Abstract
Free full text

Structural and functional aspects of liver sinusoidal endothelial cell fenestrae: a review
Abstract
This review provides a detailed overview of the current state of knowledge about the ultrastructure and dynamics of liver sinusoidal endothelial fenestrae. Various aspects of liver sinusoidal endothelial fenestrae regarding their structure, origin, species specificity, dynamics and formation will be explored. In addition, the role of liver sinusoidal endothelial fenestrae in relation to lipoprotein metabolism, fibrosis and cancer will be approached.
Introduction
Liver sinusoidal endothelial cells (LSEC) constitute the sinusoidal wall, also called the endothelium, or endothelial lining. The liver sinusoids can be regarded as unique capillaries which differ from other capillaries in the body, because of the presence of open pores or fenestrae lacking a diaphragm and a basal lamina underneath the endothelium. The first description and electron microscopic observation of LSEC fenestrae was given by Wisse in 1970 [1]. The application of perfusion fixation to the rat liver revealed groups of fenestrae arranged in sieve plates. In subsequent reports, Widmann [2] and Ogawa [3] verified the existence of fenestrae in LSEC by using transmission electron microscopy (TEM). In general, endothelial fenestrae measure 150–175 nm in diameter, occur at a frequency of 9–13 per μm2, and occupy 6–8% of the endothelial surface in scanning electron microscopy (SEM) (Fig. (Fig.1)1) [4]. In addition, differences in fenestrae diameter and frequency in periportal and centrilobular zones were demonstrated; in SEM the diameter decreases slightly from 110.7 ± 0.2 nm to 104.8 ± 0.2 nm, whereas the frequency increases from 9 to 13 per μm2, resulting in an increase in porosity from 6 to 8 % from periportal to centrilobular [5]. Other ultrastructural characteristics of LSEC are: the presence of numerous bristle-coated micropinocytotic vesicles and many lysosome-like vacuoles in the perikaryon, indicating a well developed endocytotic activity. The nucleus sometimes contains a peculiar body, the sphaeridium [1,6].
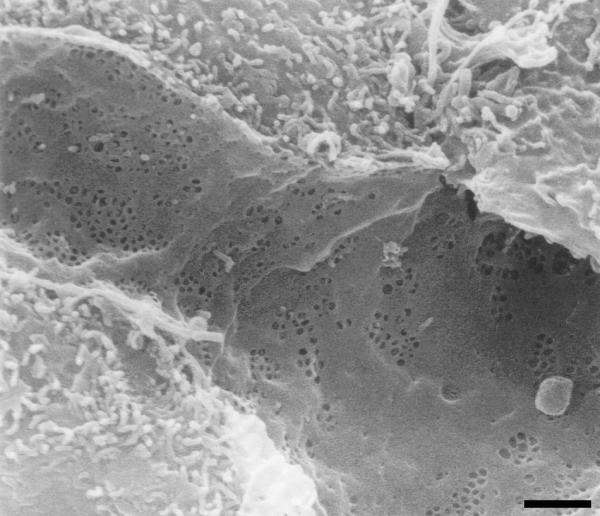
Low magnification scanning electron micrograph of the sinusoidal endothelium from rat liver showing the fenestrated wall. Notice the clustering of fenestrae in sieve plates. Scale bar, 1 μm.
On the basis of morphological and physiological evidence, it was reported that the grouped fenestrae act as a dynamic filter [7-9]. Fenestrae filter fluids, solutes and particles that are exchanged between the sinusoidal lumen and the space of Disse, allowing only particles smaller than the fenestrae to reach the parenchymal cells or to leave the space of Disse (see § Role of liver sinusoidal endothelial cell fenestrae in relation to lipoprotein metabolism and atherosclerosis).
Another functional characteristic of LSEC is their high endocytotic capacity. This function is reflected by the presence of numerous endocytotic vesicles and by the effective uptake of a wide variety of substances from the blood by receptor-mediated endocytosis [10]. This capacity, together with the presence of fenestrae and the absence of a regular basal lamina, makes these cells different and unique from any other type of endothelial cell in the body. In general, LSEC can be regarded: (I) as a "selective sieve" for substances passing from the blood to parenchymal and fat-storing cells, and vice versa, (II) and as a "scavenger system" which clears the blood from many different macromolecular waste products, which originate from turnover processes in different tissues [10,11].
Liver sinusoidal endothelial cell fenestrae
The capillary endothelium plays a central and active role in regulating the exchange of macromolecules, solutes and fluid between the blood and the surrounding tissues. The high permeability of capillary endothelium to macromolecules, solutes and water are reflected in the presence of special transporting systems represented by vesicles, channels, diaphragms and fenestrae. Actually, endothelial transport appears to be a very complex process in which the substances are transported according to their size, charge and chemistry. Some substances are delivered to and processed by the endothelial cell itself (endocytosis), whereas others are transported across the endothelium to the surrounding tissues (transcytosis). In case of the capillaries of the liver, LSEC transport substances simultaneously along both pathways [12,13].
Besides endocytosis and transcytosis, endothelial transport in the liver sinusoidal endothelium occurs through fenestrae without a diaphragm. During this process the endosomal and lysosomal compartments are bypassed. The exchange of fluids, solutes and particles is bidirectional, allowing an intensive interaction between the sinusoidal blood and the microvillous surface of the parenchymal cells. LSEC-fenestrae measure between 100 and 200 nm in diameter, and appear to be membrane bound round cytoplasmic holes (Fig. (Fig.1).1). Their morphology resembles that of a sieve, suggesting their filtration effect (Fig. (Fig.2).2). In the past decade, many challenging questions regarding the ultrastructure of LSEC-fenestrae has been addressed, including: what determines the structure and size of fenestrae? (see § Contraction and dilatation mechanism of fenestrae); and, how are fenestrae formed? (see § Formation of fenestrae).
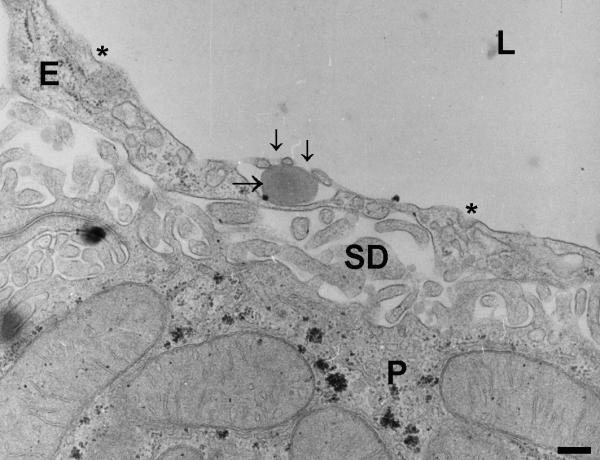
High-magnification transmission electron micrograph of a hepatic sinusoid of rat liver, fixed by perfusion-fixation with glutaraldehyde, postfixed in osmium, dehydrated in alcohol, and embedded in Epon (reference[1]). The lumen of the sinusoid (L) is lined by the endothelium (E), showing the presence of fenestrae (small arrows) and coated pits (asterisks). Note a lipid particle (large arrow) which passed the fenestrae, illustrating the sieving effect of fenestrae. The space of Disse (SD) contains numerous microvilli of the parenchymal cells (P). (Courtesy of Drs R. De Zanger, reference [7]). Scale bar, 300 nm.
In the following paragraphs some aspects of LSEC-fenestrae regarding their origin, species specificity, dynamics and formation will be discussed. In addition, the role of LSEC-fenestrae in relation to lipoprotein metabolism, fibrosis and cancer will be approached.
Fenestrae in fetal and postnatal liver tissue
In contrast with the large amount of information available on LSEC-fenestrae in the mature liver [11], very few studies have been performed to discern the fenestration patterns in the sinusoids of fetal [14-18] and postnatal [14-19] liver. Naito and Wisse [14] provided the first evidence that fetal LSEC contain fenestrae. Unlike the adult rat liver, the fetal LSEC possess fenestrae consistently spanned by a diaphragm. However, open fenestrations, typical of adult liver, appear around 17 days gestation, increasing in number for the remainder of the gestation. In addition, Barberá-Guillem et al. [16,17] found an insignificant variation in the number of fenestrae in the fetal (18–21 days of gestation) to adult period of LSEC in the periportal zone. However, it becomes three times larger in the adult liver sinusoids in the centrilobular zone than in the fetal ones. This rise in the number of fenestrae in the centrilobular zone starts at day one of the newborn and proceeds in the subsequent neonatal period. During the fetal stage the porosity, i.e., the accumulated surface of fenestrae, was three times greater in the centrilobular than in the periportal zone. This difference decreases only slightly after birth: although large fenestrae disappear, they are replaced by smaller ones, characteristic of the adult liver.
These zonal variations in fenestration pattern suggest that in the fetal period a regional definition of the microcirculatory endothelium within the context of the liver lobule already exists, although the definition is different from that of adult liver endothelium. Evidence for this was found by relating the fenestration pattern with the hemopoietic activity of the liver: i.e., large fenestrae in the periportal zone disappear in the fetal period which coincides with the reduction of hemopoietic activity in this domain, and large fenestrae persist in the centrilobular zone of newborn livers until all hemopoietic activity disappear, suggesting the involvement of fenestrae in the transendothelial passage of new blood cells [15-17].
Bankston et al. [15] illustrated that fetal LSEC already have a sieving function, by injecting colloidal carbon into 14–22 day gestation fetuses via de umbilical vein. Although fenestrae possessing diaphragms are permeable to carbon before 16 days gestation, open fenestrae, first seen at 17 days gestation, allowed large amounts of carbon to reach the extravascular space. In addition, Naito and Wisse [19] could demonstrate the role of the liver sieve in lipoprotein metabolism in newborn rats. When neonatal rats drank mother's milk, a condition of physiological hyperlipemia, numerous chylomicrons with a size smaller than fenestrae could be observed in the space of Disse. These results indicate the existence of a substantial filtration effect of endothelial fenestrations in the newborn rat. The researchers concluded that circulating chylomicrons larger than the largest diameter of endothelial fenestrations are unable to pass through the endothelial lining to reach the space of Disse.
Thus, LSEC are present in fetal liver at all gestational ages and fenestrae, diaphragmed or non-diaphragmed, provide a direct communication between the sinusoidal lumen and the space of Disse. The postnatal liver endothelium shares the morphological features and permeability properties of adult liver sinusoidal endothelium.
Fenestrae in different species
Since the first description of LSEC-fenestrae in 1970 by Wisse [1], fenestrae have been the object of numerous studies in various species. Although most studies have been performed in rats and mice, LSEC-fenestrae have been described in many other animals, including fish, birds, and many mammals (Table (Table1).1). Although differences in diameter and number of fenestrae exist, the ultrastructure of LSEC is the same across species, i.e., all LSEC are characterised by their long cytoplasmatic extensions containing fenestrae clustered in so-called sieve plates.
