Abstract
Free full text

In Vivo Inhibition of Anti-Hepatitis B Virus Core Antigen (HBcAg) Immunoglobulin G Production by HBcAg-Specific CD4+ Th1-Type T-Cell Clones in a hu-PBL-NOD/SCID Mouse Model
Abstract
Hepatitis B virus (HBV) core antigen (HBcAg)-specific CD4+ T-cell responses are believed to play an important role in the control of human HBV infection. In the present study, HBcAg-specific, HLA-DR13*-restricted CD4+ Th1-type T-cell clones were generated which secreted both gamma interferon and tumor necrosis factor alpha after in vitro antigen stimulation. These HBcAg-specific CD4+ Th1-type T cells were able to lyse HBc peptide-loaded Epstein-Barr virus-transformed lymphoblastoid target cells in vitro. To examine whether these HLA-DR13*-restricted human CD4+ Th1 T cells also display the same cytotoxic effects in vivo, we transferred peripheral blood leukocytes (PBL) derived from HBV-infected donors or an HBV-naïve donor sharing the DR13*, together with the HBcAg-specific CD4+ Th1-type T cells and HBcAg, directly into the spleen of optimally conditioned Nod/LtSz-Prkdcscid/Prkdcscid (NOD/SCID) mice. The production of both secondary anti-HBc-immunoglobulin G (anti-HBc-IgG) and primary HBcAg-binding IgM in hu-PBL-NOD/SCID mice was drastically inhibited by HBcAg-specific CD4+ Th1-type T cells. No inhibition was observed when CD4+ Th1 cells and donor PBL did not share an HLA-DR13. These results suggest that HBcAg-specific CD4+ Th1 T cells may be able to lyse HBcAg-binding, or -specific, B cells that have taken up and presented HBcAg in a class II-restricted manner. Thus, HBcAg-specific CD4+ Th1-type T cells can modulate the function and exert a regulatory role in deleting HBcAg-binding, or -specific, human B cells in vivo, which may be of importance in controlling the infection.
The hepatitis B virus (HBV) is a small, enveloped virus with a circular, partially double-stranded DNA genome. It is a major cause of infectious liver disease throughout the world. The majority of acutely infected adults recover from the disease, whereas 5 to 10% become persistently infected and develop chronic liver disease. In contrast to adult infection, neonatally transmitted HBV infection is rarely cleared, and the majority of those infants become chronically infected.
Most studies suggest that HBV is not directly cytopathic and immune responses to HBV antigens are responsible for the viral clearance and disease pathogenesis. Antiviral CD8+ T cells are believed to play a major role in the control of HBV infection by virtue of their capacity to identify and kill virus-infected cells (8). Recent studies suggest that viral clearance requires additional cytotoxic T lymphocyte (CTL) functions besides their ability to kill infected cells and that noncytopathic antiviral mechanisms are considered very important in the control of disease (19, 20). It was recently shown that HBV core antigen (HBcAg)-binding B cells are common even in a naive host (5, 27). HBcAg-binding B cells, which take up HBcAg and present viral peptides through class II molecules, may represent up to 15% of the B-cell repertoire in a naive host (5, 27). This suggests that HBV has targeted HBcAg to B cells, although the importance of this targeting is still unknown.
During acute self-limited HBV infection, a vigorous HBcAg-specific HLA class II-restricted CD4+ T-cell response is observed, while the HLA class II-restricted, HBV surface antigen (HBsAg)-specific response appears much less vigorous (14, 25). The HBcAg-specific fraction of peripheral blood T cells in acute self-limited hepatitis B selectively secrete Th1-type cytokines, suggesting that Th1-mediated effects may contribute not only to liver cell injury but probably also to recovery from disease and successful control of infection (35). It is becoming increasingly evident that the HBcAg-specific CD4+ T-cell response may play an important role in viral clearance by providing help for the growth and maturation of B cells and CD8+ T cells, by being directly cytotoxic for the infected targets or by modulating the viral replication via secretion of cytokines such as gamma interferon (IFN-γ) and tumor necrosis factor alpha (TNF-α) (29).
HBsAg-specific HLA class II-restricted CD4+ cytotoxic T-cell clones have been isolated from the liver of chronic active hepatitis B patients and from the peripheral blood leukocytes (PBL) of HBsAg-vaccinated individuals (4, 7). However, the role of HLA class II-restricted HBsAg- and HBcAg-specific CD4+ cytotoxic T cells in the HBV infection, protection, and pathogenesis is not well-defined. There is no direct way to demonstrate in humans that the HLA class II-restricted CD4+ cytotoxic T cells, which have been described in several human viral infections (4, 16, 24, 43), have the same cytotoxic capacity in vivo as in vitro.
In the present study, HBcAg-specific HLA class II-restricted CD4+ T-cell clones were generated from the PBL of a DR13-positive subject that had fully recovered from an acute self-limited HBV infection. These HBcAg-specific CD4+ Th1-type T cells partially expressed CD56 and were able to lyse the human target cells (Epstein-Barr virus [EBV]-transformed lymphoblastoid cell lines [LCLs]) in vitro. In vivo experiments in the hu-PBL-NOD/SCID mouse model revealed that HBcAg-specific CD4+ Th1 T cells drastically inhibited the production of HBcAg-specific antibodies, suggesting that these cells were able to specifically lyse the HBcAg-specific human B cells that had taken up and processed HBcAg. These CD4+ Th1-type cytotoxic T cells may exert a regulatory role on the HBcAg-specific antibody production by deleting HBcAg-specific (or -binding) B cells in vivo during natural HBV infection and thus contribute to the successful control of virus and recovery of HBV infection of DR13-positive patients.
