Abstract
Free full text

Frequency and Phenotype of Circulating Vα24/Vβ11 Double-Positive Natural Killer T Cells during Hepatitis C Virus Infection
Abstract
Natural killer T (NKT) cells are thought to be involved in innate responses against infection. We investigated one specific type of NKT cell, Vα24/Vβ11 double positive, in hepatitis C virus (HCV) infection. Lower frequencies of this population were detected in the blood of HCV PCR-positive patients than in controls. Unlike Vα24/Vβ11 NKT cells found in blood, those in the liver appeared to be recently activated.
Hepatitis C virus (HCV) readily sets up persistent infection in the liver (17). A proportion of those infected control virus spontaneously, and host cellular immune responses are thought to play a key role in this (4). Although much work has focused on the role of conventional antigen-specific T-cell responses, less is known about the role of other lymphocyte subsets, including innate responses (6, 30). A variety of natural killer T (NKT) cells exist with different definitions. Here we focus on a readily defined set of these cells that coexpress Vα24 and Vβ11. In a previous study, the Vα24/Vβ11 double-positive NKT cells were found to overlap almost completely with the cells binding a CD1d-α-galactosylceramide tetramer (14). It is important to note, however, that other CD1d-restricted NKT-cell subsets also exist (1, 2, 8, 9, 31), and other groups have used other markers (such as CD161) or combinations (such as CD3+ CD56+) to identify different subsets that have also been described as “NKT.” We have previously shown that Vα24/Vβ11 double-positive NKT cells are readily detectable in the livers of patients infected with HCV by using either CD1d-glycolipid tetramers (14, 19) or, equivalently, T-cell receptor alpha (TCR-α) and -β-chain-specific antibodies (Vα24 and Vβ11, respectively). This simple, readily available combination of antibodies was thus used in the present study.
NKT cells were initially identified in mice, where a variety of different markers have been used to define them (unlike in humans, where a pair of TCR antibodies is available), although a specific subset is known to be Vα14 positive (15, 29). However, importantly, the same CD1d-glycolipid tetramer recognized by human Vα24/Vβ11 double-positive cells is recognized in the mouse (2). In murine systems, such NKT cells have been shown to express high levels of cytokines, both pro- and anti-inflammatory, upon stimulation (5, 10, 27). As a result, a role for such cells has been proposed in immunoregulation, autoimmunity, transplant rejection, tumor immunity, immunopathology, and immunity against infection (10, 13, 25, 26, 28). Similar functions have been proposed for human Vα24/Vβ11 double-positive NKT cells (10, 16, 22, 23). Little is known about their role in chronic infection, although recent studies of human immunodeficiency virus infection noted that such cells (which can express CD4) were present at reduced frequencies (20, 24, 32). Here, we investigated Vα24/Vβ11 double-positive NKT-cell responses in a cohort of subjects currently or previously infected with HCV and assessed the frequency and phenotype of these cells in the presence or absence of virus, focusing on markers relating to activation and function.
For the analysis of NKT-cell frequencies, HCV-seropositive patients were selected at random from a cohort of patients described previously (18). Forty-seven patients were studied, of whom 23 were consistently PCR negative and 24 were consistently PCR positive. Of the PCR-negative patients, 12 of 23 had previously received therapy (alpha interferon [IFN-α]-ribavirin combination for 6 to 12 months), and of the PCR-positive patients, 10 of 24 had received therapy.
