Abstract
Free full text

Prevalence of Genes Encoding Pyrogenic Toxin Superantigens and Exfoliative Toxins among Strains of Staphylococcus aureus Isolated from Blood and Nasal Specimens
Abstract
A total of 429 different Staphylococcus aureus isolates encompassing 219 blood isolates and 210 isolates taken from anterior nares were systematically searched by two multiplex PCR-DNA enzyme immunoassays (PCR-DEIA) for exfoliative toxin (ET) genes eta and etb, as well as for the classical members of the pyrogenic toxin superantigen (PTSAg) gene family comprising the staphylococcal enterotoxin (SE) genes sea-see and the toxic shock syndrome toxin 1 gene tst. In addition, a third PCR-DEIA was established to investigate the possession of four recently described SE genes, viz. seg-sej. The most frequent PTSAg/ET genes amplified were seg and sei, which were found strictly in combination in 55.0% of the S. aureus isolates tested. Other frequently detected toxin genes were tst (20.3%), sea (15.9%), and sec (11.2%). Only five isolates harbored ET genes. Regarding the origin of the S. aureus isolates, a significant difference (P = 0.037) was found for the possession of the sed/sej gene combination (10.5% of blood isolates versus 3.3% of nasal strains). Overall, about half of S. aureus isolates tested harbored genes of the classical members of the PTSAg family and ETs (50.8%), whereas 73.0% of S. aureus isolates were toxin gene positive if the recently described SE genes were included. This notable higher prevalence indicates that the possession of PTSAg genes in particular seems to be a habitual feature of S. aureus. Moreover, mainly due to the fixed combinations of seg plus sei, as well as sed plus sej, the possession of multiple PTSAg genes (62.9%) is more frequent than assumed so far.
Staphylococcus aureus, which has its ecologic niche in the anterior nares, has been shown to cause a variety of infectious diseases ranging from superficial skin infections to severe systemic infections including toxin-mediated diseases (23). By virtue of exfoliative toxins (ETs) and pyrogenic toxin superantigens (PTSAgs) comprising toxic shock syndrome toxin 1 (TSST-1) and the staphylococcal enterotoxins (SEs), S. aureus causes the staphylococcal scalded-skin syndrome (SSSS), the staphylococcal toxic shock syndrome (TSS), and staphylococcal food poisoning (SFP) (3, 7). Besides their specific toxic properties, the members of the PTSAg family share several structural features and biological characteristics, such as pyrogenicity, superantigenicity, and the ability to enhance the susceptibility to endotoxin shock (20, 36).
In addition to the classical five major antigenic types of SEs (SEA, SEB, SEC, SED, and SEE) (11), four additional SEs (SEG, SEH, SEI, SEJ) have been reported, and their corresponding genes have been described (30, 34, 42). For SEC and SEG, several subtypes have been characterized (1, 24). Most recently, the “alphabet” of the SE family was expanded by the detection of further genes (sek, sel, sem, and seo) encoding enterotoxin homologues (18, 21, 31).
In previous studies, the prevalence of classical-toxin-producing S. aureus strains has been investigated by conventional methods based on immunological procedures measuring the toxins in the culture supernatants of suspected strains (22, 27, 37). As a consequence of the availability of commercial tests, these studies were restricted to five classical enterotoxins: SEA to SED and TSST-1. Furthermore, the immunological methods used thus far are known to be limited in sensitivity and specificity (6, 16, 32). To date, no valid data are available concerning the prevalence of the more recently described enterotoxins in S. aureus isolates derived from clinical specimens.
Molecular methods offer alternative ways to detect S. aureus isolates with the genetic information to produce toxins irrespective of their expression. Previously, we described the rapid and specific detection of toxigenic S. aureus isolates by the use of two multiplex PCR-DNA enzyme immunoassays (PCR-DEIA) for amplification and hybridization of the classical ET genes (eta and etb), the SE genes (sea, seb, sec1 to sec3, sed, and see), and the TSST-1 gene (tst) (4). For the study reported here, this approach was extended by developing a further multiplex PCR-DEIA targeting SEG-SEJ genes (seg, seh, sei, and sej).
