Abstract
Free full text

Chromatin assembly factor 1 is essential and couples chromatin assembly to DNA replication in vivo
Abstract
De novo chromatin assembly maintains histone density on the daughter strands in the wake of the replication fork. The heterotrimer chromatin assembly factor 1 (CAF-1) couples DNA replication to histone deposition in vitro, but is not essential for yeast cell proliferation. Depletion of CAF-1 in human cell lines demonstrated that CAF-1 was required for efficient progression through S-phase. Cells lacking CAF-1 accumulated in early and mid S-phase and replicated DNA slowly. The checkpoint kinase Chk1, but not Chk2, was phosphorylated in response to CAF-1 depletion, consistent with a DNA replication defect. CAF-1-depleted cell extracts completely lacked DNA replication-coupled chromatin assembly activity, suggesting that CAF-1 is required for efficient S-phase progression in human cells. These results indicate that, in contrast to yeast, human CAF-1 is necessary for coupling chromatin assembly with DNA replication.
In eukaryotic cells, DNA is packaged into chromatin that is the in vivo substrate for the DNA replication machinery. Parental nucleosomes are transiently removed from DNA and quickly assemble behind the DNA replication fork (1–4). New nucleosomes are also deposited de novo at the replication fork in a reaction catalyzed by chromatin assembly factor 1 (CAF-1) (5, 6). CAF-1 consists of three polypeptides with apparent masses of 150, 60, and 48 kDa that bind histones H3 and H4 and deposits the histone tetramer preferentially onto replicating DNA (7). H2A/B dimers are then loaded in a second step that is not necessarily coupled to DNA replication (8). The biochemical activity of CAF-1 in nucleosome deposition depends on its interaction with the DNA polymerase sliding clamp, proliferating cell nuclear antigen (PCNA) (9–11) and consistent with its activity in vitro, CAF-1 is found in replication foci during S-phase and is also associated with PCNA foci after DNA damage (9, 12, 13). CAF-1 also deposits nucleosomes during DNA repair on damaged DNA templates in vitro. This intimate connection between CAF-1 and the replication machinery suggests that tightly coupling DNA replication and chromatin assembly may be required for normal S-phase progression.
Given that de novo nucleosome assembly is likely an essential process, it is surprising that yeast lacking CAF-1 are viable with no apparent cell proliferation phenotype (14, 15). This observation suggested that other chromatin assembly pathways compensate for the loss of yeast CAF-1. Indeed, other factors, such as Asf1 and the histone regulating (Hir) proteins, are known histone binding proteins (16, 17), and deletion of these genes in combination with CAF-1 leads to severe gene silencing and cell proliferation defects (18–20). The human HIRA protein, an ortholog of yeast Hir1, Hir2, and Hir3 proteins, has recently been shown to participate in replication-independent nucleosome assembly, and a similar pathway may suffice in yeast deficient in both the CAF-1 and Asf1 pathways for chromatin assembly (21).
Disruption of CAF-1 in yeast results in gene silencing defects at telomeres and at the silent mating type loci, and these phenotypes are enhanced when Asf1 is deleted (14, 15, 18). Similarly, overexpression of a truncated version of CAF-1 leads to expression of a silenced transgene in human cells and developmental defects in Xenopus embryos (22, 23). Thus, the loss or inhibition of CAF-1 can affect the assembly of chromatin structures that repress transcription in both yeast and humans.
Because CAF-1 is tightly linked to replication foci in vivo and because human chromatin is significantly more complex than yeast chromatin, we speculated that CAF-1 might play an important role in human cell proliferation. The function of human CAF-1 was investigated by small interfering RNA (siRNA)-mediated knockdown of the complex. In contrast to yeast, human CAF-1 was required for efficient progression through S-phase and the loss of CAF-1 activated a specific checkpoint. Furthermore, loss of CAF-1 completely eliminated any replication-coupled chromatin assembly activity in cell extracts, indicating that this protein is the predominant chromatin assembly machine at the replication fork. These results demonstrate that CAF-1 is required for efficient replication fork progression and that the S-phase checkpoint machinery monitors chromatin assembly.
Materials and Methods
Cell Culture and Transfection. RKO and HeLa cells were maintained in standard media. Transfection of RKO and HeLa cells was done by using Oligofectamine (Invitrogen) using a 200 or 100 nM oligo concentration, respectively. Two rounds of transfection at 0 and 24 h were done for all experiments. siRNA oligos were from Dharmacon and the targeting sequences of each were control GL3 (directed against firefly luciferase): CUUACGCUGAGUACUUCGAdTdT; 150-1, AGGGGAAAGCCGAUGACAUdTdT; and 150-2, CUGUCAUGUGGGUUCUGACdTdT.