MATERIALS AND METHODS
Subjects and HLA typing.
One subject who fully recovered from an acute HBV infection 5 years prior to the study (AHB), two HBsAg-seropositive chronic hepatitis B patients (CHB1 and CHB2), and one healthy donor (HD) without serological signs of past or present exposure to HBV were studied. All subjects gave written informed consent to participate in this study that was approved by the Ethical Review Board of the Ghent University Hospital, Ghent, Belgium.
HLA-DP, -DQ, and -DR haplotypes were determined with INNO-LiPA (Innogenetics N.V., Zwijnaarde, Belgium) according to the manufacturer's guideline as described previously (10). HLA class II haplotypes of those subjects were (i) for AHB, DRB1*1302, DRB1*0401, DPB1*0401, DPB1*0401, DQB1*0302, and DQB1*0604; (ii) for CHB1, DRB1*03*, DRB1*13*, DPB1*0201, DPB1*02012, DQB1*02*, and DQB1*0604; (iii) for CHB2, DRB1*0301, DRB1*0401, DPB1*0401, DPB1*0401, DQB1*0201/2, and DQB1*0201/2; and (iv) for HD, DRB1*13*, DRB1*0701, DPB1*1001, and DPB1*1101.
Preparation of PBL suspension.
PBL were isolated from fresh heparinized blood of the four subjects by Ficoll-Hypaque (Nycomed Pharma, Oslo, Norway) density gradient centrifugation. PBL were extensively washed, suspended in phosphate-buffered saline, and kept on ice until analyzed or transferred into recipient mice.
Reagents.
HBcAg is a 21-kDa protein that assembles to form a nucleocapsid particle. Recombinant HBcAg (rHBcAg) (ayw subtype), expressed in Escherichia coli was purchased from DiaSorin, Saluggia, Italy. Seventeen overlapping 20-mer peptides covering the entire HBV core sequence (ayw subtype) (amino acids 1 to 183) were synthesized with a multiple-peptide synthesizer using standard 9-fluorenylmethoxy carbonyl chemistry (Syro, MultiSynTech, Bochum, Germany).
The following mouse anti-human monoclonal antibodies were used for cytometry analysis: anti-CD3, anti-CD4, anti-CD8, and anti-CD56 (all from Becton Dickinson Benelux N.V., Aalst, Belgium). Mouse anti-human HLA class I; anti-HLA-DP, -DQ, and -DR antibodies; and mouse isotype control antibodies were from Serotec (Oxford, United Kingdom).
Generation of HBcAg-specific T-cell clones.
PBL (4 × 106 cells/ml/well) from the subject AHB were stimulated with rHBcAg (0.5 μg/ml) in a 24-well plate in RPMI 1640 medium supplemented with 25 mM HEPES, penicillin (50 U/ml), streptomycin (50 μg/ml), and 2 mM l-glutamine (all from Life Technologies, Inc., Grand Island, N.Y.); 5 ×10−5 M 2-mercaptoethanol (Sigma Chemical Co., St. Louis, Mo.); and 10% heat-inactivated human AB+ serum. After 5 days, fresh complete medium containing human recombinant interleukin-2 (rIL-2) (10 U/ml; Eurocetus, Leiden, The Netherlands) was added to the cultures. T-cell clones were generated on day 12 by culturing the cells at limiting dilution at 0.3 cell per well into 96-well flat-bottom plates in the presence of rHBcAg (0.1 μg/ml), human rIL-2 (5 U/ml), and irradiated autologous PBL (3 ×104 PBL/well). Every 2 weeks the cultures were restimulated with rHBcAg and irradiated autologous PBL (2,500 rads of gamma radiation) as antigen presenting cells (APCs). Eight weeks later, the growing T-cell clones were tested for HBcAg specificity, and the HBcAg-specific clones were subsequently analyzed for their fine specificity by confronting them with a series of overlapping synthetic HBc peptides. The phenotype of HBcAg-specific T-cell clones was analyzed by flow cytometry (FACScan; Becton Dickinson) with different mouse monoclonal antibodies.
Proliferation and restriction assay.
Proliferation assays of T-cell clones were performed by incubating 2 ×104 T cells per well for 4 days in the presence of irradiated (2,500 rads) autologous PBL as APCs (5 ×104 PBL/well). All cultures were performed in triplicate. Eighteen hours before harvesting, 0.5 μCi of [3H]thymidine was added, and the radioactivity incorporated by the cells was determined by β-counting (11).
In order to determine the restriction of the T-cell recognition, blocking assays were performed wherein the T-cell proliferation was measured in the presence of mouse anti-human HLA class I and class II (HLA-DR, -DP, and -DQ) antibodies and isotypic control antibodies (1 μg/ml). In confirmatory assays the T-cell proliferation was measured following antigen presentation by HLA-typed allogeneic, haploidentical PBL.