Table 1
Fenestration pattern in different species Brief overview of fenestrae characteristics of different species. Notice the large variations in diameter and number of fenestrae between the different species. The reported data from this table were obtained by at random measurements along the sinusoids. "n.d." = no data available. Data are expressed as mean ± S.D. In case of baboon, human and rainbow trout the data correspond with the minimum and maximum diameter or number of fenestrae measured.
Species (ref.) | Diameter (nm) | Number of fenestrae / μm2 |
Rat [25] | 98.0 ± 13.0 | 20.0 ± 6.3 |
Mouse [26] | 99.0 ± 18.0 | 14.0 ± 5.0 |
Rabbit [27] | 59.4 ± 4.8 | 17.3 ± 3.8 |
Chicken [23] | 89.6 ± 17.8 | 2.9 ± 0.3 |
Baboon [20] | 92 – 116 | 1.4 – 1.9 |
Human [28] | 50 – 300 | 15 – 25 |
Rainbow trout [21] | 75 – 120 | n.d. |
Sheep [29] | 60.0 ± 1.9 | n.d. |
Goat [30] | n.d. | n.d. |
Cat [31] | n.d. | n.d. |
Moreover, not only may the diameter and number of fenestrae vary from species to species, but also between individuals of a species, and within a single individual under the influence of various physiological and pharmacological circumstances (see § Dynamic changes of fenestrae). Fenestrae of both sexes of a species appear to be similar [20,21].
According to information available to date, it seems likely that variations in fenestration pattern between different species may explain the susceptibility of different species to dietary cholesterol. For example, in comparison with the rat, rabbits have smaller fenestrae and chickens have fewer fenestrae. Thus, both species have a liver sieve of lower porosity than the rat, resulting in a prolonged circulation of cholesterol-rich chylomicrons which are considered to be atherogenic. This correlates well with the vulnerability of rabbits and chickens to dietary cholesterol, resulting in hyperlipoproteinemia and the development of atherosclerosis [22-24] (see also § Role of fenestrae in lipoprotein metabolism and atherosclerosis).
Dynamic changes of fenestrae
LSEC-fenestrae are dynamic structures, whose diameter and number vary in response to a variety of hormones, drugs, toxins, diseases or even to changes in the underlying extracellular matrix (for an overview, see Table Table2).2). Structural integrity of the fenestrated sinusoidal liver endothelium is believed to be essential for the maintenance of a normal exchange of fluids, solutes, particles and metabolites between the hepatocytes and sinusoidal blood. Its alteration can have adverse effects on hepatocytes and liver function in general [4]. In the past twenty years, numerous publications appeared about the role of these dynamic structures under various physiological and pathological situations (Table (Table2).2). Their role and involvement in processes such as lipoprotein metabolism [32], hypoxia [33], endotoxic shock [34], virus infection [35], cirrhosis [36], fibrosis [37] and liver cancer [38] has been explored.
Table 2
Overview of agents and experimental conditions which alter the fenestrae diameter and number Overview of fenestrae dynamics under various experimental and pathological conditions. Notice that conflicting data concerning the dynamics in fenestrae diameter after treatment with cytochalasin B were reported. In addition, contradictory results concerning the alterations in fenestral number also exists and were described after phorbol myristate acetate and endotoxin treatment, or when LSEC were cultured on laminin. These discrepancies in fenestral dynamics can probably be explained by the different experimental designs, influencing culture conditions and species specificity.
Fenestrae alterations by | Diameter | Number | ||
Increase | Decrease | Increase | Decrease | |
Acethylcholine [43-45] | + | - | ? | ? |
Adrenalin [43,45] | - | + | ? | ? |
Bethanechol [43,45] | + | - | ? | ? |
Calmodulin antagonist W-7 [40] | + | - | ? | ? |
Carbon tetrachloride [37,46] | + | - | - [46] | + [46] |
Cocaine combined with ethanol [47] | ? | ? | - | + |
Collagen, IV-V [48] | - | - | + | - |
Cytochalasin B [26,35,40,42,45,48-56] | + [40,45,51] | + [52,55] | + | - |
Diethylnitrosamine [57-59] | ? | ? | - | + |
Dimethylnitrosamine [39,60-62] | - [60] | - [60] | - | + |
Endothelin [63] | - | + | - | + |
Endotoxin [34,62,64-66] | + [65] | + [34,66] | + [64] | + [34,62,66] |
Ethanol, acute dose [37,51,53,54,67-69] | + | - | - | - |
Ethanol, chronic dose [20,22,28,70,71] | + [20,70] | - [20,70] | - | + |
Fatty liver [72,73] | ? | ? | - | + |
Hypoxia [33] | + | - | ? | ? |
Hepatectomy [74] | + | - | - | + |
Hepatitis virus, type 3 [35,75] | - [35] | + [35] | - | + |
Ionophore A23187 [40,45] | - | + | ? | ? |
Irradiation [33] | + | - | ? | ? |
Isoproterenol [45] | + | - | ? | ? |
Jasplakinolide [76] | - | + | + | - |
Laminin [48,77] | - [48] | - [48] | + [48], - [77] | - [48], + [77] |
Latrunculin A [55,78] | - | + | + | - |
Misakinolide [79] | - | + | + | - |
Neuropeptide Y [45] | - | + | ? | ? |
Noradrenalin [44,45,80] | - | + | ? | ? |
Nicotine [25,81,82] | - | + | - | + |
Pantethine [81,82] | + | - | ? | ? |
Phalloidin [50] | + | - | ? | ? |
Phorbol myristate acetate [83,84] | - [84] | - [84] | + [83], - [84] | - [83], + [84] |
Pressure [41,85] | + | - | ? | ? |
Prostaglandine E1[63,86] | + | - | ? | ? |
Serotonin [53,54,80,84,87-90] | - | + | ? | ? |
Swinholide A [79] | - | + | + | - |
Temperature, 4°C [42] | ? | ? | - | + |
Thioacetamide [36,91] | - | + | - | + |
Tumor cells [38,92-97] | - [38,92] | + [38,92] | - | + |
Tumor necrosis factor-alpha [98] | ? | ? | - | + |
Vasoactive intestinal peptide [45] | + | - | ? | ? |
References connected to the agents and experimental conditions mean unanimity in the literature of the observed fenestral dynamics; references connected to symbols indicate that the described effects are only reported in the corresponding literature; "+" = Yes; "-" = No; and "?" = no data available.
To date, a widely accepted hypothesis stipulates that drugs which dilate fenestrae, such as pantethine, acethylcholine or ethanol improve the extraction of dietary cholesterol from the circulation; whereas drugs such as nicotine, long-term ethanol abuse, adrenalin, noradrenalin or serotonin, which decrease the endothelial porosity, play a role in the development of drug- and stress-related atherogenesis. Although this hypothesis was postulated by others [5,20,28], it was mainly the group of Fraser et al. [32] who actually demonstrated that drugs which alter the fenestral diameter and number also affect the pathogenesis of atherosclerosis, by increasing or decreasing the access of atherogenic lipoproteins to the hepatocytes.
An exciting development in the field of fenestral dynamics has been the exploration of the mechanisms by which hepatotoxins, such as ethanol, endotoxin, carbon tetrachloride, dimethylnitrosamine and thioacetamide, induce defenestration. In general, it has been noted that defenestration of the sinusoidal endothelium occurs early in the pathogenesis of cirrhosis, both in humans exposed to hepatotoxins and in animal models of cirrhosis. This process seems to be reversible upon removal of the hepatotoxin and before the formation of an endothelial basement membrane [32]. In addition, it was demonstrated that defenestration not only contributes to the genesis of hyperlipoproteinaemia, but also blocks retinol metabolism and therefore probably promotes perisinusoidal fibrosis by altering fat-storing cell function [39]. However, the exact mechanism by which these hepatotoxins bring about the defenestration remains to be elucidated. Although nothing realistic can be said about this aspect, it might be possible that the reduction of fenestrae may occur either by fusion or extensive constriction and disappearance of fenestrae through membrane fusion [4].
Another emerging field comprises the recent studies which explore the mechanism whereby hormones and cytoskeletal altering drugs change the fenestral diameter and number. From these studies it became clear that drugs which alter the calcium concentration within LSEC also change the fenestrae diameter [40] (see § Contraction and dilatation mechanism of fenestrae), whereas drugs which interfere with the LSEC-cytoskeleton mainly alter the number of fenestrae [26] (see § Formation of fenestrae).
Finally, peculiar reports appeared describing fenestral dynamics in various pathological conditions of the liver, such as hypoxia [33], increasing venous pressure [41], irradiation [33], cold storage [42] and invasion of the liver by metastatic tumor cells [38] or viruses [35].
Contraction and dilatation mechanism of fenestrae
Current interest focuses on the mechanisms by which LSEC alter the diameter of fenestrae (for reviews, see references [83] and [89]). Immunoelectron microscopic studies on LSEC in the early 80's revealed the first information regarding the structural basis for the contraction and dilatation machinery of fenestrae. Oda et al. [43] described in 1983 the presence of actin filaments in the neighbourhood of fenestrae, indicating that the cytoskeleton of LSEC plays an important role in the modulation of fenestrae. In the following years this notion was supported by several authors, they all confirmed the presence of actin [87,99], myosin [51,100] and calmodulin [99-102] in LSEC by using immunofluorescence microscopy.
Van Der Smissen et al. [51] and Oda et al. [102] postulated at first in 1986 that a calcium-calmodulin-actomyosin system around fenestrae has probably a key role in the regulation of the fenestral diameter. This hypothesis was nicely proven in subsequent years by studying the role of calcium ions and calmodulin in fenestrae regulation using immunoelectron microscopy [99,101], electron microprobe analysis [102], microfluometric digital image analysis [40] and patch clamp technique [88]. Oda et al. [103] showed that the addition of a calcium ionophore to LSEC induced fenestral contraction. This contraction could be suppressed by chelating extracellular calcium or by pretreatment of LSEC with a calmodulin antagonist, demonstrating the messenger function of calcium ions and the role of the intracellular Ca2+-receptor calmodulin in the fenestrae diameter regulation. In addition, Arias [83] and Arias et al. [83,100] demonstrated that the serotonin induced contraction of fenestrae occurs together with phosphorylation of the 20-kd subunit of myosin light chain kinase. All these findings suggest the crucial role of a calcium-calmodulin-actomyosin complex in the regulation of the fenestral diameter [40] (Fig. (Fig.33).