Flow Cytometric Analysis. For cell cycle flow cytometric analysis, cells were collected by trypsinization, washed, fixed in cold methanol, washed, and stained in 20 μg/ml propidium iodide (PI) plus 10 μg/ml RNase. Cells were analyzed on a Coulter Epics Elite instrument. For BrdUrd/PI flow cytometric analysis, cells were collected, fixed in methanol, and stained with FITC conjugated anti-BrdUrd (Becton Dickinson) and propidium iodide (for details, see Supporting Text, which is published as supporting information on the PNAS web site, www.pnas.org).
Western Blots and Immunofluorescence. Total cell extracts were separated on SDS/10% PAGE gels transferred to nitrocellulose and processed for Western analysis. CAF-1 p150 was detected with the SS1 or SS48 monoclonal antibodies, CAF-1 p60 was detected with the SS53 antibody, and PCNA was detected with the PC10 antibody. Anti-Chk1, anti-Chk1 phospho-S317, anti-Chk2 phospho-T68, anti-p53 phospho-S15 (Cell Signaling Technologies), anti-p53 (CM1, NovoCastra), and anti-p21/CIP1/WAF1 (BD Transduction Laboratories) were also used. Details of antibody dilutions can be found in Supporting Text.
For immunofluorescence analysis, cells were plated on coverslips 16 h before transfection. After transfection, cells were fixed in 2% paraformaldehyde in PBS and processed for immunofluorescence (see Supporting Text for detailed protocols). To visualize chromatin bound PCNA, cells were preextracted with Triton X-100 in CSK buffer (13). Primary antibodies used were anti-CAF-1 p150 SS1, anti-PCNA PC10, anti-p53 phospho-S15, anti-Chk1 phospho-S317, and Alexa Fluor 594-conjugated anti-BrdUrd (Molecular Probes). Secondary antibodies were goat Texas Red anti-rabbit or FITC anti-mouse (The Jackson Laboratory). Cells were visualized on a Zeiss Axioplan or Axiophot microscope using ×40 or ×63 oil-immersion objectives. Images were processed by using OPENLAB v. 3.1.1 software and transferred to photoshop (version 6.0; Adobe Systems, Mountain View, CA) for assembly.
In Vitro Chromatin Assembly Assays. HeLa cell extracts were prepared from two plates of siRNA-transfected cells at 48 h, by using an extraction protocol (24) in which cytoplasmic cell extracts (designated the S100 fraction) and nuclei were prepared by hypotonic lysis and mechanical disruption of cells through a 25G ½ needle. Nuclear extracts containing CAF-1 were prepared by high salt extraction of nuclear pellets (see Supporting Text). Chromatin assembly assays were performed as described (9). In the one-step assay, 10, 20, and 30 μg of nuclear extract was used as a source of CAF-1. For the two-step assay, 20 fmol of in vitro-replicated DNA purified by filtration through a spun column was assembled in the presence or absence of 40 fmol of recombinant CAF-1 and 25 or 50 μg of S100 extract as a source of histone H3/H4 and other unidentified factors necessary for assembly. Exogenous topoisomerase I (1 unit per reaction, Takara Shuzo, Kyoto), topoisomerase II (100 ng), and histone H2A/B dimers (280 ng), which were limiting in the reaction, were also added. Recombinant CAF-1 and simian virus 40 (SV40) T antigen were from baculovirus infected Sf9 cells (9), topoisomerase II was from calf thymus (25), and H2A/B dimers (26) were from human cells.
Results
CAF-1 Is Required for Normal S-Phase Progression. To examine the function of CAF-1 in human cells, two siRNAs against the mRNA encoding the p150 subunit of the complex were designed (27, 28). Transfection of either of these oligos, but not a nonspecific control oligo, resulted in dramatic knockdown of CAF-1 in RKO cells. By transfecting twice at 24-h intervals, significant knockdown was achieved in >95% of cells by 48 h after transfection (Fig. 1A and data not shown). A concomitant decrease in the p60 protein of the CAF-1 complex was also detected by Western blot analysis. Because immunodepletion experiments indicate that the two largest subunits of CAF-1 in 293 nuclear extracts exist almost entirely in a complex (data not shown), the synchronous decrease of the p60 subunit probably reflects increased turnover of the free subunit. Therefore, knockdown of p150 significantly depletes the levels of CAF-1 activity in RKO cells. In contrast, the levels of PCNA, a CAF-1-associated protein (9), were not affected (Fig. 1 A).
