Abstract
Free full text

Human Orc2 localizes to centrosomes, centromeres and heterochromatin during chromosome inheritance
Associated Data
Abstract
The initiation of DNA replication in S phase requires the prior assembly of an origin recognition complex (ORC)-dependent pre-replicative complex on chromatin during G1 phase of the cell division cycle. In human cells, the Orc2 subunit localized to the nucleus as expected, but it also localized to centrosomes throughout the entire cell cycle. Furthermore, Orc2 was tightly bound to heterochromatin and heterochromatin protein 1α (HP1α) and HP1β in G1 and early S phase, but during late S, G2 and M phases tight chromatin association was restricted to centromeres. Depletion of Orc2 by siRNA caused multiple phenotypes. A population of cells showed an S-phase defect with little proliferating cell nuclear antigen (PCNA) on chromatin, although MCM proteins remained. Orc2 depletion also disrupted HP1 localization, but not histone-H3-lysine-9 methylation at prominent heterochromatic foci. Another subset of Orc2-depleted cells containing replicated DNA arrested with abnormally condensed chromosomes, failed chromosome congression and multiple centrosomes. These results implicate Orc2 protein in chromosome duplication, chromosome structure and centrosome copy number control, suggesting that it coordinates all stages of the chromosome inheritance cycle.
Introduction
The inheritance of eukaryotic genomes requires that DNA replication, chromosome segregation and cell division are coordinated. The origin recognition complex (ORC) serves as a landing pad for the ordered assembly of Cdc6, Cdt1 and the MCM proteins to form a pre-replication complex (pre-RC) at each origin of DNA replication prior to S phase (Kelly and Brown, 2000; Bell and Dutta, 2002; DePamphilis, 2003; Méndez and Stillman, 2003). First described in Saccharomyces cerevisiae (Bell and Stillman, 1992), ORC contains six conserved subunits Orc1–6 and is essential for the initiation of DNA replication in diverse organisms (Bell and Dutta, 2002).
In addition to its role in establishing pre-RCs on chromosomes prior to DNA replication, ORC is involved in other chromosome-associated processes. ORC associates with genetic elements called cis-acting silencers that form repressed chromatin at certain loci in S. cerevisiae (Bell et al, 1993; Kelly et al, 1994; Loo et al, 1995) and is associated with heterochromatin in Drosophila (Huang et al, 1998; Loupart et al, 2000; Gerbi and Bielinsky, 2002). In Drosophila and Xenopus, ORC interacts with heterochromatin protein 1 (HP1) (Pak et al, 1997). The Orc6 subunit of ORC binds to the outer kinetochore only during mitosis and localizes to the mid-plane of cell division in anaphase where it is required for cytokinesis via interaction with a septin protein (Prasanth et al, 2002; Chesnokov et al, 2003).
Although ORC is bound to chromatin at origins of replication throughout the cell cycle in yeast (Diffley et al, 1994; Aparicio et al, 1997; Weinreich et al, 1999), ORC is highly dynamic in mammalian cells (DePamphilis, 2003). In human cells, the largest subunit of ORC (Orc1) dissociated from chromatin during the G1- to S-phase transition and reloaded at the M–G1 transition when new pre-RCs were formed (Kreitz et al, 2001; Méndez et al, 2002; Tatsumi et al, 2003). The Orc2–5 subunits form a stable core and the Orc1 and Orc6 are loosely bound to the complex (Dhar et al, 2001a; Vashee et al, 2001).
The dynamics of human Orc2, an integral component of the core of ORC was examined. Orc2 was detected in the nucleus of interphase cells, but surprisingly a subset of Orc2 localized to centrosomes throughout the cell division cycle. In addition, Orc2 bound in a cell cycle-regulated manner to heterochromatin and HP1 proteins, initially localizing to heterochromatic foci, but only on centric heterochromatin during mitosis. Depleting Orc2 from human cells produced two cell cycle-arrested phenotypes. In some cells cell cycle progression was prevented and HP1 localization was disrupted, whereas other cells arrested in an aberrant mitotic-like state with replicated DNA. The latter cells had abnormal chromosome structure and congression as well as abnormal centrosome copy number. We propose that Orc2 participates in multiple aspects of the chromosome inheritance cycle, possibly coordinating DNA replication with chromosome segregation.
Results
Orc2 localizes to centrosomes
The localization of Orc2 throughout the cell division cycle was studied by immunofluorescence (IF) using polyclonal (pAb) and monoclonal (mAb) antibodies, both of which gave similar results. IF in HeLa cells using the anti-Orc2 mAb showed a nuclear, punctate labeling pattern during interphase (Figure 1Aa). A subset of Orc2 localized to a cytoplasmic dot (Figure 1Aa; see arrowhead) reminiscent of centrosome labeling. During metaphase and subsequent stages of mitosis, a fraction of Orc2 decorated the astral poles in addition to a diffuse labeling pattern that mostly excluded chromatin (Figure 1Ab–d). Orc2 redistributed to the daughter nuclei during telophase (Figure 1Ae). Double labeling with an anti-Orc2 pAb and an antibody against the centrosome protein γ-tubulin showed colocalization at centrosomes throughout the cell cycle, in addition to the punctate nuclear localization during interphase (Figure 1B). Peptide-blocking experiments demonstrated that the two Orc2 antibodies recognized different epitopes, confirming the specificity of Orc2 staining (Supplementary Figure S1).
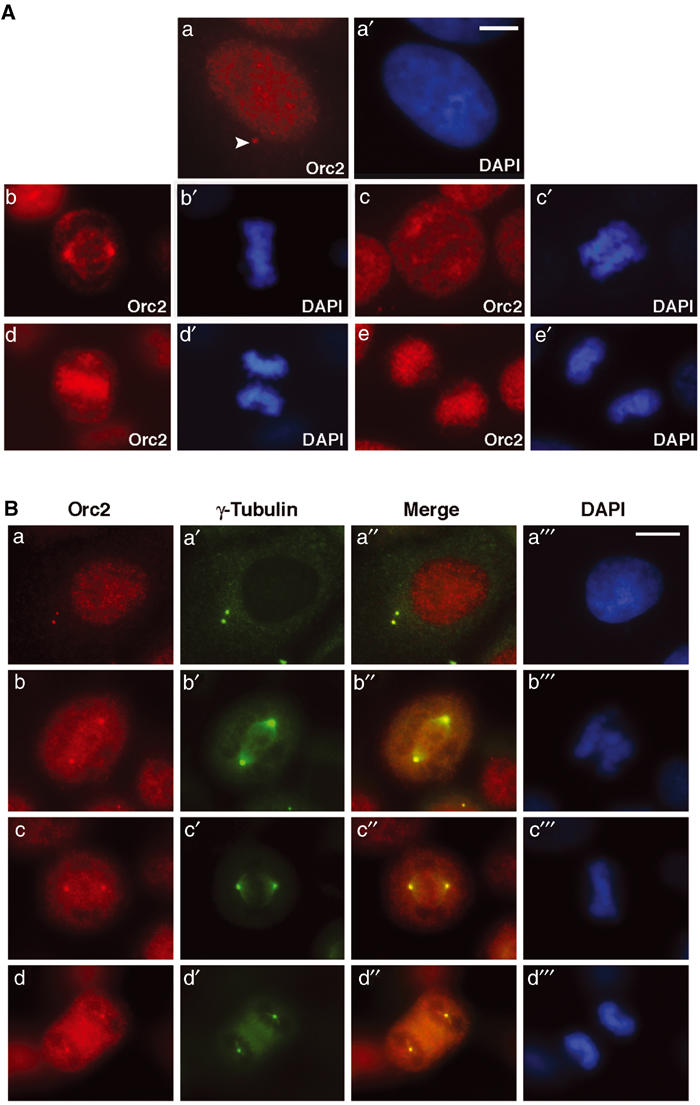
Orc2 localizes to the centrosomes. (A) Cell cycle distribution of Orc2. Cells were fixed with 1.0% paraformaldehyde and immunolabeling was carried out as described in Materials and methods. Indirect IF localization (red) of Orc2 proteins in HeLa cells during interphase (a), metaphase (b), early anaphase (c), late anaphase (d) and telophase (e). The arrow in (a) denotes the ‘dot' seen as the centrosome association during interphase. Chromatin was stained with 4′, 6-diaminidino-2-phenylindole (DAPI; a′–e′) in blue. Scale bar denotes 5 μm (a, a′) and 10 μm (b–e′). (B) Centrosome localization of Orc2. Dual-color indirect IF analysis using anti-Orc2 (red; a–d) together with γ-tubulin (green; a′–d′). Chromatin was stained with DAPI (blue; a–d
). Merges highlight the areas of colocalization (yellow; a″–d″). The centrosome association of Orc2 was present during interphase, as single dot during G1 (A, a), as a doublet during S and G2 (B, a–a
) and during subsequent mitotic stages, prometaphase (b–b
), metaphase (c–c
) and anaphase (d–d
). Scale bar, 5 μm.