The goals of the present study were (i) to extend the two multiplex PCR-DEIAs by a third one targeting the newly described SE genes, (ii) to investigate the prevalence of PTSAgs and ETs in a well-defined S. aureus strain collection obtained during the course of a German multicenter study, and (iii) to analyze possible differences in toxin gene equipment between colonizing isolates and isolates involved in severe systemic infection.
MATERIALS AND METHODS
Bacterial strains.
A total of 429 S. aureus isolates were collected and identified during the course of a German multicenter study that included general and intensive care units at 32 university and community hospitals (40). All 219 blood isolates obtained from patients with S. aureus bacteremia during the multicenter study were included here. The most frequently clinically presumed causes of bacteremia were catheter-related infections (46%); osteomyelitis, skin and soft tissue infections such as cutaneous abscesses, and cutaneous ulcerations (27%); and lower-respiratory-tract infections (11%). In addition to the blood isolates, a total of 210 S. aureus nasal isolates prospectively collected over a 6-year period during routine surveillance were also tested for the exotoxin genes. None of the patients who were nasally colonized and who were selected for the present study subsequently developed S. aureus bacteremia within that study period (40). Twenty (9.1%, exhibiting nine genotypes) of the patients with S. aureus bacteremia and six (2.9%, exhibiting five genotypes) of the S. aureus nasal carriers harbored methicillin-resistant S. aureus strains.
DNA isolation procedures.
Staphylococcal cells were collected from 0.5 ml of an overnight brain heart infusion broth culture. Cells were pelleted by centrifugation at 5,000 × g for 20 min, resuspended in 185 μl of TE buffer (20 mM Tris chloride, 2 mM EDTA [ph 8.0]) with 15 μl of recombinant lysostaphin (15 mg/ml; Sigma, St. Louis, Mo.), and incubated at 37°C for 30 min. DNA was subsequently extracted with a QIAamp tissue kit (Qiagen, Hilden, Germany) according to the manufacturer's recommendations. Nucleic acid samples were eluted with distilled water and adjusted to a final concentration of 1 μg/ml according to A260 values.
Multiplex PCR-DEIA procedure.
Both the multiplex PCR-DEIA to detect simultaneously the classical SE genes (sea, seb, sec1 to sec3, sed, and see) and the multiplex PCR-DEIA to detect the ET genes (eta and etb) and the TSST-1 gene (tst) were performed as previously described (4). The newly designed oligonucleotide primers to detect the recently described SE genes seg, seh, sei, and sej by a third multiplex PCR procedure are listed in Table Table1.1. The assay conditions for these genes corresponded with that of the published multiplex PCR assays. For carryover prevention, the uracil DNA glycosylase system (Boehringer Mannheim, Mannheim, Germany) was used for all multiplex PCR assays. The amplification was performed in an iCycler (Bio-Rad, Munich, Germany). For hybridization of the amplified DNA generated by the multiplex PCR assays, a generic DNA enzyme immunoassay (GEN-ETI-K DEIA; Sorin, Saluggia, Italy) was used according to the manufacturer's recommendations and as described elsewhere (4). Four additional probes were developed to control the specificity of the multiplex PCR by using reference strains harboring seg to sej. (Table (Table2).2). Positive controls for the PCR-DEIAs included DNA from toxin-producing S. aureus reference strains (Table (Table3).3). In addition to standard PCR controls for contamination events, S. aureus Cowan 1 and S. epidermidis DSM 20044 served as negative controls.
TABLE 1.
Base sequences, gene locations, and predicted sizes of PCR products for the oligonucleotide primers to amplify specifically fragments of the SE genes seg to sej
Genea | Primer | Oligonucleotide sequence (5′-3′) | Location within gene (nucleotide range) | Size of PCR product (bp) |
---|---|---|---|---|
segb | SEG-1W | AAT GCY CAA CCY GAT CCT A | 223-241 | 116 |
SEG-4 | CTT CCT TCA ACA GGT GGA GAC | 318-338 | ||
seh | SEH-1 | TTA GAA ATC AAG GTG ATA GTG GC | 407-429 | 235 |
SEH-2 | TTT TGA ATA CCA TCT ACC CAA AC | 619-641 | ||
sei | SEI-1 | GCC ACT TTA TCA GGA CAA TAC TT | 469-491 | 330 |
SEI-2 | AAA ACT TAC AGG CAG TCC ATC TC | 776-798 | ||
sej | SEJ-1 | CTC CCT GAC GTT AAC ACT ACT AAT AA | 940-965 | 641 |
SEJ-2 | TTG TCT GGA TAT TGA CCT ATA ACA TT | 1580-1605 |
TABLE 2.