Peripheral blood mononuclear cells (PBMCs) were obtained from patients by centrifugation over Lymphoprep (Nycomed, Oslo, Norway) and stained with monoclonal antibodies (MAbs)specific for TCR Vα24 and Vβ11 (Serotec, Ltd., Oxford, United Kingdom) that were either directly conjugated to phycoerythrin (PE) or fluorescein isothiocyanate (FITC) or biotinylated and used in combination with steptavidin-PerCP (SA-PerCP; Becton Dickinson, Franklin Lakes, N.J.). These stains were combined with FITC- or allophycocyanin (APC)-conjugated antibodies for phenotypic analysis (anti-CD3, CD161, CD4, CD8 α, CD94, CD45RO, CD57, CD27, CD28, CD62L, CD69, CD38, CD25, Ki67, and perforin [all from Becton Dickinson]). Unconjugated antibodies such as anti-CCR7 (Becton Dickinson) and anti-LIR were combined with a secondary goat anti-mouse immunoglobulin conjugated to FITC (Dako, Ltd., Glostrup, Denmark). A mixture of PE-coupled KIR-specific MAbs consisting of the anti-KIR reagents EB6 (anti-KIR2DL1 and anti-KIR2DS1; Coulter, Hialeah, Fla.), DX27 (anti-KIR2DL2/L3 and anti-KIR2DS2), DX9 (anti-KIR3DL1), and DX31 (anti-KIR3DL2) were used to screen for the expression of KIR surface expression. All of the DX antibodies were manufactured by DNAX (Palo Alto, Calif.). HPF1 (anti-ILT2/LIR1) was kindly provided by Miguel Lopez-Botet. Preparation of intrahepatic mononuclear cells for fluorescence-activated cell sorter (FACS) analysis and antibody staining was performed as previously described (14). Because of the low frequency of Vα24/Vβ11 double-positive T cells in some of the patients, a minimum of 500,000 cells was acquired.
Previous studies have often defined NKT cells by use of Vα24 staining alone (30). Therefore, we first identified the optimal methods of staining with TCR antibodies to provide an accurate equivalent to tetramer staining (14). As suggested in a previous study (14), expression of Vα24 on Vβ11-positive lymphocytes was approximately 1 log higher than that on single-positive cells (Fig. (Fig.1a).1a). This distinct pattern of staining was observed in both normal controls and seropositive patients across all subjects studied (Fig. (Fig.1b).1b). Figure Figure1b1b illustrates the mean fluorescence intensity of staining for Vα24 in double- and single-positive cells (left panel) in 18 subjects; in this group, a mean 7-fold difference was seen in staining intensity (range, 2- to 10-fold; P < 0.0001). The levels of expression of Vβ11 were not statistically different between single- and double-positive cells (Fig. (Fig.1b,1b, right panel). Therefore, these cells possess high levels of surface Vα TCR as assessed by antibody binding, a result that is distinct from the murine model, where they are classically Vα TCR intermediate (2). The reason for this apparently high level of Vα24 without an increased level of Vβ11 is not yet clear. It is possible that it relates to an increased affinity of the antibody for the specific Vα24JαQ used by Vα24/Vβ11 double-positive NKT cells. In practical terms, studies relying on Vα24 staining alone may overestimate the numbers of double-positive NKT cells, unless Vα24 high cells are carefully distinguished.
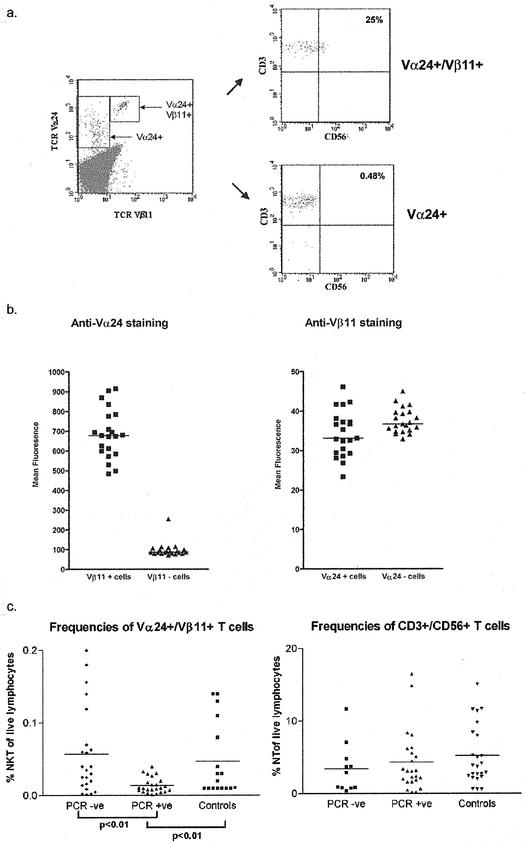