Cell cycle-dependent binding of Orc2 to heterochromatin
Following pre-extraction with detergent to remove the soluble fraction of Orc2, Orc2 in the nucleus localized in two different patterns. Small, punctate spots throughout the nucleus (Figures 2Aa and Ca, b) disappeared as cells progressed through S phase (Figure 2Cc, d). The remaining Orc2 colocalized with the pronounced, HP1α-containing heterochromatic foci that were prominent in human MCF7 breast epithelial cells (Figure 2Aa–a′′). Paraformaldehyde (PF) fixation without detergent pre-extraction also revealed the presence of Orc2 at the heterochromatin foci, although partially masked by the soluble pool (Supplementary Figure S2Aa–a′). The association of Orc2 with heterochromatic foci was also observed in HeLa cells (Supplementary Figure S2Ba–a). Epitope-tagged versions of Orc2, including YFP-Orc2 and T7-Orc2, showed heterochromatin association, corroborating the IF studies (Supplementary Figure S3). Immunoprecipitation of Orc2 showed association with HP1α and HP1β, but not with HP1γ (Figure 2B). Furthermore, Orc2 IF in MCF7 cells revealed cell cycle-dependent binding of Orc2 to the heterochromatin as cells progressed through S phase, as monitored by the pattern of proliferating cell nuclear antigen (PCNA) labeling after detergent pre-extraction (Figure 2C). Orc2 localized with heterochromatic foci during G1 phase and early S phase (Figure 2Ca–a′′), but localized to fewer punctate spots in mid-S (Figure 2Cb–b′′, c–c′′) and even fewer in late S (Figure 2Cd–d′′; data not shown). The punctate pattern of Orc2 also decreased during S phase (compare Figure 2Ca–d). The late S-phase pattern was shown to be centric heterochromatin by dual-color immunolocalization of Orc2 with the auto-antibody (AnaC) that recognized centromere proteins (Figure 2Da–a′′). Similar centromeric association of Orc2 was also obtained in other human cell lines (MCF10A, IMR90, RKO, 293, HCT and HS68). Heterochromatin association of Orc2 was also observed in mouse embryonic fibroblasts (Figure 2Ea–a′). Orc2 localization at centromeres was confirmed by labeling metaphase chromosome spreads of human and mouse cells (Figure 2F).
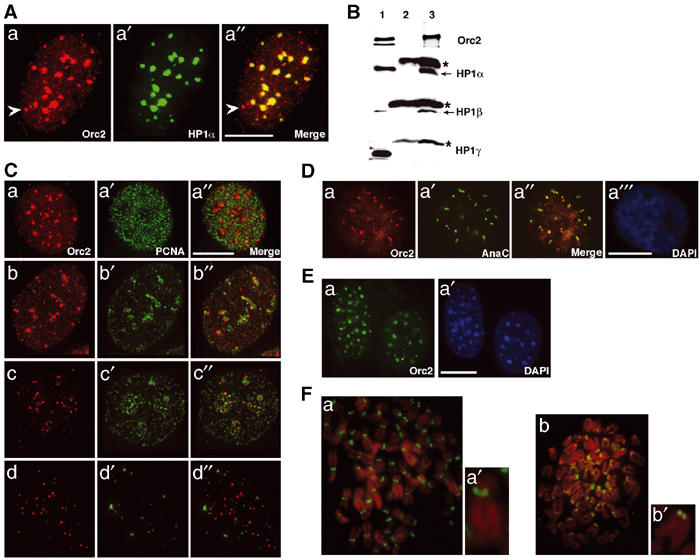
Heterochromatin association of Orc2. (A) IF following pre-extraction procedure was carried out to study colocalization of Orc2 (a) and HP1α (a′) in MCF7 cell line. Merge (a″) represents the areas of colocalization. The arrow in (a) and (a″) denotes the centrosome association of Orc2. (B) Orc2 immunoprecipitates HP1α and HP1β. Immunoprecipitation (IP; Nielsen et al, 1999) using Orc2 mAb and immunoblot with Orc2 pAb, HP1α, HP1β and HP1γ. Lane 1 represents input, lane 2 is 12CA5 mAb IP (mouse IgG negative control) and lane 3 is Orc2 IP. Note the light chain of IgG in HP1α, HP1β and HP1γ immunoblots (‘*' lanes 2 and 3). (C) Differential binding of Orc2 to heterochromatin. Dual-color immunolocalization studies in MCF7 cells of Orc2 (red; a–d) and PCNA (green; a′–d′) did not reveal significant colocalization (merges; a″–d″). (a–a′′) Early S, (b–b″, c–c″) mid-S and (d–d″) late S. (D) Orc2 decorates centromeres. Orc2 (a) and centromere marker AnaC (a′) show significant colocalization (a″) in MCF10A cells. DAPI (a) staining shows a typical late G2 cell. (E) Mouse Orc2 localizes to heterochromatin. Immunolocalization using Orc2 mAb in MEFS (a) shows Orc2 in DAPI dense pericentric heterochromatin blobs (a′). (F) Orc2 at centromeres is evolutionarily conserved. Orc2 (green) labeling in metaphase chromosome spreads from IMR-90 (a) and MEFs (b). (a′) and (b′) are higher magnification of individual chromosome from (a) and (b), respectively. Scale bars in (A), (C), (D) and (E) represent 5 μM.
Orc2 depletion has two arrest points: a DNA replication defect and an aberrant mitotic arrest
RNA interference (RNAi) was used to determine the consequences of loss of Orc2 in human cells (Elbashir et al, 2001). Transfection of HeLa cells with two independent, duplex, small interfering RNAs (siRNA) caused significant loss of Orc2 by 72 h post siRNA (Figure 3A and Supplementary Figure S4). Orc2 mRNA was eliminated (data not shown). Interestingly, in Orc2 siRNA-treated cells, the level of Orc3 decreased concomitant with the reduction in Orc2 levels (Figure 3A). A similar dependency of Orc2 stability on Orc3 levels was observed following Orc3 siRNA (Supplementary Figure S5); however, the levels of other ORC subunits were unaltered in Orc2-depleted cells (see Orc1 and Orc6 in Figure 4B; Orc4 and Orc5, data not shown).
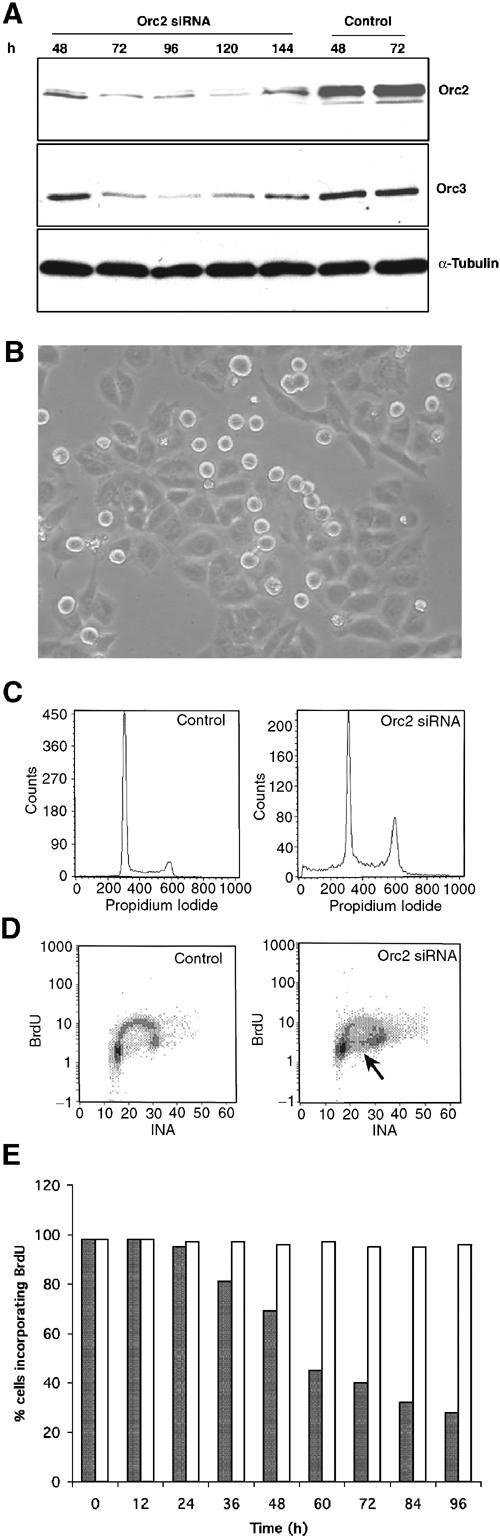
Orc2 depletion has dual execution points. (A) Immunoblot of whole-cell extract from cells transfected with Orc2 siRNA duplex or control luciferase siRNA and harvested at different time points. Cells were transfected at 0, 24 and 48 h at about 20% starting confluence. Efficacy of RNAi was assessed by immunoblotting to detect Orc2. α-Tubulin levels were used as loading controls. Orc3 levels were also modulated and, with the reappearance of Orc2 in the cells at 144 h, the levels of Orc3 also increased. (B) Orc2 depletion at 72 h post siRNA shows morphologically two distinct populations of cells: the flat adherent cells and the rounded mitotic cells. (C) DNA content of control and Orc2 siRNA-treated cells at 48 h was determined by flow cytometry. Note a broader S phase and an increase in G2/M in Orc2 siRNA-treated cells. (D) Two-dimensional FACS to assess BrdU incorporation (10 min pulse) and DNA content by propidium iodide (PI) was assessed by flow cytometry in control and Orc2-depleted cells at 72 h. Note an increase in G2/M and accumulation of cells in S phase without incorporation of BrdU (see arrow) in Orc2-depleted cells. (E) Histogram showing the percentage of cells incorporating BrdU during a 24 h label at different time points after transfection, with Orc2 (gray bars) or luciferase control (white bars) siRNA duplexes. BrdU incorporation was allowed for 24 h to ensure that all cells had a chance to pass through at least one S phase.