Base sequences and gene locations for the seg-sej-specific 5′-biotinylated oligonucleotide probes
Genea | Probeb | Oligonucleotide sequence (5′-3′) | Location within gene (nucleotide range) |
---|---|---|---|
segc | SEG-8B | GAT TCA TTA CAT TAC CCA TAG TTC CC | 555-580 |
seh | SEH-3B | ACT GCT GAT TTA GCT CAG AAG TTT A | 454-478 |
sei | SEI-3B | ACA CTG GTA AAG GCA AAG AAT ATG | 656-679 |
sej | SEJ-3B | GGG TAT CTC TGA AAA GAT AAT GAC A | 1087-1111 |
TABLE 3.
Results of testing reference strains for SE genotypes seg to sej derived from agarose gel analysis of multiplex PCR and colorimetric microtiter plate DEIA
Reference strain | Toxin genotype determined by previous study | Genotype as determined by:
| Reference or source | |||||||
---|---|---|---|---|---|---|---|---|---|---|
Agarose gel analysisa
| Colorimetric assayb (OD ratio at 450/630 nm)
| |||||||||
seg | seh | sei | sej | seg | seh | sei | sej | |||
ATCC 13565 | seac | − | − | − | + | 0.035 | 0.118 | 0.035 | 1.617 | ATCC |
ATCC 14458 | seb | − | − | − | − | 0.035 | 0.044 | 0.042 | 0.070 | ATCC |
ATCC 19095 | sec | + | + | + | − | 1.023 | 2.127 | >2.500 | 0.071 | ATCC |
ATCC 23235 | sed | + | − | + | + | 2.399 | 0.022 | 2.384 | 2.361 | ATCC |
ATCC 27664 | see | − | − | − | − | 0.056 | 0.090 | 0.120 | 0.076 | ATCC |
MJB 1320 | seid,e | − | − | + | − | 0.033 | 0.048 | >2.500 | 0.053 | 30 |
FRI 445 | seg seid,f | + | − | + | − | 2.228 | 0.052 | >2.500 | 0.052 | 28, 30 |
FRI 569 | sehd | − | + | − | − | 0.047 | 2.131 | 0.105 | 0.054 | 38 |
FRI 572 | seg seid | + | − | + | − | 1.976 | 0.045 | >2.500 | 0.062 | 28, 30, 38 |
FRI 1472 | sed seg sei sejd | + | − | + | + | 2.346 | 0.039 | >2.500 | 2.067 | 42 |
KN813 | tst | + | − | + | − | 0.139 | 0.045 | 0.067 | 0.058 | 4 |
BM 10458 | eta | − | − | − | − | 0.080 | 0.079 | 0.083 | 0.077 | 4 |
BM 10143 | etb | − | − | − | − | 0.096 | 0.074 | 0.129 | 0.065 | 4 |
Cowan 1g | Nontoxigenic | + | − | + | − | 0.070 | 0.046 | 0.083 | 0.085 | 4 |
ATCC 20044g | Nontoxigenic | − | − | − | − | 0.036 | 0.041 | 0.033 | 0.079 | 4 |
Fingerprinting by ERIC-PCR.
Genotyping of sed/sej-positive isolates by using ERIC2 primer for the enterobacterial repetitive intergenic consensus (ERIC) sequence was performed as described by van Belkum et al. (39) with modifications concerning the thermal cycling conditions and the use of 0.5 U of AmpliTaq DNA polymerase, Stoffel fragment (Perkin-Elmer, Branchburg, N.J.). The thermal cycling conditions were 35 cycles of denaturation at 94°C for 1 min (5 min for the first cycle), annealing at 35°C for 1 min, and polymerization at 72°C for 2 min. Drastically prolonged ramp times (5 min) were used (12). Amplified products (10 μl) were resolved by 2% agarose gel electrophoresis at 150 V for 1.5 h. The gel was stained with ethidium bromide and then exposed to UV light (254 nm) to visualize the amplified products.
