Abstract
Free full text

Association of EWS-FLI1 Type 1 Fusion with Lower Proliferative Rate in Ewing’s Sarcoma
Abstract
The Ewing’s sarcoma (ES) family of tumors, including peripheral neuroectodermal tumor (PNET), is defined genetically by specific chromosomal translocations resulting in fusion of the EWS gene with a member of the ETS family of transcription factors, either FLI1 (90–95%) or ERG (5–10%). A second level of molecular genetic heterogeneity stems from the variation in the location of the translocation breakpoints, resulting in the inclusion of different combinations of exons from EWS and FLI1 (or ERG) in the fusion products. The most common type of EWS-FLI1 fusion transcript, type 1, is associated with a favorable prognosis and appears to encode a functionally weaker transactivator, compared to other fusion types. We sought to determine whether the observed covariation of structure, function, and clinical course correlates with tumor cell kinetic parameters such as proliferative rate and apoptosis, and with expression of the receptor for insulin-like growth factor I (IGF-1R). In a group of 86 ES/PNET with defined EWS-ETS fusions (45 EWS-FLI1 type 1, 27 EWS-FLI1 non-type 1, 14 EWS-ERG), we assessed proliferation rate by immunostaining for Ki-67 using MIB1 antibody (n = 85), apoptosis by TUNEL assay (n = 66), and IGF-1R expression by immunostaining with antibody 1H7 (n = 78). Ki-67 proliferative index was lower in tumors with EWS-FLI1 type 1 than those with non-type 1 EWS-FLI1, whether analyzed as a continuous (P = 0.049) or categorical (P = 0.047) variable. Logistic regression analysis suggests that this association was secondary to the association of type 1 EWS-FLI1 and lower IGF-1R expression (P = 0.04). Comparing EWS-FLI1 to EWS-ERG cases, Ki-67 proliferative index was higher in the latter (P = 0.01, Mann-Whitney test; P = 0.02, Fisher’s exact test), but there was no significant difference in IGF-1R. TUNEL results showed no significant differences between groups. Our results suggest that clinical and functional differences between alternative forms of EWS-FLI1 are paralleled by differences in proliferative rate, possibly mediated by differential regulation of the IGF-1R pathway.
The Ewing’s sarcoma (ES) family of tumors, including peripheral neuroectodermal tumor (PNET), represents a clinicopathological entity with a variable neural differentiation, usually appearing as a bone or soft tissue lesion in a child or young adult. The primary genetic event is chromosomal translocation resulting in fusion of the EWS gene with a member of the ETS family of transcription factors. 1 The most frequent translocation partner of EWS is FLI1 (90–95%), followed by ERG (5–10%). These fusion products function as oncogenic aberrant transcription factors. 2 Detection of these fusions is considered to be specific for ES/PNET, and has become a valuable tool for the differential diagnosis of primitive small round cell tumors. 3,4
There is a considerable molecular genetic heterogeneity within ES/PNET. As mentioned above, either FLI1 or ERG can rearrange with EWS in these gene fusions. Furthermore, for either gene fusion, additional heterogeneity stems from the location of the genomic breakpoints of the translocation, resulting in different combinations of exons from EWS and FLI1 (or ERG). 5 The most common fusion joins EWS exon 7 in frame with FLI1 exon 6 (type 1 fusion). There are at least 12 other EWS-FLI1 types, and at least 4 types of EWS-ERG fusion types described. We and others 6 have previously reported that among patients with EWS-FLI1 fusions, those having type 1 transcripts have a better outcome regardless of conventional prognostic factors such as stage, age, or tumor location. 7
The biological mechanisms underlying this reproducible and significant association of fusion structure and clinical behavior are unclear. As a first clue to how these variables may be linked, we have recently found that the EWS-FLI1 type 1 fusion may encode a transcription factor with lower transactivation efficiency than other EWS-FLI1 fusion types. 8 Because studies using various approaches to inhibit EWS-FLI1 function have shown that it is a critical determinant of proliferation in ES/PNET cell lines, 9-11 we have now sought to determine whether the observed covariation of structure, function, and clinical course extends to tumor cell kinetic parameters such as proliferative rate and apoptosis. We have also examined expression of the receptor for insulin-like growth factor 1 (IGF-1R), because there is evidence from several groups implicating it in autocrine or paracrine control of ES/PNET growth. 12-15 The results of our analysis, described below, are consistent with the notion that type 1 EWS-FLI1, functionally a weaker transcription factor, may result in lesser activation of direct or indirect target genes, possibly including IGF-1R, controlling proliferative rate in ES/PNET.
