Abstract
Free full text

Long COVID-19 and Postural Orthostatic Tachycardia Syndrome- Is Dysautonomia to Be Blamed?
Abstract
While the increased arrhythmic tendency during acute COVID-19 infection is recognised, the long-term cardiac electrophysiological complications are less well known. There are a high number of patients reporting ongoing symptoms post-infection, termed long COVID. A recent hypothesis is that long COVID symptoms could be attributed to dysautonomia, defined as malfunction of the autonomic nervous system (ANS). The most prevalent cardiovascular dysautonomia amongst young people is postural orthostatic tachycardia syndrome (POTS). Numerous reports have described the development of POTS as part of long COVID. Possible underlying mechanisms, although not mutually exclusive or exhaustive, include hypovolaemia, neurotropism, inflammation and autoimmunity. Treatment options for POTS and other long COVID symptoms are currently limited. Future research studies should aim to elucidate the underlying mechanisms of dysautonomia to enable the development of targeted therapies. Furthermore, it is important to educate healthcare professionals to recognise complications and conditions arising from COVID-19, such as POTS, to allow prompt diagnosis and access to early treatment.
Introduction
The Hubei Integrated Chinese and Western Medicine hospital in Wuhan reported a clustered point-source outbreak of pneumonia, of unknown viral origin toward the end of December 2019. Within 30 days, the rapid geographic spread of the disease, subsequently called Coronavirus Disease 2019 (COVID-19), implied propagation by human-to-human transmission (1, 2). A pandemic was declared on March 11th 2020, by World Health Organization (WHO) (3). As of November 2021, the disease has caused over 5 million deaths worldwide (4). The disease primarily associated with respiratory pathology, also has well-known cardiac complications, including acute coronary syndrome, myocarditis, heart failure and arrhythmia (5). Indeed, in one cohort of hospitalised COVID-19 patients, heart palpitations were part of the presenting complaint in 7.3% of patients (6). An early study on patients hospitalised with COVID-19 in Wuhan showed the incidence for cardiac arrhythmic events was 17%, rising to 44% in those admitted to the intensive care unit (7). A more recent meta-analysis demonstrated an arrhythmic incidence of 19% in patients with COVID-19, and these patients had an increased risk of poor outcomes (8). While the increased arrhythmic tendency during acute infection is recognised, the long-term cardiac complications are less clear and remain to be reported. The following review is focussed on the associations between long COVID and tachycardia, specifically postural orthostatic tachycardia syndrome (POTS), the potential aetiologies and a discussion on the future direction of research.
Long COVID
Although the symptoms and complications of acute COVID-19 infection are becoming better understood, more research is required to understand the longer-term impact on patients. Mortality rates and hospital admissions have declined due to vaccination rollouts and evolving treatments (9, 10), but there are a high number of patients reporting ongoing symptoms post-infection, termed long COVID (11, 12). For example, one study showed a persistence of symptoms in 87.4% of 143 patients with COVID-19, with fatigue being the most common (53.1%) (13). A prospective cohort study with a larger sample size of 1,733 and longer follow-up showed 76% of patients reported at least one persistent symptom after COVID-19 infection (14). Thus, growing clinical and scientific evidence suggests long-lasting effects of COVID-19 impact multiple organ systems. Symptoms can include palpitations, chronic cough, shortness of breath, chest pain, cognitive dysfunction, fatigue, arthralgia, and overall decline in quality of life (11, 15, 16). Arguably, this is not surprising, as similar patterns have been observed in previous coronavirus infections, such as the severe acute respiratory syndrome (SARS) and Middle East respiratory syndrome (MERS) outbreaks (15). There is clearly a clinical need to understand the pathophysiology behind long COVID, in order to develop targeted therapeutic interventions.