Statistical analysis.
Differences between groups were assessed by using the chi-square test. If more than one exotoxin gene in various combinations was found, multivariate analysis by logistic regression was used to establish their importance (10). Epi-Info 2000 (Centers for Disease Control and Prevention, Atlanta, Ga.) was used to perform calculations. An odds ratio higher than 1 within a confidence interval of 95% were taken into account for associations. P values of <0.05 were considered statistically significant.
RESULTS
Evaluation of the detection system.
The previously published PCR results (4) and the newly established multiplex PCRs with primer pairs specifically for (i) sea to see; (ii) tst, eta, and etb; and (iii) seg to sej genes successfully amplified fragments from the DNA of S. aureus reference strains (Fig. (Fig.11 and Table Table3).3). PCR amplifications were confirmed by the respective hybridization reactions in the DEIA system for all reference strains used (Table (Table3).3). The sensitivity of the multiplex PCR-DEIAs reached the expected range, as previously described (4). None of the primer pairs and the respective hybridization probes reacted with the negative control strain Staphylococcus epidermidis ATCC 20044. S. aureus Cowan 1, shown to be nontoxigenic regarding the classical PTSAg and ET genes (4), was found to be PCR positive for seg-sei gene combination but was below the detection point for DEIA hybridization for both genes. The same findings regarding the seg-sei combination were observed for S. aureus KN 813, which is known to be tst positive (Table (Table3).3). It is worth noting that amplification products with primers specific for sed and sej were found for S. aureus ATCC 13565 in addition to the detection of the sea gene. To date, this strain has been described only as SEA positive (American Type Culture Collection [ATCC] catalog, 19th edition) and has not been recognized as possessing the sed-sej gene combination. This finding was confirmed by a respective hybridization procedure. Production of SED by this strain was verified by staphylococcal enterotoxin test-reversed passive latex agglutination and Ridascreen-EIA as previously described (4). The possession of the newly described SE genes was also detected for ATCC strains 19095 and 23235, hitherto only known to be sec and sed positive, respectively (ATCC catalog) (Table (Table33).
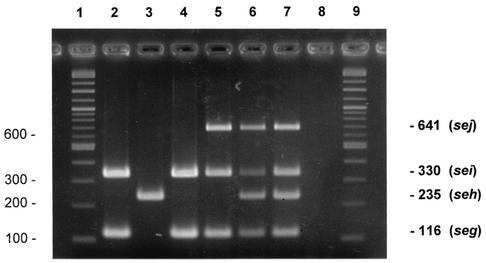
Agarose gel electrophoreses patterns showing PCR-amplified products in multiplex PCR for the SE genes seg, seh, sei, and sej. Lanes: 1 and 9, DNA molecular weight marker (1 kb/100-bp DNA ladder); 2, FRI 572 (seg and sei positive); 3, FRI 569 (seh positive); 4, FRI 445 (seg and sei positive); 5, FRI 1472 (seg, sei, and sej positive); 6, multiplex PCR with all enterotoxin genes simultaneously (seg to sej); 7, artificial arrangement of the amplification fragments of seg to sej; 8, S. epidermidis ATCC 20044 (negative control). Sizes are indicated in base pairs on the left and right of the figure.
Detection of PTSAg and ET genes.
Data on the prevalence of PTSAg and ET genes testing 429 clinical S. aureus isolates by multiplex-PCRs are shown in Table Table4.4. Overall, 313 (73.0%) isolates were positive for at least one of the exotoxin genes tested.
TABLE 4.