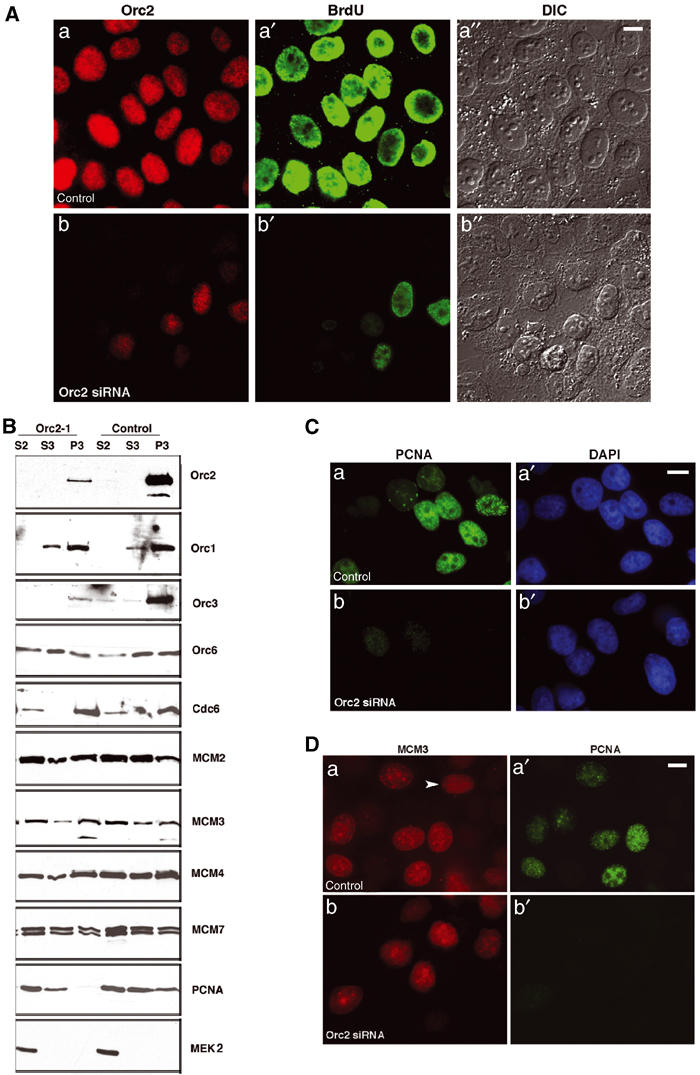
Orc2 depletion affects S-phase progression. (A) Immunolabeling of cells at 72 h after transfection with control or Orc2 siRNA duplexes (a, b); BrdU incorporation during a 24 h pulse (a′, b′); Nomarski images (a″, b″). Scale bar, 10 μM. (B) Luciferase (control) and Orc2 siRNA duplex-treated cells were subjected to biochemical fractionation (Méndez and Stillman, 2000). The relative abundance of different replication proteins was tested by immunoblotting. S2, soluble cytosolic fraction; S3, soluble nuclear fraction; P3, chromatin-enriched fraction. Antibodies against Orc2, Orc1, Orc3, Orc6, Cdc6, MCM2, MCM3, MCM4, MCM7 and PCNA were used. Cytosolic kinase MEK2 was used as a control. Note that Orc3 and PCNA loading was affected in Orc2-depleted cells. (C) Chromatin association of PCNA was assessed by IF in control (a–a′) and Orc2-depleted cells (b–b′). DNA was stained with DAPI (a′, b′). Scale bar, 10 μM. (D) Chromatin-bound MCM3 was analyzed by a detergent pre-extraction procedure in control (a) and Orc2-depleted cells (b) by dual-color IF for MCM (red; a–b) and PCNA (green; a′–b′). The arrow in (a) denotes an MCM-positive, PCNA-negative G1 cell (Prasanth et al, 2004). Total number of cells positive for MCM2 and MCM3 in Orc2-depleted cells was 77 and 76%, respectively, whereas the same for the control was 62 and 60%. In control cells, the MCM2+/PCNA+ was 24%, MCM2+/PCNA− 38%, MCM2−/PCNA+ 2% and MCM2−/PCNA− 36%. Similar results were obtained for MCM3 in control cells. In contrast, for Orc2 siRNA-treated cells, MCM2+/PCNA+ was 15%, MCM2+/PCNA− 62% and MCM2−/PCNA− 23%. Scale bar, 10 μm. RNAi-treated cells were assessed at 72 h.
Following Orc2 depletion, two morphologically distinct populations consisting of flat and round cells were observed (Figure 3B). Flow cytometry showed an increase in cells in S-phase and with a replicated, 4C DNA content compared to the normal DNA content in cells transfected with control siRNA (Figure 3C). Dual-color flow cytometry analyzing bromodeoxyuridine (BrdU) incorporation versus DNA content showed that Orc2 depletion prevented cells from progressing through S phase and accumulated cells with a 4C DNA content (Figure 3D). The unusual presence of cells with an S-phase DNA content but not incorporating BrdU (see arrow) suggested that loss of Orc2 could block DNA replication. The loss of BrdU incorporation in a 24-h pulse label paralleled the loss of Orc2 protein (Figure 3E). Since there were two morphologically distinct cell phenotypes in the Orc2-depleted population, they were characterized separately. The flat cells that remained attached to the dish were first characterized.
Silencing Orc2 expression affects S-phase progression
The flat cells were pulse-labeled with BrdU for 24 h from 72 to 96 h following three rounds of transfection of siRNA and dual immunolabeling of Orc2 and BrdU was examined. Following Orc2 siRNA, the majority of cells lacking Orc2 did not incorporate BrdU (compare control siRNA in Figure 4Aa–a′′ with Orc2 siRNA in Figure 4Ab–b′′). Even the cells that incorporated BrdU showed differential signal intensity as compared to control.
The chromatin association of DNA replication proteins in Orc2-depleted cells was determined (Figure 4B). The majority of the Orc2 protein was bound to chromatin in control cells, whereas Orc2 siRNA-treated cells had significantly reduced levels of chromatin-associated Orc2 and Orc3, but not Orc1 or Orc6 (Figure 4B). Surprisingly, no dramatic difference was found in the chromatin association of the pre-RC proteins Cdc6 and MCM (MCM2, MCM3, MCM4 and MCM7), but chromatin loading of PCNA was reduced (Figure 4B). To confirm the observations made by biochemical fractionation, IF following detergent pre-extraction of non-chromatin-bound proteins was performed. Compared to control siRNA, PCNA association with chromatin was severely compromised in Orc2 siRNA-treated cells (12–15% positive (all weak) compared to 31% in control cells; Figure 4C). MCM3 labeling on the chromatin by IF did not show significant variation when compared to the control (Figure 4D), but the pattern of MCM IF (Dimitrova et al, 1999; Prasanth et al, 2004) clearly demonstrated that some cells had entered or were at different stages of S phase (see Supplementary Figure S6A). In addition, MCM-positive cells had duplicated centrosomes that had moved apart, again suggesting that the cells were in S and not only arrested in G1 (Supplementary Figure S6B).
The S-phase pattern identification based on the BrdU profile following a 10 min BrdU pulse (O'Keefe et al, 1992) showed 26% cells incorporating BrdU in control cells, of which 60% were in early S, 25% in mid-S and 15% in late S (N=500). In contrast, in Orc2 siRNA-treated cells, only 14–15% of cells incorporated BrdU with low signal intensity and, of these, 75% were in early, 19% in mid-S and only 6% in late S (N=1500). The IF statistics corroborated the BrdU/PI flow cytometry profile, suggesting slow S-phase progression and defective late DNA replication.
Disruption of HP1α and HP1β localization in Orc2-depleted cells
Since Orc2 was found to bind to heterochromatin, the status of heterochromatin proteins in the attached, flat Orc2-depleted cells was examined. HP1α labeling after detergent pre-extraction in control HeLa cells showed pronounced heterochromatin foci (Figure 5Aa–a′), whereas cells treated with Orc2 siRNA showed a homogenous labeling of HP1α (>60% of the cells; Figure 5Ab–b′). Similar observations were made with HP1β (Figure 5Ba, b). However, the trimethyl lysine 9 modification on histone H3 was comparable in control versus the Orc2 siRNA-treated cells (Figure 5Ba′, b′). Similarly, HPIγ staining was normal in cells lacking Orc2 (Supplementary Figure S7A). HP1α, HP1β and trimethyl K9 at histone H3 levels were comparable in lysates from control and Orc2-depleted cells (Supplementary Figure S7B).