“Post-COVID-19 Tachycardia Syndrome”
From a cardiovascular point of view, recent papers have highlighted an association between orthostatic intolerance (OI), including orthostatic hypotension (OH) and postural orthostatic tachycardia syndrome (POTS), with long COVID (17–19). OH is defined by a systolic or diastolic blood pressure decrease of 20 mmHg or 10 mHg, respectively, within 3 min of standing and it is the most common autonomic manifestation of long COVID (18, 20, 21). Studies suggest the prevalence of OH with long COVID may range between 10% (17) up to 41% (20, 21). Furthermore, tachycardia has been found to be a common symptom associated with long COVID, with 25–50% of patients in a tertiary post-COVID multidisciplinary team clinic reporting persistent tachycardia or palpitations (22). It has been suggested that persistent tachycardia seen in long COVID, labelled “post-COVID-19 tachycardia syndrome,” may present as inappropriate sinus tachycardia or POTS (22). Inappropriate sinus tachycardia occurs when there is a higher heart rate response or faster resting rate than necessary for the current physiological demand (23). At present, there is limited data available on this in the context of long COVID. POTS is defined as a persistent increase in heart rate of at least 30 beats per minute within 10 min of standing and this can present with symptoms of palpitations, chest pain and exercise or orthostatic intolerance (24). Numerous reports have described the development of POTS as part of long COVID (19, 25–27) although the aetiology remains debated.
Mechanisms of Dysautonomia Leading to Postural Orthostatic Tachycardia Syndrome
A recent hypothesis is that long COVID symptoms could be attributed to dysautonomia (16, 28). Dysautonomia can be defined as malfunction of the autonomic nervous system (ANS) (29), and it can be acute or chronic and progressive or reversible (28). Many symptoms can manifest from dysautonomia, including fatigue, heart rate variability (HRV) dysfunction and orthostatic hypotension (28). The most prevalent cardiovascular dysautonomia amongst young people is postural orthostatic tachycardia syndrome (POTS) (30). The interaction of the autonomic nervous system and cardiac rhythm abnormalities has been previously described (31) but there remains limited data on long COVID, dysautonomia and the aetiology of POTS. Possible underlying mechanisms, although not mutually exclusive or exhaustive, include hypovolaemia, neurotropism, inflammation and autoimmunity (Figure 1) (24, 28).
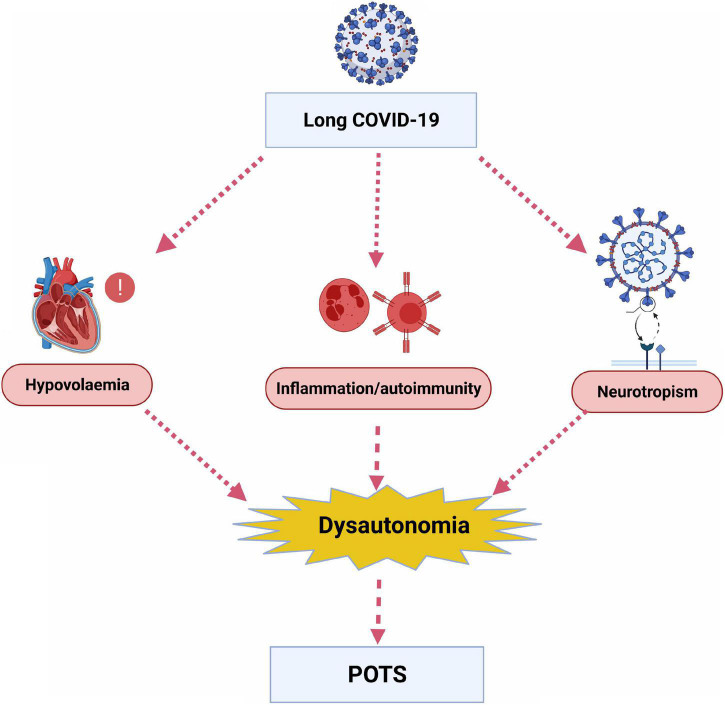
Potential underlying mechanisms by which long COVID leads to dysautonomia and postural orthostatic tachycardia syndrome (POTS). (Created with BioRender.com).
Hypovolaemia
Firstly, it has been suggested that POTS occurs due to decreased blood volume and deconditioning of the heart, which subsequently increases cardiac sympathetic nervous system (SNS) output (24, 32, 33). COVID-19 can be associated with fever and nocturnal sweating, leading to hypovolaemia, and prolonged bed rest, leading to deconditioning and cardiac atrophy. Furthermore, it has been shown that patients with POTS had a lower plasma volume compared to controls (34). The rein-angiotensin-aldosterone system (RAAS) may have a role in the aetiology of POTS as despite lower plasma volumes, it has been shown that patients with POTS had lower levels of plasma renin and aldosterone (34–36). This RAAS deficit could be due to partial renal sympathetic denervation (34). SARS-CoV-2 has been implicated in binding and down-regulating the ACE2 receptor (37), and introducing a RAAS imbalance (38). It has also been proposed that SARS-CoV-2 infection causes a deficit of cellular furin, leading to dysfunction of the epithelial sodium channel (ENaC) and changes in fluid balance that activates the RAAS (39).