Results of testing 429 clinical S. aureus isolates for staphylococcal PTSAg and ET genes by multiplex PCR
Result of PCR testing | No. and percentages of isolates
| Pa | |||||
---|---|---|---|---|---|---|---|
Blood (n = 219)
| Nasal swabs (n = 210)
| Total (n = 429)
| |||||
n | % | n | % | n | % | ||
sea positive | 38 | 17.4 | 30 | 14.3 | 68 | 15.9 | 0.756 |
seb positive | 13 | 5.9 | 16 | 7.6 | 29 | 6.8 | 0.214 |
sec positive | 19 | 8.7 | 29 | 13.8 | 48 | 11.2 | 0.911 |
sed positive | 23 | 10.5 | 7 | 3.3 | 30 | 7.0 | 0.037 |
see positive | 1 | 0.5 | 1 | 0.5 | 2 | 0.5 | 0.902 |
seg positive | 116 | 53.0 | 120 | 57.1 | 236 | 55.0 | 0.062 |
seh positive | 10 | 4.6 | 13 | 6.2 | 23 | 5.4 | 0.290 |
sei positive | 116 | 53.0 | 120 | 57.1 | 236 | 55.0 | 0.062 |
sej positive | 23 | 10.5 | 7 | 3.3 | 30 | 7.0 | 0.037 |
tst positive | 40 | 18.3 | 47 | 22.4 | 87 | 20.3 | 0.498 |
eta positive | 1 | 0.5 | 4 | 1.9 | 5 | 1.2 | ND |
etb positive | 0 | 0.0 | 2 | 1.0 | 2 | 0.5 | ND |
The most commonly found genes were the more recently described enterotoxin genes seg and sei (n = 236 [55.0%]), which were strictly found in association with one another. Regarding the classical enterotoxin genes, the tst gene (n = 87 [20.3%]) was the most frequently detected gene, followed by sea (n = 68 [15.9%]) and sec (n = 48 [11.2%]). Fragments of the sed gene were amplified in a total of 30 isolates (7.0%), always in combination with the newly described enterotoxin gene sej. Isolates harboring other PTSAg genes were infrequently recovered (Table (Table44).
The possession of more than a single PTSAg and/or ET gene was observed in the majority of both nasal and blood isolates (62.9%); this was mainly due to the fixed combinations of seg plus sei (n = 236 [55.0%]) and sed plus sej (n = 30 [7.0%]) (Table (Table5).5). Overall, a multitude of PTSAg gene combinations were observed, including isolates with the combination of two (n = 115), three (n = 103), four (n = 46), or five (n = 6) different genes. Apart from the fixed gene combinations, the most frequent combination detected was seg-sei plus tst occurring in 8.6% of all isolates. Altogether, 80 (18.6%) isolates harbored tst in various combinations, including sea-seg-sei (5.8%), seh-seg-sei (1.4%), and several other gene combinations. In contrast, seven (1.6%) isolates were observed with tst only. Further frequent nonfixed gene combinations were found for sea-positive isolates (11.9%) and sec-positive isolates (10.0%). Similar to tst, the sole presence of sea (4.0%) or sec (1.2%) is much less common than their presence in combination with other toxin genes. The second frequent gene combination was seg-sei plus sec (7.7%), followed by seg-sei plus sea and tst (5.8%). The seg-sei gene combination was detected in all isolates simultaneously bearing four (n = 46) or five (n = 6) different PTSAg genes. Overall, only 42 (9.8%) of all isolates tested harbored a single PTSAg gene, representing 13.5% of all PTSAg gene-positive isolates.
TABLE 5.
