Abstract
Free full text
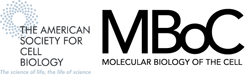
Regulation of Macropinocytosis by p21-activated Kinase-1
Abstract
The process of macropinocytosis is an essential aspect of normal cell function, contributing to both growth and motile processes of cells. p21-activated kinases (PAKs) are targets for activated Rac and Cdc42 guanosine 5′-triphosphatases and have been shown to regulate the actin-myosin cytoskeleton. In fibroblasts PAK1 localizes to areas of membrane ruffling, as well as to amiloride-sensitive pinocytic vesicles. Expression of a PAK1 kinase autoinhibitory domain blocked both platelet-derived growth factor- and RacQ61L-stimulated uptake of 70-kDa dextran particles, whereas an inactive version of this domain did not, indicating that PAK kinase activity is required for normal growth factor-induced macropinocytosis. The mechanisms by which PAK modulate macropinocytosis were examined in NIH3T3 cell lines expressing various PAK1 constructs under the control of a tetracycline-responsive transactivator. Cells expressing PAK1 (H83,86L), a mutant that dramatically stimulates formation of dorsal membrane ruffles, exhibited increased macropinocytic uptake of 70-kDa dextran particles in the absence of additional stimulation. This effect was not antagonized by coexpression of dominant-negative Rac1-T17N. In the presence of platelet-derived growth factor, both PAK1 (H83,86L) and a highly kinase active PAK1 (T423E) mutant dramatically enhanced the uptake of 70-kDa dextran. Neither wild-type PAK1 nor vector controls exhibited enhanced macropinocytosis, nor did PAK1 (H83,86L) affect clathrin-dependent endocytic mechanisms. Active versions of PAK1 enhanced both growth factor-stimulated 70-kDa dextran uptake and efflux, suggesting that PAK1 activity modulated pinocytic vesicle cycling. These data indicate that PAK1 plays an important regulatory role in the process of macropinocytosis, perhaps related to the requirement for PAK in directed cell motility.
Introduction
p21-activated kinases (PAKs 1, 2, 3) are a group of 62–68-kDa serine/threonine kinases originally identified as targets of the small guanosine 5′-triphosphatases (GTPases) Rac and Cdc42 (Manser et al., 1994; Sells and Chernoff, 1997; Knaus and Bokoch, 1998). GTP-bound forms of Rac and Cdc42 stimulate the activity of PAKs by binding to a specific N-terminal p21-binding site (Manser et al., 1994; Burbelo et al., 1995; Sells and Chernoff, 1997), thereby relieving the influence of an adjacent kinase autoinhibitory domain (Zhao et al., 1998; Zenke et al., 1999). PAKs have been implicated as effectors in several Rac- and Cdc42-regulated signaling pathways, including regulation of the phagocyte NADPH oxidase (Knaus et al., 1995), p38 and C-jun N-terminal kinase activation (Zhang et al., 1995; Bagrodia et al., 1995; Tang et al., 1997), and modulation of the actin cytoskeleton (Dharmawardhane et al., 1997; Manser et al., 1997; Sells et al., 1997; Daniels et al., 1998).
Introduction of a constitutively active PAK1 (H83,86L) N-terminal mutant into a variety of adherent cells induces the formation of cortical actin structures similar to those known to be regulated by Rac and Cdc42, including membrane ruffles and lamellipodia, filopodia, and focal complexes (Dharmawardhane et al., 1997; Manser et al., 1997; Sells et al., 1997). PAK1 (H83,86L) is also effective at inducing the extension of circular dorsal ruffles and colocalizes with polymerized actin in these structures (Dharmawardhane et al., 1997; Edwards et al., 1999). Such actin-rich circular ruffles have been previously implicated in the process of macropinocytosis, ultimately contracting to f1orm macropinosomes of >0.2 μm (Bar-Sagi and Feramisco, 1986; Dowrick et al., 1993; Swanson and Watts, 1995). Macropinocytosis is an efficient route for the nonselective uptake of solute macromolecules, and may be either constitutive or stimulated by growth factors such as epidermal growth factor and platelet-derived growth factor (PDGF) (Brunk et al., 1976; Hewlett et al., 1994), macrophage colony-stimulating factor (Racoosin and Swanson, 1989, 1992), interleukin-4 (Sallusto et al., 1995), and by phorbol esters (Swanson, 1989b). Interestingly, these agents are known to initiate signaling pathways leading to activation of the Rac GTPase, which has been implicated in regulating macropinocytosis (Ridley et al., 1992). The prevalence of macropinocytosis in many cell types suggests that it may contribute to cellular processes in addition to nutrient uptake, although these roles have remained elusive, except in the case of host–pathogen interactions (Francis et al., 1993; Swanson and Watts, 1995; Chen et al., 1996). It is possible that the internalization of plasma membrane resulting from the rapid and transient stimulation of macropinocytosis by growth factors may be involved in directed cell movement. For example, regulation of membrane flux via pinocytosis may contribute to the membrane flow that is thought to be a driving force for cell locomotion (Bretscher, 1996, 1998).
We previously showed that endogenous PAK1 in Swiss 3T3 fibroblasts localized to pinocytic vesicles by colocalizing an affinity-purified PAK1 antibody with a marker for bulk fluid-phase uptake (Dharmawardhane et al., 1997). Coupled with the observation that PAK1 can induce the formation of circular dorsal ruffles similar to those associated with the macropinocytic uptake process, a potential role for PAK in regulating this activity is suggested. We show here that inhibition of endogenous PAK activity using a PAK-specific autoinhibitory domain blocks growth factor-induced macropinocytosis, indicating that PAK activity is required for normal macropinocytosis. In addition, PDGF-stimulated macropinocytosis is enhanced by activated PAK1 mutants, with both uptake and efflux rates affected. These data indicate a direct regulatory role for PAK in macropinocytosis that involves both PAK kinase activity and cytoskeletal remodeling.
MATERIALS AND METHODS
Transfection and Cell Culture
Culturing and handling of Swiss 3T3 cells was as described (Dharmawardhane et al., 1997). The NIH3T3 variant S2-6 bearing a tetracycline-regulated transactivator (Shockett et al., 1995) was used to construct cell lines that inducibly express PAK1 and various mutants; the establishment and characterization of these clones has been described (Sells et al., 1999). The NIH3T3 cell lines were cultured in DMEM with 10% calf serum, 2.5 mM histidinol, 2 μg/ml puromycin, and 0.5 μg/ml tetracycline (Sigma, St. Louis, MO). Three to five days after seeding, the cells were incubated for 24 h in the culture medium with or without tetracycline, and for 16 h in serum-free medium to obtain quiescent cells expressing PAK1 protein constructs. In some experiments, proteins were expressed by using a Semliki Forest virus protein expression system, as described in Sanders et al. (1999). Transfection efficiency was routinely >90% by using the virus system.