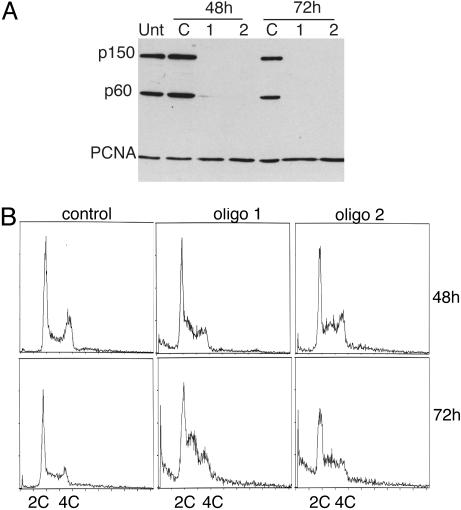
RNAi of CAF-1 induces the accumulation of cells in S-phase (A) Western blot analysis of total cell extract of cells treated with control oligo or with two different oligos targeting CAF-1 p150. Equal amounts of cell extract were loaded and probed with antibodies against CAF-1 p150, CAF-1 p60, and PCNA. Note that the bulk of p60 is depleted in p150 knockdown cells. (B) Cell cycle analysis of control and CAF-1-depleted cells at 48 and 72 h after transfection. DNA content was measured by flow cytometry.
The cell cycle distribution of cells lacking CAF-1 was compared with cells treated with control siRNA. RKO cells lacking CAF-1 accumulated with an increased S-phase DNA content by 48 h and further increased by 72 h (Fig. 1B). A population of cells with sub-2C DNA content also became apparent at 72 h and may represent an early apoptotic population. Similar accumulation of cells in S-phase was seen with both HeLa and HCT116 cells (data not shown). To further examine the nature of the defect in S-phase progression, cells were pulsed for either 10 min or 24 h with BrdUrd and immunostained for BrdUrd and CAF-1. Cells exhibited reduced or no staining for BrdUrd after a 10-min pulse and greatly reduced staining compared with cells that contained CAF-1 after a 24-h label, indicating that DNA replication had slowed but was not completely inhibited (Fig. 2A). Examining BrdUrd incorporation in cells with S-phase DNA content by using two-dimensional flow cytometric analysis confirmed this result. As before, knockdown of p150 resulted in an increase in the amount of cells in S-phase by 48 h, but the cells incorporated BrdUrd poorly as evidenced by the spread of the arc of S-phase cells in CAF-1-depleted cells compared with control cells, indicating a defect in DNA replication (Fig. 2B). The defect in BrdUrd incorporation was more severe at 72 h. Pretreatment of RKO cells with 2 mM hydroxyurea (HU) for 1 h blocked all BrdUrd incorporation, demonstrating that the nonzero amount of incorporation in CAF-1-depleted cells was not caused by background staining (data not shown). We conclude that loss of CAF-1 resulted in slow DNA replication and accumulation of cells in S-phase.
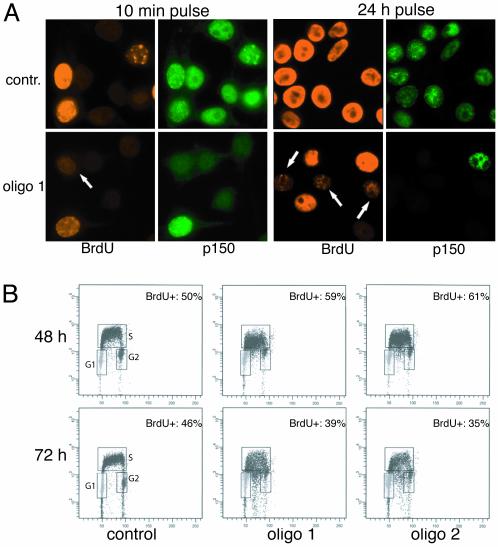
Loss of CAF-1 leads to slow S-phase progression. (A) CAF-1 knockdown cells pulsed for 10 min (48 h after transfection) or 24 h (36–60 h after transfection) with 40 μM BrdUrd and stained with antibodies specific for BrdUrd and p150. Control cells display high levels of BrdUrd incorporation, whereas CAF-1-depleted cells do not incorporate BrdUrd efficiently (arrows). (B) Two-dimensional flow cytometry analysis monitoring BrdUrd incorporation and DNA content. Cells were pulsed with BrdUrd for 10 min, fixed, and stained with FITC-conjugated anti-BrdUrd antibody and propidium iodide to stain the DNA. BrdUrd intensity is plotted on a log scale on the y axis, and DNA content is plotted on the x axis. G1, G2, and S-phase populations are gated in each sample, and the numbers in the upper right indicate the percentage of BrdUrd-positive cells in each sample.
