Abstract
Free full text

Prevention and Cure of Systemic Escherichia coli K1 Infection by Modification of the Bacterial Phenotype
Abstract
Escherichia coli is a common cause of meningitis and sepsis in the newborn infant, and the large majority of isolates from these infections produce a polysialic acid (PSA) capsular polysaccharide, the K1 antigen, that protects the bacterial cell from immune attack. We determined whether a capsule-depolymerizing enzyme, by removing this protective barrier, could alter the outcome of systemic infection in an animal model. Bacteriophage-derived endosialidase E (endoE) selectively degrades the PSA capsule on the surface of E. coli K1 strains. Intraperitoneal administration of small quantities of recombinant endoE (20 μg) to 3-day-old rats, colonized with a virulent strain of K1, prevented bacteremia and death from systemic infection. The enzyme had no effect on the viability of E. coli strains but sensitized strains expressing PSA to killing by the complement system. This study demonstrates the potential therapeutic efficacy of agents that cure infections by modification of the bacterial phenotype rather than by killing or inhibition of growth of the pathogen.
Capsulate bacteria cause a range of severe systemic infections in humans, including septicemia, meningitis, pneumonia, osteomyelitis, septic arthritis, and pyelonephritis (3). There is firm evidence linking their invasive potential to the expression of the polysaccharide capsule at the cell surface; this relationship is particularly strong with gram-negative bacteria causing sepsis and meningitis in the newborn infant and in adolescents (13). The rates of mortality and morbidity associated with these infections remain high in spite of recent advances in intensive care management and the introduction of newer, more potent antibiotics (21). There is widespread recognition (21, 31) that novel therapeutic strategies against the major pathogens in meningitis, sepsis, and other life-threatening systemic infections are urgently needed, both to reduce the severity of the disease and to counteract the emergence of further drug resistance, which compromises a successful therapeutic outcome. Bacterial meningitis and sepsis in the neonate are caused predominantly by strains of Escherichia coli, Streptococcus agalactiae, and Listeria monocytogenes (9, 21). Approximately 80 to 85% of these neuropathogenic E. coli express the K1 capsule (12), a homopolymer of α-2,8-linked N-acetylneuraminic acid that is structurally identical to the meningococcal B capsular polysaccharide (30). Expression of polysialic acid (PSA) capsule appears to be necessary in order for K1 strains to cause infection, since mutants lacking PSA are not virulent (3, 19). The presence of PSA on the bacterial surface suppresses activation of the alternative pathway of complement (19), reduces uptake by phagocytes (11), and affords protection against complement-mediated bacterial killing (27). It also enables the bacteria to avoid lysosomal degradation in microvascular endothelial cells when crossing the blood-brain barrier (10). The contribution of PSA to overall virulence is probably enhanced by its structural identity to PSA expressed on neuronal cells during embryonic development (30).
We are investigating the potential of modification of the bacterial phenotype as an alternative approach to the treatment of bacterial infections (28). Agents that do not kill pathogenic bacteria but modify them to produce a “less-fit” phenotype, unable to survive at the site of infection, may be viable alternatives to conventional antibiotics that invariably select for the drug-resistant genotype. Compromising the expression of PSA at the surface of E. coli K1 strains during the course of infection would allow mediators of the host's defenses to interact with underlying structures and eliminate the bacteria. We have identified, characterized, cloned, sequenced, and expressed in high yield in bacteria (15, 17, 29) an enzyme, endosialidase E (endoE), carried by a bacteriophage infecting E. coli K1 strains, that has an absolute specificity for α-2,8 sialic acid linkages and rapidly hydrolyzes the E. coli K1 capsular polysaccharide (29). We investigated the capacity of endoE to modulate the virulence of pathogenic E. coli K1 strains in vivo and in vitro.
MATERIALS AND METHODS
Bacterial strains.
E. coli LP1674 (serotype O7:K1) and LP1395 (O18:K?) were isolated from patients with urinary tract infections (25). K1 strains A53 (O18ac:K1:H7) and A192 (O18:K1) were isolated from patients with, respectively, neonatal meningitis and septicemia (1) and were obtained from the Deutsche Sammlung von Mikroorganismen und Zellkulturen GmbH, Braunschweig, Germany, as DSM strains 10723 and 10719. E. coli Bos12 belongs to capsule serogroup K92 and was obtained from the American Type Culture Collection as ATCC 35860. E. coli A192PP was obtained by serial passage in neonatal rats; a colony isolated from the blood culture of an animal colonized by E. coli A192 was passaged once more in the same way, bacteria were isolated from the blood, and a colony was designated A192PP.