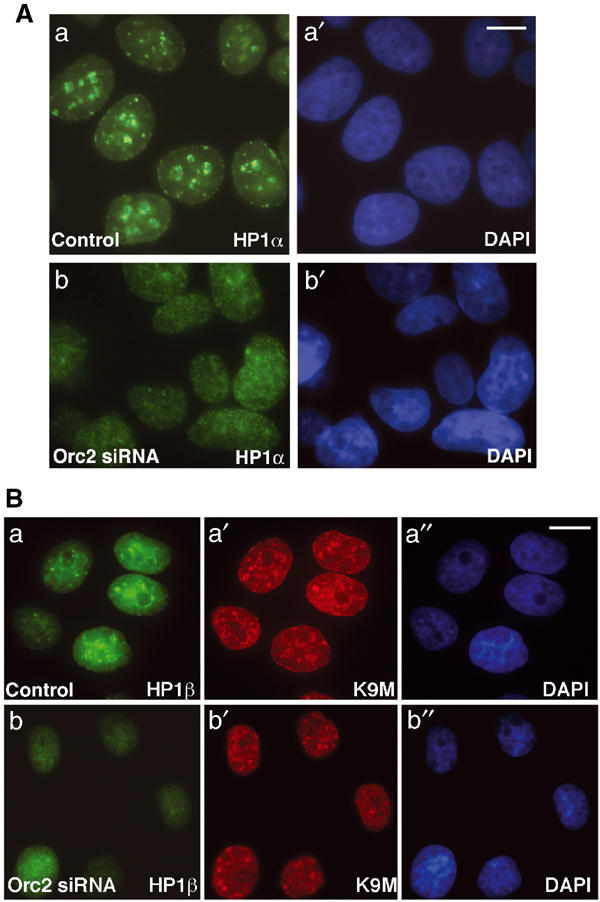
Orc2 depletion disrupts heterochromatin organization. (A) Prominent HP1α labeling in heterochromatin foci (a) in luciferase siRNA control cells as compared to loss of these foci in Orc2 siRNA-treated cells (72 h post transfection) (b). DNA was stained with DAPI (a′, b′). (B) Luciferase siRNA-treated cells showing HP1β-positive foci (a) at the sites of trimethyl K9 foci of histone H3 (a′); in contrast, in the Orc2 siRNA-treated cells, note the loss of HP1β foci (b) but no significant loss of trimethyl K9 foci (b′). Chromatin was stained with DAPI (blue; a″, b″). Scale bar in (A) and (B) represents 5 μM.
A population of Orc2-depleted cells arrest in an abnormal mitosis with chromosome segregation, condensation and spindle defects
Depletion of Orc2 caused some cells to arrest with a mitotic-like rounded morphology (Figure 3B). α-Tubulin staining of the entire cell population showed that the round cells were in an abnormal prophase or prometaphase with very few cells in anaphase and telophase (Supplementary Figures S8A and S9). The mitotic index was ~30±2.3% by 96 h as compared to the control constant value of 6.8±1.5% (Figure 6A). The marked increase in prophase+prometaphase cells and the significant reduction in the number of anaphases and telophases in Orc2 siRNA-treated cells suggested that cells did not complete mitosis. Phospho-H3 (serine 10) labeling was normal in these Orc2-depleted, mitotic cells (Supplementary Figure S8B).
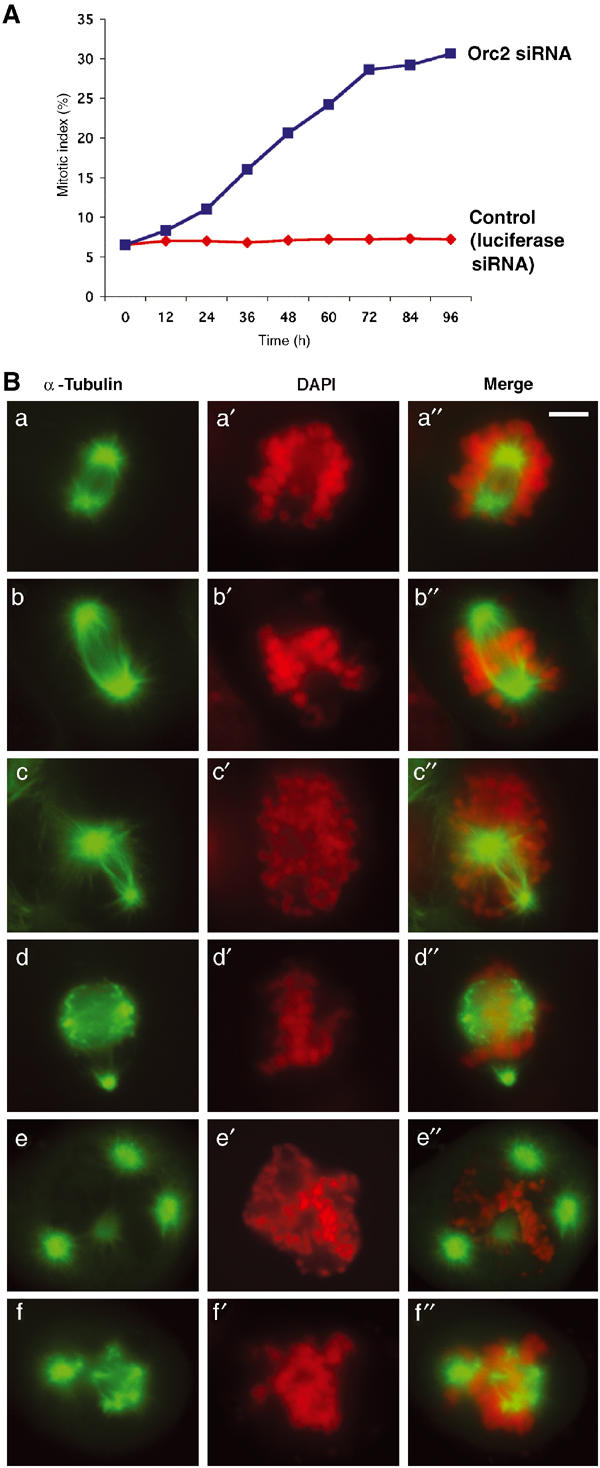
Orc2 depletion affects chromosome segregation and spindle organization. (A) Time course of mitotic index in control (luciferase siRNA treated, red) versus Orc2 siRNA-treated cells (blue) carried out by counting the α-tubulin-staining cells with mitotic asters. (B) IF with α-tubulin (green) and DAPI (red) was used to study the spindle structure and chromosome alignment in mitotically arrested cells depleted of Orc2 at 72 h post transfection. Chromosome congression failure with unaligned chromosomes (a–a″, b–b″, c–c″, d–d″, e–e″, f–f″), and spindle defects with asymmetric, bipolar (c–c″) and multipolar spindles (d–d″, e–e″, f–f″) were observed. More than 55% of mitotic cells showed lagging chromosomes, as compared to 11% in control. Merges are shown in (a″), (b″), (c″), (d″), (e″) and (f″). Scale bar, 5 μM.
The chromosomes in Orc2-depleted cells were not organized by the formed spindles, suggesting a centromere or kinetochore defect (Figure 6Ba–a′′, b–b′′, c–c′′ and Table I). Gross abnormalities in spindle formation were found, with ~50% of the mitotic cells forming multipolar asters (Figure 6Bd–d′′, e–e′′, f–f′′, Table I, and Supplementary Movie 1) and ~20% possessing asymmetric, bipolar asters (Figure 6Bc–c′′ and Table I). At 72 h, mitotic cells with multipolar asters contained multiple centrosome-like structures (anti-γ-tubulin labeling; Table I and Supplementary Figure S10A). The centrosomes of HeLa cells arrested in the aberrant mitotic state failed to label with anti-Orc2 antibodies, suggesting that the Orc2 centrosome labeling was specific (Supplementary Figure S10B).
Table 1
Tabulation of spindle defects and chromosome condensation and alignment defects following depletion of Orc2 from human cells
![]() | |||||||
---|---|---|---|---|---|---|---|
24 (11%) | 2.8 | 14.5 | 61.5 | 12.6 | 8.6 | 39 | 46 |
48 (20.6%) | 5 | 15.7 | 12.9 | 15.4 | 51 | 77 | 57 |
72 (28.6%) | 10 | 16.5 | ~5 | 21 | 50 | 96 | 55 |
Control (~7%) | 2 | 3 | 87 | 3 | 5 | — | 11 |
N=550 each. |
Like Orc2, CENP-F (mitosin) localizes to both kinetochores and centrosomes (Yang et al, 2003). Thus the localization of CENP-F in siRNA-treated cells was examined. In the Orc2-depleted cells, but not in the control cells, centrosome localization of CENP-F was abolished, but the kinetochore-associated CENP-F increased compared to control siRNA-treated cells, suggesting abnormalities in mitotic regulation (Figure 7).
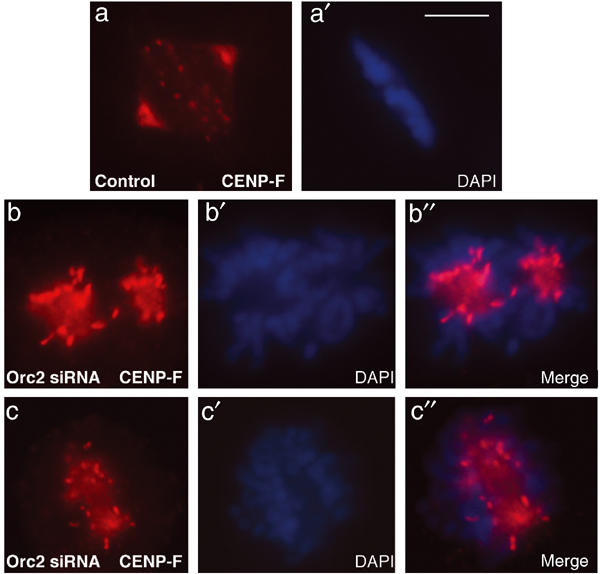
Immunolocalization of CENP-F following Orc2 siRNA. CENP-F in control and Orc2 siRNA-treated cells was determined by IF. Control siRNA showed kinetochore and centrosome staining (a–a′). Cells lacking Orc2 showed increased CENP-F staining at unaligned kinetochores but lacked centrosome staining (b–b′, c–c′). DNA was stained with DAPI (blue). Scale bar, 5 μM.