Quantification of Macropinocytosis
Labeling of pinocytic vesicles and measuring uptake of fluorescent dextran was performed as described previously (Swanson 1989a; Racoosin and Swanson, 1994). Cells serum starved for 18 h were additionally preincubated on ice for 30 min in 24-well plates to reduce basal levels of macropinocytosis, and then activated by addition of 5 ng/ml PDGF-BB (Upstate Biotechnology, Lake Placid, NY) as indicated, and immediately before addition of the 70-kDa dextran fluorescent probe. Cells were incubated at 37°C in 0.5 mg/ml 70-kDa fluorescein-tagged dextran (fDx) (Molecular Probes, Eugene, OR) for various times. In some experiments, 100 nM wortmannin or 10 μg/ml cytochalasin D was added for 15 min before addition of PDGF and fluorescent dextran. To terminate dextran uptake, cell plates were transferred to ice and washed three times for 5 min each in 1 liter of ice-cold phosphate-buffered saline (PBS). Cells were lysed by incubation for 30 min at 37°C in 0.5% Triton-X 100 in PBS. Cell lysates were excited at 494 nm and the emission at 520 nm was determined in a luminescence spectrometer LS50B (Perkin-Elmer/Cetus, Norwalk, CT) to quantitate the internalized 70-kDa fDx. Conditions for variations on the uptake experiments, and for fDx efflux experiments are described in the relevant figure legends.
Fluorescence Microscopy
Immunofluorescence microscopy was performed as described in Racoosin and Swanson (1994). NIH3T3 cells expressing vector alone or PAK1 mutant proteins were incubated with 0.5 mg/ml lysine-fixable 70-kDa Texas Red dextran and/or 5 ng/ml PDGF for 30 min, as indicated. After fDx uptake, the cells were fixed for 1 h in a modified periodate–lysine–paraformaldehyde fixative. In the experiments with the Semliki Forest virus gene expression system, the cells were permeabilized in ice-cold MeOH for 1.5 min, and the transfected cells were detected by staining for 1 h in 1:200 anti-myc (9E10) antibody. The secondary antibody incubation was for 1 h in 1:500 fluorescein-conjugated anti-rabbit mouse IgG (Cappel Laboratories, Cochranville, PA). Washed coverslips were mounted in Anti-Fade (Molecular Probes).
Measurement of Transferrin Uptake (Clathrin-mediated Endocytosis)
Transferrin uptake as a measure of clathrin-mediated endocytosis was assayed according to Damke et al. (1994). Briefly, quiescent cells expressing PAK1 (H83,86L) mutation or vector control cells, with or with out the addition of 5 ng/ml PDGF, were incubated with 4 mg/ml transferrin in PBS with 1 mM CaCl2, 1 mM MgCl2, 5 mM glucose, and 0.2% bovine serum albumin (BSA) for various times at 37°C. Cells were recovered by the addition of 5 mM EDTA in PBS, and each treatment was divided into two aliquots. One aliquot was used to determine the total transferrin bound and the other aliquot was treated with avidin and biocytin to block surface-bound transferrin. Cells were lysed in 1% Triton-X 100 lysis buffer containing 0.5% BSA and plated in wells coated with anti-transferrin antibody. After an overnight incubation in primary antibody, the washed wells were incubated in 1:5000 streptavidin:horseradish peroxidase and the color was developed by the addition of 0.4 mg/ml l-phenylendiamine and 0.44 μl/ml hydrogen peroxide. The color reaction was read at 490-nm absorption in an EIA Autoreader, model LL310, Biotek Instruments, Luton, United Kingdom. The total transferrin bound for each treatment condition was used to calculate the percentage of transferrin internalized for each sample, as in Damke et al. (1994).
RESULTS
PAK Activity Is Required for PDGF-stimulated Macropinocytosis
We previously demonstrated that a portion of endogenous PAK1 in Swiss 3T3 cells localized to vesicles characterized by their ability to internalize rhodamine-labeled BSA from solution (Dharmawardhane et al., 1997). In cells pretreated with 3 mM amiloride, a potent inhibitor of Na+/H+ exchange that effectively abolishes uptake of 70-kDa fDx under our assay conditions (West et al., 1989; Dowrick et al.,1993; Swanson and Watts, 1995), PAK1 redistributed from the vesicular structures to a diffuse localization throughout the cytoplasm, consistent with the conclusion that PAK1 colocalizes with pinocytic vesicles.
To determine whether the association of PAK1 with pinocytic vesicles was indicative of a regulatory role of PAK1 in macropinocytosis, we assessed the uptake of fDx in NIH3T3 cells expressing the PAK1 autoinhibitory domain, aa 83–149 (AID). This domain interacts in trans with the PAK catalytic domain to specifically block the ability of PAK to phosphorylate exogenous substrates (Zhao et al., 1998; Zenke et al., 1999). As shown in Table Table1,1, expression of the AID did not affect the low levels of fDx uptake in quiescent cells. However, in the presence of PDGF, AID expression almost completely suppressed fDx uptake. In contrast, when we inactivated the AID by mutating it at L107F (Zenke et al., 1999), fDx uptake was restored to the levels seen in control cells. These data indicate that PAK1 kinase activity is required for the normal process of growth factor-stimulated macropinocytosis.
Table 1
Inhibition of fDx uptake induced by PDGF- and PAK1 (H83,86L) by the PAK1 AID
![]() |
Serum-starved NIH3T3 cells in which PAK1 (H83,86L) expression was induced by tetracycline withdrawal or repressed by tetracycline maintenance (Control) were infected with Semliki Forest virus containing myc-tagged PAK1 autoinhibitory domain (aa 83–149) or inactivated PAK1 AID (aa 83–149, L107F), as in Edwards et al. (1999). Cells were then either treated without (−) or with (+) 6 ng/ml PDGF, followed by the addition of 0.5 mg/ml lysine-fixable 70-kDa fDx, for 30 min as in MATERIALS AND METHODS. Virally transfected cells were detected by staining with an anti-myc 9E10 antibody followed by a FITC-tagged secondary antibody. Cells were quantitated in a fluorescence microscope using a 40X objective, and scored as −, +, or ++ according to the relative level of fDx uptake, as in the representative fluorescent micrographs shown. A total of 100–216 cells (only myc-staining cells were counted for each condition after viral transfections) were counted per experimental condition in 10 separate fields per coverslip. The values shown are given as a percentage of cells in each scoring category and were calculated as the mean ± SE by using data from three separate experiments performed in duplicate.
A role for Rac in regulating growth factor-stimulated fluid-phase uptake was previously suggested by the observation (Ridley et al., 1992) that dominant-negative Rac1 (T17N) reduced cellular uptake of dextrans. We confirmed that expression of a constitutively active Rac1 Q61L stimulated 70-kDa fDx uptake by NIH3T3 cells (Figure (Figure1).1). This stimulatory effect of Rac Q61L could be almost completely blocked by the expression of the PAK1 AID, but not by the inactivated L107F version. Thus, Rac-mediated macropinocytosis is also dependent upon PAK catalytic activity.

Inhibition of Rac Q61L-induced fDx uptake by PAK1 AID. Serum-starved NIH3T3 cells stably transfected with a tetracycline-inducible vector only were infected with Semliki Forest virus containing myc-tagged PAK1 (83–149), PAK1 (83–149, L107F). Rac1 (Q61L), or combinations thereof as indicated (methods as in Edwards et al., 1999; Sanders et al., 1999). After 10 h of infection, the cells were incubated in 5 mg/ml lysine-fixable 70-kDa fDx for 30 min, and then fixed, permeabilized, and stained for the myc epitope by using anti-myc 9E10 antibody, followed by a fluorescein isothiocyanate-tagged secondary antibody. Cells were scored by using a fluorescence microscope with 40× objective as −, +, or ++ according to the level of fDx uptake, as illustrated in Table Table1.1. A total of ~200 cells was counted per experimental condition by using 10 separate fields per coverslip. Values, given as a percentage of cells in each scoring category, from three separate experiments are shown as the mean ± SE.