Production of His6-endoE.
The endoE gene open reading frame was amplified by PCR with cloned full-length DNA as a template and ligated into plasmid pQE30 (Qiagen, Ltd., Crawley, United Kingdom) by standard cloning technology to introduce a histidine tag at the N terminus of the recombinant protein; since we have found that the translated gene product undergoes proteolytic cleavage at a site close to the C terminus (15), the location of this tag is critical for subsequent purification of the fusion product. The plasmid was introduced into E. coli BL21(DE3), and the vector was grown in 2xTY broth (16 g of Bacto tryptone, 10 g of yeast extract, and 5 g of NaCl per liter) in controlled batch fermentation culture by using an Electrolab 300 Series Microprocessor-steered 10-liter fermentor with IPTG (isopropyl-β-d-thiogalactopyranoside) at optical density at 600 nm (OD600) of 0.3 for up to 6 h. Recombinant protein was purified by using immobilized metal (Ni) affinity chromatography of freeze-thawed sonicated bacterial lysates to yield up to 6 mg of His6-tagged recombinant endoE/liter. Sodium dodecyl sulfate-polyacrylamide gel electrophoresis (SDS-PAGE) and immunoblotting indicated a very high purity of product, with no proteins detectable other than the 76-kDa recombinant endoE fusion product. Batches of His6-tagged recombinant enzyme were shown to be catalytically active by using the thiobarbiturate assay for release of N-acetylneuraminic acid from K1 polysaccharide extracted from E. coli LP1674 (15, 17). There was no loss of enzyme function due to storage of solutions at 4°C for periods of up to 1 year. The hydrolytic capacity of endoE was retained in the presence of normal human serum, rat serum, and human cerebrospinal fluid.
Complement sensitivity assay.
Pooled normal human serum was obtained from the UK Blood Transfusion Service and stored at −80°C for up to 6 months; bactericidal capacity, as judged by the capacity to kill a panel of rough strains of E. coli, did not diminish during storage. No anti-K1 antibodies were detected in an agar immunodiffusion assay. Heating (56°C, 30 min) removed all serum bactericidal activity.
Bacteria were grown to an OD578 of 0.5 in Mueller-Hinton broth with agitation at 37°C. Cells were recovered by centrifugation, washed with phosphate-buffered saline (PBS), and then resuspended in PBS to ensure 1 × 105 to 3 × 105 CFU/ml in the serum incubation mix. Cells were pretreated with His6-endoE (10 μg/ml) or PBS at 37°C for 15 min. Human serum was added to give a final concentration of 20% (vol/vol), followed by incubation at 37°C for up to 2 h. Samples (100 μl) were removed at various time intervals and diluted in PBS, and viable counts were performed by using the pour plate technique.
Detection of C3 cleavage products and C9 on bacterial membranes.
Bacteria were grown to an OD578 of 0.5 in Mueller-Hinton broth with agitation at 37°C; 25-ml aliquots were centrifuged, and bacterial cells washed once with PBS. Harvesting was performed at room temperature in order to avoid temperature shocking of bacterial cells. Bacteria were then suspended in 20 ml of prewarmed (37°C) PBS, and 5 ml of normal human serum was added, followed by rapid mixing. After incubation at 37°C, the reaction mixture was rapidly cooled in a CO2-methanol bath, washed once with PBS, and resuspended in 1.25 ml of 0.05 M HEPES (pH 7.2) containing 0.75 M sucrose and 50 μg of egg white lysozyme (Sigma)/ml. After 10 min at room temperature, plasmolysed cells were carefully pipetted into 30 ml of gently stirred water containing DNase I (Sigma) at room temperature. The cells generally underwent extensive lysis after a few seconds; the extent of lysis was monitored microscopically. When lysis was complete, membranes were collected by ultracentrifugation, and proteins were separated by SDS-PAGE. Proteins were transferred to nitrocellulose and the sheets probed with anti-C3 and anti-C9 polyclonal antibodies; bound antibodies were visualized by using rabbit anti-mouse immunoglobulin G monoclonal antibodies conjugated to horseradish peroxidase and enhanced chemiluminescence.