Thus, the persistent tachycardia is a physiological compensation against the smaller stroke volume of the deconditioned heart (40) and those that are more predisposed to the condition have smaller hearts (32). Interestingly, POTS is observed in patients with chronic fatigue syndrome (41) and a prevent study demonstrated many patients with chronic fatigue syndrome had small hearts (42).
Neurotropism
Secondly, a suggested and more direct cause of POTS could be related to the spread of COVID-19 to the central nervous system (CNS) by neural propagation, causing direct damage on nerves or indirect damage via a cytokine storm (28). Cerebrospinal spinal fluid (CSF) in patients with acute COVID-19 have shown the presence of the virus (37) and PET scans have shown hypometabolism in the olfactory bulb as well as extension to other brain areas at the post-viral stage of COVID-19 disease (43). Similar to other coronaviruses, the COVID-19 virus binds to the ACE2 receptor on host membranes by using a spike protein S1 (37). ACE2 receptors are highly expressed in the olfactory epithelium and thus a proposed propagation route is via the nose through the cribriform plate of the ethmoid bone to the olfactory epithelium (43), which could be responsible for the hyposmia observed with COVID-19 infection. Thus, it has been proposed that SARS-Cov2 could infect and damage extracardiac postganglionic SNS, leading to dysautonomia in a similar way to neuropathic POTS. This disruption could lead to tachycardia due to splanchnic venous pooling or reduced mesenteric vasoconstriction during orthostasis (24).
Inflammation and Autoimmunity
Thirdly, heart rate variability (HRV) can be used as a marker of dysautonomia (44) and HRV has been shown to have an inverse relationship to inflammation in a recent meta-analysis (45). In addition, the role for the autoimmune system and inflammation in the aetiology of POTS is supported by the increased antibodies and incidence of autoimmune conditions seen in patients with POTS compared to controls (46). COVID-19 infection can cause a cytokine storm and inflammation that could trigger chronic neuronal dysregulation (47). One mechanism could be via the development of autoantibodies which cross-react with autonomic ganglia as part of acute and long-term COVID-19 infection, and lead to dysautonomia and POTS. Li et al. showed patients with POTS had high levels of autoantibodies which cross-reacted with muscarinic and beta receptors (48). The role of the immune system in dysautonomia and long COVID-19 could have implications for treatment options as 75% of patients with POTS were positive for α3-AChR antibodies that could respond to immunotherapy (49).
Management of Postural Orthostatic Tachycardia Syndrome
Currently, conservative treatment options include graduated exercise programmes, avoiding triggers, high fluid and salt intake, compression stockings and cognitive behavioural therapy (50, 51). Pharmacological options are generally unlicensed for this indication but aim to correct several physiological parameters. These include agents, such as fludrocortisone, erythropoietin and desmopressin, to increase blood volume, β-blockers or ivabradine to reduce tachycardia, midodrine, methylphenidate or octreotide to induce vasoconstriction and pyridostigmine to facilitate synaptic transmission (34, 50, 52). Evidently, further research is needed to elucidate the underlying mechanisms of dysautonomia to direct targeted treatment options. Indeed, in the absence of novel therapies, it is a viable option to repurpose drugs (53) once a better mechanistic understanding of long COVID and dysautonomia is established.
Central to the management of POTS is prompt recognition as the correct diagnosis of POTS can be delayed by several years (54). Unfortunately, POTS is often misdiagnosed as patients present with many non-specific symptoms, and the significance of the symptoms, such as asymptomatic tachycardia in an otherwise well patient, may not be appreciated. From a physiological perspective, persistent tachycardia will inevitably lead to remodelling of the heart and predispose to cardiac failure in the future. Thus, persistent tachycardia as a sole symptom still warrants consideration of further investigation and treatment. The impact of long COVID-19 on the incidence cardiac failure secondary to persistent tachycardia remains to be seen but should be considered in future research studies.