Active PAK1 Mutants Increase PDGF-stimulated Macropinocytosis
Stable NIH3T3 cell lines expressing either vector alone, wild-type PAK1, or a series of PAK1 functional mutants under control of a tetracycline-regulated transactivator were used to investigate the mechanism(s) through which PAK1 modulated macropinocytosis. PAK1 (H83,86L) is a cytoskeletally active form of PAK that no longer binds Rac or Cdc42 and which has moderate constitutive kinase activity, PAK1 (K299R) is mutated in the ATP-binding site and is inactive as a kinase, and PAK1 (T423E) has high constitutive kinase activity. The effects of these PAK1 mutants on the actin cytoskeleton have been described in detail (Sells et al., 1997, 1999). Elimination of tetracycline from the growth medium for 24 h resulted in expression of each hemagglutinin-tagged PAK1 construct to comparable levels as determined by immunoblot. When these cells were stimulated with PDGF in the presence of tetracycline, each cell line exhibited quantitatively enhanced macropinocytosis, typically ranging from 30 to 50% higher in the presence of PDGF than in the absence of stimulation (Figure (Figure2A). 2A). Induced expression (−tetracycline) of wild-type PAK1 and PAK1 (K299R) proteins did not cause statistically significant changes in PDGF-stimulated macropinocytosis compared with uninduced cells. However, the clones expressing the activated PAK1 (H83,86L) and (T423E) mutations showed statistically significant higher levels of PDGF-stimulated macropinocytosis, on the order of 150–200% greater than were observed in the noninduced cells (Figure (Figure2A).2A). Expression of the PAK AID (aa 83–149) substantially suppressed the enhanced mactropinocytic response induced by PAK (H83,86L) (Table (Table1).1). Thus, constitutive activation of PAK1, either by mutating the GTPase binding/anti-inhibitory domain or by directly activating the kinase domain, results in a significantly greater PDGF-stimulated macropinocytosis.
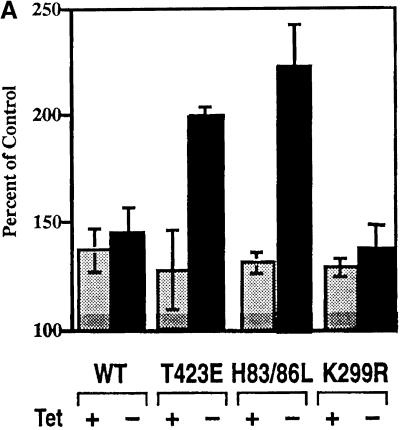

PDGF-stimulated pinocytosis in cell lines expressing PAK1 or PAK1 mutants. (A) NIH3T3 cells transformed with wild-type PAK1, PAK1 (T423E), PAK1 (H83,86L), or PAK1 (K299R) were either maintained in tetracycline (+) or grown for 24 h in media without tetracycline (−) to induce PAK protein expression. Expression of PAK proteins was determined to be equal by immunoblot, as in Sells et al. (1999). During the induction period, the cells were serum starved for 18 h, and then cooled on ice for 30 min before the start of the experiment to render them quiescent. The uptake of 70-kDa fDx was measured for 60 min in the presence or absence of 5 ng/ml PDGF as described in MATERIALS AND METHODS. The absorption/emission spectra for fluorescein at 494/520 nm were determined, and data plotted as the percentage of control (i.e,. the amount of fDx70 taken up in the presence of 5 ng/ml PDGF/amount of fDx70 uptake in the absence of PDGF × 100) for each treatment. The values shown are the mean ± SD of the mean for n = 4 determinations in two separate experiments. Tet, tetracycline. (B) Uptake of 70-kDa fDx was performed as above, except that cells were not precooled before the addition of lysine fixable 70-kDa fDx and 5 ng/ml PDGF. The cells were fixed in a periodate–lysine–paraformaldehyde fixative as detailed in MATERIALS AND METHODS, and the fluorescence of fDx was visualized in the various induced PAK1 NIH3T3 cell lines at t = 30 min. Micrographs shown are at 5000×.
We visualized the uptake of 70-kDa fDx in the presence and absence of PDGF in the control and PAK1 mutant clones by fluorescence microscopy (Figure (Figure2B).2B). As shown in Figure Figure2A,2A, PDGF addition stimulated fDx uptake in all cell lines. Of particular note, PAK1 (H83,86L) exhibited enhanced fDx uptake even in the absence of PDGF, notably in association with dorsal ruffles and large macropinocytic vesicles. The microscopic images emphasize the effectiveness of PAK1 (H83,86L), but not PAK1 (T423E), to stimulate macropinocytosis even in the absence of PDGF, although addition of PDGF results in a synergistic enhancement of uptake. Macropinocytosis induced by PAK1 (H83,86L) alone was sensitive to expression of the active PAK1 AID (Table (Table11).
PAK Modulates Both Uptake and Recycling Kinetics of Macropinocytic Vesicles
The accumulation of 70-kDa fDx we measured at steady state reflects both uptake and regurgitation kinetics (Cupers et al., 1994). We attempted to assess the effects of PAK on the actual uptake process by measuring linear fDx uptake during a 15-min time course after stimulation for 45 min with PDGF (Figure (Figure3A).3A). Under these conditions, we observed that PAK1 (T423E) and PAK1 (H83,86L), but not PAK1 wild type or PAK1 (K299R), consistently increased uptake of 70-kDa fDx by 200 to 500%, respectively. We also performed the experiment of measuring fDx uptake over short 10-min intervals after preincubation for 0, 10, 20, 30, 45, or 60 min with PDGF. An increased level of fDx uptake was also detected for PAK1 (T423E) and (H83,86L) by using this protocol (data not shown).
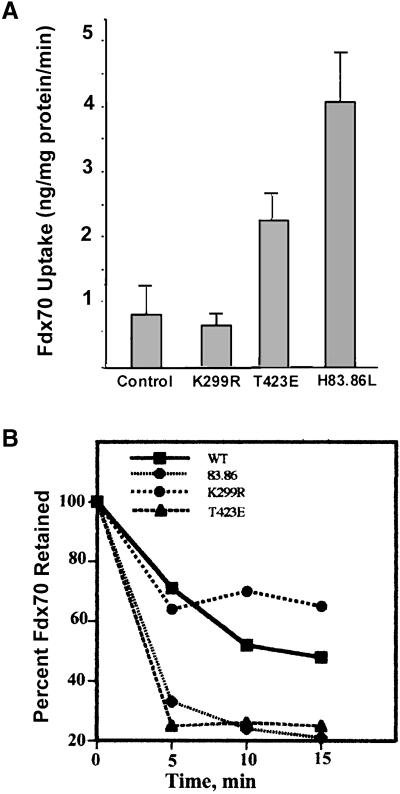
Effects of PAK on fDx uptake and efflux kinetics. (A) Effects of preincubation of cells with PDGF on the regulation of fDx uptake by PAK were examined. NIH3T3 cells expressing either vector alone (control), PAK1 wild type, or the indicated point mutants were maintained for 24 h in the absence of tetracycline to allow PAK1 protein expression. Cells were serum starved for 18 h during the expression period, and then PDGF (5 ng/ml) added to each cell line for 45 min. At the end of each incubation period, 5 mg/ml 70-kDa fDx was added and uptake measured at 0, 2.5, 5, 10, and 15 min. Cells were washed free of noninternalized fDx and the amount of fDx in cell lysates quantified by spectrofluorometry. The amount of fDx taken up per minute in the linear phase was plotted (±SE; n = 6). (B) NIH3T3 cell lines were handled as described above. After serum starvation for 18 h, PDGF and 70-kDa fDx were added to the cells for 45 min to allow uptake, and then washed three times in ice cold PBS and incubated in PBS containing 5.5 mM glucose, 1 mM CaCl2, and 10 mM MgCl2 for the indicated times. Cells were lysed and the fDx retained was quantitated by spectrofluorometry. One hundred percent represents the amount of fDx contained within each cell line at t = 0 min. SD for each data point was <18% of the mean (n = 6).