Induction of bacteremia in neonatal rats.
The model used for the induction of bacteremia was adapted from the neonatal rat model developed by Glode et al. (5) and Pluschke et al. (20). Litters were retained with the natural mothers after birth; each cage contained one litter. Two-to-three-day-old Wistar rats (normally about 10 pups per litter) were fed 20 μl of E. coli A192PP (ca. 2 × 106 to 6 × 106 bacteria at 37°C) by using an Eppendorf micropipette. Gastrointestinal colonization of rats was determined by culture on MacConkey agar of anal swabs or material obtained from the perianal area by using toothpicks. Samples were taken daily beginning 1 day after feeding of bacterial cultures. Bacteremia was detected by culture of blood samples on MacConkey agar taken daily by tail vein puncture by using a microlance or by cardiac puncture. Lactose-fermenting colonies were picked and examined for sensitivity to K1-specific bacteriophages (8). Animals were examined twice daily for clinical signs of systemic infection and weighed.
RESULTS
Administration of endoE to infected rats.
To determine whether recombinant endoE has therapeutic and/or prophylactic potential in E. coli K1 bacteremia and meningitis, we induced systemic infection by using a neonatal rat model adapted from earlier studies (5, 20). This noninvasive model mimics many of the features of human disease, and the experimental infection follows the natural route of spontaneous infection in neonates (21): colonization of the gut is followed by translocation of the bacteria from the gut to the mesenteric lymph nodes and invasion of the bloodstream and the meninges after extraintestinal multiplication (20). Cultures (20 μl) of K1 strain A192PP were fed to 2-to-3-day-old rat pups; this complement-resistant strain, isolated from a Dutch patient with septicemia (1), gave consistently high rates of intestinal colonization and bacteremia after serial passage in neonatal rats. A litter of, typically, 10 to 12 pups was used for each experimental evaluation. Intestinal colonization of all members of the litter occurred within 24 h of feeding of bacteria and persisted throughout the period of evaluation (Fig. (Fig.1A).1A). In untreated groups, E. coli K1 was detected in blood during the first 2 days after K1 feeding. Viable counts of E. coli K1 in blood were between 102 and 107 per ml. The number of bacteremic pups continued to increase over the course of the experiment, and by days 6 to 7 all animals had blood-borne infection. Death rates paralleled bacteremia, and experiments were terminated on days 7 to 8 (Fig. (Fig.1A).1A). In test groups, each pup received 20 μg of recombinant endoE by intraperitoneal injection on days 1, 2, 3, 4, and 5; care was taken to ensure minimal damage to the lining of the peritoneal cavity. As is evident from the typical experiment shown in Fig. Fig.1B,1B, endoE administration reduced the number of pups with bacteremia to zero, or close to zero. Animals with bacteremia were, typically, free of E. coli K1 bacteria in the blood within 24 h of administration of the first dose, and subsequent doses prevented the appearance of K1 in the blood compartment. In marked contrast to animals in the control groups, deaths occurred in only 0 to 10% of endoE-treated pups. endoE administration had no effect on the extent of intestinal colonization by E. coli A192PP (Fig. (Fig.1B).1B). The experiment shown in Fig. Fig.1B1B was repeated twice on further litters with the same outcome. Our data indicate that endoE has both therapeutic and prophylactic potential. Administration of a single 20-μg dose on day 1 confirmed that endoE prevents the death of K1-colonized pups (Fig. (Fig.2A);2A); death was always preceded by K1 bacteremia, and by day 7 all survivors examined were free of bacteremia. If pups were given a single endoE dose on day 3, the effect was less clear (Fig. (Fig.2B):2B): although more endoE-treated animals survived than untreated controls, 60 to 70% had died prior to termination of the experiments on day 7. Again, in these experiments, all survivors were free of bacteremia, and death was preceded by K1 bacteremia.