The Orc2-depleted mitotic cells lacked HP1α at centric heterochromatin similar to that observed in Orc2-depleted flat cells (Supplementary Figure S11). The structure of the chromosomes in the aberrant mitotic cells was examined by preparing chromosome spreads. Orc2-depleted cells showed abnormally condensed chromosomes, with much thicker and wider sister chromatids as compared to the control (Figure 8Aa, b). These chromosomes were replicated as evidenced by the well-separated sister chromatids. In all, 80% of the metaphase spreads showed the thick, dumpy chromosomes and 10% showed pulverized and fragmented chromosomes. These chromosomes were shorter end-to-end than normal chromosomes. To assess the DNA replication pattern on these dumpy chromosomes, chromosomes were prepared from cells that had been labeled for 24 h with BrdU and the incorporation was analyzed by immunolabeling on the spread, mitotic chromosomes. In the chromosomes from control cells, complete arms of the sister chromatids were labeled, whereas the dumpy chromosomes from Orc2-depleted cells showed sparse incorporation at discrete foci (Figure 8B). This suggests that these chromosomes had completed late S phase during the labeling.
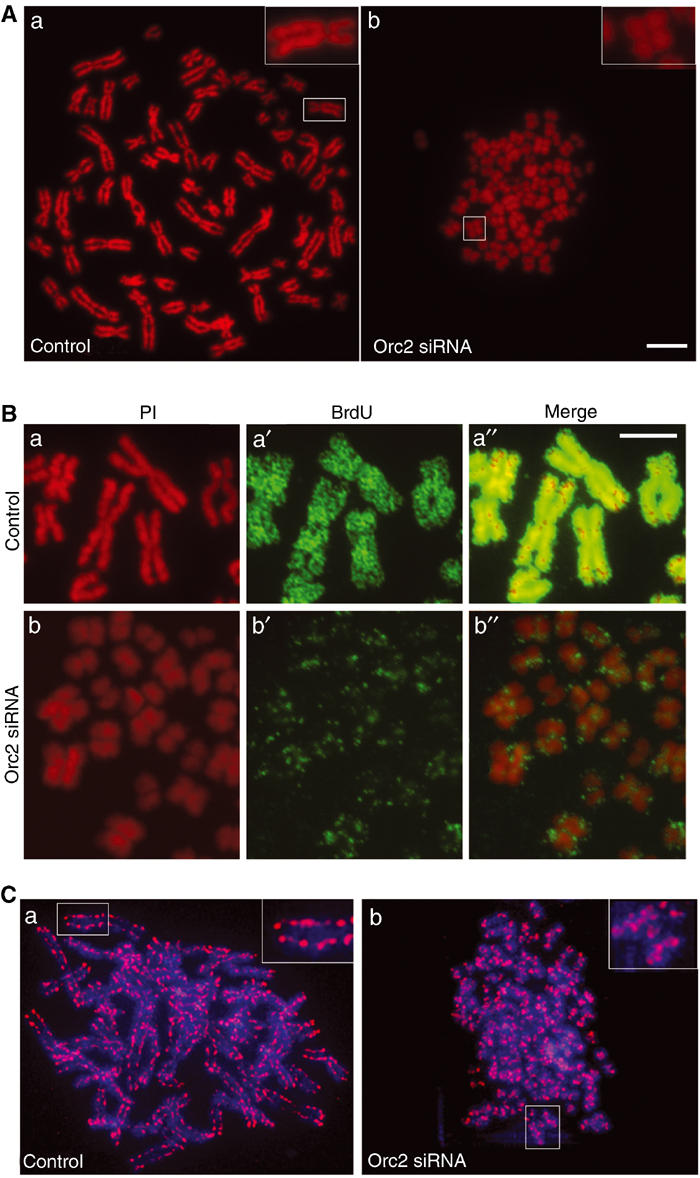
Orc2 depletion causes chromosome condensation defects. (A) Metaphase plate preparation in control (a) and in Orc2-depleted (b) cells (72 h). Insets in (a) and (b) are higher magnification of the boxed portion of the image. Scale bar, 10 μM. (B) Cells were labeled with BrdU for 24 h (from 72 to 96 h) and mitotic chromosomes spread as in (A). Control metaphase chromosomes (a–a″) and Orc2-1-depleted cells (b–b″). Red, PI staining (a, b); green, BrdU labeling (a′, b′); merge (a″–b″). Scale bar, 5 μM. (C) Status of a non-SMC subunit of condensin I (h-CAP-G, red) in metaphase chromosomes of control (a) and Orc2 depleted (b). Insets in (a) and (b) are higher magnification of the boxed portion of the image. DNA was stained with DAPI (blue).
The failure of chromosomes to condense correctly could be due to an absence of condensin (Ono et al, 2003). The status of condensin in the control and dumpy chromosomes was examined by labeling with an antibody against CAP-G, a non-SMC subunit of condensin I. Overall condensin levels were similar in both types of chromosomes; however, in control cells, condensin decorated the chromosomes as ‘beads on a string' along the axis of the two sister chromatids (Figure 8Ca and Supplementary Movie 2), whereas the Orc2-depleted cells showed a spiral conformation of condensin (Figure 8Cb, Supplementary Movie 3 and Supplementary Figure S12).
PCNA knockdown results in cells undergoing apoptosis
To address whether the mitotic arrest following Orc2 knockdown was a consequence of a defect in DNA replication, depletion of a crucial DNA replication protein PCNA was studied (Supplementary Figure S13). The resulting phenotype was very different since depletion of PCNA caused immediate apoptosis (see Supplementary Note 1 and Supplementary Figures S13 and S14). Thus inhibition of ongoing DNA replication did not necessarily result in condensed chromosomes and aberrant mitosis.
Discussion
Orc2 localizes to multiple sites involved in chromosome duplication and segregation
The Orc6 subunit associates with kinetochores only during mitosis (Prasanth et al, 2002), raising the possibility that other ORC subunits also localize to centromeres. In addition to the expected localization of Orc2 to small, punctate spots in the interphase nucleus in human cells, the protein also localized to multiple sites involved in chromosome structure and segregation. Orc2 colocalized with large HP1-containing heterochromatic foci in the early half of the cell division cycle but as S phase progressed and cells entered into M phase, Orc2 localization on chromosomes was restricted to centric heterochromatin. A recent report also described Orc2 association with mitotic centromeres in human and mouse cells (Craig et al, 2003). Unexpectedly, Orc2 was also found to be associated with centrosomes throughout the cell division cycle.
Depletion of Orc2 from human cells using siRNA resulted in a DNA replication defect. ORC is required for initiation of DNA replication in yeast and vertebrate cells (Kelly and Brown, 2000; Kiely et al, 2000; Dhar et al, 2001b; Gillespie et al, 2001; Shimada et al, 2002; DePamphilis, 2003). Unexpectedly, however, depletion of Orc2 did not reduce MCM chromatin association. In rapidly proliferating cells, however, the pre-RC is formed during a short time window as cells exit mitosis (Carpenter et al, 1996; Romanowski et al, 1996; Dutta and Bell, 1997; Kelly and Brown, 2000; Méndez and Stillman, 2000; Dimitrova et al, 2002; DePamphilis, 2003). If Orc2 depletion were to inhibit pre-RC formation, and hence MCM loading, the cells with replicated DNA would have to enter and then exit from mitosis. Depletion of Orc2, however, led to an arrest in M phase in ~30% of the cells and thus it is likely that cells did not progress to the stage in late mitosis where an effect on pre-RC formation could occur. Thus there was not an opportunity to reduce MCM loading due to a failure in pre-RC formation. The majority of the flat cells were in G1 or S phase with MCM on chromatin, and in such cells Orc2 depletion did not cause removal of chromatin-bound MCM. Similar results have been reported when ORC was depleted from MCM-containing chromatin formed in a Xenopus egg extract (Hua and Newport, 1998; Rowles et al, 1999). In the present study, there was, however, a loss of PCNA loading onto chromatin and BrdU incorporation. Many of these cells had an MCM staining pattern consistent with an arrest in G1 or S phase (Prasanth et al, 2004). These observations suggest that ORC is also required in cells for stages of DNA replication after formation of pre-RCs. These results contrast observations in Xenopus egg extract where ORC was not required for DNA synthesis after pre-RC formation (Hua and Newport, 1998; Rowles et al, 1999; Sun et al, 2002). We suggest that in intact cells Orc2 is part of a structure on which DNA replication occurs. Orc1 is not part of this structure since it is degraded as cells enter into and progress through S phase. As expected, depleting Orc1 or Cdc6 from human cells with siRNA did prevent MCM loading onto chromatin (Ohta et al, 2003; J Méndez and B Stillman, unpublished observations).
The depletion of Orc2 also caused a reduction in Orc3 levels and therefore the observed effects could be due to simultaneous loss of Orc3. Similarly, a human cell line with a hypomorphic form of Orc2 (Orc2*) resulted in an approximate four-fold reduction in chromatin-bound Orc2* and Orc3 but relatively normal amounts of chromatin-bound RPA (Dhar et al, 2001b). The mutant cells proliferated at about 50% the level of wild-type cells. In these cells, a chromosome initiation defect was not detected, nor was there a reported mitotic defect, but there was a loss of replication from a plasmid with one origin of DNA replication. In contrast, acute loss of Orc2 completely blocked all DNA replication and caused a mitotic defect.