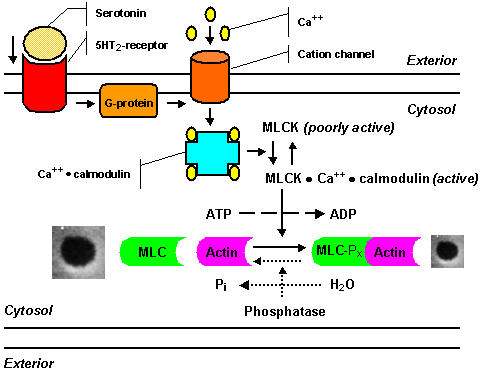
Scheme of the serotonin signal pathway showing the steps in fenestral contraction and relaxation, as postulated by Gatmaitan et al.[89,90,104]: Serotonin binds to a ketanserin-inhibitable receptor, coupled to a pertussis-toxin sensitive G-protein; a calcium channel opens, causing an influx of calcium ions; the intracellular calcium level increases rapidly, and calcium binds to calmodulin; the calcium-calmodulin complex activates myosin light chain kinase, and as a result phosphorylation of the 20-kd light chain of myosin occurs, resulting in an increased actin-activated myosin ATPase activity, which finally initiates contraction of fenestrae. The mechanism for the relaxation of LSEC fenestrae is presently unclear and probably involves dephosphorylation of myosin light chains as represented by dashed lines: a decrease in the cytosolic free calcium concentration leads to dissociation of calcium and calmodulin from the kinase, thereby inactivating myosin light chain kinase, under these conditions myosin light chain phosphatase, which is not dependent on calcium for activity, dephosphorylates myosin light chain and finally causes relaxation of fenestrae.
Brauneis et al. [88] demonstrated that fenestral contraction induced by serotonin is associated with an increment of intracellular calcium, using a serotonin-sensitive cation channel with permeability to calcium. In addition, Gatmaitan et al. [89,90] illustrated that fenestral contraction induced by serotonin could be abolished by preincubation of LSEC with (I) the Ca2+-channel blockers verapamil and dilthiazem, (II) the serotonin antagonist ketanserin, illustrating that LSEC contain a serotonin receptor type 5HT2, and (III) pertussis-toxin, suggesting that the 5HT2-receptor may be coupled to a pertussis-toxin sensitive G-protein. Arias and co-workers [89,90,104] postulated a sequence of events as presented in Figure Figure33.
Oda et al. [63] and Yokomori et al. [86] demonstrated the possible role of a plasma membrane Ca2+-ATPase in the dilatation of fenestrae by studying the effect of prostaglandin E1 on LSEC. They found that prostaglandin E1 enhances the Ca2+-pump ATPase activity in the neighbourhood of the plasmamembrane of LSEC fenestrae, leading to the dilatation of fenestrae due to the extrusion of cytoplasmic calcium. Oda et al. [63] postulated that an active extrusion of cytoplasmic free calcium leads to an actomyosin-mediated relaxation of the LSEC fenestrae. This statement was nicely illustrated by treating LSEC with endothelin, i.e., endothelin attenuated the Ca2+-pump ATPase activity and at the same time an increased level of cytoplasmic calcium and fenestrae contraction could be observed.
The results of our whole mount electron microscopic studies on LSEC have added the following new insights in the structure and function of the cytoskeleton in fenestrated areas [42,53]: (I) Sieve plates and fenestrae are delineated by cytoskeleton elements; (II) fenestrae are delineated by a filamentous, fenestrae-associated cytoskeleton ring (FACR) (Fig. (Fig.4)4) with a mean filament thickness of 16 nm, (III) sieve plates are surrounded by a ring-like orientation of microtubuli, which form a network together with additional branching cytoskeletal elements; (IV) because of the fact that the fenestrae-associated cytoskeleton ring opens and closes like fenestrae in response to different treatments such as ethanol or serotonin, it is assumed that this ring regulates the size changes of the fenestrae; and (V) therefore, the fenestrae-associated cytoskeleton probably controls the important function of endothelial filtration.
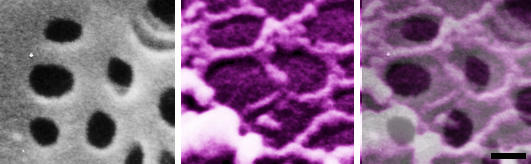
High-power scanning electron micrographs of a nonextracted (left); and of a formaldehyde prefixed, cytoskeleton buffer extrated rat liver sinusoidal endothelial cell (middle). Notice the grouped fenestrae on the cell surface (left); and a remarkable series of rings of fenestrae-associated cytoskeleton (middle). Layering a colored scanning electron micrograph on top of the cell surface of a nonextracted rat liver sinusoidal endothelial cell clearly illustrates the relation between both structures (right). Scale bar, 200 nm.
Formation of fenestrae
In 1986, Steffan et al. [49,105] provided the first evidence that LSEC fenestrae are inducible structures. Treatment of LSEC in situ and in vitro with the microfilament-inhibiting drug cytochalasin B resulted in an increased number of fenestrae. Scanning electron microscopic observations of detergent-extracted LSEC revealed that the increase in the number of fenestrae was related to an alteration of the cytoskeleton. In addition, the effect of cytochalasin B on the number of fenestrae and cytoskeleton could be reversed after removal of the drug. However, when LSEC were treated with colchicine, a microtubule-disrupting agent, there was no effect on the number of fenestrae, thereby demonstrating that microtubules are not involved in the formation of the endothelial pores [26]. These observations indicate that fenestrae are dynamic structures which may undergo changes in number in response to local external stimuli and that the actin-cytoskeleton has a major role in this process.
Later, Bingen et al. [52] noticed, in freeze-fracture replicas of cytochalasin B-treated LSEC, areas which were more or less devoid of intramembrane particles having the size of fenestrae. In general, they proposed that fenestrae are formed by fusion between opposite sheets of plasma membrane which are depleted of intramembrane particles. These authors proposed the following sequence in the process of fenestrae formation: (I) The process begins with the depletion of intramembrane particles in small areas; (II) then follows an encirclement of these zones by a microridge devoid of intramembrane particles; subsequently (III), membrane fusion occurs with small pores appearing inside the marked zones; and finally (IV), the size of the pore increases to reach that of a well recognizable fenestra and the microridge vanishes progressively.
This hypothesis was elaborated by Taira [106], who found some new evidence about the formation of sieve plates. The study of the luminal cell membrane of freeze-fractured LSEC revealed the presence of trabecular meshworks which were attached to the E and P-faces of the cell membrane of both the cell body and the attenuated cell processes. Trabecular meshworks are a cell membrane-attached reticulum of anastomosing trabeculae composed of the cell membrane and cytosol, and the surface appears as a sieve on the cell membrane. Taira [106] postulated the following sequence of events in the formation of sieve plates: (I) The process starts with the formation of plasmalemmal invaginations which are triggered by external stimuli; (II) Rapid clustering of these cell membrane invaginations would then occur by some yet unknown mechanism, followed by ballooning and fusion of these invaginations; (III) As a consequence, the cytosol located among plasmalemmal invaginations becomes thinner and remains as anastomosing trabeculae in trabecular meshworks; (IV) which in turn give rise to the formation of sieve plates by flattening.
In the past we demonstrated that treatment of LSEC with latrunculin A (Fig. (Fig.5),5), swinholide, misakinolide, jasplakinolide, halichondramide or dihydrohalichondramide, all induces an increased number of fenestrae [79,107]. However, only by treating LSEC with misakinolide or dihydrohalichondramide, we were able to capture a novel structure indicative of fenestrae formation, which we propose to call fenestrae-forming center (FFC) (Fig. (Fig.6)6) [79,107]. This illustrates the importance of the use of different anti-actin drugs to study the dynamic cellular processes that depend on the integrity and function of actin. A comparison of all anti-actin agents tested so far, revealed that the only biochemical activity that misakinolide and dihydrohalichondramide have in common is their barbed end capping activity; this activity seems to slow down the process of fenestrae formation to such extent that it becomes possible to resolve fenestrae-forming centers.
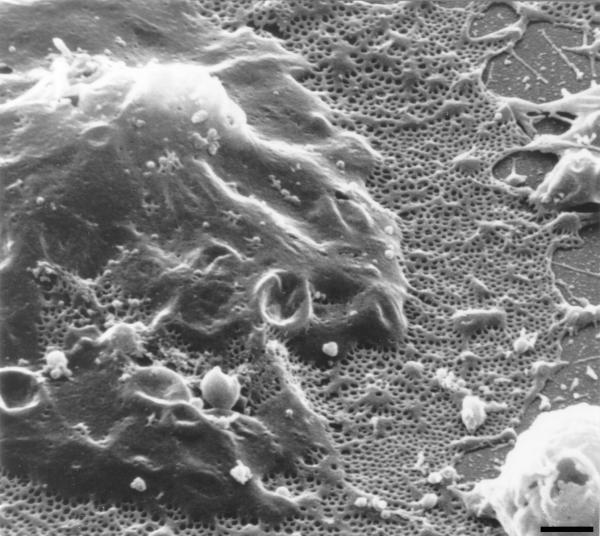
Scanning electron micrograph of an LSEC treated with 0.1 μg/ml of latrunculin A for 2 hours, showing huge fenestrated areas. Thin cytoplasmic arms divide flat fields containing numerous fenestrae. The bulging area corresponds with the nucleus. Scale bar, 2 μm.
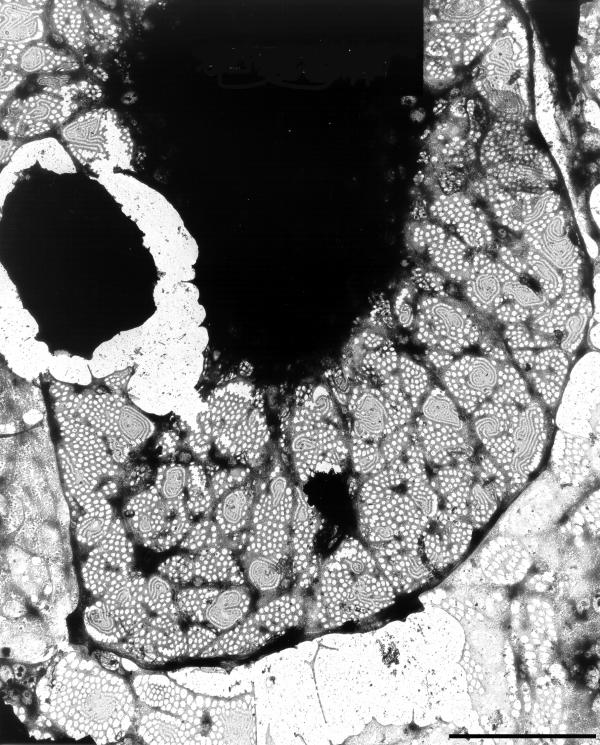
Whole-mount transmission electron micrograph of an LSEC treated with 100 nM dihydrohalichondramide for 1 hour, showing the dark nuclear area and surrounding extracted cytoplasm. Note the presence of small cytoplasmatic areas of intermediated density within the fenestrated cytoplasm. In several of these areas a very peculiar structure could be observed, consisting of rows of fenestrae with increasing diameter, fanning out into the surrounding cytoplasm, connected to the small cytoplasmatic areas with their smallest fenestrae. These structures are suggestive of de novo fenestrae formation and we therefore named them "fenestrae-forming center" (FFC) [79]. Scale bar, 5 μm.