Distinctive staining pattern of NKT cells. (a) Example of staining of PBMCs for Vα24 and Vβ11 expression. (Left panel) PBMCs were stained with directly conjugated antibodies for TCR Vα24 and Vβ11 (PE and FITC conjugates, respectively). All cells displayed fall within the livelymphocyte gate. This stain example is from a representative HCV-seropositive PCR-negative patient, but similar patterns were seen in PCR-positive and control individuals. (Right panels) The top plot shows cells that fall within the Vα24/Vβ11 double-positive gate, which were then analyzed for expression of CD3 and CD56. (PerCP- and APC-conjugated antibodies, respectively, were used.) The bottom plot shows equivalent staining for cells lying in the Vα24 single-positive gate. Note that the CD3 levels are identical in both gates. A higher proportion of CD3 cells in the top plot express CD56, but this still represents only a minority of the total population. (b) Comparison of Vα24 expression on single-positive and Vα24/Vβ11 double-positive cells. (Left panel) Cells stained exactly as in panel a were examined in 21 patients of the study cohort. The mean fluorescence intensity of the Vα24 staining was compared within individuals in the single- and double-positive subsets. (Right panel) Cells of the same cohort were stained exactly as for panel a above, and the level of Vβ11 expression was analyzed in single-positive and Vβ11/Vα24 double-positive subsets. (c) Comparison of frequencies of Vα24/Vβ11 double-positive cells and CD3+ CD56+ cells in controls and seropositive patients. (Left panel) Cells were prepared and stained for Vα24/Vβ11 as described above and as illustrated in panel a. The frequencies of double-positive cells are displayed. PCR+ and PCR− represent two groups of HCV-seropositive patients who are viremic and nonviremic, respectively, while controls are HCV-seronegative healthy donors. The frequencies were compared by using an unpaired t test, with the means for each group indicated by a horizontal bar. Significant differences between the different groups are indicated as P values. No significant difference was seen between healthy controls and HCV-seropositive but nonviremic patients. (Right panel) Equivalent comparison between groups for frequencies of CD3+ CD56+ cells (also described as natural T cells). No significant differences were seen.
By these criteria, analysis of NKT frequencies in PBMCs revealed a range of frequencies in normal individuals of 0.01 to 0.14% of PBMCs, and a similar range was seen in our HCV-seropositive cohort (mean, 0.035% of lymphocytes). However, as illustrated in Fig. Fig.1c,1c, those subjects who were persistently viremic (PCR positive) were found to possess significantly lower levels of NKT cells than PCR-negative patients (mean, 0.014%; P = 0.002). This result was independent of previous treatment (data not shown). The frequencies varied little over time—repetitive analysis of a subset of the normal controls revealed consistent staining patterns over a 12-month period (data not shown). We analyzed a set of relevant controls. First, Vα24 single-positive frequencies were similar in PCR-positive (mean ± standard error [SE], 0.3857 ± 0.0199) and PCR-negative (mean ± SE, 0.3657± 0.0181) patients. Second, frequencies of CD3+ CD56+ cells (also described as “NT,” for “natural T” cells) (7), which may also play a role in hepatic inflammation and immunity, were also not significantly different between PCR-positive (mean ± SE, 4.335 ± 4.202) and PCR-negative patients (mean ± SE, 3.446 ± 3.458) or normal controls (mean ± SE, 5.222 ± 4.094) (Fig. (Fig.1c).1c). Thus, the changes in Vα24/Vβ11 double-positive cell frequencies appeared to be specific for this particular subset.