Intracellular cytokine staining and cytokine quantitation.
For intracellular cytokine staining, HBcAg-specific T cells were activated in vitro with phorbol myristate acetate (20 ng/ml) and ionomycin (1 μmol/ml) in the presence of brefeldin A (10 μg/ml). The stimulated T cells were stained with anti-CD4 monoclonal antibody (fluorescein isothiocyanate labeled; Becton Dickinson Benelux N.V.) and fixed with paraformaldehyde (IC-Fix; BioSource Europe SA). Following this, the cells were stained with a R-phycoerythrin-labeled anti-human IFN-γ, TNF-α, or IL-4 antibody (BioSource Europe SA) in the presence of a permeabilizing agent (IC-Perm; BioSource Europe SA). The stained cells were then analyzed by flow cytometry.
Supernatants from the proliferation assays of HBcAg-specific T-cell clones stimulated by HBcAg were collected at 72 h and kept frozen at −20°C until tested. IFN-γ, TNF-α, and IL-5 were quantitated by commercial kits (MEDGENIX IFN-γ EASIA kit and human TNF-α immunoassay kit [BioSource Europe SA]; Genzyme human interleukin-5 kit [Genzyme Diagnostics, Cambridge, Mass.]).
In vitro cytotoxicity assay.
Cytotoxicity of cloned T cells was tested in a 4-h JAM (“just another method”) assay described by P. Matzinger (28). Effector cells were incubated in triplicate in U-bottom microtiter wells containing 0.5 × 104 or 1 × 104 [3H]thymidine-labeled target cells. The target cells were either autologous or allogeneic EBV-transformed LCLs, which were previously labeled with [3H]thymidine (7.5 μCi/ml) for 20 h, and loaded with HBc peptide (50 μg/ml) during an overnight incubation. The content of the wells was harvested after 4 h of incubation at 37°C. Specific lysis was calculated according to the following formula: 100 × [labeled DNA (3H) retained in the absence of effector cells − labeled DNA (3H) retained in the presence of effector cells]/labeled DNA (3H) retained in the absence of effector cells. Spontaneous lysis was less than 20%.
Mice and intrasplenic injection of human PBL and T-cell clones.
Homozygous Nod/LtSz-Prkdcscid/Prkdcscid (NOD/SCID) mice were bred and maintained under specific-pathogen-free conditions in the animal facility of the Department of Clinical Biology, Microbiology, and Immunology of Ghent University. All mice used in this study were 9 to 12 weeks old. One day before the transfer of human cells, NOD/SCID mice were irradiated (300 rads of gamma irradiation) and received intraperitoneal injections of 1 mg of TMβ1 in 0.5 ml of phosphate-buffered saline to reduce endogenous mouse natural killer activity. TMβ1 is a rat monoclonal antibody directed against the mouse IL-2 receptor β chain (39). We previously showed that TMβ1 could strongly improve human PBL survival in SCID mice (41). All manipulations were performed by experienced hands, and the study protocol was approved by the local animal ethics committee.
HBcAg-specific CD4+ Th1-type T cells were boosted in vitro with irradiated autologous PBL and HBcAg. Starting 5 days later, the cells were fed and expanded triweekly with fresh complete RPMI 1640 medium containing rIL2 (10 U/ml [final concentration]). These HBcAg-specific T cells were ready for transfer after 2 weeks of culture. Human PBL derived from two chronic hepatitis B patients and one healthy donor were isolated. These fresh human PBL (107 cells/per mouse) with or without added HBcAg-specific CD4+ T cells (2 ×106 cells/per mouse) and with or without added HBcAg (10 μg per mouse) were then injected into the spleen of the conditioned NOD/SCID mice. The detailed procedure of the intrasplenic transplantation of human cells has been described (9). Plasma of each of the recipient chimeric NOD/SCID mice was collected at day 7 and 14 after cell transfer to determine human immunoglobulin and HBcAg-specific antibody concentrations.
Determination of total human immunoglobulin and antigen-specific antibodies.
Human total IgG in the plasma of chimeric NOD/SCID mice was determined with an in-house enzyme-linked immunosorbent assay (ELISA) as described (41). For the determination of anti-HBc-IgG, the plasma of the chimeric NOD/SCID mice was serially diluted from 1/50 to 1/25,600 and measured by the in-house ELISA method described previously (5). A signal was considered positive when the absorbance value of the diluted samples was three times higher than that of a negative control plasma from the healthy donor. The results are expressed as the end-point dilution of each plasma. Anti-HBc-IgM was measured with a commercial ELISA kit (ETI-CORE-IGMK-2; DiaSorin).
Statistical analysis.
Comparisons between groups were done using the Mann-Whitney U test.
RESULTS
Phenotypic and functional characterization of HBcAg-specific T-cell clones derived from a subject that recovered from an acute HBV infection.