Receptor-stimulated macropinocytosis has previously been shown to involve effects on both the uptake and efflux phases of macropinocytic vesicle cycling (Racoosin and Swanson, 1989; Swanson, 1989b). We therefore examined the effects of PAK on efflux kinetics of fDx from preloaded cells (Figure (Figure3B).3B). Wild-type PAK exhibited efflux rates similar to those seen in control cells. PAK1 (K299R) expression modestly decreased fDx efflux at longer times, perhaps due to the ability of this construct to interact with Rac to form a nonproductive complex. The inhibition of 70-kDa fDx efflux is likely to mask at steady state any evident decrease in fDx uptake that would occur as a result of dominant-negative activity of this kinase-dead version of PAK. In contrast, both PAK1 (H83,86L) and PAK1 (T423E) dramatically increased the efflux rates of fDx. Because the effect of these mutants on steady-state uptake of fDx was positive even in the presence of the enhanced efflux they induce, this indicates that these activated forms of PAK1 had even greater effects on the uptake phase than is apparent in the steady-state cumulative data. PAK1 thus appears to positively regulate the cycling of pinocytic vesicles.
Effects of PAK1 (H83,86L) on Macropinocytosis Correlate with Effects on Dorsal Ruffle Formation
To assess whether the increased macropinocytosis observed in the presence of PAK1 (H83,86L) was associated with the increased dorsal ruffling that had been previously observed in cells into which PAK1 (H83,86L) was introduced (Dharmawardhane et al., 1997; Edwards et al., 1999), the distribution of PAK1 and F-actin was monitored in serum-starved (but not precooled) cells after incubation in PDGF and nonfluorescent 70-kDa dextran (Figure (Figure4).4). Before PDGF addition, PAK1 was localized to small vesicles in the cytosol and the perinuclear region of both control and PAK (H83,86L) cell lines, presumably representing a basal level of constitutive pinocytic vesicle formation. The induced PAK (H83,86L) cell line exhibited constitutive membrane and dorsal ruffling in a portion of the cell population even in the absence of PDGF. Immediately after PDGF addition, the PAK1 (H83,86L)-expressing cells started forming large numbers of dorsal ruffles, which were evident beginning at 5 min after PDGF. In contrast, the other cell lines exhibited varying lesser degrees of dorsal ruffle formation, as well as small membrane ruffles at 10 min after PDGF. At 20-min post-PDGF, large macropinocytic vesicles lined with PAK1 were observed in the cytosol. The PAK1 (H83,86L) cells exhibited a higher number of the phase dense macropinocytic vesicles, and these were often closely associated with dorsal ruffles (Figure (Figure2B,2B, bottom). Interestingly, even though PAK1 and F-actin were found to be colocalized in the dorsal ruffles (Figure (Figure44 and Dharmawardhane et al., 1997), only PAK1 localizes to the subsequent pinocytic vesicles formed. This suggests that once the surface ruffles take up fluids and constrict to close off the vesicle, F-actin is only transiently associated with the resulting mature macropinosome.

PAK1 and F-actin localization in PAK1 (H83,86L)-expressing cells. Localization of PAK1, determined with a specific affinity purified anti-PAK1 antibody, or of F-actin, determined by rhodamine phalloidin staining, was examined in either vector control (left two columns) or PAK1 (H83,86L) (right two columns)-expressing cell lines at various times after PDGF (5 ng/ml) stimulation, as described in MATERIALS AND METHODS. The arrowheads indicate areas where PAK1 and F-actin colocalize in sites of membrane ruffling. The short arrows point to remnants of macropinocytic vesicles whose outline is visible in the stained cells. Micrographs shown are at 3000×.
Increased PDGF-stimulated Pinocytosis Induced by Expression of Activated PAK1 Is Sensitive to Wortmannin and Cytochalasin D, but Is Not Inhibited by Dominant-Negative Rac
Because fluid-phase pinocytosis, and not receptor-mediated endocytosis, has been reported to be inhibited by the phosphatidylinositol (PI) 3-kinase inhibitor wortmannin (Barker et al., 1995; Clague et al., 1995; Araki et al., 1996), we examined the effect of wortmannin on macropinocytosis stimulated by PDGF in the transformed NIH3T3 cell lines. Wortmannin inhibited the PDGF-stimulated 70-kDa fDx uptake in all cell lines to control levels, including the enhanced pinocytosis observed in the lines expressing PAK1 (T423E) and PAK1 (H83,86L) (Figure (Figure5).5). This result indicates that PI 3-kinase is involved in the regulation of the same macropinocytotic process enhanced by PAK1, and that the pharmacology of the process differs from clathrin-mediated endocytosis. We also examined the effect of the actin filament-disrupting agent cytochalasin D on the regulation of macropinocytosis by PAK1. Macropinocytosis induced by PDGF was inhibited substantially by cytochalasin D in control and PAK1 mutant cell lines (Figure (Figure5).5). The effects of wortmannin and cytochalasin D correlated with inhibition of the formation of actin-rich dorsal ruffles required for the bulk uptake of fluids.
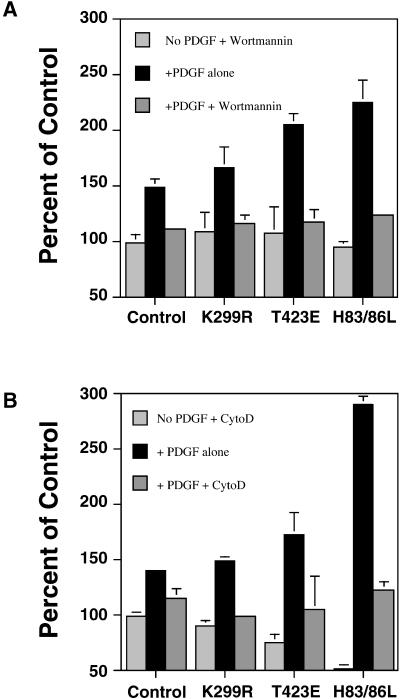
Inhibition of PAK1-enhanced macropinocytosis by the PI 3-kinase inhibitor wortmannin and by the F-actin disrupting agent cytochalasin D. Uptake of 70-kDa fDx was measured in cells expressing control vector, PAK1 wild-type, and PAK1 point mutations. As indicated, the cells were pretreated with either 100 nM wortmannin (A) or 10 μg/ml cytochalasin D (B) for 10 min, and then stimulated with 5 ng/ml PDGF and fDx added at t = 0. Uptake was for 45 min. Percentage of control is the amount of fDx70 taken up under each condition compared with the nondrug- or PDGF-treated cells (set to 100%). Results shown are the mean ± SD of 2–3 experiments performed in duplicate.