Role of fenestrae in lipoprotein metabolism and atherosclerosis
Dietary fats, absorbed by the epithelium of the small intestine, are assembled with specific apolipoproteins to form chylomicrons, which have a size between 100 and 1000 nm. After synthesis by the enterocytes, the triglyceride-rich chylomicrons are secreted into the mesenteric lymph and extracellular fluid to eventually enter the systemic circulation via the thoracic duct. Once into the circulation, triglycerides are hydrolysed to fatty acids in the capillaries of adipose tissue and muscles through the action of lipoprotein lipase present on the endothelium of capillaries. The resulting smaller particles have a mean diameter of 90–250 nm and are called chylomicron-remnants, which are taken up rapidly by the apo E (remnant) receptors of the liver parenchyma. Before hepatic recognition and uptake of chylomicron remnants can occur, these remnants must first enter the space of Disse through the fenestrated sinusoidal endothelium [4,32,108]. Wisse [1] suggested at first that fenestrae might play an important role in the exchange of lipid particles between the sinusoidal blood and the parenchymal cells. This hypothesis of sieving was mainly elaborated by Fraser and co-workers [22-25,27], who demonstrated a filtration effect by comparing chylomicrons in the portal blood with those in the space of Disse, illustrating that the largest particles in the space of Disse were as large as fenestrae.
It must be noticed that large chylomicrons are small in number. However, their relative volume, as a third power function, may account for 70 to 80% of the total circulating fat. This fraction is obviously not admitted to the space of Disse and it is well known that these triglyceride-rich lipoproteins are atherogenic [109]. On the other hand, the smaller admitted chylomicrons are richer in cholesterol and their uptake influences the de novo synthesis and excretion of cholesterol by the parenchymal cells [110]. This suggests a relationship between fenestrae and the pathogenesis of atherosclerosis.
Since 1978 [8], there have been many studies supporting the hypothesis that the liver sieve plays a role in lipoprotein metabolism and the occurrence of atherosclerosis [32]. For example, among the mammals, rabbit livers have smaller fenestrae, whose average diameter sharply contrasts with that of similar endothelial pores in rodents (Table (Table1).1). Wright et al. [24] found that rabbits fed cholesterol rapidly develop high serum cholesterol levels which lead to the development of atheroslerosis. The researchers found that the small size of endothelial fenestrae in the liver sinusoids of rabbits hinders the egress of large chylomicron remnants from the sinusoidal blood, explaining the subsequent development of hypercholesterolemia and atherosclerosis. In addition, chylomicron remnants were rapidly removed from the circulation by the liver in cholesterol fed rats. Besides the different dimensions of fenestrae among rats and rabbits, variations in size of chylomicrons and differences in liver cell membrane receptors were taken into account. They concluded that different sieving capacities of chylomicron remnants in different species by the liver may largely explain the differences in lipoprotein metabolism among the species.
Microscopic studies have shown that drugs which alter the porosity of the liver sieve also affect the pathogenesis of atherosclerosis, by increasing or decreasing the access of atherogenic lipoproteins to the hepatocytes [32]. Nicotine, fed orally to rats at a dose equivalent to that of a human being smoking two packets of cigarettes per day, induces a decline in liver sieve porosity and a concomitant increase in serum cholesterol levels, illustrating that this decreased porosity may be an etiological factor in the known correlation between tobacco smoking and atherosclerosis [25,81,82]. Conversely, when the hypolipidemic agent pantethine was fed with cholesterol to rabbits, it halved the resultant hypercholesterolemia and at the same time the porosity was increased by 80% [81,82].
Other evidence supporting the hypothesis that the liver sieve has a key role in lipoprotein metabolism comes from studies where the effect of ethanol was studied on fenestrae. Alcoholic defenestration, resulting from chronic alcohol abuse, induces a decrease in the number of fenestrae in rats [71], baboons [20] and human beings [22,28], resulting in a decrease in sinusoidal porosity. This effect has been postulated as a factor in the pathogenesis of the hyperlipoproteinemia associated with alcoholism [37]. The hyperlipoproteinemia and the simultaneous decrease in porosity of the liver sieve observed in an alcoholic patient seems to support this notion [22]. In addition, abstinence has been shown to allow the restoration of the fenestrated sinusoidal endothelium in the alcoholic human [72]. On a short time basis, alcohol enlarges fenestrae [51,67,70]. It has been suggested that this phenomenon enhances the hepatic clearance of dietary lipids accounting, in part, for the protection from atherosclerosis ascribed to low-dose alcohol consumption [32]. On the other hand, the development of acute fatty liver after alcohol consumption is probably caused by a more porous sieve, allowing the admission of large triglyceride-rich chylomicrons to contact and be taken up by the parenchymal cells [70]. Evidence for this can be found in the work of Morsiani et al. [74], who demonstrated that the transient fatty liver occurring after partial hepatectomy correlated with an increase in sinusoidal porosity.
Other important drugs believed to be essential in the process of fenestral sieving are serotonin, adrenalin and noradrenalin which decrease the sinusoidal porosity. Their effects have been suggested as possible factors in stress-related atherogenesis [43,45,80]. However, to what extent these hormones contribute to the process of atherogenesis is not yet fully understood.
There also exists a reverse pathway for the transport of lipid, in the form of very low density lipoproteins (VLDL), which assemble in the Golgi apparatus of the parenchymal cell, are transported in vesicles to the sinusoidal cell surface and secreted into the space of Disse. These lipoprotein particles have a size of about 90 nm which allows them to pass the endothelial filter and as a consequence the fenestrae should not act as a barrier [19,111]. However, important questions remain to be answered about the role of the liver sieve in reverse lipid transport; for instance, (I) how does VLDL reach the sinusoidal blood in the defenestrated liver? and (II) does the liver sieve act as a barrier when enlarged VLDL-particles, formed during high rates of synthesis of triglyceride, want to pass the fenestrae? In both pathological circumstances, it was suggested that VLDL may leave the space of Disse via an alternate exit, namely via the hepatic lymphatics and the thoracic duct [32,112]; however, this has not yet been proven.
The filtration of lipoproteins by open pores is the simplest mechanism for steric selection. However, the fenestrae limit the free access to the parenchymal cell by a factor of 10 [5]. To overcome the difficulty of bringing fresh fluids, solutes and (lipid) particles into the space of Disse and to refresh the fluids, solutes and particles in contact with the parenchymal cells, the mechanisms of "forced sieving" and "endothelial massage" might be important as postulated by Wisse et al. [4]. The hypothesis of "forced sieving" is based on the consideration that red blood cells unilaterally restrict the space in which lipoproteins move in Brownian motion. Red blood cells therefore increase the chance that lipoprotein droplets will escape through the fenestrae. Taking into account that red blood cells pass by in endless numbers, while gently touching the fenestrated lining and in the meantime constantly adapting their shape to the dimensions of the sinusoid, it is assumed that red blood cells in their turn exert an important effect on the passage of any molecule larger than water through the liver sieve.
According to the hypothesis of endothelial massage, white blood cells plug the sinusoid because they have an average size of 8.5 μm and therefore do not fit into a sinusoid, which measures from 5.9 μm in the portal region to 7.1 μm in the centrilobular region. In addition, white blood cells are less plastic than other blood cells and do not easily adapt to obstacles or diameter changes of sinusoids. As a result, white blood cells impress the fenestrated endothelium and the space of Disse. As a consequence, fluid in the space of Disse is pushed down stream and when fenestrae are encountered, fluid will be flushed out of Disse's space. After passage of the white blood cells, the space of Disse resumes its original shape, which causes a suction of fresh fluids into the space. In this way, "endothelial massage" will be more pronounced in the periportal areas.
Role of fenestrae in fibrosis and cirrhosis
Cirrhosis is defined as a diffuse process characterized by fibrosis and the conversion of normal liver architecture into structurally abnormal nodules. Hepatic fibrosis occurs in the evolution of most chronic liver diseases as a precursor of cirrhosis. Cirrhosis finally results in hepatic failure [113]. Besides alcohol-induced cirrhosis in human [72,73,114], many animal models of fibrosis and / or cirrhosis exist and were described after chronic di(m)ethylnitrosamine- [39,57-62], endotoxin- [34,62], carbon tetrachloride- [37,115], porcine serum- [116] and thioacetamide- [36] exposure. Although multiple causes and morphologies of hepatic cirrhosis exist, all forms of cirrhosis are characterized by a defenestrated sinusoidal endothelium and the presence of a subendothelial basement membrane [36,57,114-116]. In addition, it has been demonstrated that the disappearance of the normal filtration barrier in cirrhotic livers results in an impaired bidirectional exchange between the sinusoidal blood and the parenchymal cells [32,39,115]. As a consequence, defenestration and capillarization of the sinusoidal endothelium may therefore be a major contributor to hepatic failure in cirrhosis.
Data obtained from animal models used to investigate the effects of cirrhotic agents indicate that defenestration precedes hepatic fibrosis [39,60,61]. In addition, before the establishment of fibrosis, defenestration seems to be reversible on removal of the hepatotoxin [61,72]. On the other hand, it has been postulated that defenestration becomes irreversible after the formation of an endothelial basement membrane [32]. From these reports it is clear that defenestration is an early event in the pathogenesis of cirrhosis, preceding the initiation of fibrosis. Defenestration and capillarization are believed to be important in the initiation of perisinusoidal fibrosis by altering retinol metabolism [60]. Rogers et al. [39] demonstrated that these structural alterations block the hepatic uptake of dietary retinol within the chylomicron remnants. This dietary retinol, after processing by the parenchymal cells, is stored in the lipid droplets of fat-storing cells. It is known that retinol deficiency transforms fat-storing cells into myofibroblasts with enhanced extracellular matrix production, resulting in perisinusoidal fibrosis and ultimately in cirrhosis.
Role of fenestrae in cancer
Primary liver cancer and invasion of the liver by metastatic tumor cells have been reported to affect the normal fenestration pattern in both animals [38,92,96] and humans [94,95,97,117]. A significant decrease in the number of fenestrae was reported in hepatic sinusoids colonized by B16F10 melanoma cells [38,92], Lewis lung carcinoma cells [38,92] and colon 38 adenocarcinoma cells [96]. Defenestration was also observed in hepatocellular carcinoma [94,95,97]. At the moment it is still unclear why the sinusoidal endothelium reacts by reducing its porosity, or which (common) factor is involved in the process of tumor-induced defenestration. However, Nagura et al. [96] found evidence that the defenestration caused by metastatic tumor cells was induced by the local microenvironment of the liver or by direct cellular contact of tumor cells with the sinusoidal endothelium. Defenestration could only be observed when tumor cells were injected via the mesenteric vein and not when implanted subcutaneously. The role of local factors in the tumor-induced defenestration was also suggested by others [38,92].
The effects of defenestration on the progression of the tumor is still unclear. In general, it is obvious that a decreased permeability of the sinusoidal endothelium creates a situation that will cause the loss of normal liver functions [94,95]. For example, defenestration may block retinol metabolism in fat-storing cells and therefore promote the development of some tumors. Retinol is known to be important in the differentiation of cells, and low levels are correlated with the development of certain cancers [32,118,119].