Thirty HBcAg-specific T-cell clones were generated from a subject who recovered from an acute hepatitis B infection. Ten of these HBcAg-specific T-cell clones have been analyzed, and all recognized the HBc peptide spanning amino acids 141 to 160 (presented in a separate paper [T. Cao, I. Desombere, M. Sällberg, and G. Leroux-Roels, submitted for publication]). Three of these HBcAg-specific T-cell clones (clones 121, 124, and 135) were characterized in detail and selected for the experiments described herein. Phenotypic analysis by flow cytometry showed that these T-cell clones were CD3+, CD4+, CD8− T cells, and partially coexpressed CD56 (16%) (Fig. (Fig.1).1). To investigate the HLA restriction of the HBcAg-specific T-cell clones, we analyzed their ability to proliferate in response to antigen in the absence or presence of mouse monoclonal anti-HLA class I and class II antibodies and isotypic controls. As shown in Fig. Fig.2,2, T-cell clones 124 and 135 were HBcAg-specific, and their proliferation in response to HBcAg was blocked (90 and 80%, respectively) by anti-human HLA-DR monoclonal antibody. Clone 121 was also HLA-DR restricted (data not shown). Additional experiments (not shown here) showed the restriction element to be the HLA-DRB1*13* molecule.

Phenotypic analysis of HBcAg-specific T-cell clone 121. HBcAg-specific T cells were double stained with different monoclonal antibodies (Becton Dickinson) and analyzed by flow cytometry. Clones 124 and 135 displayed the same pattern.
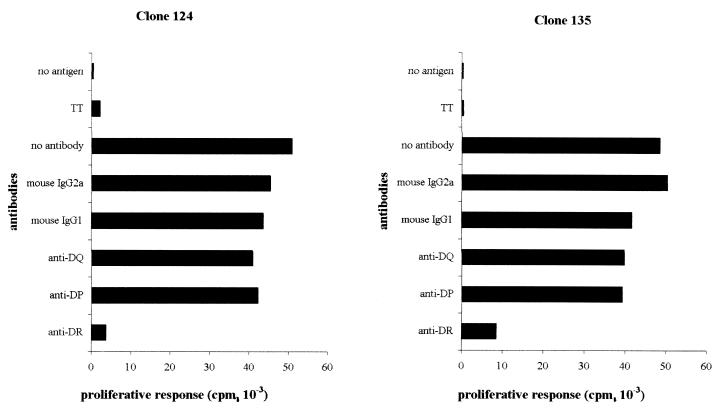
HLA restriction of T-cell clones 124 and 135. HBcAg-specific T-cell clones were stimulated by HBcAg (0.1 μg/ml) and irradiated (2,500 rads) autologous PBL as APCs in the absence or presence of mouse anti-human HLA-DR, -DP, and -DQ antibodies and isotypic controls (1 μg/ml; Serotec). [3H]thymidine (0.5 μCi/well; Amersham International, Little Chalfont, United Kingdom) was added 18 h before harvesting on day 4. They were then assayed for [3H]thymidine incorporation by liquid scintillation counting in an LKB-Wallac 8100 counter (LKB, Bromma, Sweden). All cultures were performed in triplicate, and the data are expressed as the mean counts per minute. TT, tetanus toxoid.
Intracellular cytokine staining following phorbol myristate acetate-ionomycin stimulation showed that the HBcAg-specific T cells produced both IFN-γ and TNF-α, but no IL-4 (Fig. (Fig.3).3). The HBcAg-induced lymphokine secretion in the supernatant of the proliferation assays of these clones was also quantified with commercial ELISA kits for IFN-γ, TNF-α, and IL-5. The HBcAg-induced TNF-α production of clones 121, 124, and 135 was 857, 960, and 900 pg/ml, respectively. The production of IFN-γ was 528, 575, and 463 U/ml, respectively, and that of IL-5 was 141, 61, and 133 pg/ml, respectively. These results indicate that the HBcAg-specific CD4+ T-cell clones display a predominant Th1 phenotype.
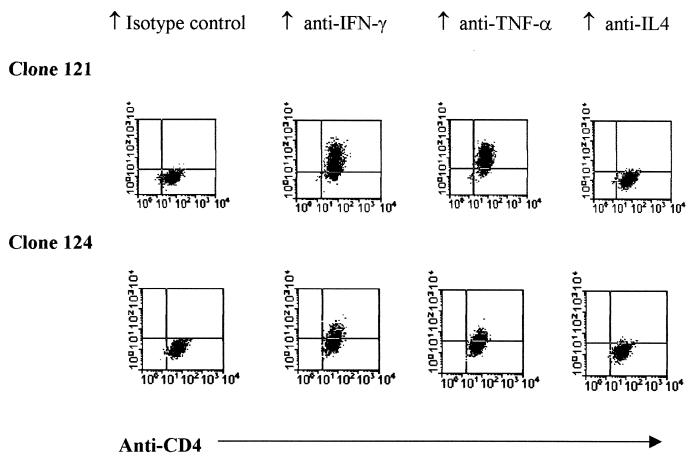
Intracellular cytokine staining of HBcAg-specific T-cell clones 121 and 124. Activated HBcAg-specific CD4 T cells were stained with both anti-CD4 (fluorescein isothiocyanate-labeled) and phycoerythrin-labeled anti-human cytokine (IFN-γ, TNF-α, and IL-4) antibodies as described. The two clones expressed both IFN-γ and TNF-α but no IL-4 and thus behaved like Th1-type CD4 cells.
In vitro cytotoxic activity of HBcAg-specific CD4-positive T-cell clones.