To rule out the possibility that the dramatic stimulatory effects of PAK1 (H83,86L) on macropinocytosis that we observed were an indirect result of Rac activation, we measured 70-kDa fDx uptake in the presence of wild-type or dominant-negative Rac1 (T17N) (Figure (Figure6).6). Approximately 70–80% of the PAK1 (H83,86L) and PAK1 (H83,86L), Rac1 (T17N) double mutants demonstrated enhanced fDx uptake. Coexpression of Rac1 wild type with PAK1 (H83,86L) did not significantly modify the effect of PAK on macropinocytosis. These results indicate that PAK regulates macropinocytosis independently of, or downstream from, Rac1.


Macropinocytosis in cells expressing PAK1 and Rac1 mutants. NIH3T3 cell lines expressing PAK1 (H83,86L) were incubated in media with or without (control) tetracycline for protein induction. These cells were then infected with a Semliki Forest virus expression construct containing either myc-tagged dominant-negative Rac1 (T17N) or Rac1 wild type (WT) (Sanders et al., 1999). (A) After 12 h of infection, the cells were incubated in 5 ng/ml PDGF and 5 mg/ml fixable 70-kDa fDX for 45 min, and then fixed, permeabilized, and stained by using the 9E10-myc epitope antibody (Racoosin and Swanson, 1994). Row A, fluorescence micrographs of representative cells demonstrating fDx retained in macropinocytic vesicles. Row B, same cells stained with anti-myc 9E10 to detect Rac1-WT and -T17N expression. Row C, same cells under phase contrast microscopy. (B) After 12 h of infection, the cells were incubated in 5 ng/ml PDGF and 5 mg/ml lysine-fixable 70-kDa fDx for 45 min, and then fixed, permeabilized, and stained for the myc epitope by using anti-myc 9E10 antibody, followed by a fluorescein isothiocyanate-tagged secondary antibody. Cells were scored by using a fluorescence microscope with 40× objective as −, +, or ++ according to the level of fDx uptake, as illustrated in Table Table1.1. A total of ~200 cells was counted per experimental condition by using 10 separate fields per coverslip. Values, given as a percentage of cells in each scoring category, from three separate experiments are shown as the mean ± SE.
PAK1 Does Not Regulate Clathrin-mediated Endocytosis
To confirm that the enhanced effect of the PAK1 (H83,86L) mutant on PDGF-stimulated 70-kDa fDx uptake was due to macropinocytosis, we quantified the uptake of transferrin in control and PAK1 (H83,86L) cell lines. Transferrin is known to be internalized via clathrin-coated endocytic vesicles (Hopkins, 1983; Sandvig and van Deurs, 1990). As seen in Figure Figure7,7, PAK1 (H83,86L) cells did not differ from control cells in the uptake of transferrin. Both cell lines exhibited peak transferrin uptake at 10 min, and the uptake was unaffected by stimulation with PDGF. In conjunction with the inhibitory effect of wortmannin, we conclude that the enhanced uptake of 70-kDa fDx induced by active PAK1 in the presence of PDGF is due to macropinocytosis, and does not involve receptor- and clathrin-mediated endocytic mechanisms.
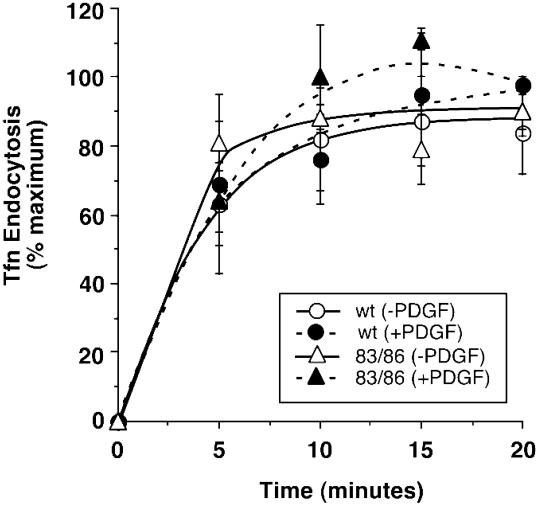
PAK1 has no effect on transferrin uptake via clathrin-mediated endocytosis. Uptake of transferrin was determined as described in MATERIALS AND METHODS in the induced vector control (circles) or PAK1 (H83,86L)-expressing (triangles) stable cell lines. The percentage of transferrin uptake is the amount of transferrin internalized as a function of the total amount of transferrin bound for each treatment. The results shown are of one experiment representative of two similar experiments, both of which showed no differences in transferrin uptake stimulated by PDGF (closed symbols) in either the absence or presence of PAK1 (H83,86L) expression. No significant differences in total transferrin binding were observed between the two cell lines.
DISCUSSION
It has previously been established that PAK1 regulates cytoskeletal dynamics, including formation of membrane ruffles, lamellipodia, and organization of focal complexes (Dharmawardhane et al., 1997; Sells et al., 1997; Daniels et al., 1998; Edwards et al., 1999). One of the most intriguing observations from our earlier localization studies was the fact that a fraction of endogenous PAK1 colocalized with a marker for fluid-phase uptake (Dharmawardhane et al., 1997). Additionally, at early times after stimulation of quiescent cells with PDGF, PAK1 was predominantly associated with F-actin in circular ruffles on the cell surface (dorsal ruffles). Indeed, PAK1 initially localized to ring-like structures that preceded the F-actin–associated dorsal ruffles, suggesting that PAK1 may be regulating the early organization of such ruffles. Finally, microinjection of an activated PAK1 (H83,86L) resulted in a marked increase in the formation of dorsal ruffles. Because dorsal or circular ruffles are known to be precursors of macropinocytic vesicles (Swanson and Watts, 1995), the possible regulation of macropinocytosis by PAK was investigated in the present study.
To preferentially focus on the process of macropinocytosis, we used a large 70-kDa dextran molecule in uptake studies (Araki et al., 1996). Using the PAK1 AID as a specific means to inhibit endogenous PAK kinase activity, we showed that PDGF-stimulated uptake of fDx was blocked by >85% (Table (Table1).1). This was a specific inhibitory effect on endogenous PAK because introduction of an L107F mutation into the AID, which prevents it from binding to and inhibiting the PAK catalytic domain (Zenke et al., 1999), prevented any significant effect on macropinocytosis. The stimulatory effects of growth factors on macropinocytosis may be mediated through activation of Rac1, and we observed that the ability of constitutively active Rac1 Q61L to stimulate fDx uptake was also specifically attenuated by inhibition of PAK kinase activity (Figure (Figure11).
Role of PAK 1 in Dorsal Ruffling Leading to Macropinocytosis
Quantification of macropinocytosis, as well as fluorescent microscopic observation of cells after addition of 70-kDa fDx, indicates that activated forms of PAK1 increase macropinocytosis, especially after PDGF addition to quiescent cells. In the absence of PDGF, only PAK1 (H83,86L) significantly stimulated dextran uptake (Figure (Figure2B).2B). This stimulatory effect appears to correlate with the ability of PAK1 (H83,86L) to induce dorsal ruffle formation (Figures (Figures2B 2B and and4).4). PAK1 (T423E), although highly active as a kinase, does not effectively induce the formation of dorsal ruffles (Sells et al., 1997; Edwards et al., 1999), presumably due to the inability to make necessary N-terminal protein–protein interactions. Accordingly, expression of the PAK1 (T423E) mutant did not stimulate dextran uptake in the absence of PDGF (Figure (Figure22B).