Single and multiple possession of staphylococcal PTSAg and ET genes
SE genotype | S. aureus PTSAg/ET gene-positive isolates
| |||||
---|---|---|---|---|---|---|
Blood (n = 219)
| Nasal swabs (n = 210)
| Total (n = 429)
| ||||
n | % | n | % | n | % | |
Single possession | ||||||
![]() ![]() ![]() ![]() | 9 | 4.1 | 8 | 3.8 | 17 | 4.0 |
![]() ![]() ![]() ![]() | 7 | 3.2 | 3 | 1.4 | 10 | 2.3 |
![]() ![]() ![]() ![]() | 3 | 1.4 | 2 | 1.0 | 5 | 1.2 |
![]() ![]() ![]() ![]() | 1 | 0.5 | 2 | 1.0 | 3 | 0.7 |
![]() ![]() ![]() ![]() | 3 | 1.4 | 4 | 1.9 | 7 | 1.6 |
![]() ![]() ![]() ![]() | 0 | 0.0 | 1 | 0.5 | 1 | 0.2 |
![]() ![]() ![]() ![]() | 23 | 10.5 | 20 | 9.5 | 43 | 10.0 |
Multiple possessionb | ||||||
![]() ![]() ![]() ![]() | 29 | 13.2 | 22 | 10.5 | 51 | 11.9 |
![]() ![]() ![]() ![]() | 6 | 2.7 | 13 | 6.2 | 19 | 4.4 |
![]() ![]() ![]() ![]() | 16 | 7.3 | 27 | 12.9 | 43 | 10.0 |
![]() ![]() ![]() ![]() | 23 | 10.5 | 7 | 3.3 | 30 | 7.0 |
![]() ![]() ![]() ![]() | 1 | 0.5 | 1 | 0.5 | 2 | 0.5 |
![]() ![]() ![]() ![]() | 116 | 53.0 | 120 | 57.1 | 236 | 55.0 |
![]() ![]() ![]() ![]() | 9 | 4.1 | 11 | 5.2 | 20 | 4.7 |
![]() ![]() ![]() ![]() | 37 | 16.9 | 43 | 20.5 | 80 | 18.6 |
![]() ![]() ![]() ![]() | 1 | 0.5 | 3 | 1.4 | 4 | 0.9 |
![]() ![]() ![]() ![]() | 0 | 0.0 | 2 | 1.0 | 2 | 0.5 |
![]() ![]() ![]() ![]() | 140 | 63.9 | 130 | 61.9 | 270 | 62.9 |
Total positivec | 163 | 74.4 | 150 | 71.4 | 313 | 73.0 |
![]() ![]() ![]() ![]() | 111 | 50.7 | 107 | 51.0 | 218 | 50.8 |
Total negativee | 56 | 25.6 | 60 | 28.6 | 116 | 27.0 |
![]() ![]() ![]() ![]() | 108 | 49.3 | 103 | 49.0 | 211 | 49.2 |
Among the 429 isolates examined, only five (1.2%) isolates were positive for one or both ET genes tested (Tables (Tables44 and and5).5). Of these, one eta-positive blood isolate was found that also carried the seg-sei gene combination. The other four ET gene-positive isolates were derived from nasal swabs. Two of these isolates harbored both eta and etb genes (Table (Table55).
Comparing blood and nasal isolates.
The association between the detection of PTSAg and ET genes and the origin of isolation was studied by multivariate analysis (Table (Table4).4). No significant difference (P = 0.782) was found with regard to the overall possession of all genes tested between blood isolates (n = 163 [74.4% PCR positive)] and those isolates derived from nasal swabs (n = 150 [71.4% PCR positive]). This was also true for the group of isolates that were PCR negative in assays of only the classical PTSAg and ET genes (P = 0.975). However, an analysis of the subgroups showed that the sed-sej gene combination was significantly more present in blood culture isolates (odds ratio = 2.65; 95% confidence interval = 1.1 to 6.6; P = 0.037). The likelihood ratio chi-square test showed a value of 22.85 with 7 degrees of freedom (P = 0.0027). Genotyping of sed-sej-positive isolates by ERIC-PCR (data not shown) revealed that the 23 sed-sej-positive blood isolates belonged to 18 genotypes, since the seven nasal isolates accounted for four genotypes. Three of the sed-sej-possessing blood isolates were identified as methicillin-resistant S. aureus representing different genotypes.
DISCUSSION
Epidemiological studies of well-defined clinical S. aureus populations by molecular methods for the investigation of both classical and newly described PTSAg and ET genes are generally lacking. Therefore, the presence of these genes was studied in 429 well-characterized S. aureus isolates (40).