To examine whether the HBcAg-specific CD4+ T-cell clones displayed cytotoxic activity, we performed in vitro cytotoxicity assays. We selected a JAM assay format and a 4-h incubation period. The cytotoxic capacity of clones 121, 124, and 135 was examined using peptide-pulsed autologous and allogeneic LCLs (Fig. (Fig.44 and and5).5). When autologous EBV-transformed LCLs loaded with selected HBcAg-peptide were used as target cells, specific cell lysis was observed in an effector/target-dependent manner (Fig. (Fig.4).4). However, no lysis occurred when non-peptide-loaded autologous LCLs were used as targets. Cell lysis was also observed when allogeneic LCL targets sharing the restricted DR13 molecule were pulsed with the HBc peptide containing amino acids 141 to 160. No lysis was measured when another HBc peptide (amino acids 11 to 30) was used to pulse autologous LCLs, or when allogeneic LCLs target lacking DR13 molecule were pulsed with the HBc peptide containing amino acids 141 to 160 (Fig. (Fig.5).5). These data not surprisingly show that the proliferative and cytotoxic responses of the clones are governed by the same restricting element and show the same peptide specificity. However, the cytotoxic activities measured in the JAM assay were blocked by neither anti-class I antibody nor anti-HLA-DR antibody (data not shown), which differs from the proliferative responses that were largely blocked by anti-HLA-DR.
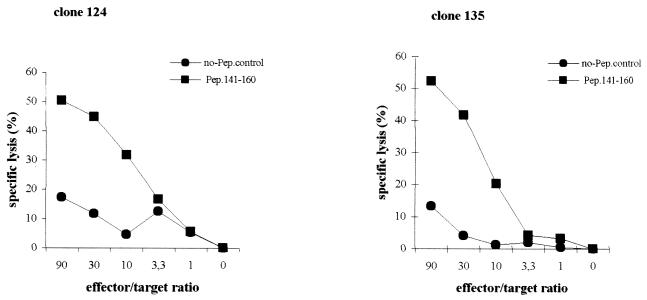
In vitro cytotoxic assays of HBcAg-specific CD4+ Th1 T-cell clones 124 and 135. JAM assays were performed as described in Materials and Methods. Peptide-loaded autologous EBV-transformed LCLs were used as target cells. Assays were set up at different effector/target ratios (90/1, 30/1, 10/1, 3.3/1, 1/1, and 0/1). All conditions were tested in triplicate. Specific lysis of target cells at different effector/target ratios is shown. Spontaneous lysis was always less than 20%.

HLA class II restriction of the cytotoxicity of HBcAg-specific CD4+ Th1 T-cell clones 124 and 135. JAM assays were performed as described. Based on the results of experiments shown in Fig. Fig.4,4, an effector/target ratio of 30/1 was selected in these experiments. Autologous and allogeneic EBV-transformed LCLs sharing different HLA class II molecules were loaded with the HBc peptide containing amino acids 141 to 160 and served as target cells. Specific lysis of the target cells is shown.
HBcAg-specific CD4-positive Th1 T-cell clones inhibit the secondary HBcAg-specific human IgG response in the hu-PBL-NOD/SCID mice in a HLA-DR13-restricted fashion.
We recently observed that injection of human PBL into the spleen of optimally conditioned NOD/SCID mice induced rapid and massive expansion of human B cells (9). In this model we further noticed that transfer of PBL from subjects with recovered or ongoing HBV infection induced an anti-HBc-IgG response that was enhanced by adding HBcAg to the PBL inoculum (5). To investigate whether our HBcAg-specific T-cell clones were able to lyse antigen-specific human B cells in the hu-PBL-NOD/SCID mice, we transferred human PBL (107 PBL/per mouse) from the two chronic HBV patients, CHB1 (HLA-DR13-positive subject) and CHB2 (HLA-DR13-negative subject), with cells of clone 135 (2 × 106 cells/per mouse) together with HBcAg (10 μg/per mouse) directly into the spleen of conditioned NOD/SCID mice. The following experimental groups were made: (i) group 1 consisted of three mice receiving PBL plus clone 135 cells plus HBcAg; (ii) the three mice of group 2 received PBL plus clone 135 cells and no HBcAg; (iii) the three mice of group 3 received PBL plus HBcAg and no clone 135 cells; and (iv) the three mice of group 4 received PBL alone. Plasma of each chimeric mouse was collected at day 7 and day 14 after cell transfer, and HBcAg-specific human antibodies were determined as described.
The data of this experiment are summarized in Table Table1.1. When only PBL from DRB1*13*-positive and DRB1*13*-negative chronic HBV patients were transferred into the mice, HBcAg-specific human IgG was detectable both at day 7 and day 14 after cell transfer (group 4). The addition of HBcAg to the PBL enhanced the production of HBcAg-specific human IgG (group 3). When HBcAg-specific CD4+ Th1-type T cells and HBcAg were added to PBL, a drastic reduction of the HBcAg-specific IgG production could be observed in the DR13-positive donor but not in the DR13-negative donor. This suggests that the cells of clone 135 executed an inhibitory effect on the HBcAg-specific IgG production and that this action was DR13 restricted (group 1). This effect was less pronounced when clone cells and PBL were injected without added HBcAg (group 2).