Concluding remarks
Despite the multidisciplinary approach taken to study the structure, origin, dynamics and formation of fenestrae, there are still important gaps left open. Our knowledge needs considerable consolidation and expansion on the biochemical level to understand how the different proteins form a contractile unit, and which signal transduction pathways are involved in the fenestral dilatation and relaxation. For the near future, we expect that detailed investigation of the LSEC-cytoskeleton, with cytoskeleton-altering drugs; and further biochemical investigation of the contraction and dilatation machinery of LSEC-fenestrae, with probes that activate or inhibit a certain signal transduction pathway, will contribute to a better understanding of how LSEC control their porosity.
Since the first description of endothelial fenestrae the important function served by LSEC as a selective sieve between the sinusoidal blood and the parenchymal cells has been explored. Today, it is clear that alteration of the endothelial filter affects the bidirectional macromolecular exchange, and therefore may determine the balance between health and disease. Although the majority of the research has been descriptive, the role of the liver sieve has been demonstrated in various diseases such as hyperlipoproteinemia, cirrhosis and cancer. Recently, peculiar reports appeared exploring the role of fenestrae in various conditions, such as liposomal transport [120], hydrodynamics [121], regenerating liver [122], the role of VEGF as an upregulator of the porosity [123], nitric oxide synthesis [124], when people get older [125], and in health and disease in general [126].
We expect that future research will focus on therapeutic strategies by altering the sieve's porosity. For example, discovery of new drugs that increase the porosity of the liver sieve may be of great benefit. Such agents may not only improve the extraction of atherogenic lipoproteins from the circulation, they can also be used for enhancing the efficiency of liposome-mediated gene or drug delivery to parenchymal cells.
Acknowledgements
We thank Ronald De Zanger, Katrien Vekemans, Maarten Timmers, David Vermijlen, Chris Derom, Marijke Baekeland, Danielle Blijweert, Carine Seynaeve and Andrea De Smedt from our laboratory for expert assistance. This work would have been impossible without the collaboration with other departments; Special thanks to Bård Smedsrød (Tromsø, Norway), Ilan Spector (New York, USA), Wouter Kalle (Waga Waga, Australia) and Manfred Radmacher (Göttingen, Germany). Filip Braet is a postdoctoral researcher of the "Fund for Scientific Research-Flanders".
References
- Wisse E. An electron microscopic study of the fenestrated endothelial lining of rat liver sinusoids. J Ultrastruct Res. 1970;31:125–150. [Abstract] [Google Scholar]
- Widmann JJ, Cotran RS, Fahimi HD. Mononuclear phagocytes (Kupffer cells) and endothelial cells in regenerating rat liver. J Cell Biol. 1972;52:159–170. [Europe PMC free article] [Abstract] [Google Scholar]
- Ogawa K, Minase T, Enomoto K, Onoé T. Ultrastructure of fenestrated cells in the sinusoidal wall of rat liver after perfusion fixation. Tohoku J Exp Med. 1973;110:89–101. [Abstract] [Google Scholar]
- Wisse E, De Zanger RB, Charels K, Van Der Smissen P, McCuskey RS. The liver sieve: Considerations concerning the structure and function of endothelial fenestrae, the sinusoidal wall and the space of Disse. Hepatology. 1985;5:683–692. [Abstract] [Google Scholar]
- Wisse E, De Zanger RB, Jacobs R, McCuskey RS. Scanning electron microscope observations on the structure of portal veins, sinusoids and central veins in rat liver. Scan Electron Microsc. 1983;3:1441–1452. [Abstract] [Google Scholar]
- Wisse E. An ultrastructural characterization of the endothelial cell in the rat liver sinusoid under normal and various experimental conditions, as a contribution to the distinction between endothelial and Kupffer cells. J Ultrastruct Res. 1972;38:528–562. [Abstract] [Google Scholar]
- De Zanger R, Wisse E. The filtration effect of rat liver fenestrated sinusoidal endothelium on the passage of (remnant) chylomicrons to the space of Disse. In: Knook DL, Wisse E, editor. Sinusoidal Liver Cells. Amsterdam, Elsevier; 1982. pp. 69–76. [Google Scholar]
- Fraser R, Bosanquet AG, Day WA. Filtration of chylomicrons by the liver may influence cholesterol metabolism and atherosclerosis. Atherosclerosis. 1978;29:113–123. [Abstract] [Google Scholar]
- Wisse E, De Zanger R, Jacobs R. Lobular gradients in endothelial fenestrae and sinusoidal diameter favour centrolobular exchange processes: a scanning EM study. In: Knook DL, Wisse E, editor. Sinusoidal Liver Cells. Amsterdam, Elsevier; 1982. pp. 61–67. [Google Scholar]
- Smedsrød B, De Bleser PJ, Braet F, Lovisetti P, Vanderkerken K, Wisse E, Geerts A. Cell biology of liver endothelial and Kupffer cells. Gut. 1994;35:1509–1516. [Europe PMC free article] [Abstract] [Google Scholar]
- De Leeuw AM, Brouwer A, Knook DL. Sinusoidal endothelial cells of the liver: fine structure and function in relation to age. J Electron Microsc Tech. 1990;14:218–236. [Abstract] [Google Scholar]
- Irie S, Tavassoli M. Transendothelial transport of macromolecules: the concept of tissue-blood barriers. Cell Biol Rev. 1991;25:317–331. [Abstract] [Google Scholar]
- Simionescu N, Simionescu M. The cardiovascular system. In: Weiss L, editor. Cell and tissue biology. Munich, Urbam & Schwarzenberg GmbH; 1988. pp. 355–400. [Google Scholar]
- Naito M, Wisse E. Observations on the fine structure and cytochemistry of sinusoidal cells in fetal and neonatal rat liver. In: Wisse E, Knook DL, editor. Kupffer cells and Other Liver Sinusoidal Cells. Amsterdam, Elsevier; 1977. pp. 497–505. [Google Scholar]
- Bankston PW, Pino RM. The development of the sinusoids of fetal rat liver: morphology of endothelial cells, Kupffer cells, and the transmural migration of blood cells into the sinusoids. Am J Anat. 1980;159:1–15. [Abstract] [Google Scholar]
- Barberá-Guillem E, Arrue JM, Ballesteros J, Vidal-Vanaclocha F. Structural changes in endothelial cells of developing rat liver in the transition from fetal to postnatal life. J Ultrastruct Mol Struct Res. 1986;97:197–206. [Abstract] [Google Scholar]
- Barberá-Guillem E, Vidal-Vanaclocha F. Sinusoidal structure of the liver. In: Barberá-Guillem E, editor. Liver sinusoids II. Bilbao, Cell Biology Reviews; 1988. pp. 1–68. [Abstract] [Google Scholar]
- Macchiarelli G, Makabe S, Motta PM. Scanning electron microscopy of adult and fetal liver sinusoids. In: Bioulac-Sage P, Balabaud C, editor. Sinusoids in human liver: Health and disease. Rijswijk, Kupffer Cell Foundation; 1988. pp. 63–85. [Google Scholar]
- Naito M, Wisse E. Filtration effect of endothelial fenestrations on chylomicron transport in neonatal rat liver sinusoids. Cell Tiss Res. 1978;190:371–382. [Abstract] [Google Scholar]
- Mak KM, Lieber CS. Alterations in endothelial fenestrations in liver sinusoids of baboons fed alcohol: a scanning electronic microscopic study. Hepatology. 1984;4:386–391. [Abstract] [Google Scholar]
- McCuskey PA, McCuskey RS, Hinton DE. Electron microscopy of the hepatic sinusoids in rainbow trout. In: Kirn A, Knook DL, Wisse E, editor. Cells of the Hepatic Sinusoid 1. Rijswijk, Kupffer Cell Foundation; 1986. pp. 489–494. [Google Scholar]
- Clark SA, Angus HB, Cook HB, Oxner RBG, George PM, Fraser R. Defenestration of hepatic sinusoids as a cause of hyperlipoproteinaemia in alcoholics. Lancet. 1988;2:1225–1227. 10.1016/S0140-6736(88)90813-6. [Abstract] [CrossRef] [Google Scholar]
- Fraser R, Heslop VR, Murray FE, Day WA. Ultrastructural studies of the portal transport of fat in chickens. Br J Exp Pathol. 1986;67:783–791. [Europe PMC free article] [Abstract] [Google Scholar]
- Wright PL, Smith KF, Day WA, Fraser R. Small liver fenestrae may explain the susceptibility of rabbits to atherosclerosis. Arteriosclerosis. 1983;3:344–348. [Abstract] [Google Scholar]
- Fraser R, Clark SA, Day WA, Murray FEM. Nicotine decreases the porosity of the rat liver sieve: a possible mechanism for hypercholesterolaemia. Br J Exp Pathol. 1988;69:345–350. [Europe PMC free article] [Abstract] [Google Scholar]
- Steffan AM, Gendrault JL, Kirn A. Increase in the number of fenestrae in mouse endothelial liver cells by altering the cytoskeleton with cytochalasin B. Hepatology. 1987;7:1230–1238. [Abstract] [Google Scholar]
- Fraser R, Day WA, Fernando NS. Atherosclerosis and the liver sieve. In: Kirn A, Knook DL, Wisse E, editor. Cells of the Hepatic Sinusoid 1. Rijswijk, Kupffer Cell Foundation; 1986. pp. 317–322. [Google Scholar]
- Horn T, Christoffersen P, Henriksen JH. Alcoholic liver injury: defenestration in noncirrhotic livers – a scanning electron microscopic study. Hepatology. 1987;7:77–82. [Abstract] [Google Scholar]
- Wright PL, Smith KF, Day WA, Fraser R. Hepatic sinusoidal endothelium in sheep: an ultrastructural reinvestigation. Anat Rec. 1983;206:385–390. [Abstract] [Google Scholar]
- Wright PL, Clement JA, Smith KF, Day WA, Fraser R. Hepatic sinusoidal endothelium in goats. Aust J Exp Biol Med Sci. 1983;61:739–741. [Abstract] [Google Scholar]