Regarding classical staphylococcal members of the PTSAg family, the overall percentage was ca. 10% higher compared to data given in the literature (22, 27, 37). However, most previous studies were based on the detection of toxin production. The fact that toxin gene-positive strains tested negative in immunological assays may be caused either by real nonproduction of the respective toxins or by a weak expression below the detection limit (2, 5). It is well documented that as little as smallest amounts of PTSAgs may induce T-cell stimulation triggering systemic illness such as PTSAg-induced shock and autoimmunity (14, 26, 29). Furthermore, it is known that toxin production can be influenced by many factors that do not influence molecular methods (19). Thus, strains without detectable production of PTSAgs in vitro should not be debarred from epidemiological studies.
Whereas nearly all strains of S. aureus produce enzymes and hemolysins that contribute to their pathogenicity, it has been generally accepted that only some strains produce ETs and PTSAgs (8, 11). However, considering both the newly published SE genes (seg, seh, sei, and sej) and the classical toxins, the overall rate of toxin gene-positive isolates in the study presented here reached 73.0%. More than half of the isolates (55.0%) harbored the combination of seg and sei either alone (20.7%) or in further combinations with other toxin genes tested. Recently, Monday and Bohach (28) showed in a limited number of isolates (n = 15) carrying the seg determinant that this gene is linked to the sei gene, which is located 2,002 bp upstream of seg. For all 236 seg-sei-positive isolates, this fixed combination was confirmed in the context of our study. In contrast, McLauchlin et al. (25) reported on 25 strains, isolated from suspected SFP incidents, that harbored seg alone. Possible explanations for these contradictionary observations may be the special food-borne origin of these isolates or the existence of still-unknown variants of sei gene as already known for sec and seg (1, 24). Sequence variations might explain why some isolates tested positive for seg and sei by PCR but remained negative by using the respective DEIA gene probes.
A further coexisting gene combination was recently reported for the SED-encoding plasmid which encodes a further SE designated SEJ. The SEJ gene is transcribed in a direction opposite to sed and is separated by an 895-bp intergenic region (42). The suggestion of Zhang et al. (42) that the sej determinant may be present on all sed-encoding plasmids originally tested on six strains could be confirmed in our study with 30 sed-positive isolates, which always tested as sej positive.
The role of the recently described SEs as causative agents in toxin-mediated staphylococcal diseases or in enhancing the severity of S. aureus infections still remains unclear. Nevertheless, the isolation of S. aureus strains implicated in outbreaks of SFP without producing any of the classical SEs but possessing genes encoding SEG to SEJ has been described (25). In S. aureus strains isolated from patients with TSS or SSSS but known to be negative for the classical PTSAgs and ETs, Jarraud et al. (17) detected the seg-sei gene combination. Thus, these authors suggested that both SEs might be capable of causing TSS or SSSS. In view of our results, this has to be further investigated since more than 50% of all isolates tested possess these genes.
In addition to the fixed combinations seg-sei and sed-sej, nonfixed PTSAg/ET combinations were found to be more common than has been reported in the literature (22, 41). Overall, the possession of more than one of the genes tested (including fixed combinations) was more frequent (62.9%) compared to the presence of only a single gene (10.0%). In contrast to a previous report (35), seh was found in association with a number of other PTSAg genes such as sea, seb, sec, seg-sei, and tst.
Regarding ET encoding genes, the low rates of positive isolates (eta [1.2%] and etb [0.5%]) found here are in accordance with the results of other investigations even though epidemiological data on S. aureus strains producing epidermolytic toxins are scarce (9, 13, 15).
Upon comparing S. aureus isolates derived from blood versus carriage isolates, we found no significant differences with regard to the overall possession of all PTSAg- and ET-encoding genes tested. However, when we analyzed the prevalence of single genes in both study populations, the fixed gene combination sed-sej was significantly more common in blood isolates (P = 0.037). By genotyping the sed-sej carrying isolates, these isolates were found to belong predominantly to different clones. This finding is in agreement with a previous observation reporting on five of six SED-producing isolates originated from blood cultures (22). Most recently, Peacock et al. showed (33) that sej contributed independently to the virulence of S. aureus.