PDGF stimulated steady-state uptake of 70-kDa fDx by 30 to 50% in control cells, and this level of stimulation did not change in any of the stable cell lines in the absence of PAK1 protein induction (Figure (Figure2A).2A). In cells in which expression of a vector control, wild-type PAK1, or a kinase-inactive PAK1 (K299R) had been induced, there was still no significant effect on macropinocytosis of the 70-kDa fDx. In contrast, both the PAK1 (T423E) and PAK1 (H83,86L) proteins markedly enhance PDGF-induced macropinocytosis. Time course studies indicated that the PAK1 (H83,86L) was more effective than PAK1 (T423E) in enhancing dextran uptake at early times. This is likely to relate to the ability of this PAK1 mutant to stimulate dorsal ruffle formation in this time period. Treatment of a variety of cell types with growth factors is often followed by an intensive burst of ruffling of the apical surface membranes, which appears to be the earliest structural change observed during macropinocytosis (Swanson and Watts, 1995). PDGFβ-receptors are especially known to induce such circular ruffles and macropinocytosis (Westmark et al., 1990; Erickson et al., 1992). At later times, we observed that the PAK1 (T423E) also enhanced fDx uptake. The effectiveness of this highly kinase-active PAK1 mutant, in conjunction with the observed inhibition by the PAK AID, suggests that the kinase activity of PAK must play some role in regulating the uptake process. It is tempting to speculate that this effect may be due to the ability of PAK to modulate contractile activity of myosin (Tuazon and Traugh, 1984; Brzeska et al., 1997; Ramos et al., 1997; Sanders et al., 1999) because myosin II localizes to dorsal ruffles during macropinocytosis (Dowrick et al., 1993) and myosin function has been shown to be required for the contractile activity, which closes macropinosomes (Swanson et al., 1999). Alternatively, the ability of PAK to modulate actin dynamics via regulation of LIM kinase and cofilin (Edwards et al., 1999) may also be important.
PAK1 Is a Specific Regulator of Macropinocytosis
Our data indicate that PAK1 specifically regulates macropinocytosis as opposed to clathrin- or receptor-mediated endocytosis, although the exact biochemical mechanism(s) accounting for the fluid-phase uptake we are measuring is unknown. The PAK1 (H83,86L) mutant, which was most effective at enhancing PDGF-stimulated pinocytosis, did not have an effect on receptor-regulated transferrin uptake in the presence (or absence) of PDGF (Figure (Figure7).7). Additionally, amiloride blocked the uptake of 70-kDa fDx and abolished PAK1 immunolocalization to cytosolic vesicles. Amiloride is an inhibitor of Na+/H+ exchange that has been found to considerably reduce pinocytosis stimulated by growth factor receptors (West et al., 1989; Dowrick et al., 1993; Swanson and Watts, 1995).
Inhibition of PI 3-kinase activity by wortmannin and LY294002 is known to attenuate macropinocytosis (Barker et al., 1995; Clague et al., 1995; Swanson and Watts, 1995). Araki et al. (1996) showed that PI 3-kinase activity is necessary for the completion of actin-dependent macropinocytosis but not for the initial ruffling phase in macrophages. We observed that addition of wortmannin decreased fDx uptake in all the cell lines tested, including the enhanced PDGF-stimulated uptake observed with PAK1 (H83,86L) (Figure (Figure5).5). Because clathrin-mediated endocytosis is resistant to PI 3-kinase inhibitors, these data also indicate that the observed internalization of 70-kDa fDx is likely to be due to macropinocytosis.
The actin filament-disrupting agent cytochalasin D has been shown to reduce internalization of ricin and fluid-phase markers without reducing the uptake of transferrin (Sandvig and van Deurs, 1990). We observed that cytochalasin D inhibited PDGF-stimulated macropinocytosis in all the cell lines tested (Figure (Figure5).5). Cytochalasin D also reduced the enhanced fDx uptake induced by PAK1 (H83,86L) in the absence of PDGF. The inhibitory effect of cytochalasin D is likely to be due to inhibition of the PDGF-mediated actin polymerization required to induce membrane ruffling as a prerequisite for formation of the pinosome.
Conclusion
The data presented here show that PAK1 is capable of regulating macropinocytic uptake of solutes. Expression of a cytoskeletally active PAK1 (H83,86L) was capable by itself of stimulating uptake of 70-kDa dextran. In the presence of PDGF, both PAK1 (H83,86L) and a highly kinase active form of PAK1 (T423E) caused synergistic increases in PDGF-stimulated macropinocytosis. The role of PAK in inducing cytoskeletal changes, particularly the formation of circular ruffles on the dorsal surface of cells, in combination with regulatory effects on contractile activities of myosin (Ramos et al., 1997; Chew et al., 1998; Sanders et al., 1999) or on actin polymerization via LIM kinase (Edwards et al., 1999), provide a possible mechanistic basis for the enhancement of pinocytosis we report here. Such regulation may be important for processes ranging from nutrient uptake during cell growth to the invasion of cells by certain pathogenic bacteria.
Several recent studies have shown that PAK is required for normal cell motility and directed migration (Kiosses et al., 1999; Sells et al., 1999). Although the relationship among macropinocytosis, membrane ruffling, and cell motility remains unclear, it is intriguing to speculate that PAK1-regulated cycling of plasma membrane via macropinocytosis may contribute toward directed cell movement as predicted in the model described by Bretscher (1996, 1998). Indeed, the dramatic effects of PAK on both the uptake and efflux of 70-kDa fDx could reflect a redistribution of macropinocytic vesicles and the accompanying membrane from the rear of polarized cells to the leading edge. We plan to investigate this possibility in future studies.
ACKNOWLEDGMENTS
We thank Luraynne C. Sanders, Ph.D., for producing the Semliki Forest virus containing PAK and Rac mutant constructs, and we acknowledge the assistance of Yan Wang, M.D., with cell culture; Charles C. King, Ph.D., with graphics; Laura Terlecky with initial transferrin uptake analysis; Dr. Malcolm Wood with confocal microscopy, and Abina Reilly with preparation of the PAK1 R2124/3 antibody used in these studies. A special acknowledgment to Dawn Jones (University of Texas at Austin) for assistance with fluorometry and protein assays. We also thank Dr. William Balch (The Scripps Research Institute) for the use of the luminescence spectrophotometer, and Dr. Joel Swanson (University of Michigan, Ann Arbor) for comments on the manuscript. The editorial assistance of Antonette Lestelle is sincerely appreciated. This work was supported by National Institutes of Health grants GM-39434 (G.M.B.), AHA-9930121N (S.D.), CA-58689 (S.L.S.), and GM-54168 (J.C.). This is publication number 11434-IMM.