CAF-1-Negative Cells Accumulate in Early and Mid S-Phase. CAF-1 localizes to DNA replication foci throughout S-phase, and loss of CAF-1 might affect replication by interfering with the recruitment of replication factors (9). CAF-1 also functions in the replication and maturation of heterochromatin because it localizes at sites of heterochromatin replication in late S-phase (12) and binds to and recruits the heterochromatic HP-1 proteins to DNA in vitro (29), and loss of CAF-1 leads to silencing defects in yeast and human cells (14, 15, 22). Thus, loss of CAF-1 might disproportionately affect replication of heterochromatin, leading to an altered distribution of replication foci in cells. To examine this possibility, cells were stained with PCNA after RNA interference (RNAi) treatment and the distribution of replication foci was determined. The stage of S-phase can be distinguished by characteristic patterns of PCNA staining that cells exhibit in early, mid, and late S-phase (30–32) (Fig. 3A). Late S-phase patterns (4 and 5) have less numerous, large foci throughout the nucleoplasm and at the nuclear periphery. Cells were stained for chromatin-bound PCNA, and replication foci in control or CAF-1 RNAi-treated cells were examined. A substantial decrease in the number of cells displaying late S-phase patterns in CAF-1-deficient cells (Fig. 3 B and C) and a corresponding increase in both early and mid S-phase patterns were observed. No abnormal replication patterns were noted, suggesting that replication factories were assembled correctly and that the replication defect occurred downstream of PCNA recruitment. The lower amount of late S-phase cells may be due to difficulty replicating heterochromatin, but could also be due to a more global defect in S-phase progression.
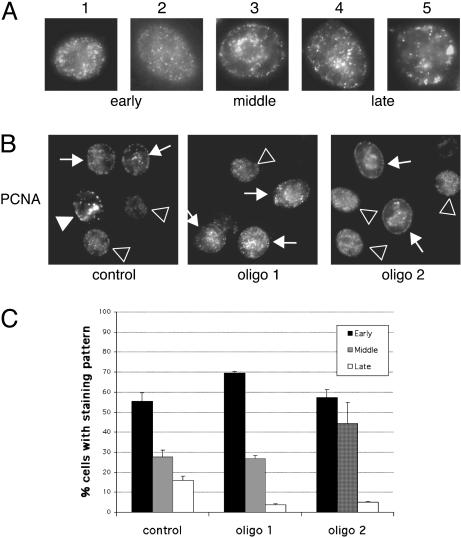
CAF-1-depleted cells assemble normal replication foci and accumulate in early to mid S-phase. (A) Cells were stained for chromatin-bound PCNA and scored for replication patterns. Examples of early, mid, and late S-phase patterns are provided. (B) Late S-phase patterns are easily seen in control cells but are found less frequently in cells treated with either CAF-1-targeted siRNA. Arrows indicate examples of early, middle, and late replication sites (, early S-phase patterns; →, middle S-phase patterns;
, late S-phase patterns). (C) Distribution of S-phase cells displaying early, mid, or late S-phase replication patterns 48 h after transfection. Three hundred S-phase cells were counted per sample, and the results are the mean from two independent experiments.
Loss of CAF-1 Activates Checkpoints. Reduced rates of DNA replication and slow or stalled replication forks can cause activation of an intra-S-phase checkpoint (33). The checkpoint kinase ATR activates the downstream kinase Chk1 by phosphorylation on S317 and S345 preferentially in response to replication fork stress or the presence of bulky adducts in DNA (34). In contrast, ATM is activated preferentially in response to double-strand DNA breaks in human cells and activates the Chk2 kinase by phosphorylation of T68 (35–37). Both ATM and ATR phosphorylate p53 on S15, leading to increased transcriptional transactivation potential of this protein (38). To test whether loss of CAF-1-activated checkpoint pathways in RKO cells, phospho-specific antibodies against Chk1, Chk2, and p53 were used. Phosphorylation of S317 of Chk1 was detected in cells lacking CAF-1, but phosphorylation of Chk2 was not induced (Fig. 4A). p53 levels were induced by 48 h, and the induced protein was phosphorylated on S15 (Fig. 4A). Accumulation of the p53 transcriptional target, p21 was also detected, indicating that p53 was active in these cells (Fig. 4A). The activation of Chk1 and not Chk2 implied that the ATR branch of the DNA damage response pathway had been activated, consistent with CAF-1 deficiency causing a defect in ongoing DNA replication.