- Tanuma Y, Ohata M, Ito T. An electron microscopic study of the kitten liver with special reference to fat-storing cells. Arch Histol Jpn. 1981;46:401–426. [Abstract] [Google Scholar]
- Fraser R, Dobbs BR, Rogers GWT. Lipoproteins and the liver sieve: the role of the fenestrated sinusoidal endothelium in lipoprotein metabolism, atherosclerosis, and cirrhosis. Hepatology. 1995;21:863–874. [Abstract] [Google Scholar]
- Frenzel H, Kremer B, Hucker H. The liver sinusoids under various pathological conditions. A TEM and SEM study of rat liver after respiratory hypoxia, telecobalt-irradiation and endotoxin application. In: Wisse E, Knook DL, editor. Kupffer and other liver sinusoidal cells. Amsterdam, Elsevier; 1977. pp. 213–222. [Google Scholar]
- Dobbs BR, Rogers GWT, Xing HY, Fraser R. Endotoxin-induced defenestration of the hepatic sinusoidal endothelium: a factor in the pathogenesis of cirrhosis? Liver. 1994;14:230–233. [Abstract] [Google Scholar]
- Steffan AM, Pereira CA, Bingen A, Valle M, Martin JP, Koehren F, Royer C, Gendrault JL, Kirn A. Mouse hepatitis virus type 3 infection provokes a decrease in the number of sinusoidal endothelial fenestrae both in vivo and in vitro. Hepatology. 1995;22:395–401. [Europe PMC free article] [Abstract] [Google Scholar]
- Mori T, Okanoue T, Sawa Y, Hori N, Ohta M, Kagawa K. Defenestration of the sinusoidal endothelial cell in a rat model of cirrhosis. Hepatology. 1993;17:891–897. [Abstract] [Google Scholar]
- Fraser R, Bowler LM, Wisse E. Agents related to fibrosis, such as alcohol and carbon tetrachloride, acutely effect endothelial fenestrae which cause fatty liver. In: Gerlach V, Pott J, Rauterberg J, Vob B, editor. Connective Tissue of the Normal and Fibrotic Human Liver. Stuttgart, Thierne G verlag; 1982. pp. 159–160. [Google Scholar]
- Vidal-Vanaclocha F, Alonso-Varona A, Ayala R, Barberá-Guillem E. Functional variations in liver tissue during the implantation process of metastatic tumour cells. Virchows Archiv A Pathol Anat Histopathol. 1990;416:189–195. [Abstract] [Google Scholar]
- Rogers GWT, Dobbs BR, Fraser R. Decreased hepatic uptake of cholesterol and retinol in the dimethylnitrosamine rat model of cirrhosis. Liver. 1992;12:326–329. [Abstract] [Google Scholar]
- Oda M, Kazemoto S, Kaneko H, Yokomori H, Ishii K, Tsukada N, Watanabe N, Suematsu M, Tsuchiya M. Involvement of Ca++-calmodulin-actomyosin system in the contractility of hepatic sinusoidal endothelial fenestrae. In: Knook DL, Wisse E, editor. Cells of the Hepatic Sinusoid 4. Leiden, Kupffer Cell Foundation; 1993. pp. 174–178. [Google Scholar]
- Nopanitaya W, Lamb JC, Grisham JW, Carson JL. Effect of hepatic venous outflow obstruction on pores and fenestration in sinusoidal endothelium. Br J Exp Pathol. 1976;57:604–609. [Europe PMC free article] [Abstract] [Google Scholar]
- Braet F, De Zanger R, Kalle WHJ, Raap AK, Tanke HJ, Wisse E. Comparative scanning, transmission and atomic force microscopy of the microtubular cytoskeleton in fenestrated endothelial cells. Scanning Microscopy. 1996;10:225–236. [Abstract] [Google Scholar]
- Oda M, Nakamura M, Watanabe N, Ohya Y, Sekuzuka E, Tsukada N, Yonei Y, Komatsu H, Nagata H, Tsuchiya M. Some dynamic aspects of the hepatic microcirculation – demonstration of sinusoidal endothelial fenestrae as a possible regulatory factor-. In: Tsuchiya M, Wayland H, Oda M, Okazaki I, editor. Intravital Observation of Organ Microcirculation. Amsterdam, Excerpta Medica; 1983. pp. 105–138. [Google Scholar]
- Tsukada N, Oda M, Yonei Y, Honda K, Akaiwa Y, Kiryu Y, Tsuchiya M. Alterations of the hepatic sinusoidal endothelial fenestrae in response to vasoactive substances in the rat liver -in vivo and in vitro studies-. In: Kirn A, Knook DL, Wisse E, editor. Cells of the Hepatic Sinusoid 1. Rijswijk, Kupffer Cell Foundation; 1986. pp. 515–516. [Google Scholar]
- Oda M, Azuma T, Watamabe N, Nishizaku Y, Nishida Y, Ischii K, Suzuki H, Kaneko H, Komatsu H, Tsukada N, Tsuchiya M. Regulatory mechanism of hepatic microcirculation: Involvement of the contraction and dilatation of sinusoids and sinusoidal endothelial fenestrae. In: Messemer K, Hammersen F, editor. Gastrointestinal Microcirculation – Prog Appl Microcirc. Basel, Karger; 1990. pp. 103–128. [Google Scholar]
- Oda M, Tsukada N, Watanabe N, Tsuchiya M. Heterogeneity of hepatic lobule – some ultrastructural aspects of hepatic microcirculation system -. J Clin Electron Microscopy. 1983;16:5–6. [Google Scholar]
- McCuskey RS, Eguchi H, Nishida J, Krasovich MA, McDonell D, Jolley CS, Abril ER, Earnest DL, Watson RR. Effects of ethanol and cocaine alone or in combination on the hepatic sinsusoids of mice and rats. In: Knook DL, Wisse E, editor. Cells of the Hepatic Sinusoid 4. Leiden, Kupffer Cell Foundation; 1993. pp. 376–380. [Google Scholar]
- McGuire RF, Bissell DM, Boyles J, Roll FJ. Role of extracellular matrix in regulating fenestrations of sinusoidal endothelial cells isolated from normal rat liver. Hepatology. 1992;15:989–997. [Abstract] [Google Scholar]
- Steffan AM, Gendrault JL, Kirn A. Phagocytosis and surface modulation of fenestrated areas – two properties of murine endothelial liver cell (EC) involving microfilaments. In: Kirn A, Knook DL, Wisse E, editor. Cells of the Hepatic Sinusoid 1. Rijswijk, Kupffer Cell Foundation; 1986. pp. 483–488. [Google Scholar]
- Tsukada N, Oda M, Yonei Y, Komatsu H, Kaneko K, Honda K, Akaiwa Y, Mizuno Y, Fujiwara T, Tsuchiya M. Ultrastructural alterations in the hepatic sinusoidal endothelial fenestrae by dysfunction of actin filaments. In: Imura T, Maruse S, Suzuki T, editor. Electron Microscopy 1986 – 3. Tokyo, Japanese Society of Electron Microscopy; 1986. pp. 2915–1916. [Google Scholar]
- Van Der Smissen P, Van Bossuyt H, Charels K, Wisse E. The structure and function of the cytoskeleton in sinusoidal endothelial cells in the rat liver. In: Kirn A, Knook DL, Wisse E, editor. Cells of the Hepatic Sinusoid 1. Rijswijk, Kupffer Cell Foundation; 1986. pp. 517–522. [Google Scholar]
- Bingen A, Gendrault JL, Kirn A. Cryofracture study of fenestrae formation in mouse liver endothelial cells treated with cytochalasin B. In: Wisse E, Knook DL, Decker K, editor. Cells of the Hepatic Sinusoid 2. Leiden, Kupffer Cell Foundation; 1989. pp. 466–470. [Google Scholar]
- Braet F, De Zanger R, Baekeland M, Crabbé E, Van Der Smissen P, Wisse E. Structure and dynamics of the fenestrae-associated cytoskeleton of rat liver sinusoidal endothelial cells. Hepatology. 1995;21:180–189. [Abstract] [Google Scholar]
- Braet F, Kalle WHJ, De Zanger RB, de Grooth BG, Raap AK, Tanke HJ, Wisse E. Comparative atomic force and scanning electron microscopy; an investigation on fenestrated endothelial cells in vitro. J Microsc. 1996;181:10–17. [Abstract] [Google Scholar]
- Braet F, De Zanger R, Jans D, Spector I, Wisse E. Microfilament-disrupting agent latrunculin A induces an increased number of fenestrae in rat liver sinusoidal endothelial cells: comparison with cytochalasin B. Hepatology. 1996;24:627–635. [Abstract] [Google Scholar]
- Kalle WHJ, Braet F, Raap AK, de Grooth BG, Tanke H, Wisse E. Imaging of the membrane surface of sinusoidal rat liver endothelial cells by atomic force microscopy. In: Wisse E, Knook DL, Balabaud C, editor. Cells of the Hepatic Sinusoid 6. Leiden, Kupffer Cell Foundation; 1997. pp. 94–96. [Google Scholar]
- Ogawa H. Scanning electron microscopy of rat liver hyperplastic nodules induced by diethylnitrosamine. Scanning Microscopy. 1982;4:1793–1798. [Abstract] [Google Scholar]
- Ogawa H, Itoshima T, Ito T, Kiyotoshi S, Kawaguchi K, Kitadai M, Hattori S, Mizutani S, Ukida M, Tobe K, Nagashima H, Kobayashi T. Absence of Kupffer cells in carcinogen induced liver hyperplastic nodules: demonstration by intravenous injection of indian ink. Acta Med Okayama. 1983;37:79–84. [Abstract] [Google Scholar]
- Ogawa H, Itoshima T, Ukida M, Ito T, Kiyotoshi S, Kitadai M, Hattori S, Mizutani S, Kita K, Tanaka R. Scanning electron microscopy of experimentally induced sequential alterations of rat liver hyperplastic nodules. Scanning Microscopy. 1984;1:369–374. [Abstract] [Google Scholar]
- Fraser R, Rogers GWT, Bowler LM, Day WA, Dobbs BR, Baxter JN. Defenestration and vitamin A status in a rat model of cirrhosis. In: Wisse E, Knook DL, McCuskey RS, editor. Cells of the Hepatic Sinusoid 3. Leiden, Kupffer Cell Foundation; 1991. pp. 195–198. [Google Scholar]
- Tamba-Lebbie B, Rogers GWT, Dobbs BR, Fraser R. Defenestration of the hepatic sinusoidal endothelium in the dimethylnitrosamine fed rat: is this process reversible? In: Knook DL, Wisse E, editor. Cells of the Hepatic Sinusoid 4. Leiden, Kupffer Cell Foundation; 1993. pp. 179–181. [Google Scholar]
- Fraser R, Rogers GWT, Sutton LE, Dobbs BR. Single dose models of defenestration: tools to explore mechanisms, modulation and measurement of hepatic sinusoidal porosity. In: Wisse E, Knook DL, Wake K, editor. Cells of the Hepatic Sinusoid 5. Leiden, Kupffer Cell Foundation; 1995. pp. 263–266. [Google Scholar]