REFERENCES
- Araki N, Johnson MT, Swanson JA. A role for phosphoinositide 3-kinase in the completion of macropinocytosis and phagocytosis by macrophages. J Cell Biol. 1996;135:1–12. [Europe PMC free article] [Abstract] [Google Scholar]
- Bagrodia S, Derijard B, Davis RJ, Cerione RA. Cdc42, and PAK-mediated signaling leads to Jun kinase and p38MAP kinase activation. J Biol Chem. 1995;270:27995–27998. [Abstract] [Google Scholar]
- Barker SA, Caldwell KK, Hall A, Martinez AM, Pfeiffer JR, Oliver JM, Wilson BS. Wortmannin blocks lipid and protein kinase activities associated with PI 3-kinase and inhibits a subset of responses induced by FcRl cross-linking. Mol Biol Cell. 1995;6:1145–1148. [Europe PMC free article] [Abstract] [Google Scholar]
- Bar-Sagi D, Feramisco JR. Induction of membrane ruffling and fluid-phase pinocytosis in quiescent fibroblast by ras proteins. Science. 1986;233:1061–1068. [Abstract] [Google Scholar]
- Bretscher MS. Getting membrane flow and the cytoskeleton to cooperate in moving cells. Cell. 1996;87:601–606. [Abstract] [Google Scholar]
- Bretscher MS. Membrane traffic during locomotion. Curr Opin Cell Biol. 1998;10:537–541. [Abstract] [Google Scholar]
- Brunk U, Schellens J, Westermark B. Influence of epidermal growth factor (EGF) on ruffling activity pinocytosis and proliferation of cultivated human glia cells. Exp Cell Res. 1998;103:295–302. [Abstract] [Google Scholar]
- Brzeska H, Knaus UG, Wang Z, Bokoch GM, Korn ED. p21-activated kinase (PAK) has substrate specificity similar to Acanthamoeba myosin I heavy chain kinase and activates Acanthamoeba myosin I. Proc Natl Acad Sci USA. 1997;94:1092–1095. [Europe PMC free article] [Abstract] [Google Scholar]
- Burbelo PD, Dreschsel D, Hall A. A conserved binding motif defines numerous candidate target proteins for both Cdc42 and Rac GTPases. J Biol Chem. 1995;270:29071–29074. [Abstract] [Google Scholar]
- Chen LM, Hobbie S, Galan JE. Requirement of Cdc42 for Salmonella-induced cytoskeletal and nuclear responses. Science. 1996;274:2115–2118. [Abstract] [Google Scholar]
- Chew TL, Masaracchia RA, Goeckeler ZM, Wysolmerski RB. Phosphorylation of non-muscle myosin II regulatory light chain by p21-activated kinase (γ-PAK) Muscle Res Cell Motil. 1998;19:839–854. [Abstract] [Google Scholar]
- Clague MJ, Thorpe C, Jones AT. Phosphatidylinositol 3-kinase regulation of fluid phase endocytosis. FEBS Lett. 1995;367:272–274. [Abstract] [Google Scholar]
- Cupers P, Veithen A, Kiss A, Baudhuin P, Courtoy PJ. Clathrin polymerization is not required for bulk-phase endocytosis in rat fetal fibroblasts. J Cell Biol. 1994;127:725–735. [Europe PMC free article] [Abstract] [Google Scholar]
- Damke H, Baba T, Warnock DE, Schmid SL. Induction of mutant dyamin specifically blocks endocytic coated vesicle formation. J Cell Biol. 1994;127:915–934. [Europe PMC free article] [Abstract] [Google Scholar]
- Daniels RH, Hall PS, Bokoch GM. Membrane targeting of p21-activated kinase 1 (PAK1) induces neurite outgrowth from PC12 cells. EMBO J. 1998;17:754–764. [Europe PMC free article] [Abstract] [Google Scholar]
- Dharmawardhane S, Sanders LC, Martin SS, Daniels RH, Bokoch GM. Localization of p21-activated kinase 1 (PAK1) to pinocytic vesicles and cortical actin structures in stimulated cells. J Cell Biol. 1997;138:1265–1278. [Europe PMC free article] [Abstract] [Google Scholar]
- Dowrick P, Kenworthy P, McCann B, Warn R. Circular ruffle formation and closure lead to macropinocytosis in hepatocyte growth factor/scatter factor-treated cells. Eur J Cell Biol. 1993;61:44–53. [Abstract] [Google Scholar]
- Edwards DC, Sanders LC, Bokoch GM, Gill GN. Activation of LIM-kinase by Pak1 couples Rac/Cdc42 GTPase signaling to actin cytoskeletal dynamics. Nat Cell Biol. 1999;1:253–259. [Abstract] [Google Scholar]
- Erickson A, Siegbahn A, Westmark B, Heldin C-H, Claesson-Welsh L. PDGF α, and β-receptors activate unique and common signal transduction pathways. EMBO J. 1992;11:543–550. [Europe PMC free article] [Abstract] [Google Scholar]
- Francis CL, Ryan TA, Jones BD, Smith SJ, Falkow S. Ruffles induced by Salmonella and other stimuli direct macropinocytosis of bacteria. Nature. 1993;364:639–642. [Abstract] [Google Scholar]
- Hewlett LJ, Prescott AR, Watts C. The coated pit and macropinocytic pathways serve distinct endosome populations. J Cell Biol. 1994;124:689–703. [Europe PMC free article] [Abstract] [Google Scholar]
- Hopkins CR. Intracellular routing of transferrin and transferrin receptors in epidermal carcinoma A431 cells. Cell. 1983;35:321–330. [Abstract] [Google Scholar]
- Kiosses WB, Daniels HR, Otey C, Bokoch GM, Schwartz MA. A role for p21-activated kinase in endothelial cell migration. J Cell Biol. 1999;147:831–843. [Europe PMC free article] [Abstract] [Google Scholar]
- Knaus UG, Bokoch GM. The p21Rac/Cdc42-activated kinases (PAKs) Int J Biochem Cell Biol. 1998;30:857–862. [Abstract] [Google Scholar]
- Knaus UG, Morris S, Dong H, Chernoff J, Bokoch GM. Regulation of human leukocyte p21-activated kinases through G protein-coupled receptors. Science. 1995;269:221–223. [Abstract] [Google Scholar]
- Manser E, Leung T, Salihuddin H, Zhao Z, Lim L. A brain serine/threonine protein kinase activated by Cdc42 and Rac1. Nature. 1994;367:40–46. [Abstract] [Google Scholar]
- Manser E, Huang H, Lou T, Chen X, Dong J, Leung T, Lim L. Expression of constitutively active α-PAK reveals effects of the kinase on actin and focal complexes. Mol Cell Biol. 1997;17:1129–1143. [Europe PMC free article] [Abstract] [Google Scholar]
- Racoosin EL, Swanson JA. Macrophage colony-stimulating factor (rM-CSF) stimulates pinocytosis in bone marrow-derived macrophages. J Exp Med. 1989;170:1635–1648. [Europe PMC free article] [Abstract] [Google Scholar]
- Racoosin EL, Swanson JA. M-CSF-induced macropinocytosis increases solute endocytosis but not receptor-mediated endocytosis in mouse macrophages. J Cell Sci. 1992;102:867–880. [Abstract] [Google Scholar]
- Racoosin EL, Swanson JA. Labeling of endocytic vesicles using fluorescent probes for fluid-phase endocytosis. In: Celis JE, editor. Cell Biology Handbook. New York: Academic Press; 1994. pp. 375–380. [Google Scholar]
- Ramos E, Wysolmerski RB, Masaracchia RA. Myosin phosphorylation by human Cdc42-dependent S6/H4 kinase/γPAK from placenta and lymphoid cells. Recept Signal Transduct. 1997;7:99–110. [Abstract] [Google Scholar]
- Ridley AJ, Paterson HF, Johnston CL, Diekmann D, Hall A. The small GTP-binding protein Rac regulates growth factor-induced membrane ruffling. Cell. 1992;70:401–410. [Abstract] [Google Scholar]
