharmacol. Res. 2016;103:1–12. [Abstract] [Google Scholar]
- Russo S., Menard C., Pfau M., Hodes G., Kana V., Wang V. Social stress induces neurovascular pathology promoting immune infiltration and depression. Biol. Psychiatr. 2018;83(9):S36. [Google Scholar]
- Saleki K., Banazadeh M., Saghazadeh A., Rezaei N. The involvement of the central nervous system in patients with COVID-19. Rev. Neurosci. 2020;31(4):453–456. [Abstract] [Google Scholar]
- Shah A.S., Wong A.W., Hague C.J., Murphy D.T., Johnston J.C., Ryerson C.J., Carlsten C. A prospective study of 12-week respiratory outcomes in COVID-19-related hospitalisations. Thorax. 2021;76(4):402–404. [Abstract] [Google Scholar]
- Snaith R.P., Hamilton M., Morley S., Humayan A., Hargreaves D., Trigwell P. A scale for the assessment of hedonic tone the Snaith–Hamilton Pleasure Scale. Br. J. Psychiatr. 1995;167(1):99–103. [Abstract] [Google Scholar]
- Sommerfeldt S.L., Cullen K.R., Han G., Fryza B.J., Houri A.K., Klimes-Dougan B. Executive attention impairment in adolescents with major depressive disorder. J. Clin. Child Adolesc. Psychol. 2016;45(1):69–83. [Europe PMC free article] [Abstract] [Google Scholar]
- Taquet M., Geddes J.R., Husain M., Luciano S., Harrison P.J. 6-month neurological and psychiatric outcomes in 236 379 survivors of COVID-19: a retrospective cohort study using electronic health records. The Lancet Psychiatry. 2021;8(5):416–427. [Europe PMC free article] [Abstract] [Google Scholar]
- Taquet M., Luciano S., Geddes J.R., Harrison P.J. Bidirectional associations between COVID-19 and psychiatric disorder: retrospective cohort studies of 62 354 COVID-19 cases in the USA. The Lancet Psychiatry. 2021;8(2):130–140. [Europe PMC free article] [Abstract] [Google Scholar]
- Toniolo S., Di Lorenzo F., Scarioni M., Frederiksen K.S., Nobili F. Is the frontal lobe the primary target of SARS-CoV-2? J. Alzheim. Dis. 2021 10.3233/JAD-210008. Published online January 01, 2021. [Abstract] [CrossRef] [Google Scholar]
- Treadway M.T., Admon R., Arulpragasam A.R., Mehta M., Douglas S., Vitaliano G. Association between interleukin-6 and striatal prediction-error signals following acute stress in healthy female participants. Biol. Psychiatr. 2017;82(8):570–577. [Europe PMC free article] [Abstract] [Google Scholar]
- Watson D., Weber K., Assenheimer J.S., Clark L.A., Strauss M.E., McCormick R.A. Testing a tripartite model: evaluating the convergent and discriminant validity of anxiety and depression symptom scales. J. Abnorm. Psychol. 1995;104(1):3–14. [Abstract] [Google Scholar]
- Welcome M.O., Mastorakis N.E. Neuropathophysiology of coronavirus disease 2019: neuroinflammation and blood brain barrier disruption are critical pathophysiological processes that contribute to the clinical symptoms of SARS-CoV-2 infection. Inflammopharmacology. 2021 10.1007/s10787-021-00806-x. Published online April 06, 2021. [Europe PMC free article] [Abstract] [CrossRef] [Google Scholar]
- Xu J., Zhong S., Liu J., Li L., Li Y., Wu X. Detection of severe acute respiratory syndrome coronavirus in the brain: potential role of the chemokine mig in pathogenesis. Clin. Infect. Dis. 2005;41(8):1089–1096. [Europe PMC free article] [Abstract] [Google Scholar]
- Zalli A., Jovanova O., Hoogendijk W.J.G., Tiemeier H., Carvalho L.A. Low-grade inflammation predicts persistence of depressive symptoms. Psychopharmacology. 2016;233(9):1669–1678. [Europe PMC free article] [Abstract] [Google Scholar]
Articles from Brain, Behavior, & Immunity - Health are provided here courtesy of Elsevier
Citations & impact
Impact metrics
Citations of article over time
Alternative metrics

Discover the attention surrounding your research
https://www.altmetric.com/details/113455489
Smart citations by scite.ai
Explore citation contexts and check if this article has been
supported or disputed.
https://scite.ai/reports/10.1016/j.bbih.2021.100347
Article citations
A digital intervention for cognitive deficits following COVID-19: a randomized clinical trial.
Neuropsychopharmacology, 02 Oct 2024
Cited by: 0 articles | PMID: 39358543
Long-Term Impairment of Working Ability in Subjects under 60 Years of Age Hospitalised for COVID-19 at 2 Years of Follow-Up: A Cross-Sectional Study.
Viruses, 16(5):688, 26 Apr 2024
Cited by: 0 articles | PMID: 38793570 | PMCID: PMC11125725
Substantial health and economic burden of COVID-19 during the year after acute illness among US adults not at high risk of severe COVID-19.
BMC Med, 22(1):47, 02 Feb 2024
Cited by: 1 article | PMID: 38302942 | PMCID: PMC10835856
Anxiety and depression symptoms among patients with long COVID: a retrospective cohort study.
Eur Arch Psychiatry Clin Neurosci, 17 Jan 2024
Cited by: 4 articles | PMID: 38231397
Substantial health and economic burden of COVID-19 during the year after acute illness among US adults at high risk of severe COVID-19.
BMC Med, 22(1):46, 01 Feb 2024
Cited by: 2 articles | PMID: 38303065 | PMCID: PMC10836000
Go to all (44) article citations
Similar Articles
To arrive at the top five similar articles we use a word-weighted algorithm to compare words from the Title and Abstract of each citation.
Chronic Disease Self-Management of Post-Acute Sequelae of COVID-19 Among Older Adults: A Mixed-Methods Analysis.
Clin Interv Aging, 18:607-617, 14 Apr 2023
Cited by: 3 articles | PMID: 37082741 | PMCID: PMC10112475
Cognitive functioning in patients with neuro-PASC: the role of fatigue, mood, and hospitalization status.
Front Neurol, 15:1401796, 27 Jun 2024
Cited by: 0 articles | PMID: 38994492 | PMCID: PMC11236596
Outcomes in post-acute sequelae of COVID-19 (PASC) at 6 months post-infection Part 1: Cognitive functioning.
Clin Neuropsychol, 36(4):806-828, 08 Feb 2022
Cited by: 30 articles | PMID: 35130818
Neurological post-acute sequelae of SARS-CoV-2 infection.
Psychiatry Clin Neurosci, 77(2):72-83, 17 Oct 2022
Cited by: 15 articles | PMID: 36148558 | PMCID: PMC9538807
Review Free full text in Europe PMC
Funding
Funders who supported this work.
NIMH NIH HHS (2)
Grant ID: R01 MH095809
Grant ID: R37 MH068376