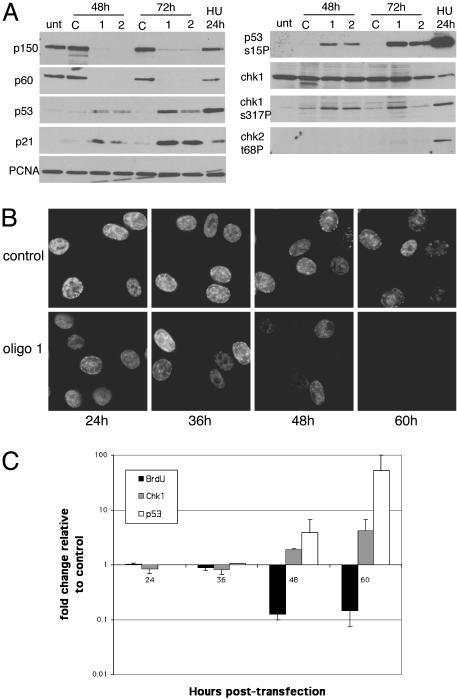
Checkpoint activation in CAF-1-depleted cells. (A) Western analysis of total cell lysate after CAF-1 RNAi to monitor checkpoint activation by using phospho-specific antibodies to p53 S15, Chk1 S317, and Chk2 T68. Overall Chk2 levels do not change (data not shown). Extract of cells treated with hydroxyurea (20 mM) for 24 h, which leads to phosphorylation of all three proteins, was included as a positive control. (B) Time course of checkpoint activation and BrdUrd incorporation in control and CAF-1-depleted cells. Three coverslips were seeded per well and transfected according to the standard protocol. Cells were collected at 24, 36, 48, and 60 h after transfection, pulsed for 10 min with BrdUrd, and stained with phospho-specific antibodies against S15 of p53, S317 of Chk1, or BrdUrd. Cells in the 24-h time point had only been transfected once. Some loss in BrdUrd incorporation was seen by 36 h in comparison to control, and BrdUrd incorporation drops dramatically at 48 h after transfection. (C) Plot comparing checkpoint activation and BrdUrd incorporation in individual CAF-1 knockdown cells normalized to control transfected cells. Three hundred cells were counted per time point for each antibody for control and CAF-1 targeting oligo 1, and the results shown are the mean for two experiments. All cells displaying checkpoint activation above background and only brightly BrdUrd-labeled cells were scored as positive. Significant checkpoint activation was not seen until 48 h after transfection.
The slow replication seen in CAF-1 knockdown cells might be caused by a primary defect in DNA replication or by the activation of the checkpoint machinery. To analyze this, the onset of the DNA replication defect was compared with checkpoint activation by monitoring p53 and Chk1 phosphorylation and BrdUrd incorporation by immunofluorescence analysis between 24 and 60 h after transfection. A decrease BrdUrd incorporation was detected by 36 h, and DNA replication appeared largely inhibited by 60 h after transfection, although very dim replication foci were still detectable (Fig. 4 B and C). However, significant phosphorylation of p53 was seen only by 48 h and accumulated in more cells by 60 h (Fig. 4C and Fig. 6, which is published as supporting information on the PNAS web site). p53 phosphorylation increased ≈50-fold by 60 h as compared with control cells (Fig. 4C), whereas Chk1 phosphorylation increased 4-fold relative to control, probably because there was some activation even in control cells (Fig. 4A, lane C, 48 h). These data indicate that activation of p53 and Chk1 occur concomitant with or slightly after the onset of the replication defect.
CAF-1 Is Necessary and Sufficient for Replication-Coupled Chromatin Assembly. CAF-1 purified from human cell nuclei assembled chromatin in the presence of an S100 extract that supported SV40 DNA replication (5, 7). Because CAF-1 is not essential in yeast, it was possible that other factors present in the nuclear extract could substitute for CAF-1 in the DNA replication-coupled chromatin assembly reaction. Therefore, a nuclear extract from cells transfected with the CAF-1 siRNA was tested for chromatin assembly activity. Small-scale nuclear and S100 extracts were prepared from HeLa cells 48 h after they had been transfected with control or p150 targeting siRNAs. CAF-1 partitioned exclusively to the nuclear extract, whereas PCNA was found in both fractions (Fig. 5A). Extract from cells transfected with control oligonucleotide was capable of supporting chromatin assembly preferentially on replicating templates as measured by the increased supercoiling of the replicated template in the presence of CAF-1, whereas extract from p150 knockdown cells was unable to support chromatin assembly (Fig. 5B). This was due explicitly to the defect in CAF-1 protein because the addition of purified recombinant CAF-1 restored assembly activity to normal levels. This demonstrated that CAF-1 is essential for DNA-replication-coupled chromatin assembly in human cell extracts.