A widely distributed, punctate pattern of Orc2 localization was observed in G1- and early S-phase cells, but these small spots disappeared as cells progressed through S phase (Figure 2). Because a subset of similar spots containing Xenopus Orc1 was shown to colocalize with sites of initiation of DNA replication (Romanowski et al, 1996), the smaller Orc2 spots probably correspond to sites of pre-RC formation. Their gradual disruption during S phase parallels the disruption of MCM localization that occurs at the same time (Tye, 1999).
Previous reports suggested that Orc2 was bound to chromatin throughout the cell cycle, based on biochemical fractionation and immunoblotting (Ritzi et al, 1998; Méndez and Stillman, 2000; Natale et al, 2000; Méndez et al, 2002; Ohta et al, 2003). This has led to speculation that Orc2 and some of the other ORC subunits may form a stable core of ORC on chromatin onto which the Orc1 subunit may assemble prior to formation of the pre-RC (see discussion in DePamphilis, 2003). However, it is clear from the results presented here that Orc2 association with chromatin is dynamic throughout interphase and simple chromatin fractionation does not reveal the full story. The Orc2 previously found to be associated with fractionated chromatin in late S, G2 and M phases probably represents Orc2 bound to centric heterochromatin.
Association of Orc2 with heterochromatin and HP1
The colocalization of Orc2 and HP1α and HP1β to prominent, heterochromatic foci was most apparent in mouse cells and some human cells such as MCF7, but existed in all human cells tested. Interestingly, the association of Orc2 with these heterochromatic foci was also cell cycle regulated. As cells progressed through S phase, Orc2 was no longer associated with the prominent HP1-containing heterochromatic foci and, by the end of S phase, Orc2 localized only with centromere-associated heterochromatin that contains HP1 (Kellum, 2003). In G1- and early S-phase cells, Orc2 was required to maintain the localization of HP1 at the heterochromatic foci since HP1 was found in a diffuse pattern throughout the nucleus in cells where Orc2 was depleted. Mutations in Drosophila Orc2 also disrupt HP1 association with heterochromatin (Huang et al, 1998). HP1 is dynamically associated with heterochromatin in interphase and rearranges during mitosis (Cheutin et al, 2003; Festenstein et al, 2003). The chromodomain of HP1 recognizes histone H3 that is methylated on lysine 9 (H3-K9M) and is required for HP1-induced gene silencing and also for centromere function in fission yeast and Drosophila (Kellum and Alberts, 1995; Firestein et al, 2000; Bannister et al, 2001; Grewal and Moazed, 2003). Orc2 depletion did not affect this modification and, therefore, the loss of HP1 from heterochromatin after Orc2 depletion suggests a role for ORC in recruiting or maintaining HP1 in the heterochromatin. The present study and results from Drosophila on the interaction between ORC and HP1 (Pak et al, 1997; Huang et al, 1998; Shareef et al, 2003) suggest that DNA-binding activities of ORC might be responsible for recruiting HP1 to heterochromatin, just as ORC recruits the S. cerevisiae Sir1 heterochromatin protein to specific, transcriptionally silenced loci (Triolo and Sternglanz, 1996).
HP1 was found to interact with the N-terminal domain of the Drosophila and Xenopus Orc1 subunit and to colocalize in embryo cells with the Orc2 subunit (Pak et al, 1997). The similar colocalization of Orc2 and HP1 in human cells suggests that ORC might control heterochromatin structure. In human cells, however, Orc1 is degraded after entry into S phase (Kreitz et al, 2001; Méndez et al, 2002; DePamphilis, 2003; Tatsumi et al, 2003) at the time when the Orc2 that is associated with HP1 begins to be restricted to centric heterochromatin (Figure 2). The association of Orc2 with HP1 in the latter half of the cell cycle when Orc1 is greatly reduced or not present suggests that Orc2 must associate with HP1 via another interaction. This interaction could be either direct, via another ORC subunit or via an ORC/HP1-associated protein (Shareef et al, 2003).
Orc2 remained localized to centromeric heterochromatin in metaphase chromosomes. This observation is in apparent contrast to previous studies in Drosophila cells where Orc2 only associated with chromosomes after anaphase (Loupart et al, 2000); however, others have found Orc2 at centric heterochromatin in Drosophila (Pak et al, 1997). The dynamic localization of Orc2 on chromosomes during the cell cycle might reflect multiple roles for this protein in chromosome structure and function. For example, it is possible that Orc2 and other subunits of ORC like Orc6 (Prasanth et al, 2002) localize to centromeres and are involved in kinetochore assembly and chromosome segregation, perhaps by helping to organize the microtubules.
The Drosophila Orc2 k43 mutant allele results in suppression of position effect variegation (PEV), consistent with the suggestion that ORC plays a role in formation or maintenance of heterochromatin (Pak et al, 1997). HP1/Swi6 has been shown to be required for the recruitment of cohesin to centromeric regions, promoting proper chromosome segregation (Grewal and Moazed, 2003). It is possible that the chromosomes fail to align and segregate after Orc2 siRNA because of improper cohesin loading at centromeres due to mislocalization of HP1 on centric heterochromatin. It is also possible that the defects are due to inadequate condensation in the heterochromatin only, causing these regions to stretch and fail to translate the pulling force of the spindle to the remainder of the chromosome (Kellum and Alberts, 1995; Grewal and Moazed, 2003).
Orc2 is required for correct chromosome structure and condensation in mitosis
In about 30% of the RNAi-treated cells, Orc2 depletion resulted in an arrest in mitosis with abnormally condensed chromosomes. It is noteworthy that the abnormally condensed chromosomes replicated very slowly, or more likely were completing S phase at the time when Orc2 was depleted. Similar mitotic defects have been reported in Orc2, Orc3 and Orc5 mutants in Drosophila (Loupart et al, 2000; Pflumm and Botchan, 2001; McHugh and Heck, 2003). Interestingly, Orc3 siRNA treatment in human cells also showed an altered G1 to G2/M ratio suggesting an M-phase arrest (Supplementary Figure S5). Mutations in genes encoding other initiation proteins such as Mcm10, Cdc45 and Mcm2 in Drosophila have also been shown to cause abnormal chromosome condensation (Christensen and Tye, 2003). However, depletion of other replication proteins such as PCNA (present study), Orc1 and Cdc6 (J Méndez and B Stillman, unpublished observations) and Rfc4 in Drosophila (Krause et al, 2001) did not result in abnormal chromosome condensation, suggesting that aberrant DNA replication does not ineluctably lead to mitotic defects. Although the abnormalities observed following Orc2 depletion could be a consequence of infrequent initiation of DNA replication in the genome, as previously suggested (Pak et al, 1997; Loupart et al, 2000), we suggest that the specific mitotic phenotype is due to abnormalities in both centrosome and centromere/kinetochore function. Since Orc2 localizes to both structures and disrupts centrosome localization of proteins such as CENP-F, it is likely that depletion of Orc2 has a direct role in causing the phenotype. The partially condensed and short, supercoiled chromosomes we observed are remarkably similar to the supercoiled chromosomes observed when a Condensin I subunit was depleted from human cells (Ono et al, 2003), suggesting a link between DNA replication and condensin activity later in the cell cycle.
Association of Orc2 with centrosomes
The spindle checkpoint is able to detect a single unaligned chromosome causing a prometaphase arrest until proper bipolar attachment is achieved (Rieder et al, 1995). Depletion of Orc2 from human cells impaired chromosome congression and, in many cases, none of the chromosomes were correctly aligned on the spindle. This suggested a kinetochore defect and, consistent with this, we observed increased CENP-F localization on chromosomes in such cells. In addition, the spindle itself was not correctly assembled and there was an abnormal number of mitotic asters in Orc2-depeleted cells. Moreover, CENP-F failed to localize to centrosomes in the absence of Orc2. Many studies have shown a role for centromeric heterochromatin in the assembly of a functional kinetochore that in turn regulates microtubule attachment and the spindle checkpoint (Allshire, 1995; Ekwall et al, 1995). There is also compelling evidence that the spindle checkpoint is linked to centrosome (spindle pole body in yeast) function and centrosomes participate in the control of cell cycle progression and cytokinesis (Doxsey, 2001; Hinchcliffe et al, 2001; Khodjakov and Rieder, 2001; Piel et al, 2001; Lange, 2002).
The presence of Orc2 at both centromeres and centrosomes suggests the possible involvement of Orc2 in linking DNA replication to control of centrosome copy number. Orc2-depleted cells showed multiple centrosome-like structures only during mitosis and not during interphase, suggesting that the centrosomes do not duplicate in the preceding interphase, but they either continue to duplicate in the absence of DNA replication or centrioles split in mitotic cells (Hut et al, 2003). The fact that we see cells with multiple mitotic asters as early as 24 h post Orc2 siRNA (Table I) suggests that centrosome copy number control may be defective in these cells. Mitotic centrosomes are sensitive to stress, suggesting that they are a part of a mechanism that halts mitotic progression or activates the spindle checkpoint when the fidelity of cell division is compromised. Orc2 may be a part of this mechanism. We are currently examining the integrity of spindle checkpoint mechanisms in Orc2-depleted cells.