Materials and Methods
Patients
We studied 86 patients with a histopathological diagnosis of ES/PNET and molecular evidence of the EWS-FLI1 or EWS-ERG fusion transcripts, 66 from the Memorial Sloan-Kettering Cancer Center (MSKCC), 14 from Clínica Universitaria de Navarra (CUN), and 6 from the University of Pennsylvania Medical Center/Children’s Hospital of Philadelphia (UPMC/CHOP). Of 72 cases with the EWS-FLI1 fusion, 54 were included in our previous study. 7 The 14 EWS-ERG cases, consisting of 7 MSKCC cases, 6 UPMC/CHOP cases, and 1 case from CUN, were also included in another study. 16 EWS-ERG cases were actively sought and therefore their proportion in the overall series (16%) is not representative of their actual prevalence. There were 55 males and 31 females. The mean age was 23 years (range, 5–72 years). Primary tumor dimensions were available in 63 patients. Volume calculations for bony (spheroidal) and soft tissue (spherical) tumors were performed as described elsewhere. 17 Mean and median tumor volumes were, respectively, 456 ml and 72 ml.
Immunohistochemical and Apoptosis Analysis
All immunohistochemical and apoptosis analyses were done on 4-μm sections of formalin-fixed, paraffin-embedded, non-decalcified tumor. In 80 cases, prechemotherapy primary tumor was available for study, and in the remaining 6 cases metastases were used as the source of tumor. Immunohistochemistry was performed on an automated immunostainer (TechMate 500; Dako, Copenhagen, Denmark) with the EnVision+ system (Dako), in which the secondary antibody is coupled to a dextran polymer linked to peroxidase. Endogenous peroxidase activity was quenched by treatment with 5% hydrogen peroxide in methanol for 30 minutes at room temperature. Antigen retrieval by microwave treatment for 20 minutes in an 800W microwave oven was performed. A blocking step with normal rabbit serum was used. Primary antibodies were applied for 120 minutes at room temperature. Primary antibodies and dilutions were as follows: Ki-67 (MIB1 antibody, Zymed, South San Francisco, CA) 1/800 with microwave antigen retrieval, IGF-1R (1H7 antibody, RDI, Flanders, NJ) 1/1200 with microwave retrieval and trypsinization.
The sections were then rinsed with washing buffer at room temperature. The next step was addition of EnVision+ system reagents and incubation 30 minutes at room temperature. The slides were rinsed with washing buffer, and treated with a solution containing 0.05% diaminobenzidine hydrochloride and 0.1% hydrogen peroxide in 0.05 mol/L Tris-buffered saline, pH 7.4, at room temperature for 5 minutes. After rinsing in distilled water for 3 minutes, the slides were counterstained with modified Harris hematoxylin, dehydrated, and mounted. For negative controls, incubation with normal goat serum instead of primary antibody was used. Omission of the primary antibody likewise gave no background staining.
In situ detection of apoptosis by use of the terminal deoxynucleotidyl transferase-mediated dUTP nick-end labeling (TUNEL) technique was performed as previously described using the ApopTag In Situ Apoptosis Detection Kit (Intergen, Gaithersburg, MD) that identifies cells with internucleosomal fragmentation of DNA. Sections from selected formalin-fixed, paraffin-embedded tissue blocks were placed on coated slides. Briefly, tissue sections were dewaxed and rehydrated routinely. After rehydration, the slides were incubated with proteinase K (20 μg/ml) at room temperature for 15 minutes, per the manufacturer’s protocol. Endogenous peroxidase was inactivated by 3% hydrogen peroxide. Tissue sections were then subjected to the ApopTag reaction. The reaction was terminated after 60 minutes by transfer of the slides to a coplin jar containing pre-warmed working strength stop-wash buffer and incubated for 30 minutes at 37°C. The sections were then covered with 55 μl of anti-digoxigenin-peroxidase and incubated for 30 minutes at room temperature, and stained with diaminobenzidine to detect the labeled nuclei. For negative controls, deionized water was used instead of terminal deoxynucleotidyl transferase. Positive controls consisted of inflamed human tonsil and acute myocardial infarction. Cells were considered positive when brown reactivity was detected in the nuclei.