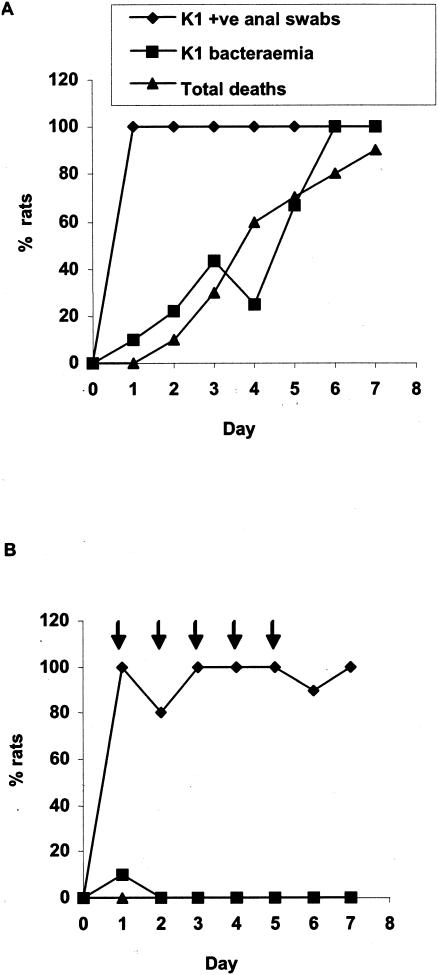
Effect of intraperitoneal administration of endoE on the incidence of bacteremia and death in E. coli K1-infected neonatal rats. All pups in a litter were fed E. coli A192PP on day 0. Animals in the control group received vehicle (A); 20 μg of endoE was administered to animals in the test group on days 1 to 5 (indicated by arrows) (B).
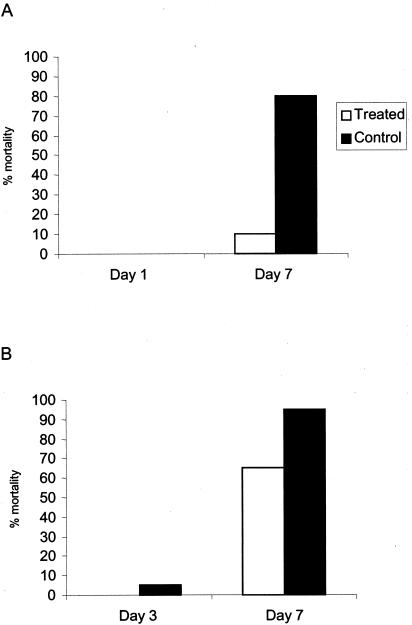
Effect of single intraperitoneal dose of endoE on survival of E. coli K1-infected neonatal rats. All pups in a litter were fed E. coli A192PP on day 0. Animals received 20 μg endoE on either day 1 (A) or day 3 (B). The data from two experiments were combined (total number of rat pups = 20). P < 0.05 for panel A, and 0.1 ≤ P ≤ 0.2 for panel B (Student’s t test).
Action of complement.
A likely explanation for the therapeutic effect of recombinant endoE in the neonatal rat model is the removal of bacterial surface PSA, allowing complement activation. The weight of available evidence indicates that PSA protects against the bactericidal action of complement by modulating the interaction of C3b with the inhibitor protein factor H, and it thus restricts the activation of the alternative pathway (26). Since anti-K1 antibodies are not normally formed in response to infection due to E. coli K1 strains, there is no classical pathway for the generation of membrane perturbing poly-C9 on the bacterial surface. To determine whether endoE could sensitize E. coli K1 strains to complement, we incubated mid-logarithmic-phase cultures of complement-resistant clinical isolates with 20% normal human serum (as a source of complement) in the presence or absence of small quantities (10 μg) of His6-tagged recombinant endoE. As shown in Fig. Fig.3A,3A, the passaged K1 strain A192PP was completely refractory to complement, and bacterial numbers increased over the 2-h incubation period. However, incubation with serum in the presence of the enzyme resulted in conversion to the complement-sensitive phenotype, with a >4-log reduction in bacterial numbers in the first hour. The enzyme had no effect on bacterial viability (Fig. (Fig.3A).3A). Essentially identical data were obtained with the complement-resistant K1 strains LP1674 and A53: the latter is a neuropathogenic K1 strain isolated from a case of newborn meningitis (1). Both of these strains were converted fully to complement-sensitive phenotype by endoE, which had no effect on their viability (data not shown). Interestingly, the E. coli K92 strain Bos12 (18) was also converted to the complement-sensitive phenotype after exposure to endoE (Fig. (Fig.3B).3B). This strain produces a PSA capsule containing alternating α-2,8 and α-2,9 sialic acid linkages, and this polysaccharide is known to be susceptible to endoE-mediated hydrolysis (29). endoE had no effect on the complement sensitivity of E. coli LP1395 (Fig. (Fig.3C);3C); this strain does not produce a PSA capsule and is complement resistant (25).