- Sallusto F, Cella M, Danieli C, Lanzavecchia A. Dendritic cells use macropinocytosis and the mannose receptor to concentrate macromolecules in the major histocompatibility complex class II compartment: downregulation by cytokines and bacterial products. J Exp Med. 1995;182:389–400. [Europe PMC free article] [Abstract] [Google Scholar]
- Sanders LC, Matsumura F, Bokoch GM, de Lanerolle P. Inhibition of myosin light chain kinase by p21-activated kinase. Science. 1999;283:2083–2085. [Abstract] [Google Scholar]
- Sandvig K, van Deurs B. Selective modulation of the endocytic uptake of ricin and fluid phase markers without alteration in transferrin endocytosis. J Biol Chem. 1990;265:6382–6388. [Abstract] [Google Scholar]
- Sells MA, Boyd JT, Chernoff J. p21-Activated kinase 1 (Pak1) regulates cell motility in mammalian fibroblasts. J Cell Biol. 1999;145:837–849. [Europe PMC free article] [Abstract] [Google Scholar]
- Sells MA, Chernoff J. Emerging from the Pak: The p21-activated protein kinase family. Trends Cell Biol. 1997;7:162–167. [Abstract] [Google Scholar]
- Sells MA, Knaus UG, Ambrose D, Bagrodia S, Bokoch GM, Chernoff J. Human p21 activated kinases regulate actin reorganization in mammalian cells. Curr Biol. 1997;7:202–210. [Abstract] [Google Scholar]
- Shockett P, DiFilippantonio M, Hellman N, Schatz DG. A modified tetracycline-regulated system provides autoregulatory inducible gene expression in cultured cells and transgenic mice. Proc Natl Acad Sci USA. 1995;92:6522–6526. [Europe PMC free article] [Abstract] [Google Scholar]
- Swanson JA. Fluorescent labeling of endocytic compartments. In: Wang Y, Taylor DL, editors. Methods in Cell Biology. New York: Academic Press. pp.; 1989a. pp. 137–142. [Abstract] [Google Scholar]
- Swanson JA. Phorbol esters stimulate macropinocytosis and solute flow through macrophages. J Cell Sci. 1989b;94:135–142. [Abstract] [Google Scholar]
- Swanson JA, Johnson MT, Beningo K, Post P, Mooseker M, Araki N. A contractile activity that closes phagosomes in macrophages. J Cell Sci. 1999;112:307–316. [Abstract] [Google Scholar]
- Swanson JA, Watts C. Macropinocytosis. Trends Cell Biol. 1995;5:424–427. [Abstract] [Google Scholar]
- Tang Y, Chen Z, Ambrose D, Liu J, Gibbs JB, Chernoff J, Field J. Kinase deficient Pak1 mutants inhibit Ras transformation of Rat-1 fibroblasts. Mol Cell Biol. 1997;17:4454–4464. [Europe PMC free article] [Abstract] [Google Scholar]
- Tuazon PT, Traugh JA. Activation of actin-activated ATPase in smooth muscle by phosphorylation of myosin light chain with protease-activated kinase I. J Biol Chem. 1984;259:541–546. [Abstract] [Google Scholar]
- West MA, Bretscher MS, Watts C. Distinct endocytotic pathways in epidermal growth factor-stimulated human carcinoma A431 cells. J Cell Biol. 1989;109:2731–2739. [Europe PMC free article] [Abstract] [Google Scholar]
- Westmark B, Siegbahn A, Heldin C-H, Claesson-Welsh L. β-Type receptor for platelet derived growth factor mediated a chemotactic response by means of ligand-induced activation of the receptor protein-tyrosine kinase. Proc Natl Acad Sci USA. 1990;87:128–132. [Europe PMC free article] [Abstract] [Google Scholar]
- Zenke FT, King CC, Bohl BP, Bokoch GM. Identification of a central phosphorylation site in p21-activated kinase regulating autoinhibition and kinase activity. J Biol Chem. 1999;274:32565–32573. [Abstract] [Google Scholar]
- Zhang S, Han J, Sells MA, Chernoff J, Knaus UG, Ulevitch RJ, Bokoch GM. Rho family GTPases regulate p38 MAP kinase through the downstream mediator PAK1. J Biol Chem. 1995;270:23934–23936. [Abstract] [Google Scholar]
- Zhao ZS, Manser E, Chen ZQ, Chong C, Leung T, Lim L. A conserved negative regulatory region in αPAK inhibition of PAK kinases reveals their morphological roles downstream of Cdc42 and Rac1. Mol Cell Biol. 1998;18:2153–2163. [Europe PMC free article] [Abstract] [Google Scholar]
Articles from Molecular Biology of the Cell are provided here courtesy of American Society for Cell Biology
Full text links
Read article at publisher's site: https://doi.org/10.1091/mbc.11.10.3341
Read article for free, from open access legal sources, via Unpaywall:
https://europepmc.org/articles/pmc14996?pdf=render
Citations & impact
Impact metrics
Citations of article over time
Alternative metrics
Smart citations by scite.ai
Explore citation contexts and check if this article has been
supported or disputed.
https://scite.ai/reports/10.1091/mbc.11.10.3341
Article citations
Cellular Regulation of Macropinocytosis.
Int J Mol Sci, 25(13):6963, 26 Jun 2024
Cited by: 0 articles | PMID: 39000072
Review
Aichivirus A1 replicates in human intestinal epithelium and bronchial tissue: Lung-gut axis?
Virus Res, 342:199338, 23 Feb 2024
Cited by: 0 articles | PMID: 38373599 | PMCID: PMC10901855
The extracellular matrix supports breast cancer cell growth under amino acid starvation by promoting tyrosine catabolism.
PLoS Biol, 22(1):e3002406, 16 Jan 2024
Cited by: 1 article | PMID: 38227562 | PMCID: PMC10791009
SP-R210 isoforms of Myosin18A modulate endosomal sorting and recognition of influenza A virus infection in macrophages.
Microbes Infect, 26(3):105280, 21 Dec 2023
Cited by: 0 articles | PMID: 38135024
Targeting the RAS/RAF/MAPK pathway for cancer therapy: from mechanism to clinical studies.
Signal Transduct Target Ther, 8(1):455, 18 Dec 2023
Cited by: 56 articles | PMID: 38105263 | PMCID: PMC10725898
Review Free full text in Europe PMC
Go to all (186) article citations
Similar Articles
To arrive at the top five similar articles we use a word-weighted algorithm to compare words from the Title and Abstract of each citation.
Localization of p21-activated kinase 1 (PAK1) to pinocytic vesicles and cortical actin structures in stimulated cells.
J Cell Biol, 138(6):1265-1278, 01 Sep 1997
Cited by: 139 articles | PMID: 9298982 | PMCID: PMC2132543
Association of PI-3 kinase with PAK1 leads to actin phosphorylation and cytoskeletal reorganization.
Mol Biol Cell, 13(8):2946-2962, 01 Aug 2002
Cited by: 75 articles | PMID: 12181358 | PMCID: PMC117954
Temporal and spatial distribution of activated Pak1 in fibroblasts.
J Cell Biol, 151(7):1449-1458, 01 Dec 2000
Cited by: 99 articles | PMID: 11134074 | PMCID: PMC2150672
Spatiotemporal regulation of the Pak1 kinase.
Biochem Soc Trans, 33(pt 4):646-648, 01 Aug 2005
Cited by: 27 articles | PMID: 16042564
Review
Funding
Funders who supported this work.
BHP HRSA HHS (1)
Grant ID: AHA-9930121N
NCI NIH HHS (1)
Grant ID: CA-58689
NIGMS NIH HHS (3)
Grant ID: GM-39434
Grant ID: R01 GM039434
Grant ID: R01 GM054168