Results of immunohistochemical and apoptosis assays were evaluated by two independent observers (E. de A. and A. P.) blinded to clinicopathological and molecular data. Tissue sections were examined under high power magnification (×400). The number of immunoreactive cells was counted on three randomly selected fields, and expressed as a percentage of cells. In tumors with heterogeneous distribution of the immunoreactivity, four areas with the highest and the lowest number of reactive cells were counted and median value was reported. Cases in which discrepancy between observers was >15% were reviewed and a consensus was reached.
Molecular Study
The fusion transcripts in all but 18 cases had been characterized in previous studies. 7,16 Reverse transcriptase-polymerase chain reaction (RT-PCR) for EWS-FLI1 and EWS-ERG transcripts was performed on total RNA extracted from snap frozen tumor samples. EWS-FLI1 type 1 (7–6 fusion) and type 2 (7–5 fusion) products were typed according to the size of the RT-PCR products on agarose gel electrophoresis. Other less common fusion products were either blotted and probed with internal and junction-specific probes, or sequenced. EWS-ERG fusion transcripts were not subtyped for this analysis. Negative controls lacking either tumor RNA or reverse transcriptase were routinely used.
Statistical Analysis
Immunoreactivities with the different antibodies and apoptosis results were assessed as continuous variables. Because number of immunoreactive cells did not follow a normal distribution, we used Mann-Whitney nonparametric tests to compare qualitative (gene fusion type) with quantitative parameters (immunoreactivity). Logistic regression was used to study the univariate prognostic value of each factor for predicting proliferation, measured as Ki-67 index below or above the median value (15%). Factors with a univariate P value of not more than 0.2 for predicting Ki-67 index were considered in a multivariate logistic regression. The two factors included in final logistic regression, EWS-FLI1 transcript type, and IGF-1R expression were chosen to give the best two-factor model among all of the factors considered. All analyses were performed using SPSS statistical package, version 6.0 for Windows (SPSS, Inc. Chicago, IL). All P values were two-tailed.
Results
Immunoreactivity for Ki-67 obtained with the MIB1 antibody had a nuclear pattern with strong nucleolar staining (Figure 1) . Immunostaining for Ki-67 was most frequently seen in nuclei of dark cells, that seldom showed TUNEL reactivity. In ES/PNET, cells with hyperchromatic nuclei (dark cells) are usually seen along with cells having clear nuclei. They have traditionally been regarded as apoptotic cells. 18 Interestingly, nuclei reactive with the TUNEL technique were usually those of clear cells, whereas those of dark cells appeared positive less often. This pattern of TUNEL reactivity in some clear cells could represent an early stage of apoptosis, because the characteristic morphological appearance of apoptotic nuclei is a later event in the apoptotic process. Membranous staining was seen with the antibody to IGF-1R (Figure 2)
. Most cases showed widespread IGF-1R immunoreactivity and all cases contained at least some positive cells.
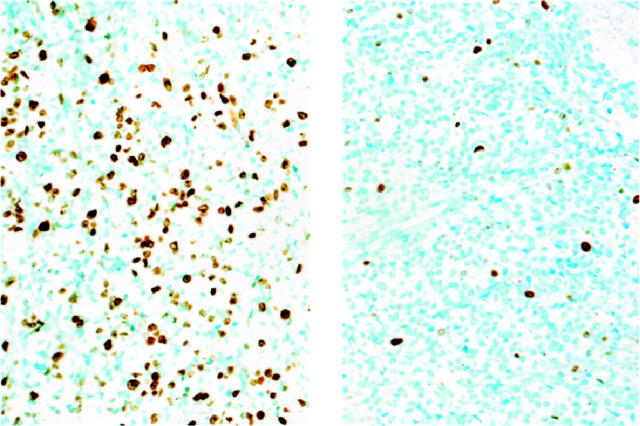
Ki-67 proliferation rate assesed by MIB1 immunohistochemistry in ES/PNET. Left panel shows ES/PNET case with non-type 1 EWS-FLI1 and high Ki-67. Right panel shows ES/PNET case with type 1 EWS-FLI1 and low Ki-67.