In terms of cell surface markers, the NKT phenotype was CD3+, with no differences in level between single- and double-positive cells, CD4− CD8α− cells, or CD4+ cells as previously described (2, 10, 27). They expressed classical markers of T-cell memory (CD45RO high, l-selectin low, CD28 high, CCR7 low) (Fig. (Fig.2),2), they were generally low in markers of activation (MHC class II low, CD38 low [although variable in some cases], CD69 low, and CD25 low), and they were not in cycle and lacked intracellular perforin (intracellular Ki67 and perforin low). As expected, the NKT (Vα24/Vβ11 double positive) population was high for the marker CD161, although staining did not reach 100%. Interestingly, the NKT population defined by double-positive TCR antibody (or tetramer) staining was low in expression of KIRs, although some expression of ILTs was observed.
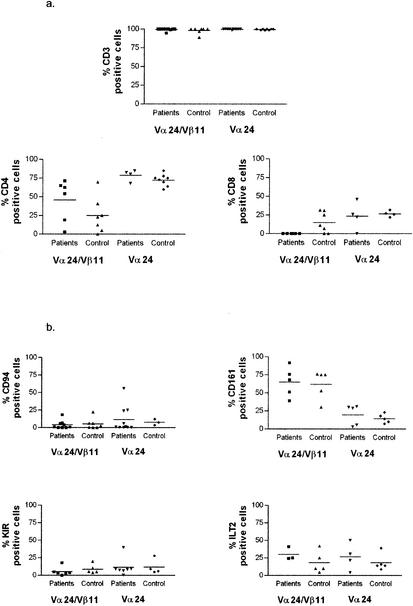
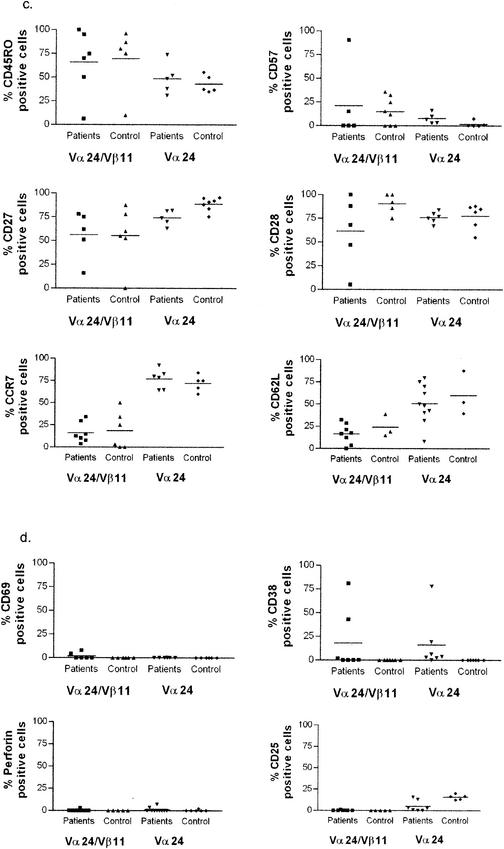
Comprehensive phenotyping of Vα24/Vβ11 double-positive NKT cells in patients and controls. Three to six HCV-seropositive patients were stained for each specific marker, using those patients with sufficiently large ex vivo clouds of double-positive cells (generally >0.05% of lymphocytes) on flow cytometric analysis to provide accurate phenotyping. Significant differences between patients and controls in the double-positive NKT subset were present only for CD8α staining (P < 0.05). The data show the staining characteristics of both double-positive NKT cells (left panel) and, for comparison, Vα24 single-positive T cells (right panel). The data are split into T-cell subset markers (a), NK markers (b), T-cell memory markers (c), and lymphocyte activation/function markers (d).
The Vα24/Vβ11 double-positive cell phenotype in HCV-seropositive individuals did not differ markedly from that of normal controls. Some minor variations in, for example, CD8α CD4 expression were noted, but no excess expression of activation markers such as CD69 or change in KIR expression was observed (Fig. (Fig.2b2b and d). The surface phenotype of Vα24 single-positive cells was distinct from that of Vα24/Vβ11 double-positive NKT cells. In particular, the single-positive cells showed lower expression of CD161 and higher expression in CD62L and CCR7 (Fig. (Fig.2b,2b, c, and d).