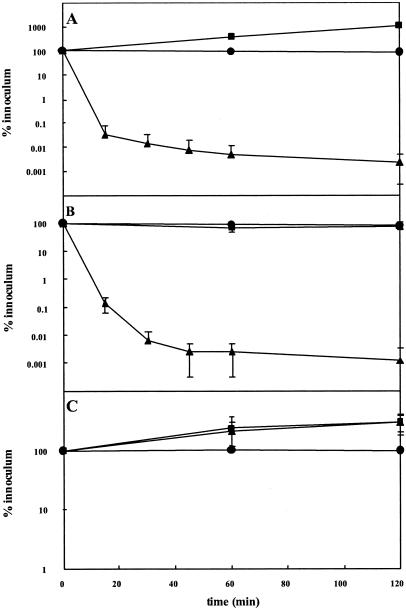
Sensitization of E. coli strains to complement following exposure to endoE. The K1 capsulate clinical isolate A192PP (A), the K92 strain Bos12 (B), and the non-K1, non-K92 isolate LP1395 (C) were exposed to 20% normal human serum containing 10 μg of recombinant endoE. Symbols: , bacteria plus serum;
, bacteria plus serum plus endoE; •, bacteria plus endoE (no serum).
PSA-mediated inhibition of alternative pathway amplification results in the binding of only very small amounts of C3 cleavage products to the bacterial surface and consequently little or no deposition of poly-C9, the terminal product of complement activation responsible for membrane perturbation and bacterial cell death (27). Analysis of cell envelopes prepared from the complement-resistant strain A192PP incubated with human serum for 1 h indicated that little C3-derived protein and no detectable C9 was deposited onto the target cell surface (Fig. (Fig.4).4). However, endoE-mediated removal of PSA from the bacterial surface led to a marked increase in binding of C3 cleavage products and to sufficient C9 deposition to account for the observed increases in complement sensitivity (Fig. (Fig.4).4). LP1395 remained refractory to C3b and C9 deposition after endoE treatment, as we have previously determined (27).
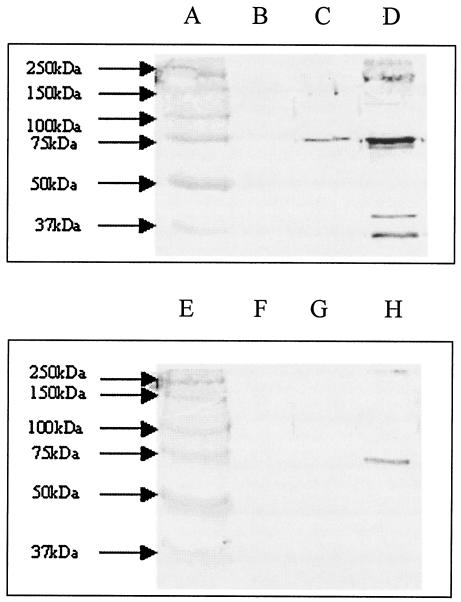
Removal of PSA from E. coli increases deposition of C3 cleavage products and C9. Membranes from mid-logarithmic-phase cells exposed to 20% normal human serum were subjected to SDS-PAGE, and proteins were transferred to nitrocellulose by electroblotting and then probed with antibodies to C3 and C9. Lanes: A and E, molecular weight standards; B and F, A192PP membranes prior to serum exposure; C and G, 60-min exposure to serum; D and H, 60-min exposure to serum plus endoE. Lanes B to D were probed with anti-C3 antiserum; lanes F to H with anti-C9 antiserum.