The mean and median percentages of tumor cells positive for these markers were respectively as follows: Ki-67 (n = 85) 21% and 14%, IGF-1R (n = 78) 73% and 80%, and TUNEL (n = 66) 5% and 2%. There was a significant positive correlation between proliferative index as assessed by immunostaining for Ki-67, and IGF-1R immunoreactivity (Spearman correlation coefficient, r = 0.23; P = 0.04). All cases with Ki-67 immunoreactivity in >20% of tumor cells had IGF-1R expression in 50% or more of cells (Figure 3) . Conversely, all cases with <40% IGF-1R-positive cells had a Ki-67 proliferative index of 10% or less.
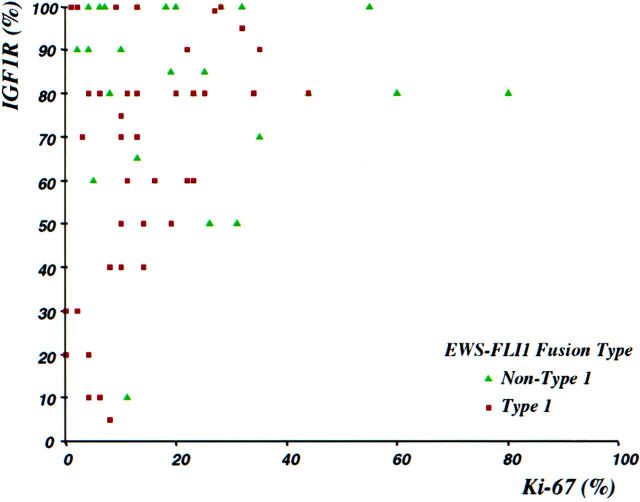
Scattergram showing correlation between Ki-67 (MIB1) and IGF-1R immunoreactivities. Significant Ki-67 immunoreactivity (>10%) was only present when over 50% of cells show expression of IGF-1R. Tumors having non-type 1 EWS-FLI1 fusions (green triangles) had significantly higher values of IGF-1R and Ki-67 (see Tables 1 and 2
).
There were 72 tumors with EWS-FLI1 transcripts: 44 type 1 fusions and 28 grouped into the non-type 1 group. Fourteen tumors had EWS-ERG fusions. Patients having EWS-FLI1 fusions had similar age, stage at diagnosis, and tumor volume with respect to those bearing EWS-ERG fusion genes (results not shown).
In the group of tumors with EWS-FLI1 transcripts, those having type 1 fusions had a lower immunoreactivity for Ki-67 (15%; P = 0.049), and for IGF-1R (65%; P = 0.015) than tumors with other EWS-FLI1 fusion types (24% and 82%, respectively) by Mann-Whitney test (Table 1) . In aggregate, tumors with EWS-FLI1 transcripts had lower values for Ki-67 (P = 0.01) than those with EWS-ERG fusions by Mann-Whitney test (Table 1)
.
Table 1.
Comparison of TUNEL, Ki-67, and IGF-1R Results as Continuous Variables
Parameter | EWS-FLI1 type 1 | EWS-FLI1 non-type 1 | P value* | EWS-FLI1 (all types) | EWS-ERG | P value* |
---|---|---|---|---|---|---|
TUNEL | 2.4 ± 0.4 | 5.3 ± 3.6 | 0.49 | 3.5 ± 1.4 | 10.1 ± 5.9 | 0.3 |
(n = 66) | ||||||
Ki-67 | 15 ± 2 | 24 ± 4 | 0.049 | 18 ± 2 | 35 ± 8 | 0.01 |
(n = 85) | median, 12 | median, 20 | median, 13 | median, 23 | ||
IGF-1R | 65 ± 5 | 82 ± 4 | 0.015 | 72 ± 3 | 79 ± 5 | 0.58 |
(n = 78) | median, 73 | median, 85 | median, 80 | median, 80 |
*Mann-Whitney test.
Values correspond to mean percentage value of immunoreactive cells ± SE.