Explanations for the lower levels of NKT cells identified in HCV infection may be the down-regulation of TCRs as a result of activation, apoptosis of NKT cells through a similar process, and/or compartmentalization into peripheral organs, including the liver. In murine models, activation of Vα14 single-positive NKT cells by α-galactosylceramide leads to initial cytokine release and then to a sustained reduction specifically in the liver (13). To investigate this specific possibility, we phenotyped hepatic NKT cells by techniques described previously (14) in two HCV PCR-positive individuals. Again in both cases, Vα24/Vβ11 double-positive cells showed high Vα24 expression, although this was not readily apparent in a previous study (14). These populations were highly activated, as defined by high expression of CD69 (Fig. (Fig.2c).2c). CD69 is a marker of recent activation on lymphocytes, including NKT cells (3). These data suggest that NKT cells can be activated within the hepatic compartment, because the level of CD69 was uniformly low on all NKT populations identified in PBMCs (Fig. (Fig.2d2d and and3)3) (11, 21). Further studies are required to assess whether this activation is disease related.
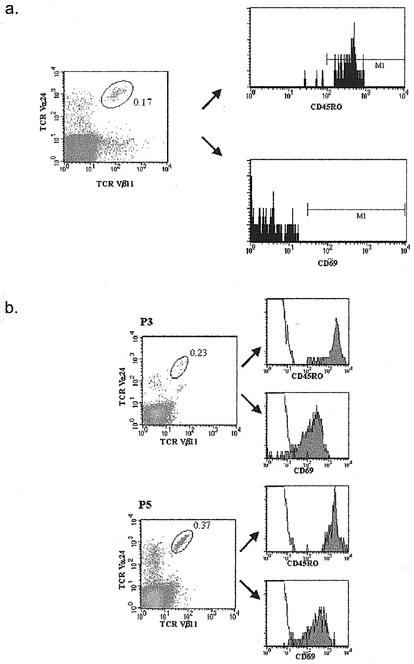
Phenotype of circulating and intrahepatic Vα24/Vβ11 double-positive NKT cells. (a) Phenotype of circulating NKT cells. PBMCs from one representative PCR-negative patient were stained with a directly conjugated antibody specific for TCR Vα24 and a biotinylated antibody to TCR Vβ11 in combination with SA-PerCP. PBMCs were costained with antibodies to CD69 or CD45RO. The histograms show the phenotype of cells falling into the Vα24/Vβ11 double-positive gate. (The gate is indicated, as is the frequency of Vα24/Vβ11 double-positive cells.) (b) Activated phenotype of intrahepatic NKT cells. Intrahepatic lymphocytes from two HCV-seropositive PCR-positive subjects (P3 and P5) were stained with directly conjugated antibodies specific for Vα24/Vβ11 TCR and costained with antibodies to CD69 or CD45RO. The histograms indicate the phenotype of cells falling into the Vα24/Vβ11 double-positive gate. (The gate is indicated, as is the frequency of Vα24/Vβ11 double-positive cells.)
Vα24/Vβ11 double-positive NKT cells may exert antiviral or immunoregulatory function in the liver, although the physiological mechanism involved in their activation in response to viral infection has yet to be determined (6, 10, 12, 30). In murine models, activation by administration of α-galactosylceramide in vivo leads to a release of gamma interferon (IFN-γ) and suppression of hepatitis B virus (HBV) (13). It is likely that the cells activated within the liver subsequently undergo apoptosis as opposed to local proliferation.