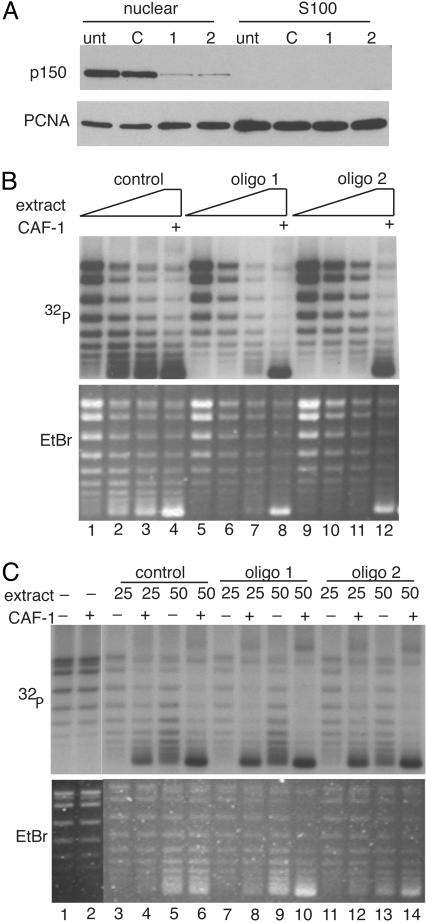
Replication coupled chromatin assembly activity depends on CAF-1. (A) Western blot analysis of CAF-1 in HeLa nuclear and S100 fractions after RNAi. (B) One-step chromatin assembly assays to monitor the ability of control and CAF-1-depleted nuclear extracts to assemble replicating SV40 DNA into nucleosomes. pSV011 DNA containing the SV40 origin was replicated in a replication mix containing 32P-dATP to label replicated DNA and was assembled into chromatin with nuclear extract or recombinant CAF-1. DNA packaged into chromatin accumulated negative supercoils and runs with enhanced mobility relative to unassembled DNA [compare lane 1 (low CAF-1) to lanes 2–4 (sufficient CAF-1)]. The bulk of unreplicated DNA visualized by ethidium bromide staining (Lower) remained relaxed, indicating that the CAF-1 preferentially loaded nucleosomes onto replicating DNA. When CAF-1 was depleted by RNAi (lanes 5–7 and 9–11), assembly did not occur. Addition of recombinant CAF-1 restored assembly activity in these extracts (lanes 8 and 12). Nuclear extract (10–30 μg) was titrated into each set of reactions. (C) Two-step chromatin assembly assays demonstrate that the S100 from CAF-1 knockdown cells contain all accessory factors (including histones H3 and H4) required to assemble chromatin. SV40 DNA was replicated in a S100 replication extract, purified away from proteins by gel filtration and incubated with S100 from control or p150-depleted extracts in the presence or absence of CAF-1. Twenty-five and 50 μg of extract were used in each reaction. Under these conditions, assembly into chromatin required CAF-1 (compare lanes 3 and 4, –/+ CAF-1) and S100 (compare lanes 2 and 4). Unreplicated DNA seen in the ethidium stain (Lower) was not assembled into chromatin and remained largely relaxed.
Ongoing histone synthesis during S-phase is required for efficient S-phase progression and ectopic expression of the Hir proteins, which repress the expression of histone genes and results in a replication defect similar to that seen with loss of CAF-1 (17, 39, 40) Therefore, one explanation for accumulation of cells in S-phase could be that loss of CAF-1 indirectly affects levels of unincorporated and newly synthesized histones. A pool of these histones is present in the cytoplasm of cells and the H3 and H4 present in this fraction is essential for the postreplicative chromatin assembly that is mediated by CAF-1 (9, 41). To determine whether histones H3 and H4 were limiting in CAF-1-depleted extracts, a two-step chromatin assembly assay was used in which SV40 DNA was first replicated and radiolabeled in vitro in a standard DNA replication reaction, then the resulting DNA–protein complexes were purified away from the other protein components required for DNA replication by a rapid gel filtration step. This “marked” DNA was subsequently assembled into chromatin in a second reaction dependent on CAF-1 (9, 41). CAF-1 was able to assemble chromatin on this replicated DNA because PCNA remains topologically linked to the DNA after replication and can recruit CAF-1. The subsequent chromatin assembly reaction required components supplied by the S100 extract, including histones H3 and H4 (41). There was no defect in the ability of the S100 extract prepared from cells lacking CAF-1 to support the ability of recombinant CAF-1 to assemble chromatin in this assay (Fig. 5C). Furthermore, the total levels of histone H3 were unaffected in CAF-1 knockdown cells (data not shown). These results suggest that depletion of CAF-1 is unlikely to have effects on histone synthesis or stability.
Discussion
Chromatin assembly and DNA replication are tightly coupled in eukaryotic cells. Nucleosomes are only transiently disrupted ahead of the replication fork and are rapidly deposited and positioned behind the replication fork (1, 3, 4, 42, 43). The chromatin assembly protein CAF-1 has long been hypothesized to couple chromatin assembly to DNA replication in eukaryotic cells, but this hypothesis was thrown into question when loss of CAF-1 led to only modest phenotypes in yeast (14, 15). In this report, we show that CAF-1 is the dominant replication-coupled chromatin assembly machine in human cells and that loss of CAF-1 leads to defects in DNA replication and S-phase progression, suggesting that, in human cells, CAF-1 couples chromatin assembly to DNA replication and is essential for cell proliferation.