We then analyzed Ki-67 proliferation index as a categorical variable by using 15%, essentially the median Ki-67 value in the entire study group (see above), to delineate low and high proliferation subgroups. By Fisher’s exact test, both the type 1 versus non-type 1 EWS-FLI1 comparison (P = 0.047; Table 2 ) and the EWS-FLI1 versus EWS-ERG comparison (P = 0.02; Table 3
) remained statistically significant.
Table 2.
Comparison of Ki-67 Results as Categorical Variables, EWS-FLI1 Type 1 vs. EWS-FLI1 Non-Type 1
Category | EWS-FLI1 type 1 | EWS-FLI1 non-type 1 |
---|---|---|
Ki-67 < 15% | 30 | 11 |
Ki-67 > 15% | 15 | 16 |
P = 0.047, Fisher’s exact test.
Values correspond to number of cases.
Table 3.
Comparison of Ki-67 Results as Categorical Variables, EWS-FLI1 vs. EWS-ERG
Category | EWS-FLI1* | EWS-ERG* |
---|---|---|
Ki-67 < 15% | 41 | 3 |
Ki-67 > 15% | 31 | 11 |
P = 0.02, Fisher’s exact test.
Values correspond to number of cases.
*all types.
To further dissect the relative contributions of these variables to proliferation index in ES/PNET, we performed a logistic regression analysis of factors determining Ki-67. In an analysis including EWS-FLI1 type 1 versus non-type 1 and IGF-1R, the latter remained significant (P = 0.049), but EWS-FLI1 transcript type was no longer significant (P = 0.3), suggesting that the impact of the latter on Ki-67 values may be due largely to its correlation with IGF-1R expression.
No significant correlations were seen between Ki-67 or transcript type and stage at diagnosis or tumor volume. Tumors metastatic at diagnosis were larger than those presenting with localized disease only (1390 ml vs. 242 ml respectively; P = 0.001). No other correlations were identified among the parameters studied. There were no significant differences in the proportion of apoptotic cells, as detected by the TUNEL assay, among the groups.
Discussion
The consistent involvement of transcription factors in the gene fusions observed in many small cell sarcomas suggests a central role for these proteins in the regulation of proliferation and differentiation of mesenchymal cells. 19 EWS-FLI1 is an aberrant transcription factor, and experimental evidence suggests that EWS-FLI1 initiates and maintains tumorigenicity in ES/PNET. 2 Indeed, specific inactivation of EWS-FLI1 in ES/PNET cell lines results in reduced proliferation and loss of tumorigenicity. 9-11
Ki-67 is expressed in late G1, S, G2, and M phases, and hence correlates closely with growth fraction in all tumor types. 20 Although its function is not well known, nuclear overexpression of Ki-67 has been associated with tumor progression and poor prognosis in many different tumors, including sarcomas. 21-24 There have been few systematic studies of proliferation markers in ES/PNET. Our median Ki-67 value with the MIB1 antibody (14%) was slightly higher than the range of proliferative rates previously reported for this tumor (7–10%) using other techniques. 25-28 The significant positive correlation between proliferative rate and IGF-1R expression level (P = 0.04) found in the present study is consistent with the known cellular biology of IGF-1R 29,30 and supports the validity of the immunohistochemical methods used to evaluate these two parameters.
Our Ki-67 data, whether analyzed as continuous or categorical variables, suggest that tumors with EWS-FLI1 type 1 have, on average, a lower proliferative rate than ES/PNET with non-type 1 EWS-FLI1. Our logistic regression analysis further suggests that the partial association of type 1 EWS-FLI1 and lower IGF-1R expression may account at least in part for the association of Ki-67 and EWS-FLI1 fusion type.