It is crucial that, in future studies, the population of NKT cells remains tightly defined. Our findings suggest that the frequency of Vα24/Vβ11 double-positive NKT cells (but not CD3/CD56 double-positive cells or Vα24 single-positive cells) in the PBMC fraction is lower in the presence of circulating virus (PCR positive) than in normal individuals and PCR-negative seropositive patients. It is possible that continuous activation and subsequent apoptosis are responsible for this decrease in frequencies, akin to exhaustion of CD8+ T cells, but many other mechanisms are possible, including disturbance of generation through modulation of CD1d or ligand or disturbance of homeostatic cytokines. Further analysis of the function of these cells and studies of intrahepatic phenotype and distribution are clearly important. While it is not generally required, as we have previously demonstrated, to use CD1d tetramers in humans, combination TCR antibody staining is nevertheless important in both blood and tissue sites, because the frequencies, phenotypes, and presumably functions of Va24 single- and Vα24/Vβ11 double-positive cell subsets are discrete (9, 14). In future studies, it should also be borne in mind that the tight relationship between Vα24/Vβ11 double-positive cells and CD1d tetramer-positive cells seen in this situation may potentially vary in different disease states and sites. This study indicates that persistent HCV infection influences the host Vα24/Vβ11 double-positive NKT response. However, how that response influences the virus is still an open question.
Acknowledgments
We are grateful to the Wellcome Trust and the EU (QLK2-CT-1 999-00356) for financial support.
We thank Scott Ward and Andrew Lucas for critical reading of the manuscript, Simon Hellier and Rodney Phillips for support in the laboratory, and Jane Collier and John Sullivan for very helpful ongoing collaborations and discussions.
REFERENCES
Articles from Journal of Virology are provided here courtesy of American Society for Microbiology (ASM)
Full text links
Read article at publisher's site: https://doi.org/10.1128/jvi.77.3.2251-2257.2003
Read article for free, from open access legal sources, via Unpaywall:
https://europepmc.org/articles/pmc140901?pdf=render
Citations & impact
Impact metrics
Article citations
Altered Frequency, Activation, and Clinical Relevance of Circulating Innate and Innate-Like Lymphocytes in Patients With Alcoholic Liver Cirrhosis.
Immune Netw, 23(3):e22, 04 Apr 2023
Cited by: 1 article | PMID: 37416928 | PMCID: PMC10320422
Innate-like T lymphocytes in chronic liver disease.
Front Immunol, 14:1114605, 15 Mar 2023
Cited by: 3 articles | PMID: 37006304 | PMCID: PMC10050337
Review Free full text in Europe PMC
New insights into iNKT cells and their roles in liver diseases.
Front Immunol, 13:1035950, 26 Oct 2022
Cited by: 14 articles | PMID: 36389715 | PMCID: PMC9643775
Review Free full text in Europe PMC
Peripheral blood iNKT cell activation correlates with liver damage during acute hepatitis C.
JCI Insight, 7(2):e155432, 25 Jan 2022
Cited by: 5 articles | PMID: 34905514 | PMCID: PMC8855829
Age-dependent frequency of unconventional T cells in a healthy adult Caucasian population: a combinational study of invariant natural killer T cells, γδ T cells, and mucosa-associated invariant T cells.
Geroscience, 44(4):2047-2060, 17 Jan 2022
Cited by: 10 articles | PMID: 35038082 | PMCID: PMC8763133
Go to all (74) article citations
Similar Articles
To arrive at the top five similar articles we use a word-weighted algorithm to compare words from the Title and Abstract of each citation.
Circulating Valpha24+Vbeta11+ NKT cell numbers and dendritic cell CD1d expression in hepatitis C virus infected patients.
Clin Immunol, 114(2):183-189, 01 Feb 2005
Cited by: 28 articles | PMID: 15639652
Selective decrease in circulating V alpha 24+V beta 11+ NKT cells during HIV type 1 infection.
J Immunol, 168(3):1490-1495, 01 Feb 2002
Cited by: 115 articles | PMID: 11801694
Atopic dermatitis and Valpha24+ natural killer T cells.
Skinmed, 6(5):218-220, 01 Sep 2007
Cited by: 8 articles | PMID: 17786098
Lymphocyte distribution and intrahepatic compartmentalization during HCV infection: a main role for MHC-unrestricted T cells.
Arch Immunol Ther Exp (Warsz), 50(5):307-316, 01 Jan 2002
Cited by: 9 articles | PMID: 12455864
Review