Our study is the first systematic and comprehensive search for the classical, as well as for the recently described, PTSAg and ET genes in a large number of well-characterized clinical S. aureus isolates. Genes of the PTSAg family were shown to be considerable more common in S. aureus than was assumed thus far. Thus, toxinogenic or superantigenic abilities seem to be a habitual feature of this pathogen. Except for the sed-sej gene combination, which was more present in blood isolates, no significant differences were found upon comparison of blood and nasal isolates. The potential contribution of sed-sej to the overall pathogenicity potential of S. aureus should be investigated further.
Acknowledgments
We are grateful to Michaela Brück, Martina Schulte, Brigitte Schuhen, and Susanne Weber for excellent technical assistance. We thank G. A. Bohach, N. El Solh, S. H. Munson, and A. C. L. Wong for kindly donating staphylococcal reference strains.
This study was supported by the Network of Competence “Pathogenomics,” Alliance “Gram-Positive Cocci,” and was conducted under the auspices of the Paul Ehrlich Society (Staphylococcus Working Group).
REFERENCES
Articles from Journal of Clinical Microbiology are provided here courtesy of American Society for Microbiology (ASM)
Full text links
Read article at publisher's site: https://doi.org/10.1128/jcm.41.4.1434-1439.2003
Read article for free, from open access legal sources, via Unpaywall:
https://europepmc.org/articles/pmc153929?pdf=render
Citations & impact
Impact metrics
Citations of article over time
Alternative metrics
Smart citations by scite.ai
Explore citation contexts and check if this article has been
supported or disputed.
https://scite.ai/reports/10.1128/jcm.41.4.1434-1439.2003
Article citations
Comparison of genotyping methods and toxin gene profiles of Staphylococcus aureus isolates from clinical specimens.
Genet Mol Biol, 46(4):e20220321, 22 Dec 2023
Cited by: 1 article | PMID: 38224487 | PMCID: PMC10789239
Clinical and Bacteriological Analyses of Biofilm-Forming Staphylococci Isolated from Diabetic Foot Ulcers.
Infect Drug Resist, 16:1737-1750, 24 Mar 2023
Cited by: 4 articles | PMID: 36999125 | PMCID: PMC10046123
Spectrum of Virulence Factors in Clinical Isolates of Staphylococcus aureus and Prevalence of SCCmec Types in Methicillin-Resistant Staphylococcus aureus in a Tertiary Care Center.
J Lab Physicians, 15(3):450-461, 27 Mar 2023
Cited by: 3 articles | PMID: 37564222 | PMCID: PMC10411210
Superantigens, a Paradox of the Immune Response.
Toxins (Basel), 14(11):800, 18 Nov 2022
Cited by: 9 articles | PMID: 36422975 | PMCID: PMC9692936
Review Free full text in Europe PMC
Local Epidemiology of Nosocomial Staphylococcus aureus Infection in a Nigerian University Teaching Hospital.
Antibiotics (Basel), 11(10):1372, 07 Oct 2022
Cited by: 2 articles | PMID: 36290030 | PMCID: PMC9598115
Go to all (187) article citations
Other citations
Similar Articles
To arrive at the top five similar articles we use a word-weighted algorithm to compare words from the Title and Abstract of each citation.
Prevalence of staphylococcal enterotoxins, toxin genes and genetic-relatedness of foodborne Staphylococcus aureus strains isolated in the Marmara Region of Turkey.
Int J Food Microbiol, 148(2):99-106, 14 May 2011
Cited by: 47 articles | PMID: 21652103
Investigation of toxin genes by polymerase chain reaction in Staphylococcus aureus strains isolated from bovine mastitis in Turkey.
Foodborne Pathog Dis, 6(8):1029-1035, 01 Oct 2009
Cited by: 20 articles | PMID: 19642915
Novel multiplex PCR for the detection of the Staphylococcus aureus superantigen and its application to raw meat isolates in Korea.
Int J Food Microbiol, 117(1):99-105, 06 Mar 2007
Cited by: 67 articles | PMID: 17439826
Characterization of colonizing Staphylococcus aureus isolated from surgical wards' patients in a Nigerian university hospital.
PLoS One, 8(7):e68721, 23 Jul 2013
Cited by: 25 articles | PMID: 23935883 | PMCID: PMC3720814