Several studies that reported overexpression of dominant-negative versions of CAF-1 have also concluded that CAF-1 is essential for cell proliferation. Overexpression of human CAF-1 p150 in Xenopus inhibited proper embryo development by interfering with cell division (23). Similarly, transient overexpression of versions of CAF-1 that were unable to bind PCNA led to an S-phase arrest in human cells (44). This study also showed that expression of dominant-negative CAF-1 induced an intra-S-phase checkpoint and that dominant negative ATR could abrogate the S-phase arrest only in cell lines also deficient for ATM, consistent with a role for both kinases in mediating dominant negative CAF-1 induced arrest. However, in both studies, cryptic functions of the dominant-negative constructs could have played a role in inducing the observed proliferation defects. Our experiments, however, using a distinct RNAi methodology to eliminate CAF-1 function are consistent with the previous studies and demonstrate a fundamental role for CAF-1 in S-phase progression. This was unlikely to be caused by depletion of cytoplasmic pools of H3 and H4 or other cellular factors required for DNA replication and chromatin assembly in vitro.
What is the role of CAF-1 in S-phase progression? Replication forks are assembled normally, because normal S-phase staining patterns of PCNA in CAF-1 depleted cells were seen and other replication proteins such as RPA, RFC, and DNA polymerase-δ were still recruited to the chromatin (data not shown). However, cells deficient in CAF-1 accumulated in early and mid-S-phase, demonstrating that progression through S-phase was inhibited. This could be caused by either stalling of replication forks in early S-phase, the activation of the intra-S-phase checkpoint in response to chromatin defects, or a specific defect in heterochromatin replication. Heterochromatin generally replicates late in S-phase, and a partial explanation of the S-phase progression defect might be that CAF-1 plays a particular and distinct role in heterochromatin replication. Proteins such as HP1 that mark heterochromatin have the potential to crosslink chromatin fibers into a compact and potentially less accessible structure through self-association (45, 46). Because CAF-1 binds the HP1 chromoshadow domain, it may play a role in destabilizing heterochromatic structures during replication, allowing the replication machinery to progress efficiently through heterochromatin, thereby explaining why the loss of CAF-1 could lead to an accumulation of early and mid-S-phase cells.
CAF-1, however, localizes to replication foci throughout S-phase and is intimately tied to all replication forks because of its interaction with PCNA. This observation suggests that CAF-1 probably plays a more general role in DNA replication that could include a role in handling nucleosomes both ahead of and behind the replication fork. Analysis of replicating SV40 minichromosomes has shown that nucleosomes are transiently disrupted just ahead of the replication fork and assemble quickly behind the replication fork (2). H2A and H2B are exchanged during replication, but H3 and H4 tetramers remain associated with DNA (1). Thus, an H3/H4 chaperone associated with the replication fork such as CAF-1 or Asf1 might be involved in the transient destabilization of nucleosomes required for replication fork passage and interfering with this function could interfere with progression of the replication fork. Alternatively, loss of CAF-1 might affect replication more indirectly by altering DNA topology. Nucleosomes contribute to the topological state of the genome because they introduce negative supercoils into the DNA. The overall superhelical density on a given piece of DNA might be significantly reduced in the absence of CAF-1 mediated chromatin assembly. Negative supercoils can contribute to local DNA unwinding, and disruption of nucleosomes may be important in replication fork progression. In support of a role for DNA topology in mammalian replication, the protein DEK-1 has recently been shown to reduce the superhelical density of nucleosomal DNA without stripping the histones (47) and incubation of SV40 chromosomes with DEK-1 significantly reduced the efficiency of DNA replication in vitro (48).
Stalled replication forks are well known to activate the intra-S-phase checkpoint and changes in DNA topology or the exposure of excessive amounts of naked DNA may be novel lesions monitored by the checkpoint machinery. We detected activation of Chk1, but not Chk2, and activation of p53 and its transcriptional target p21 in CAF-1 knockdown cells. Activated Chk1 promotes the degradation of Cdc25A, thus interfering with CDK activity and leading to slow S-phase progression (49). Additionally, up-regulation of p21 through p53 might play a role in the replication arrest, either because p21 associates with PCNA in response to DNA damage and inhibits DNA replication in vitro or hinders the progression to later stages of S-phase through its CDK inhibitory action (50–52).
Our data suggest that the onset of the replication defect precedes or occurs simultaneously with checkpoint activation, although low levels of checkpoint activity below the threshold of detection could be present at earlier time points. Thus, checkpoint activation may be caused by the presence of stalled replication forks induced by the loss of CAF-1. However, CAF-1 might also have a more intimate connection with the checkpoint machinery. In yeast, the Asf1 chromatin assembly protein interacts with Rad53, a homolog of human Chk2 (53, 54). In response to DNA damage, Asf1 is released from Rad53 and is free to bind H3 and H4 and promote chromatin assembly. Overexpression of Asf1 suppresses the hydroxyurea sensitivity of rad53–21 mutants, tying Asf1 to the checkpoint and replication functions of Rad53 (53). CAF-1 and Asf1 also function in the maintenance of genomic integrity in yeast, because loss of these genes in conjunction with checkpoint genes such as tel1, chk1, and dpb11 leads to an increase in gross chromosomal rearrangements and in the mutation rate (55). In human cells, Chk1, which is thought to monitor replication in a similar way to yeast Rad53, activates Tlk kinases that, in turn, target Asf1 and potentially other chromatin deposition factors in response to DNA damage (56). Thus, CAF-1 and Asf1 may directly signal the state of chromatin to the checkpoint machinery and loss of these proteins might lead in to checkpoint activation (13, 15).