- Oda M, Kamegaya Y, Yokomori H, Han JY, Akiba Y, Nakamura M, Ishii H, Tsuchiya M. Roles of plasma membrane Ca++ – ATPase in the relaxation and contraction of hepatic sinusoidal endothelial fenestrae – effects of prostaglandin E1 and endothelin 1. In: Wisse E, Knook DL, Balabaud C, editor. Cells of the Hepatic Sinusoid 6. Leiden, Kupffer Cell Foundation; 1997. pp. 313–317. [Google Scholar]
- Barshtein IA, Persidskii IV, Grutman MI. Ultrastructural changes in the histohematic barrier of the liver in the early period after endotoxin administration. Biull Eksp Biol Med. 1989;108:365–369. [Abstract] [Google Scholar]
- Sasaoki T, Braet F, De Zanger R, Wisse E, Arii S. The effect of endotoxin on liver sinusoidal endothelial cells. In: Wisse E, Knook DL, Wake K, editor. Cells of the Hepatic Sinusoid 5. Leiden, Kupffer Cell Foundation; 1995. pp. 366–367. [Google Scholar]
- Sarphie TG, D'Souza NB, Deaciuc IV. Kupffer cell inactivation prevents lipopolysaccharide-induced structural changes in the rat liver sinusoid: an electron-microscopic study. Hepatology. 1996;23:788–796. [Abstract] [Google Scholar]
- Charels K, De Zanger R, Van Bossuyt H, Van Der Smissen P, Wisse E. Influence of acute alcohol administration on endothelial fenestrae of rat livers: An in vivo and in vitro scanning electron microscopic study. In: Kirn A, Knook DL, Wisse E, editor. Cells of the Hepatic Sinusoid 1. Rijswijk, Kupffer Cell Foundation; 1986. pp. 497–502. [Google Scholar]
- Mori T, Okanoue T, Sawa Y, Itoh Y, Kanaoka H, Hori N, Enjyo F, Nakagawa Y, Kagawa K, Kashima K. Effect of ethanol on the sinusoidal endothelial fenestrations of rat liver – in vivo an in vitro study. In: Wisse E, Knook DL, McCuskey RS, editor. Cells of the Hepatic Sinusoid 3. Leiden, Kupffer Cell Foundation; 1991. pp. 469–471. [Google Scholar]
- Braet F, De Zanger R, Sasaoki T, Baekeland M, Janssens P, Smedsrød B, Wisse E. Assessment of a method of isolation, purification and cultivation of rat liver sinusoidal endothelial cells. Lab Invest. 1994;70:944–952. [Abstract] [Google Scholar]
- Fraser R, Bowler LM, Day WA. Damage of rat liver sinusoidal endothelium by ethanol. Pathology. 1980;12:371–376. [Abstract] [Google Scholar]
- Kanaoka H, Okanoue T, Ohta M, Kachi K, Sawa Y, Ou O, Kagawa K, Yuki T, Okuno T, Takino T. Scanning electron microscopy of endothelial fenestration in liver sinusoids of rats fed ethanol. In: Imura T, Maruse S, Suzuki T, editor. Electron Microscopy 1986 – 3. Tokyo, Japanese Society of Electron Microscopy; 1986. pp. 2919–1920. [Google Scholar]
- Madarame T, Nagaoka T, Inomata M, Kaneta H, Yoshida T, Suzuki K, Sato S, Kanno S, Satodate R. Relationship between endothelial structure and capillarization in fatty liver. In: Knook DL, Wisse E, editor. Cells of the Hepatic Sinusoid 4. Leiden, Kupffer Cell Foundation; 1993. pp. 191–192. [Google Scholar]
- Madarame T, Suzuki K, Sato S, Sakuma T, Satodate R. Relationship between morphological changes in endothelial cells and the number of Kupffer cells in human fatty liver. In: Wisse E, Knook DL, Wake K, editor. Cells of the Hepatic Sinusoid 5. Leiden, Kupffer Cell Foundation; 1995. pp. 272–274. [Google Scholar]
- Morsiani E, Mazzoni M, Aleotti A, Gorini P, Ricci D. Increased sinusoidal wall permeability and liver fatty change after two-thirds hepatectomy: an ultrastructural study in the rat. Hepatology. 1995;21:539–544. [Abstract] [Google Scholar]
- Steffan AM, Pereira CA, Kirn A. Role of sinusoidal cells in the course of the hepatitis induced by mouse hepatitis virus type 3 (MHV 3) in mice. In: Kirn A, Knook DL, Wisse E, editor. Cells of the Hepatic Sinusoid 1. Rijswijk, Kupffer Cell Foundation; 1986. pp. 375–376. [Google Scholar]
- Braet F, De Zanger R, Spector I, Wisse E. Fenestrae-forming center (FFC): A novel structure involved in the formation of liver sinusoidal endothelial cell fenestrae. In: Wisse E, Knook DL, De Zanger R, Fraser R, editor. Cells of the Hepatic Sinusoid 7. Leiden, Kupffer Cell Foundation; 1999. pp. 144–146. [Google Scholar]
- Shakado S, Sakisaka S, Noguchi K, Yoshitake M, Harada M, Mimura Y, Sata M, Tanikawa K. Effects of extracellular matrices on tube formation of cultured rat hepatic sinusoidal endothelial cells. Hepatology. 1995;22:969–973. [Abstract] [Google Scholar]
- Braet F, De Zanger R, Spector I, Wisse E. The actin disrupting marine toxin latrunculin A induces an increased number of fenestrae in rat liver sinusoidal endothelial cells. In: Wisse E, Knook DL, Balabaud C, editor. Cells of the Hepatic Sinusoid 6. Leiden, Kupffer Cell Foundation; 1997. pp. 82–84. [Google Scholar]
- Braet F, Spector I, De Zanger R, Wisse E. A novel structure involved in the formation of liver endothelial cell fenestrae revealed by using the actin inhibitor misakinolide. Proc Natl Acad Sci USA. 1998;95:13635–13640. 10.1073/pnas.95.23.13635. [Europe PMC free article] [Abstract] [CrossRef] [Google Scholar]
- Wisse E, Van Dierendonck JH, De Zanger RB, Fraser R, McCuskey RS. On the role of the liver endothelial filter in the transport of particulate fat (chylomicrons and their remnants) to parenchymal cells and the influence of certain hormones on the endothelial fenestrae. In: Popper H, Gudat F, Bianchi L, Reutter W, editor. Communications of Liver Cells. Basel, MTP Press; 1980. pp. 195–200. [Google Scholar]
- Fraser R, Clark SA, Bowler LM, Day WA. Pantethine inhibits diet-induced hypercholesterolaemia by dilating the liver sieve. N Z Med J. 1988;101:86–87. [Google Scholar]
- Fraser R, Clark SA, Bowler LM, Murray FEM, Wakasugi J, Ishihara M, Tomikawa M. The opposite effects of nicotine and pantethine on the porosity of the liver sieve and lipoprotein metabolism. In: Wisse E, Knook DL, Decker K, editor. Cells of the Hepatic Sinusoid 2. Leiden, Kupffer Cell Foundation; 1989. pp. 335–338. [Google Scholar]
- Arias IM. The biology of hepatic endothelial cell fenestrae. In: Schaffer F, Popper H, editor. Progress in Liver Diseases IX. Philadelphia, WB Saunders; 1990. pp. 11–26. [Abstract] [Google Scholar]
- De Zanger R, Braet F, Arnez Camacho MR, Wisse E. Prolongation of hepatic endothelial cell cultures by phorbol myristate acetate. In: Wisse E, Knook DL, Balabaud C, editor. Cells of the Hepatic Sinusoid 6. Leiden, Kupffer Cell Foundation; 1997. pp. 97–101. [Google Scholar]
- Fraser R, Bowler LM, Day WA, Dobbs B, Johnson HD, Lee D. High perfusion pressure damages the sieving ability of sinusoidal endothelium in rat livers. Br J Exp Pathol. 1980;61:222–228. [Europe PMC free article] [Abstract] [Google Scholar]
- Yokomori H, Oda M, Kamegaya Y, Ogi M, Yokono H, Han JY, Akiba Y, Nakamura M, Tsukada N, Ishii H. Functional significance of plasma membrane Ca++-ATPase of hepatic sinusoidal endothelium in the control of sinusoidal blood flow – dynamic, electron cytochemical and microfluometric analysis. Hepatology. 1996;24:130. [Google Scholar]
- Rice J, Gatmaitan Z, Mikkelson R, Arias IM. On the structure and function of the fenestrae of hepatic endothelial cells. Hepatology. 1985;5:1049. [Google Scholar]
- Brauneis U, Gatmaitan Z, Arias IM. Serotonin stimulates a Ca2+ permeant nonspecific cation channel in hepatic endothelial cells. Biochem Biophys Res Commun. 1992;186:1560–1566. [Abstract] [Google Scholar]
- Gatmaitan Z, Arias IM. Hepatic endothelial cell fenestrae. In: Knook DL, Wisse E, editor. Cells of the Hepatic Sinusoid 4. Leiden, Kupffer Cell Foundation; 1993. pp. 3–7. [Google Scholar]
- Gatmaitan Z, Varticovski L, Ling L, Mikkelsen R, Steffan AM, Arias IM. Studies on fenestral contraction in rat liver endothelial cells in culture. Am J Pathol. 1996;148:2027–2041. [Europe PMC free article] [Abstract] [Google Scholar]
- Mori T, Okanoue T, Sawa Y, Hori N, Kanaoka H, Itoh Y, Enjyo F, Ohta M, Kagawa K, Kashima K. The change of sinusoidal endothelial cells in experimental liver cirrhosis – in vivo and in vitro study-. In: Knook DL, Wisse E, editor. Cells of the Hepatic Sinusoid 4. Leiden, Kupffer Cell Foundation; 1993. pp. 280–282. [Google Scholar]
- Barberá-Guillem E, Vidal-Vanaclocha F. Concomitant variation of the fenestration pattern in endothelial cells of livers sinusoids colonized by malignant tumor cells. In: Kirn A, Knook DL, Wisse E, editor. Cells of the Hepatic Sinusoid 1. Rijswijk, Kupffer Cell Foundation; 1986. pp. 495–496. [Google Scholar]
- Madarame T, Masuda T, Suzuki A, Satodate R, Suzuki K, Sato S. Ultrastructural features of the vascular channel of human and experimentally induced hepatocellular carcinoma. In: Kirn A, Knook DL, Wisse E, editor. Cells of the Hepatic Sinusoid 1. Rijswijk, Kupffer Cell Foundation; 1986. pp. 399–404. [Google Scholar]
- Tabarin A, Bioulic-Sage P, Boussarie L, Balabaud C, de Mascarel A, Grimaud JA. Hepatocellular carcinoma developed on noncirrhotic livers. Sinusoids in hepatocellular carcinoma. Arch Pathol Lab Med. 1987;111:174–180. [Abstract] [Google Scholar]
- Ichida T, Hata K, Yamada S, Hatano T, Miyagiwa M, Miyabayashi C, Matsui S, Wisse E. Subcellular abnormalities of liver sinusoidal lesions in human hepatocellular carcinoma. J Submicrosc Cyto Pathol. 1990;22:221–229. [Abstract] [Google Scholar]