Conclusion
Unfortunately, given the number of people infected with COVID-19, there is likely to be an emergence of various long COVID-19 syndromes presenting to both primary and secondary care, such as patients with POTS. Thus, there is clearly a need for future studies to investigate the cause of long COVID-19 symptoms. One avenue would be to better understand the aetiology of dysautonomia to enable the development of therapies that specifically target the underlying pathology. Meanwhile, it is important to educate healthcare professionals to recognise complications and conditions arising from COVID-19, such as POTS, to allow prompt diagnosis and referral to enable early treatment access.
Author Contributions
KC and EB wrote the first draft and review all subsequent draft. CH reviewed all drafts and supervised. KJ developed the idea, reviewed all drafts, and supervised. All authors contributed to the article and approved the submitted version.
Conflict of Interest
The authors declare that the research was conducted in the absence of any commercial or financial relationships that could be construed as a potential conflict of interest.
Publisher’s Note
All claims expressed in this article are solely those of the authors and do not necessarily represent those of their affiliated organizations, or those of the publisher, the editors and the reviewers. Any product that may be evaluated in this article, or claim that may be made by its manufacturer, is not guaranteed or endorsed by the publisher.
References
Articles from Frontiers in Cardiovascular Medicine are provided here courtesy of Frontiers Media SA
Full text links
Read article at publisher's site: https://doi.org/10.3389/fcvm.2022.860198
Read article for free, from open access legal sources, via Unpaywall:
https://www.frontiersin.org/articles/10.3389/fcvm.2022.860198/pdf
Citations & impact
Impact metrics
Citations of article over time
Alternative metrics

Discover the attention surrounding your research
https://www.altmetric.com/details/124440504
Article citations
Navigating Complexity in Postural Orthostatic Tachycardia Syndrome.
Biomedicines, 12(8):1911, 20 Aug 2024
Cited by: 0 articles | PMID: 39200375 | PMCID: PMC11352109
Review Free full text in Europe PMC
Long COVID science, research and policy.
Nat Med, 30(8):2148-2164, 09 Aug 2024
Cited by: 4 articles | PMID: 39122965
Review
Combining Double-Dose and High-Dose Pulsed Dapsone Combination Therapy for Chronic Lyme Disease/Post-Treatment Lyme Disease Syndrome and Co-Infections, Including Bartonella: A Report of 3 Cases and a Literature Review.
Microorganisms, 12(5):909, 30 Apr 2024
Cited by: 0 articles | PMID: 38792737 | PMCID: PMC11124288
The knowns and unknowns of long COVID-19: from mechanisms to therapeutical approaches.
Front Immunol, 15:1344086, 04 Mar 2024
Cited by: 5 articles | PMID: 38500880 | PMCID: PMC10944866
Review Free full text in Europe PMC
Potential Alternative Receptors for SARS-CoV-2-Induced Kidney Damage: TLR-4, KIM-1/TIM-1, and CD147.
Front Biosci (Landmark Ed), 29(1):8, 01 Jan 2024
Cited by: 0 articles | PMID: 38287815 | PMCID: PMC10924798
Review Free full text in Europe PMC
Go to all (27) article citations
Similar Articles
To arrive at the top five similar articles we use a word-weighted algorithm to compare words from the Title and Abstract of each citation.
Postural orthostatic tachycardia syndrome as a sequela of COVID-19.
Heart Rhythm, 19(11):1880-1889, 16 Jul 2022
Cited by: 44 articles | PMID: 35853576 | PMCID: PMC9287587
Review Free full text in Europe PMC
Post-acute sequelae of SARS-CoV-2 syndrome presenting as postural orthostatic tachycardia syndrome.
Clin Exp Emerg Med, 10(1):18-25, 30 Jan 2023
Cited by: 2 articles | PMID: 36718484 | PMCID: PMC10090716
Review Free full text in Europe PMC
Impact of COVID-19 infection on baseline autonomic symptoms in patients with preexisting postural tachycardia syndrome and orthostatic intolerance: A retrospective study.
Am J Med Sci, 367(5):323-327, 08 Feb 2024
Cited by: 0 articles | PMID: 38340983
Postural orthostatic tachycardia syndrome and other related dysautonomic disorders after SARS-CoV-2 infection and after COVID-19 messenger RNA vaccination.
Front Neurol, 14:1221518, 16 Aug 2023
Cited by: 1 article | PMID: 37654428 | PMCID: PMC10467287
Review Free full text in Europe PMC