DISCUSSION
In the present study we provide clear evidence that attenuation of bacterial virulence by modification of the phenotype can prevent and cure severe, life-threatening infections due to pathogenic gram-negative bacteria. endoE selectively degrades PSA and removes the protective capsule from the surface of bacteria that express it (29). It has no effect on bacterial cell viability but can prevent and cure severe infections due to E. coli strains that carry on their surface the substrate for the enzyme. The most likely explanation is that the enzyme rapidly degrades the capsular material to oligosaccharides of 3 to 5 U (14) that are released from the bacterial surface, and this removes the constraints to complement activation. In addition, increased deposition of C3b and other C3 cleavage products is likely to lead to an increased uptake by phagocytic cells expressing appropriate receptors. This is currently under investigation.
endoE hydrolyzes α-2,8-linked sialic acid but fails to release α-2,3-, α-2,6-, or α-2,9-linked sialic acid residues from glycoproteins and other potential substrates (29). α-2,6- and, particularly, α-2,3-linked sialic acid residues are widely distributed within human and animal tissues, and we selected recombinant endoE for the present study partly on the basis that it would not remove terminal sialic acid residues from host glycoproteins, with likely negative consequences. α-2,8-linked PSA has a much more restricted distribution within tissues (30): its expression is highly regulated and is associated predominantly with neural cell adhesion molecules (NCAM) in the developing brain, facilitating neural tissue development by modulating cell-cell contact inhibition (30). In addition, PSA is transiently expressed during muscle development and in the embryonic kidney. Postpartum, the expression of PSA is restricted to a few areas of the brain where it is associated with regions that exhibit physiological plasticity, such as the hippocampus and olfactory bulb (23). The possibility exists, therefore, that endoE removes PSA from NCAM molecules at these restricted sites. We are currently using histochemical and histological procedures to determine the extent of endoE on PSA expression in the animal host. The effects of any PSA removal from host tissues are at present unclear, but we note in our study that all endoE-treated survivors appeared healthy.
The idea that conversion of pathogenic or drug-resistant bacteria to a “less-fit” phenotype might be a sound, alternative approach to the treatment of bacterial infections has been proposed in recent reviews (24, 28), but until now there has been no in vivo validation of the concept, at least in the modern era. More than 70 years ago, Dubos and Avery (4) used a capsule-depolymerizing enzyme preparation, isolated from cultures of a peat soil bacterium, to determine the effect of capsule removal on the outcome of experimental pneumococcal infections in mice (2) and rabbits (6). The enzyme specifically degraded the pneumococcal type III capsular polysaccharide, a major determinant of the capacity of strains to cause life-threatening infection. Intraperitoneal administration of the enzyme to mice prior to challenge with type III pneumococci gave rise to type III-specific protection and intravenous administration to rabbits with type III dermal infections resulted in early termination of the normally fatal experimental infection. With the onset of the antibiotic era, this innovative approach was not pursued. These studies reinforce our view that capsule-depolymerizing enzymes such as endoE retain their hydrolytic activity after administration to the animal host for a sufficient period to effect removal of the capsule from the surface of the invading bacterial cell; we are currently attempting to determine the extent of enzyme-mediated capsule loss in vivo.
Our initial experiments, represented by the data in Fig. Fig.1,1, were based on a 4-day treatment regimen; this course was adopted to ensure that we could detect any beneficial effects of endoE administration. However, repeated administration of a protein therapeutic may elicit the production of neutralizing antibodies, with a consequent reduction in efficacy. Therefore, once a therapeutic effect was established, we investigated the effect of a single intraperitoneal dose of the enzyme: 20 μg given on day 1 was sufficient to produce an optimal therapeutic effect (Fig. (Fig.2),2), and we will now determine the minimum effective dose and the optimal route of administration of the protein.
A case of neonatal meningitis is a medical emergency (13): the rapidly progressive nature of the infection demands a prompt and effective therapeutic response. Current best practice involves administration of combinations of broad-spectrum antibiotics, but mortality and morbidity rates remain unacceptably high. There is likely to be a delay before the broad-spectrum cephalosporins and aminoglycosides take effect (7), and this undoubtedly contributes to an unsuccessful outcome in some cases. The enzyme-mediated “capsule-stripping” approach may have an advantage in this situation, since its effects are likely to be immediate. Recent studies have proposed the use of bacteriophage lysins as treatments for streptococcal infections (16) and for anthrax (22) by the mechanism of “killing from without” through peptidoglycan hydrolysis. Although these studies have reinforced the principle that bacteriophage-derived enzymes can have therapeutic utility, they contrast with our data which demonstrate that it is possible to treat bacterial infections with an agent that does not kill directly but causes phenotypic modification of the bacteria enabling their removal by the host immune system.