IGF-1R is widely expressed by proliferating cells throughout development. 29,30 IGF-1 and its receptor, IGF-1R, are expressed in many human tumors, 29,30 including various sarcomas. 31 In at least some ES/PNET, they appear to constitute a functional autocrine or paracrine loop. 12,14 Blockade of IGF-1R stops proliferation and induces apoptosis in ES/PNET. 12-14 Consistent with these studies, we found a significant positive correlation in ES/PNET between Ki-67 proliferative index and the proportion of cells expressing IGF-1R. Tumors with EWS-FLI1 Type 1 fusions had significantly fewer cells immunoreactive for IGF-1R. The simplest model accounting for these associations of fusion type, IGF-1R expression, and proliferative rate would suggest that the IGF-1R gene is a direct or indirect target of EWS-FLI1 and therefore subject to differential regulation by alternative forms of this aberrant transcription factor. 8 Few regulatory targets of EWS-FLI1 or native FLI1 have so far been identified. 32-35 There are contradictory data on IGF-1R regulation by EWS-FLI1. In a study recently reported in abstract form, Shum et al 36 found IGF-1R gene expression to be decreased following induction of EWS-FLI1 in a human embryonal kidney cell line (EcR293). On the other hand, Toretsky et al reported no significant difference in endogenous IGF-1R protein levels in mouse fibroblast cell lines with or without stably transfected human EWS-FLI1, 37 leading them to conclude that IGF-1R expression is not regulated by the fusion protein. Whether these experimental models are representative of IGF-1R regulation in ES/PNET is unclear, however. The human and murine IGF-1R promoter regions are not identical, and mouse fibroblasts, human embryonal kidney cells, and human ES/PNET cells may differ in cell type-specific transcriptional cofactors.
We found all cases to show at least some IGF-1R expression by immunohistochemistry. This is consistent with its ubiquity at the mRNA or protein level in ES/PNET cell lines or clinical samples as shown by others. 12,14 Evidence that IGF-1R expression is required for EWS-FLI1 transformation of mouse fibroblasts 37 suggests that in vivo, this fusion protein may have a similar requirement for a IGF-1R-positive precursor cell or for induction of the IGF-1R pathway. Although essentially all cases showed at least some IGF-1R expression, its level could be critical for maintaining cell proliferation in ES/PNET, because only cases with >40% of cells expressing IGF-1R had a significant Ki-67 immunoreactivity (>10%; see Figure 3 ). The differences in extent of IGF-1R immunoreactivity between type 1 and non-type 1 EWS-FLI1 groups, although statistically significant (P = 0.015), were relatively small (65% vs. 82%). Nonetheless, there is experimental evidence that small differences in IGF-1R expression level per cell can effectively modulate cell proliferation. 38
We have recently reported in a partially overlapping series that tumors with EWS-ERG fusions have a similar clinical presentation and behavior as those with EWS-FLI1 chimeric genes. 16 It may thus be unexpected that there was a difference in proliferative index, as assessed by Ki-67 immunoreactivity, between these two groups. No differences in IGF-1R expression were seen between EWS-FLI1 and EWS-ERG cases, suggesting that the observed difference in proliferative rate could be due to differential activation of other target pathways. The extent to which activation of downstream targets may differ quantitatively or qualitatively between EWS-FLI1 and EWS-ERG is unknown. Indeed, outside their DNA-binding domains, FLI1 and ERG show major sequence differences which could be significant in the protein-protein interactions involved in the regulation of certain promoters. It is therefore not unreasonable to consider the possibility that EWS-FLI1 and EWS-ERG may deregulate some partly non-overlapping pathways to generate clinical phenotypes which are so far indistinguishable.
Because both EWS-FLI1 and EWS-ERG fusion proteins have been shown to inhibit apoptosis in model systems, 39 we were interested in comparing relative numbers of apoptotic cells in these pretreatment clinical samples. However, the numbers of apoptotic cells shown by the TUNEL technique were small and there were no significant differences among the fusion types.
Other determinants of proliferation rate may include secondary genetic alterations in cell cycle regulators. In two series of ES/PNET which partially overlapped with the present study group, we have found evidence of P53 alterations in 11% and INK4A deletion in 17%. 40,41 Similar percentages have been reported by other groups. 42-48 These genetic alterations are relatively uncommon but appear prognostically significant. 40,41,48 Because of their low prevalence, however, alterations in cell cycle regulators are unlikely to account for the significant differences in Ki-67 values between groups in the present study, but may explain some of the variability in proliferative rate within these groups. Establishing whether the distribution of these secondary genetic alterations in cell cycle regulators is random in relation to EWS-ETS fusion type will require a much larger systematic multiparameter study.