In mammalian cells, histone H3 comes in two major forms, histone H3.1 and histone H3.3. The former is loaded onto replicated DNA, whereas H3.3 replaces H3.1 in transcribed regions of the genome (57). Interestingly, Saccharomyces cerevisiae only has the H3.3 version of histone H3, probably because most of the genome is transcribed (58). Therefore, we suggest that CAF-1 is not essential in yeast because the discrimination between the two related forms of chromatin does not exist. In human cells, however, CAF-1 would predominantly deposit and maintain histone H3.1 type nucleosomes onto the replicating DNA, and is therefore essential.
Acknowledgments
We thank Nouria Hernandez, Arne Stenlund, and members of the Stillman laboratory for helpful discussions throughout this work, and Andrei Chabes and Viola Ellison for critical reading of the manuscript. This work was supported by National Institutes of Health Grant CA13106. M.H. was supported by National Cancer Institute Training Grant 5T32 CA09311.
Notes
Abbreviations: CAF-1, chromatin assembly factor 1; PCNA, proliferating cell nuclear antigen; siRNA, small interfering RNA; Hir, histone regulating.
References
Articles from Proceedings of the National Academy of Sciences of the United States of America are provided here courtesy of National Academy of Sciences
Full text links
Read article at publisher's site: https://doi.org/10.1073/pnas.1635158100
Read article for free, from open access legal sources, via Unpaywall:
https://www.pnas.org/doi/pdf/10.1073/pnas.1635158100
Citations & impact
Impact metrics
Citations of article over time
Alternative metrics
Article citations
Replication-coupled inheritance of chromatin states.
Cell Insight, 3(6):100195, 23 Aug 2024
Cited by: 0 articles | PMID: 39391004 | PMCID: PMC11462216
Review Free full text in Europe PMC
RebL1 is required for macronuclear structure stability and gametogenesis in Tetrahymena thermophila.
Mar Life Sci Technol, 6(2):183-197, 26 Mar 2024
Cited by: 0 articles | PMID: 38827131 | PMCID: PMC11136921
A novel biomarker associated with EBV infection improves response prediction of immunotherapy in gastric cancer.
J Transl Med, 22(1):90, 22 Jan 2024
Cited by: 0 articles | PMID: 38254099 | PMCID: PMC10804498
Spatiotemporal kinetics of CAF-1-dependent chromatin maturation ensures transcription fidelity during S-phase.
Genome Res, 33(12):2108-2118, 27 Dec 2023
Cited by: 2 articles | PMID: 38081658 | PMCID: PMC10760526
An oncogene regulating chromatin favors response to immunotherapy: Oncogene CHAF1A and immunotherapy outcomes.
Oncoimmunology, 13(1):2303195, 09 Jan 2024
Cited by: 0 articles | PMID: 38235318 | PMCID: PMC10793680
Go to all (172) article citations
Other citations
Wikipedia
Data
Data behind the article
This data has been text mined from the article, or deposited into data resources.
BioStudies: supplemental material and supporting data
Similar Articles
To arrive at the top five similar articles we use a word-weighted algorithm to compare words from the Title and Abstract of each citation.
Essential role of chromatin assembly factor-1-mediated rapid nucleosome assembly for DNA replication and cell division in vertebrate cells.
Mol Biol Cell, 18(1):129-141, 25 Oct 2006
Cited by: 64 articles | PMID: 17065558 | PMCID: PMC1751324
An analysis of CAF-1-interacting proteins reveals dynamic and direct interactions with the KU complex and 14-3-3 proteins.
J Biol Chem, 286(12):10876-10887, 05 Jan 2011
Cited by: 19 articles | PMID: 21209461 | PMCID: PMC3060538
Checkpoint functions are required for normal S-phase progression in Saccharomyces cerevisiae RCAF- and CAF-I-defective mutants.
Proc Natl Acad Sci U S A, 103(10):3710-3715, 24 Feb 2006
Cited by: 21 articles | PMID: 16501045 | PMCID: PMC1533778
Chromatin assembly during DNA replication in somatic cells.
Eur J Biochem, 263(1):1-5, 01 Jul 1999
Cited by: 52 articles | PMID: 10429179
Review
Funding
Funders who supported this work.
NCI NIH HHS (4)
Grant ID: CA 13106
Grant ID: 5T32 CA 09311
Grant ID: P01 CA013106
Grant ID: T32 CA009311