Acknowledgments
This research was funded by project grants GA230 and GA382 from the British Society for Antimicrobial Chemotherapy and support from the Medical Research Council to J.P.L. and P.W.T.
We thank Dan Leggate for advice on the production of recombinant enzyme and Ana Mena Ribas and Sally Gray for technical support.
REFERENCES
Articles from Antimicrobial Agents and Chemotherapy are provided here courtesy of American Society for Microbiology (ASM)
Full text links
Read article at publisher's site: https://doi.org/10.1128/aac.48.5.1503-1508.2004
Read article for free, from open access legal sources, via Unpaywall:
https://europepmc.org/articles/pmc400570?pdf=render
Citations & impact
Impact metrics
Citations of article over time
Alternative metrics
Smart citations by scite.ai
Explore citation contexts and check if this article has been
supported or disputed.
https://scite.ai/reports/10.1128/aac.48.5.1503-1508.2004
Article citations
Pharmacokinetics and Biodistribution of Phages and their Current Applications in Antimicrobial Therapy.
Adv Ther (Weinh), 7(3):2300355, 13 Dec 2023
Cited by: 0 articles | PMID: 38933919
Potential of phage depolymerase for the treatment of bacterial biofilms.
Virulence, 14(1):2273567, 31 Oct 2023
Cited by: 2 articles | PMID: 37872768 | PMCID: PMC10621286
Review Free full text in Europe PMC
Translating bacteriophage-derived depolymerases into antibacterial therapeutics: Challenges and prospects.
Acta Pharm Sin B, 14(1):155-169, 18 Aug 2023
Cited by: 5 articles | PMID: 38239242 | PMCID: PMC10792971
Review Free full text in Europe PMC
Evolutionary and functional history of the Escherichia coli K1 capsule.
Nat Commun, 14(1):3294, 15 Jun 2023
Cited by: 7 articles | PMID: 37322051 | PMCID: PMC10272209
Phage Targeting Neonatal Meningitis E. coli K1 In Vitro in the Intestinal Microbiota of Pregnant Donors and Impact on Bacterial Populations.
Int J Mol Sci, 24(13):10580, 24 Jun 2023
Cited by: 1 article | PMID: 37445758 | PMCID: PMC10341584
Go to all (76) article citations
Similar Articles
To arrive at the top five similar articles we use a word-weighted algorithm to compare words from the Title and Abstract of each citation.
Administration of capsule-selective endosialidase E minimizes upregulation of organ gene expression induced by experimental systemic infection with Escherichia coli K1.
Microbiology (Reading), 156(pt 7):2205-2215, 15 Apr 2010
Cited by: 21 articles | PMID: 20395269 | PMCID: PMC2923034
Treatment of experimental Escherichia coli infection with recombinant bacteriophage-derived capsule depolymerase.
J Antimicrob Chemother, 56(1):160-165, 24 May 2005
Cited by: 52 articles | PMID: 15914489
Genome-Wide Identification by Transposon Insertion Sequencing of Escherichia coli K1 Genes Essential for In Vitro Growth, Gastrointestinal Colonizing Capacity, and Survival in Serum.
J Bacteriol, 200(7):e00698-17, 12 Mar 2018
Cited by: 23 articles | PMID: 29339415 | PMCID: PMC5847654
A review: relation between invasiveness and the K1 capsular polysaccharide of Escherichia coli.
Pediatr Res, 10(2):82-87, 01 Feb 1976
Cited by: 45 articles | PMID: 1107953
Review
Funding
Funders who supported this work.
Medical Research Council (2)
Novel Approaches to the Treatment of Bacterial Infections
Professor Peter Taylor, School Of Pharmacy
Grant ID: G0200320
Novel Approaches to the Treatment of Bacterial Infections
Professor Peter Taylor, School Of Pharmacy
Grant ID: G0200320(62380)