In summary, the most common type of EWS-FLI1 fusion transcript, type 1, is associated with a favorable prognosis 6,7 and appears to encode a functionally weaker transactivator 8 compared to other fusion types. The present study indicates that these differences are paralleled by similar differences in proliferative rate, perhaps mediated in part by the putative differential regulation of the IGF-1R pathway by these alternative forms of EWS-FLI1. Further elucidation of the target genes of EWS-FLI1 should clarify this intriguing aspect of the biology of ES/PNET.
Acknowledgments
We thank Denis Leung, Ph.D., for statistical advice and review, Margaret Collins, M.D., for providing paraffin sections of blocks from the Department of Pathology at the Children’s Hospital of Philadelphia and Loli Martinez for technical assistance with immunohistochemical and TUNEL assays.
Footnotes
Address reprint requests to Marc Ladanyi, M.D., Department of Pathology, Memorial Sloan-Kettering Cancer Center, 1275 York Avenue, New York, NY 10021. E-mail: .gro.ccksm@miynadal
Supported in part by American Cancer Society Research Project Grant 99–216 (to M. L.) and by the Public Health Care System of Spain, Madrid, Spain (FIS grant 96/2102 to E. de A).
References
Articles from The American Journal of Pathology are provided here courtesy of American Society for Investigative Pathology
Full text links
Read article at publisher's site: https://doi.org/10.1016/s0002-9440(10)64953-x
Read article for free, from open access legal sources, via Unpaywall:
https://europepmc.org/articles/pmc1876855?pdf=render
Citations & impact
Impact metrics
Citations of article over time
Article citations
Expression of DNA Repair Genes in Ewing Sarcoma.
Cancer Diagn Progn, 4(3):231-238, 03 May 2024
Cited by: 0 articles | PMID: 38707718 | PMCID: PMC11062174
Review Free full text in Europe PMC
Pilot Study of Recurrent Ewing's Sarcoma Management with Vigil/Temozolomide/Irinotecan and Assessment of Circulating Tumor (ct) DNA.
Clin Cancer Res, 29(9):1689-1697, 01 May 2023
Cited by: 3 articles | PMID: 36780200 | PMCID: PMC10150239
Shifting from a Biological-Agnostic Approach to a Molecular-Driven Strategy in Rare Cancers: Ewing Sarcoma Archetype.
Biomedicines, 11(3):874, 13 Mar 2023
Cited by: 1 article | PMID: 36979853 | PMCID: PMC10045500
Review Free full text in Europe PMC
Primary primitive neuroectodermal tumor of the cervix confirmed with molecular analysis in a pregnant woman: A case report and literature review.
Front Genet, 13:871531, 10 Aug 2022
Cited by: 0 articles | PMID: 36035131 | PMCID: PMC9399424
Molecular Targets for Novel Therapeutics in Pediatric Fusion-Positive Non-CNS Solid Tumors.
Front Pharmacol, 12:747895, 20 Jan 2022
Cited by: 7 articles | PMID: 35126101 | PMCID: PMC8811504
Review Free full text in Europe PMC
Go to all (71) article citations
Data
Similar Articles
To arrive at the top five similar articles we use a word-weighted algorithm to compare words from the Title and Abstract of each citation.
Absence of detectable EWS/FLI1 expression after therapy-induced neural differentiation in Ewing sarcoma.
Hum Pathol, 29(3):289-294, 01 Mar 1998
Cited by: 14 articles | PMID: 9496833
The evaluation of CD99 immunoreactivity and EWS/FLI1 translocation by fluorescence in situ hybridization in central PNETs and Ewing's sarcoma family of tumors.
Pathol Oncol Res, 17(3):619-625, 26 Jan 2011
Cited by: 19 articles | PMID: 21267687
EWS-FLI1 and EWS-ERG gene fusions are associated with similar clinical phenotypes in Ewing's sarcoma.
J Clin Oncol, 17(6):1809-1814, 01 Jun 1999
Cited by: 104 articles | PMID: 10561219
Ewing's sarcoma oncoprotein EWS-FLI1: the perfect target without a therapeutic agent.
Future Oncol, 1(4):521-528, 01 Aug 2005
Cited by: 60 articles | PMID: 16556028
Review
Funding
Funders who supported this work.
American Cancer Society (2)
Grant ID: 99–216
Grant ID: 96/2102