TABLE 1
HBcAg-specific CD4+ Th1 T cells inhibit in vivo production of HBcAg-specific human IgG in hu-PBL-NOD/SCID mice
Exptl groupa | Mouse | HBcAg-specific human IgG produced with PBL from CHB donorb
| |||
---|---|---|---|---|---|
HLA-DR13 positive
| HLA-DR13 negative
| ||||
Wk 1 | Wk 2 | Wk 1 | Wk 2 | ||
Group 1 | NOD1 | <1/50 | 1/200 | 1/3,200 | 1/12,800 |
NOD2 | <1/50 | 1/1,600 | 1/3,200 | 1/12,800 | |
NOD3 | <1/50 | 1/100 | 1/1,600 | 1/6,400 | |
Group 2 | NOD1 | 1/400 | 1/3,200 | 1/3,200 | 1/12,800 |
NOD2 | 1/1,600 | 1/3,200 | 1/3,200 | NA | |
NOD3 | 1/200 | 1/800 | |||
Group 3 | NOD1 | 1/1,600 | 1/12,800 | 1/3,200 | 1/25,600 |
NOD2 | 1/1,600 | 1/12,800 | 1/3,200 | 1/25,600 | |
NOD3 | 1/800 | 1/12,800 | 1/6,400 | 1/25,600 | |
Group 4 | NOD1 | <1/50 | 1/100 | 1/3,200 | NA |
NOD2 | 1/800 | 1/6,400 | 1/3,200 | NA | |
NOD3 | 1/800 |
We also determined the total human IgG concentration in the plasma of all mice, and the detailed results are shown in Table Table2.2. The total human IgG concentrations in group 1 and group 2 chimeric mice that were reconstituted with human PBL and T-cell clones, in the presence or absence of HBcAg, respectively, were not significantly different. In the chimeric mice that received human PBL, without T-cell clones, there were no differences for total human IgG between the group with HBcAg (group 3) and that without HBcAg (group 4). However, total human IgG levels in the chimeric mice receiving human PBL (the combined groups 3 and 4), but without T-cell clone, were significantly higher than those in the chimeric mice reconstituted with human PBL and T-cell clones (the combined groups 1 and 2) (P < 0.01). Moreover, in the HLA-DR13-positive donor, both at week 1 and 2 the difference of total human IgG levels between group 1 and group 3 was more pronounced than the difference between group 2 and group 4. The difference of total IgG levels between the DR13-positive and the DR13-negative donor is donor dependent, as has been shown previously (41).
TABLE 2
Production of total human IgG in human-mouse chimeras
Exptl groupa | n | Mean IgG ± SD (μg/ml) produced with PBL from CHB donorb
| |||
---|---|---|---|---|---|
HLA-DR13* positive
| HLA-DR13* negative
| ||||
Wk 1 | Wk 2 | Wk 1 | Wk 2 | ||
Group 1 | 3 | 132.3![]() ![]() | 1,870.8![]() ![]() | 466.3![]() ![]() | 3,875.9![]() ![]() |
Group 2 | 3 | 186.8![]() ![]() | 1,250.5![]() ![]() | 647.9![]() ![]() | 3,325.7c |
Group 3 | 3 | 318.6![]() ![]() | 2,762.1![]() ![]() | 874.3![]() ![]() | 6,553.5![]() ![]() |
Group 4 | 3 | 244.7![]() ![]() | 2,112.5c | 1,077.1![]() ![]() | Not available |
HBcAg-specific CD4-positive Th1 T cells inhibit the in vivo production of HBcAg-binding human IgM in hu-PBL-NOD/SCID mice.
We recently observed that HBcAg can induce naïve human B cells to secrete HBcAg-binding human IgM in hu-PBL-NOD/SCID mice (5). To examine whether HBcAg-specific T-cell clones interfered with this process, human PBL were isolated from an HLA-DR13*-positive naïve donor who has not been exposed to HBV. These cells (107 cells/animal) were (i) mixed with HBcAg-specific CD4+ T cells (2 × 106 cells/mouse) and HBcAg (10 μg/mouse) and injected into the spleens of three optimally conditioned NOD/SCID mice (group 1), (ii) mixed with HBcAg (10 μg/mouse) and injected into three mice (group 2), and (iii) injected into three mice without any additional cells or HBcAg (group 3). The HBcAg-binding human IgM in the plasma of the chimeric mice at days 7 and 14 after cell transfer was measured using an anti-HBc-IgM kit from DiaSorin. As shown in Table Table3,3, HBcAg-binding human IgM was found on day 7 and day 14 in the chimeric mice that were reconstituted with naïve human PBL and HBcAg. No HBcAg-binding IgM was produced in the control mice that were reconstituted with naïve human PBL alone. When T cells from clone 135 were added to the naïve human PBL plus HBcAg, no HBcAg-binding human IgM could be detected in the plasma of recipient mice at days 7 and 14 after cell transfer. These data suggest that the HBcAg-specific CD4+ Th1-type T cells inhibit or kill these naïve HBcAg-binding B cells, which results in a reduced production of HBcAg-binding human IgM in the chimeric mice.
TABLE 3
HBcAg-specific CD4+ Th1 T cells inhibit in vivo production of HBcAg-binding IgM in hu-PBL-NOD/SCID mice.