Another possibility is that the extra centrosome-like structures and multipolar spindles are a result of Orc2 functioning directly as part of the centrosome. Recently, a mass spectrometric analysis of centrosome-associated proteins identified several DNA replication proteins, but they were not shown to localize there by other means (Andersen et al, 2003). The analysis, however, did not identify many known centrosome-associated proteins and did not identify Orc2 as a component of centrosomes.
The association with centrosomes of an important subunit of the initiator protein ORC suggests a direct link between chromosome duplication and segregation. Such a link also exists in bacteria where, following the initiation of DNA replication, the origins move from the middle to the quarters of the cell, the site of the septum in the next generation (Sherratt, 2003). Although the mechanism of how this occurs is not understood, the initiation of DNA replication must be linked to a structure that pulls the daughter chromosomes apart and places them at a critical mid-point for future cell division. Perhaps the link between initiator proteins in human cells with centrosomes, centromeres and the mid-plane of cell division reflects a common strategy with bacteria in coordinating replication and segregation of chromosomes.
Materials and methods
Cell culture and RNA interference
HeLa cells were grown in DMEM containing low glucose (Gibco/Life Technologies, Grand Island, NY) supplemented with penicillin–streptomycin and 10% fetal bovine serum (Hyclone, Logan, UT). MCF7, MCF10A, IMR-90 and MEFs were grown under standard conditions (ATCC). RNAi was carried out as described previously (Elbashir et al, 2001). The siRNAs from Orc2 (Orc2-1 UGCUCCU CUCAUGUGGGAU and Orc2-2 UCAUUGGUCAGUUGUCAUC), PCNA (CGGUGACACUCAGUAUGUC), Orc3 (GAGACUUGGGCGGU CAAAU) and control luciferase (CUUACGCUGAGUACUUCGA) (Elbashir et al, 2001) were synthesized by Dharmacon Inc. (Lafayette, CO). siRNA was delivered into the cells at a final concentration of 100 nM using oligofectamine (Invitrogen, Carlsbad, CA). Cells were transfected thrice at a gap of 24–30 h and analyzed for immunoblotting and IF.
Epitope-tagged Orc2 constructs
Human Orc2 cDNA was cloned into pEYFP-C3 with CMV promoter, (CLONTECH Laboratories Inc.) and into pCGT vector to generate T7-Orc2 for transient transfection studies.
Immunofluorescence and antibodies
Detailed protocols are presented in Supplementary Note 2. Fixation for IF was carried out as follows: cells were rinsed briefly in phosphate-buffered saline (PBS), pH 7.4, then either fixed for 15 min in 1.0% formaldehyde in PBS (pH 7.4) or for 7 min in cold methanol (−20oC), depending on the antibody or application. Antibody staining was performed as follows: primary antibodies were anti-Orc2 pAb205, anti-Orc2 mAb920, α-tubulin mAb, γ-tubulin mAb, anti-BrdU, anti-PCNA PC10 mAb, anti-MCM3 pAb738, anti-HP1α, anti-HP1β, anti-CAP-G pAb (Ono et al, 2003), anti-centromere, AnaC and anti-CENP-F. Immunoblots were performed with antibodies and dilutions indicated in Supplemental Materials using standard methods.
Flow cytometry
Flow cytometry using BrdU and PI as labels was conducted to study the DNA replication and DNA content. Cells were grown in medium with 10 μM BrdU for 10 min, collected and resuspended in PBS with 10% calf serum. Cells were fixed in chilled ethanol overnight and then washed in PBS with 10% calf serum. DNA was denatured in 2 N HCl for 30 min and subsequently washed in PBS with 10% calf serum and 0.5% Tween 20 (PBS+CS+T). Cells were incubated in anti-BrdU mouse mAb for 15 min at RT, washed in PBS+CS+T and incubated in FITC-conjugated anti-mouse IgG for 15 min. Cells were washed in PBS+CS+T, resuspended in PBS with 20 μg/ml of PI and 20 μg RNase A for 30 min at RT. BrdU-associated green emission (FITC, 530±20 nm band-pass filter) and DNA-associated red fluorescence for PI (620 nm long-pass filter) were measured by flow cytometry.
Metaphase spreads
Mitotic cells were collected by shake-off and washed in PBS. Cells were resuspended in 0.075 M KCl and incubated for 30 min at 37°C, and then centrifuged at 1000 rpm for 5 min. For IF procedures, the cells were cytospun onto acid-washed coverslips and fixed in 2% PF. For visualization of chromosomes or BrdU studies, cells were fixed in fresh fixative (3:1, methanol:acetic acid), left on ice for 10 min and collected by centrifugation. Fixation procedure was repeated thrice. The fixed cells were dropped on pre-cleaned slides and air-dried. Chromosomes were observed by PI or DAPI staining. BrdU studies on metaphase chromosomes were carried out as described (Prasanth et al, 2002). For antibody labeling on metaphase chromosomes, the cells grown on coverslips were lysed on the coverslip using 0.06 M KCl for 30 min at RT and fixed in 2% PF. Rest of the IF procedure was performed as described above.
Supplementary Material
Supplementary Movie 1
Supplementary Movie 2
Supplementary Movie 3
Supplementary Figure 1
Supplementary Figure 2
Supplementary Figure 3
Supplementary Figure 4
Supplementary Figure 5
Supplementary Figure 6
Supplementary Figure 7
Supplementary Figure 8
Supplementary Figure 9
Supplementary Figure 10
Supplementary Figure 11
Supplementary Figure 12
Supplementary Figure 13
Supplementary Figure 14
Online Supplemental Materials
Acknowledgments
We thank members of the Stillman laboratory for discussions and suggestions and Juan Méndez for critical reading of the manuscript. We thank Y Lazebnik and T Hirano for providing antibodies. We also thank Diane Esposito, So-Young Kim, Naoki Nakaya, Patrice Lassus, Masashi Narita and Pam McCloskey for suggestions and technical help and the graphic arts department for artwork and photography. This work was supported by a grant from the National Cancer Institute (CA13106).
References
- Allshire RC (1995) Elements of chromosome structure and function in fission yeast. Semin Cell Biol 6: 55–64 [Abstract] [Google Scholar]
- Andersen JS, Wilkinson CJ, Mayor T, Mortensen P, Nigg EA, Mann M (2003) Proteomic characterization of the human centrosome by protein correlation profiling. Nature 426: 570–574 [Abstract] [Google Scholar]
- Aparicio OM, Weinstein DM, Bell SP (1997) Components and dynamics of DNA replication complexes in S. cerevisiae: redistribution of MCM proteins and Cdc45p during S phase. Cell 91: 59–69 [Abstract] [Google Scholar]
- Bannister AJ, Zegerman P, Partridge JF, Miska EA, Thomas JO, Allshire RC, Kouzarides T (2001) Selective recognition of methylated lysine 9 on histone H3 by the HP1 chromo domain. Nature 410: 120–124 [Abstract] [Google Scholar]
- Bell SP, Dutta A (2002) DNA replication in eukaryotic cells. Annu Rev Biochem 71: 333–374 [Abstract] [Google Scholar]
- Bell SP, Kobayashi R, Stillman B (1993) Yeast origin recognition complex functions in transcription silencing and DNA replication. Science 262: 1844–1849 [Abstract] [Google Scholar]
- Bell SP, Stillman B (1992) ATP-dependent recognition of eukaryotic origins of DNA replication by a multiprotein complex. Nature 357: 128–134 [Abstract] [Google Scholar]
- Carpenter PB, Mueller PR, Dunphy WG (1996) Role for a Xenopus Orc2-related protein in controlling DNA replication. Nature 379: 357–360 [Abstract] [Google Scholar]
- Chesnokov IN, Chesnokova ON, Botchan M (2003) A cytokinetic function of Drosophila ORC6 protein resides in a domain distinct from its replication activity. Proc Natl Acad Sci USA 100: 9150–9155 [Europe PMC free article] [Abstract] [Google Scholar]
- Cheutin T, McNairn AJ, Jenuwein T, Gilbert DM, Singh PB, Misteli T (2003) Maintenance of stable heterochromatin domains by dynamic HP1 binding. Science 299: 721–725 [Abstract] [Google Scholar]
- Christensen TW, Tye BK (2003) Drosophila MCM10 interacts with members of the prereplication complex and is required for proper chromosome condensation. Mol Biol Cell 14: 2206–2215 [Europe PMC free article] [Abstract] [Google Scholar]
- Craig JM, Earle E, Canham P, Wong LH, Anderson M, Choo KH (2003) Analysis of mammalian proteins involved in chromatin modification reveals new metaphase centromeric proteins and distinct chromosomal distribution patterns. Hum Mol Genet 12: 3109–3121 [Abstract] [Google Scholar]
- DePamphilis ML (2003) The ‘ORC cycle': a novel pathway for regulating eukaryotic DNA replication. Gene 310: 1–15 [Abstract] [Google Scholar]
- Dhar SK, Delmolino L, Dutta A (2001a) Architecture of the human origin recognition complex. J Biol Chem 276: 29067–29071 [Abstract] [Google Scholar]