- Nagura T, Shiratori Y, Fukushi Y, Kiriyama H, Hai K, Takada H, Matsumoto K, Kamii K, Migita T. Morphological changes of hepatic sinusoidal cells during metastasis of colon carcinoma. In: Knook DL, Wisse E, editor. Cells of the Hepatic Sinusoid 4. Leiden, Kupffer Cell Foundation; 1993. pp. 530–533. [Google Scholar]
- Torimura T, Ueno T, Inuzuka S, Ohira H, Sakamoto M, Sata M, Tanikawa K. Sinusoids in hepatocellular carcinoma – immunohistochemical and ultrastructural observation. In: Knook DL, Wisse E, editor. Cells of the Hepatic Sinusoid 4. Leiden, Kupffer Cell Foundation; 1993. pp. 541–543. [Google Scholar]
- Persidsky YU, Frolov A, Barshtein YU. Tumor necrosis factor action on the various types of mouse liver cell in vivo. In: Knook DL, Wisse E, editor. Cells of the Hepatic Sinusoid 4. Leiden, Kupffer Cell Foundation; 1993. pp. 82–84. [Google Scholar]
- Oda M, Tsukada N, Watanabe N, Komatso H, Yonei Y, Tsuchiya M. Functional implications of the sinusoidal endothelial fenestrae in the regulation of the hepatic microcirculation. Hepatology. 1984;4:754. [Google Scholar]
- Arias IM, Peleg Y, Gatmaitan Z, Adelstein RA. The mechanism whereby serotonin produces fenestral contraction in rat hepatic endothelial cells. Hepatology. 1987;7:1026. [Google Scholar]
- Oda M, Tsukada N, Komatsu H, Yonei Y, Honda K, Tsuchiya M. Mechanism of contraction and dilatation of sinusoidal endothelial fenestrae in the liver. Hepatology. 1986;6:771. [Google Scholar]
- Oda M, Tsukada N, Komatsu H, Kaneko K, Nakamura M, Tsuchiya M. Electron microscopic localizations of actin, calmodulin and calcium in the hepatic sinusoidal endothelium in the rat. In: Kirn A, Knook DL, Wisse E, editor. Cells of the Hepatic Sinusoid 1. Rijswijk, Kupffer Cell Foundation; 1986. pp. 511–512. [Google Scholar]
- Oda M, Watanabe N, Azuma T, Komatsu H, Kaneko K, Tsukada N, Tsuchiya M. Dynamic evidence for contraction and dilatation of hepatic sinusoidal endothelial fenestrae by AVEC-DIC microscopy. Hepatology. 1988;8:1434. [Google Scholar]
- Gatmaitan Z, Arias IM. From blood to bile and back: New perspectives. In: Messmer K, Menger MD, editor. Progress in Applied Microcirculation 19. Basel, Karger; 1993. pp. 1–14. [Google Scholar]
- Steffan AM, Gendrault JL, McCuskey RS, McCuskey PA, Kirn A. Phagocytosis, an unrecognized property of murine endothelial liver cells. Hepatology. 1986;6:830–836. [Abstract] [Google Scholar]
- Taira K. Trabecular meshworks in the sinusoidal endothelial cells of the golden hamster liver: a freeze-fracture study. J Submicrosc Cytol Pathol. 1994;26:271–277. [Abstract] [Google Scholar]
- Braet F, Spector I, Shochet NR, Crews P, Higa T, Menu E, De Zanger R, Wisse E. The new anti-actin agent dihydrohalichondramide reveals fenestrae-forming centers in hepatic endothelial cells. BMC Cell Biology. 2002;3:7. 10.1186/1471-2121-3-7. [Europe PMC free article] [Abstract] [CrossRef] [Google Scholar]
- Fraser R, Day WA, Fernando NS. The liver sinusoidal cells, their role in disorders of the liver, lipoprotein metabolism and atherogenesis. Pathology. 1986;18:5–11. [Abstract] [Google Scholar]
- Karpe F, Hamsten A. Postprandial lipoprotein metabolism and atherosclerosis. Curr Opin Lipidol. 1995;6:123–129. [Abstract] [Google Scholar]
- Turley SD, Dietschy JM. The metabolism and excretion of cholesterol by the liver. In: Arias IM, Jakoby WB, Popper H, Schachter D, Shafritz DA, editor. The liver: Biology and Pathobiology. Second. New York, Raven Press; 1988. pp. 617–639. [Google Scholar]
- Glaumann H, Bergstrand A, Ericsson JLE. Studies on the synthesis and intracellular transport of lipoprotein particles in rat liver. J Cell Biol. 1975;64:356–377. [Europe PMC free article] [Abstract] [Google Scholar]
- Fraser R, Day WA, Wright PL. Fatty liver and the sinusoidal cells. In: Garlick D, editor. Festschrift for F.C. Courtice. Sydney, University of New South Wales Press; 1981. pp. 139–144. [Google Scholar]
- Anchony PP, Ishak KG, Nayak NC, Poulsen HE, Scheuer PJ, Sobin LH. The morphology of cirrhosis. J Clin Pathol. 1978;31:395–414. [Europe PMC free article] [Abstract] [Google Scholar]
- Ishak KG, Zimmerman HJ, Ray MB. Alcoholic liver disease: pathologic, pathogenetic and clinical aspects. Alcohol Clin Exp Res. 1991;15:45–66. [Abstract] [Google Scholar]
- Martinez-Hernandez A, Martinez J. The role of capillarization in hepatic failure: studies in carbon tetrachloride-induced cirrhosis. Hepatology. 1991;14:864–874. [Abstract] [Google Scholar]
- Bhunchet E, Fujieda K. Capillarization and venularization of hepatic sinusoids in porcine serum-induced rat liver fibrosis: a mechanism to maintain liver blood flow. Hepatology. 1993;18:1450–1458. [Abstract] [Google Scholar]
- Le Bail B, Bioulac-Sage P, Senuita R, Quinton A, Saric J, Balabaud C. Fine structure of hepatic sinusoids and sinusoidal cells in disease. J Electron Microsc Tech. 1990;14:257–282. [Abstract] [Google Scholar]
- De Vet HC. The puzzling role of vitamin A in cancer prevention. Anticancer Res. 1989;9:145–151. [Abstract] [Google Scholar]
- Lupulescu A. The role of vitamins A, beta-carotene, E and C in cancer cell biology. Int J Vitam Nutr Res. 1994;64:3–14. [Abstract] [Google Scholar]
- Romero EL, Morilla MJ, Regts J, Koning GA, Scherphof GL. On the mechanism of hepatic transendothelial passage of large liposomes. FEBS Lett. 1999;448:193–196. 10.1016/S0014-5793(99)00364-6. [Abstract] [CrossRef] [Google Scholar]
- Popescu D, Movileanu L, Ion S, Flonta ML. Hydrodynamic effects on the solute transport across endothelial pores and hepatocyte membranes. Phys Med Biol. 2000;45:N157–N165. 10.1088/0031-9155/45/11/404. [Abstract] [CrossRef] [Google Scholar]
- Wack KE, Ross MA, Zegarra V, Sysko LR, Watkins SC, Stolz DB. Sinusoidal ultrastructure evaluated during the revascularization of regenerating rat liver. Hepatology. 2001;33:363–378. 10.1053/jhep.2001.21998. [Abstract] [CrossRef] [Google Scholar]
- Funyu J, Mochida S, Inao M, Matsui A, Fujiwara K. VEGF can act as vascular permeability factor in the hepatic sinusoids through upregulation of porosity of endothelial cells. Biochem Biophys Res Commun. 2001;280:481–485. 10.1006/bbrc.2000.4148. [Abstract] [CrossRef] [Google Scholar]
- Yokomori H, Oda M, Ogi M, Sakai K, Ishii H. Enhanced expression of endothelial nitric oxide synthase and caveolin-1 in human cirrhosis. Liver. 2002;22:150–158. 10.1034/j.1600-0676.2002.01588.x. [Abstract] [CrossRef] [Google Scholar]
- Couteur DG, Fraser R, Cogger VC, McLean AJ. Hepatic pseudocapillarisation and atherosclerosis in ageing. Lancet. 2002;359:1612–1615. 10.1016/S0140-6736(02)08524-0. [Abstract] [CrossRef] [Google Scholar]
- Fraser R. The liver in health, and disease. New Zeal Pharmacy. 2002;22:36–40. [Google Scholar]
Articles from Comparative Hepatology are provided here courtesy of BMC
Full text links
Read article at publisher's site: https://doi.org/10.1186/1476-5926-1-1
Read article for free, from open access legal sources, via Unpaywall:
https://comparative-hepatology.biomedcentral.com/track/pdf/10.1186/1476-5926-1-1
Citations & impact
Impact metrics
Article citations
Mouse liver sinusoidal endothelial cell responses to the glucocorticoid receptor agonist dexamethasone.
Front Pharmacol, 15:1377136, 08 Oct 2024
Cited by: 0 articles | PMID: 39439887 | PMCID: PMC11494038
During Postnatal Ontogenesis, the Development of a Microvascular Bed in an Intestinal Villus Depends on Intussusceptive Angiogenesis.
Int J Mol Sci, 25(19):10322, 25 Sep 2024
Cited by: 0 articles | PMID: 39408652 | PMCID: PMC11476829
Circulating extracellular vesicle-derived miR-1299 disrupts hepatic glucose homeostasis by targeting the STAT3/FAM3A axis in gestational diabetes mellitus.
J Nanobiotechnology, 22(1):509, 24 Aug 2024
Cited by: 0 articles | PMID: 39182087 | PMCID: PMC11344378
The journey of decellularized vessel: from laboratory to operating room.
Front Bioeng Biotechnol, 12:1413518, 25 Jun 2024
Cited by: 0 articles | PMID: 38983603 | PMCID: PMC11231200
Review Free full text in Europe PMC
Depletion of Activated Hepatic Stellate Cells and Capillarized Liver Sinusoidal Endothelial Cells Using a Rationally Designed Protein for Nonalcoholic Steatohepatitis and Alcoholic Hepatitis Treatment.
Int J Mol Sci, 25(13):7447, 06 Jul 2024
Cited by: 0 articles | PMID: 39000553
Go to all (378) article citations
Similar Articles
To arrive at the top five similar articles we use a word-weighted algorithm to compare words from the Title and Abstract of each citation.
The role of liver sinusoidal cells in hepatocyte-directed gene transfer.
Am J Pathol, 176(1):14-21, 30 Nov 2009
Cited by: 61 articles | PMID: 19948827 | PMCID: PMC2797863
Review Free full text in Europe PMC
STED microscopy: A simplified method for liver sinusoidal endothelial fenestrae analysis.
Biol Cell, 110(7):159-168, 25 Jun 2018
Cited by: 2 articles | PMID: 29808906
Tracking Fenestrae Dynamics in Live Murine Liver Sinusoidal Endothelial Cells.
Hepatology, 69(2):876-888, 03 Jan 2019
Cited by: 31 articles | PMID: 30137644
Effects of ethanol on liver sinusoidal endothelial cells-fenestrae of rats.
Hepatobiliary Pancreat Dis Int, 4(3):422-426, 01 Aug 2005
Cited by: 12 articles | PMID: 16109529