Exptl group | Mouse | HBcAg-binding IgM (A450/630) at:
| |
---|---|---|---|
Wk 1 | Wk 2 | ||
PBL + HBcAg + T cells | NOD1 | 0.035 | Not available |
NOD2 | 0.036 | 0.031 | |
NOD3 | 0.034 | 0.086 | |
PBL + HBcAg | NOD1 | 0.735 | 1.109 |
NOD2 | 0.086 | 0.329 | |
NOD3 | 1.935 | 1.634 | |
PBL + PBS | NOD1 | 0.041 | 0.026 |
NOD2 | 0.033 | 0.169 | |
NOD3 | 0.035 | 0.037 |
DISCUSSION
In the present study, we describe the phenotypic and functional characteristics of three HBcAg-specific T-cell clones that were generated from the peripheral blood leukocytes of a DR13-positive subject who had fully recovered from an acute HBV infection. These T-cell clones were CD4+, produced mainly Th1-type cytokines, and were endowed with cytotoxic capacity that was HLA-DR13* restricted and HBcAg specific. This capacity led to the lysis of HBcAg peptide-loaded EBV-transformed B cells in vitro and the drastic reduction of in vivo HBc antibody production by HBcAg-specific B cells in the hu-PBL-NOD/SCID mouse model. Our data indicate that cytotoxic CD4+ T cells exist in the peripheral blood of patients that recovered from an acute HBV infection. The inhibition of the in vivo HBc antibody production is likely due to the lysis of the HBcAg-specific human B cells mediated by CD4+ Th1 T cells as demonstrated in vitro. It is tempting to speculate that these HBcAg CD4+ Th1 T cells may exert a regulatory role in the HBcAg-specific antibody production by deleting HBcAg-specific (or -binding) B cells during natural HBV infection.
Ample experimental evidence is available that puts the existence of CD4+ cytotoxic T cells beyond doubt (13, 15, 22, 42, 31). Antigen-specific CD4+ cytotoxic T cells are not unique for the HBV infection since virus-specific HLA class II-restricted CD4+ cytotoxic T-cell clones have also been described in measles virus, dengue virus, and herpes simplex virus infections (4, 16, 24, 43). In the mouse experiment, in vivo-primed CD4+ T cells efficiently killed target cells (13, 31). However, it is hard to show, especially in humans, that the HLA class II-restricted CD4+ cytotoxic T cells display the same cytotoxic capacity in vivo as can be demonstrated in vitro.
Human PBL-SCID chimeras that allow the study of the human immune system without major ethical constraints have been utilized in different research fields and became a useful research tool in the past decade. Recently, we showed that pretreatment of SCID mice with TMβ1 greatly improved the survival of human PBL (41). We further observed that injection of human PBL directly into the spleen of the mice induced rapid and massive expansion of human B cells (9), which facilitates the study of the human B-cell function in the SCID mouse model. Finally, we noticed that the antigen-specific T cells retained their normal function during the early stage of human PBL engraftment in conditioned SCID and NOD/SCID mice (6).
Employing the human PBL-SCID mouse model, we observed here that in vivo production of secondary anti-HBc-IgG or primary HBcAg-binding IgM in the hu-PBL-NOD/SCID mouse model was drastically inhibited by the addition of HBcAg-specific CD4+ Th1 T-cell clone 135 in an HLA-DR13*-restricted manner. This in vivo inhibition of HBcAg-specific antibody production could be due to the lysis of HBcAg-specific human B cells by the CD4+ Th1 T-cell clone as demonstrated in vitro. The intrasplenic transfer of human PBL into the mice, known to induce rapid and massive expansion of human B cells in the spleen, is likely to provide a close cell-to-cell interaction and may therefore sensitize the human B cells to killing mediated by CD4+ Th1 cells. The mode of B-cell stimulation (CD40 ligand and anti-IgM) may also influence the susceptibility to Th1-mediated cytotoxicity (37).
We observed that our T-cell clones secreted both TNF-α and IFN-γ, but no IL-4, after in vitro antigen stimulation. The production of these Th1-type cytokines may contribute to the cytotoxic function of these clones. In transgenic mouse models of HBV infection, it has been shown that the antiviral and inflammatory cytokine profiles contribute to virus clearance (21, 33, 34). Both perforin and Fas/Fas ligand-mediated pathways for cell lysis were previously revealed in CD4+ T-cell clones (2, 22, 42). However, Ohta et al. (34) demonstrated that both IFN-γ and TNF-α, but not Fas ligand and perforin, contributed to the liver injury induced by HBsAg-specific Th1 cells. Nakamoto et al. (33) reported that IFN-γ-producing CTL kill the hepatocytes without Fas ligand and perforin in an antigen-specific manner. In different hepatitis models, IFN-γ and TNF-α were also demonstrated to play an important role in the pathogenesis of liver injury (17, 26, 32–34).
Virus-specific CD8+ cytotoxic T cells play an essential role in the final control and clinical outcome of virus infections. However, the importance of antigen-specific CD4+ T-cell responses in viral control has also been suggested in both HBV and HCV infection (14, 15, 18, 25). During acute self-limited and chronic active HBV infection, HBcAg-specific HLA class II-restricted CD4+ T-cell responses are observed (14, 25). Both in mice and in humans HBcAg preferentially elicits Th1 T-cell responses (30, 35).
We have previously shown that both humans and mice have a high frequency of HBcAg-binding B cells (5, 27). Whether this phenomenon has a beneficial or deleterious effect on the natural course of HBV infection is still unclear. The in vivo inhibition of the production of HBcAg-specific (or -binding) antibodies suggests that HBcAg-specific (or -binding) B cells could effectively present HBcAg to HBcAg-specific CD4+ T cells, which leads to the functional silencing and/or physical elimination of these B cells. This is possibly due to the lysis of the HBcAg-specific human B cells in vivo as it has been demonstrated in vitro. It is tempting to speculate that these HBcAg-specific CD4+ Th1 T cells may modulate HBcAg-specific antibody production during natural HBV infection and also exert a regulatory role by deleting HBcAg-specific (or-binding) human B cells in vivo. If HBcAg-specific (or -binding) B cells and/or the antibodies they produce would contribute to the persistence of HBV infections, a fact not yet established, the elimination of such B cells could then be a beneficial event that promotes viral clearance. Several studies have demonstrated that the antigen-specific B cells can be eliminated by CTL (3, 36, 38).
Finally, it has been clearly demonstrated that HLA-DR13 molecules are associated with a benign course of HBV infection. Thursz et al. (40) were the first to report that in a large study population in Gambia the MHC allele DRB1*1302 was associated with protection against persistent HBV infection both in children and in adults. This observation was subsequently confirmed by two other independent studies in Caucasians (23) and in Asian subjects (1). The study by Diepolder et al. (12) suggested that the beneficial effect of the HLA-DR13 allele on the outcome of HBV infection could result from a vigorous HBV core-specific CD4+ T-cell response. The present study indicates that HBcAg-specific CD4+ cytotoxic T cells exist in the peripheral blood of the DR13-positive patients with acute self-limited HBV infection. These may also contribute to the recovery of these patients from the disease and successful control of virus infection.
ACKNOWLEDGMENTS
Part of this work was supported by the Flemish Fund for Scientific Research-Flanders (NR:G.0022.00).
We thank T. Boterberg (Department of Experimental Oncology, Ghent University Hospital) for the irradiation of the mice. We are grateful to L. Verhoye (Center for Vaccinology, Department of Clinical Chemistry, Microbiology and Immunology, Ghent University) for the preparation of monoclonal antibody (TMβ1) and to Eurocetus (Leiden, The Netherlands) for the gift of human rIL-2.
REFERENCES
Articles from Journal of Virology are provided here courtesy of American Society for Microbiology (ASM)
Full text links
Read article at publisher's site: https://doi.org/10.1128/jvi.75.23.11449-11456.2001
Read article for free, from open access legal sources, via Unpaywall:
https://europepmc.org/articles/pmc114731?pdf=render
Citations & impact
Impact metrics
Citations of article over time
Alternative metrics
Smart citations by scite.ai
Explore citation contexts and check if this article has been
supported or disputed.
https://scite.ai/reports/10.1128/jvi.75.23.11449-11456.2001
Article citations
A Systematic Review of T Cell Epitopes Defined from the Proteome of Hepatitis B Virus.
Vaccines (Basel), 10(2):257, 08 Feb 2022
Cited by: 6 articles | PMID: 35214714 | PMCID: PMC8878595
Review Free full text in Europe PMC
Negative HBcAg in immunohistochemistry assay of liver biopsy is a predictive factor for the treatment of patients with nucleos(t)ide analogue therapy.
J Cell Mol Med, 22(3):1675-1683, 29 Nov 2017
Cited by: 1 article | PMID: 29193766 | PMCID: PMC5824392
Fighting Viral Infections and Virus-Driven Tumors with Cytotoxic CD4+ T Cells.
Front Immunol, 8:197, 27 Feb 2017
Cited by: 21 articles | PMID: 28289418 | PMCID: PMC5327441
Review Free full text in Europe PMC
Induction of humoral and cell-mediated immune responses by hepatitis B virus epitope displayed on the virus-like particles of prawn nodavirus.
Appl Environ Microbiol, 81(3):882-889, 21 Nov 2014
Cited by: 19 articles | PMID: 25416760 | PMCID: PMC4292494
Go to all (11) article citations
Other citations
Wikipedia
Protocols & materials
Related Immune Epitope Information - Immune Epitope Database and Analysis Resource
Similar Articles
To arrive at the top five similar articles we use a word-weighted algorithm to compare words from the Title and Abstract of each citation.
Hepatitis B virus core antigen binds and activates naive human B cells in vivo: studies with a human PBL-NOD/SCID mouse model.
J Virol, 75(14):6359-6366, 01 Jul 2001
Cited by: 38 articles | PMID: 11413302 | PMCID: PMC114358
Plasmacytoid dendritic cells induce efficient stimulation of antiviral immunity in the context of chronic hepatitis B virus infection.
Hepatology, 56(5):1706-1718, 27 Aug 2012
Cited by: 25 articles | PMID: 22707082
Molecular basis for the interaction of the hepatitis B virus core antigen with the surface immunoglobulin receptor on naive B cells.
J Virol, 75(14):6367-6374, 01 Jul 2001
Cited by: 43 articles | PMID: 11413303 | PMCID: PMC114359