- Dhar SK, Yoshida K, Machida Y, Khaira P, Chaudhuri B, Wohlschlegel JA, Leffak M, Yates J, Dutta A (2001b) Replication from oriP of Epstein–Barr virus requires human ORC and is inhibited by geminin. Cell 106: 287–296 [Abstract] [Google Scholar]
- Diffley JF, Cocker JH, Dowell SJ, Rowley A (1994) Two steps in the assembly of complexes at yeast replication origins in vivo. Cell 78: 303–316 [Abstract] [Google Scholar]
- Dimitrova DS, Prokhorova TA, Blow JJ, Todorov IT, Gilbert DM (2002) Mammalian nuclei become licensed for DNA replication during late telophase. J Cell Sci 115: 51–59 [Europe PMC free article] [Abstract] [Google Scholar]
- Dimitrova DS, Todorov IT, Melendy T, Gilbert DM (1999) Mcm2, but not RPA, is a component of the mammalian early G1-phase prereplication complex. J Cell Biol 146: 709–722 [Europe PMC free article] [Abstract] [Google Scholar]
- Doxsey SJ (2001) Centrosomes as command centres for cellular control. Nat Cell Biol 3: E105–E108 [Abstract] [Google Scholar]
- Dutta A, Bell SP (1997) Initiation of DNA replication in eukaryotic cells. Annu Rev Cell Dev Biol 13: 293–332 [Abstract] [Google Scholar]
- Ekwall K, Javerzat JP, Lorentz A, Schmidt H, Cranston G, Allshire R (1995) The chromodomain protein Swi6: a key component at fission yeast centromeres. Science 269: 1429–1431 [Abstract] [Google Scholar]
- Elbashir SM, Harborth J, Lendeckel W, Yalcin A, Weber K, Tuschl T (2001) Duplexes of 21-nucleotide RNAs mediate RNA interference in cultured mammalian cells. Nature 411: 494–498 [Abstract] [Google Scholar]
- Festenstein R, Pagakis SN, Hiragami K, Lyon D, Verreault A, Sekkali B, Kioussis D (2003) Modulation of heterochromatin protein 1 dynamics in primary mammalian cells. Science 299: 719–721 [Abstract] [Google Scholar]
- Firestein R, Cui X, Huie P, Cleary ML (2000) Set domain-dependent regulation of transcriptional silencing and growth control by SUV39H1, a mammalian ortholog of Drosophila Su(var)3-9. Mol Cell Biol 20: 4900–4909 [Europe PMC free article] [Abstract] [Google Scholar]
- Gerbi SA, Bielinsky AK (2002) DNA replication and chromatin. Curr Opin Genet Dev 12: 243–248 [Abstract] [Google Scholar]
- Gillespie PJ, Li A, Blow JJ (2001) Reconstitution of licensed replication origins on Xenopus sperm nuclei using purified proteins. BMC Biochem 2: 15. [Europe PMC free article] [Abstract] [Google Scholar]
- Grewal SI, Moazed D (2003) Heterochromatin and epigenetic control of gene expression. Science 301: 798–802 [Abstract] [Google Scholar]
- Hinchcliffe EH, Miller FJ, Cham M, Khodjakov A, Sluder G (2001) Requirement of a centrosomal activity for cell cycle progression through G1 into S phase. Science 291: 1547–1550 [Abstract] [Google Scholar]
- Hua XH, Newport J (1998) Identification of a preinitiation step in DNA replication that is independent of origin recognition complex and cdc6, but dependent on cdk2. J Cell Biol 140: 271–281 [Europe PMC free article] [Abstract] [Google Scholar]
- Huang DW, Fanti L, Pak DT, Botchan MR, Pimpinelli S, Kellum R (1998) Distinct cytoplasmic and nuclear fractions of Drosophila heterochromatin protein 1: their phosphorylation levels and associations with origin recognition complex proteins. J Cell Biol 142: 307–318 [Europe PMC free article] [Abstract] [Google Scholar]
- Hut HM, Lemstra W, Blaauw EH, Van Cappellen GW, Kampinga HH, Sibon OC (2003) Centrosomes split in the presence of impaired DNA integrity during mitosis. Mol Biol Cell 14: 1993–2004 [Europe PMC free article] [Abstract] [Google Scholar]
- Kellum R (2003) HP1 complexes and heterochromatin assembly. Curr Top Microbiol Immunol 274: 53–77 [Abstract] [Google Scholar]
- Kellum R, Alberts BM (1995) Heterochromatin protein 1 is required for correct chromosome segregation in Drosophila embryos. J Cell Sci 108: 1419–1431 [Abstract] [Google Scholar]
- Kelly TJ, Brown GW (2000) Regulation of chromosome replication. Annu Rev Biochem 69: 829–880 [Abstract] [Google Scholar]
- Kelly TJ, Jallepalli PV, Clyne RK (1994) Replication and transcription. Silence of the ORCs. Curr Biol 4: 238–241 [Abstract] [Google Scholar]
- Khodjakov A, Rieder CL (2001) Centrosomes enhance the fidelity of cytokinesis in vertebrates and are required for cell cycle progression. J Cell Biol 153: 237–242 [Europe PMC free article] [Abstract] [Google Scholar]
- Kiely J, Haase SB, Russell P, Leatherwood J (2000) Functions of fission yeast orp2 in DNA replication and checkpoint control. Genetics 154: 599–607 [Europe PMC free article] [Abstract] [Google Scholar]
- Krause SA, Loupart ML, Vass S, Schoenfelder S, Harrison S, Heck MM (2001) Loss of cell cycle checkpoint control in Drosophila Rfc4 mutants. Mol Cell Biol 21: 5156–5168 [Europe PMC free article] [Abstract] [Google Scholar]
- Kreitz S, Ritzi M, Baack M, Knippers R (2001) The human origin recognition complex protein 1 dissociates from chromatin during S phase in HeLa cells. J Biol Chem 276: 6337–6342 [Abstract] [Google Scholar]
- Lange BM (2002) Integration of the centrosome in cell cycle control, stress response and signal transduction pathways. Curr Opin Cell Biol 14: 35–43 [Abstract] [Google Scholar]
- Loo S, Fox CA, Rine J, Kobayashi R, Stillman B, Bell S (1995) The origin recognition complex in silencing, cell cycle progression, and DNA replication. Mol Biol Cell 6: 741–756 [Europe PMC free article] [Abstract] [Google Scholar]
- Loupart ML, Krause SA, Heck MS (2000) Aberrant replication timing induces defective chromosome condensation in Drosophila ORC2 mutants. Curr Biol 10: 1547–1556 [Abstract] [Google Scholar]
- McHugh B, Heck MM (2003) Regulation of chromosome condensation and segregation. Curr Opin Genet Dev 13: 185–190 [Abstract] [Google Scholar]
- Méndez J, Stillman B (2000) Chromatin association of human origin recognition complex, cdc6, and minichromosome maintenance proteins during the cell cycle: assembly of prereplication complexes in late mitosis. Mol Cell Biol 20: 8602–8612 [Europe PMC free article] [Abstract] [Google Scholar]
- Méndez J, Stillman B (2003) Perpetuating the double helix: molecular machines at eukaryotic DNA replication origins. BioEssays 25: 1158–1167 [Abstract] [Google Scholar]
- Méndez J, Zou-Yang XH, Kim SY, Hidaka M, Tansey WP, Stillman B (2002) Human origin recognition complex large subunit is degraded by ubiquitin-mediated proteolysis after initiation of DNA replication. Mol Cell 9: 481–491 [Abstract] [Google Scholar]
- Natale DA, Li CJ, Sun WH, DePamphilis ML (2000) Selective instability of Orc1 protein accounts for the absence of functional origin recognition complexes during the M–G(1) transition in mammals. EMBO J 19: 2728–2738 [Europe PMC free article] [Abstract] [Google Scholar]
- Nielsen AL, Ortiz JA, You J, Oulad-Abdelghani M, Khechumian R, Gansmuller A, Chambon P, Losson R (1999) Interaction with members of the heterochromatin protein 1 (HP1) family and histone deacetylation are differentially involved in transcriptional silencing by members of the TIF1 family. EMBO J 18: 6385–6395 [Europe PMC free article] [Abstract] [Google Scholar]
- O'Keefe RT, Henderson SC, Spector DL (1992) Dynamic organization of DNA replication in mammalian cell nuclei: spatially and temporally defined replication of chromosome-specific alpha-satellite DNA sequences. J Cell Biol 116: 1095–1110 [Europe PMC free article] [Abstract] [Google Scholar]
- Ohta S, Tatsumi Y, Fujita M, Tsurimoto T, Obuse C (2003) The ORC1 cycle in human cells: II. Dynamic changes in the human ORC complex during the cell cycle. J Biol Chem 278: 41535–41540 [Abstract] [Google Scholar]
- Ono T, Losada A, Hirano M, Myers MP, Neuwald AF, Hirano T (2003) Differential contributions of condensin I and condensin II to mitotic chromosome architecture in vertebrate cells. Cell 115: 109–121 [Abstract] [Google Scholar]
- Pak DT, Pflumm M, Chesnokov I, Huang DW, Kellum R, Marr J, Romanowski P, Botchan MR (1997) Association of the origin recognition complex with heterochromatin and HP1 in higher eukaryotes. Cell 91: 311–323 [Abstract] [Google Scholar]
- Pflumm MF, Botchan MR (2001) Orc mutants arrest in metaphase with abnormally condensed chromosomes. Development 128: 1697–1707 [Abstract] [Google Scholar]
- Piel M, Nordberg J, Euteneuer U, Bornens M (2001) Centrosome-dependent exit of cytokinesis in animal cells. Science 291: 1550–1553 [Abstract] [Google Scholar]
- Prasanth SG, Mendez J, Prasanth KV, Stillman B (2004) Dynamics of pre-replication complex proteins during the cell division cycle. Philos Trans Biol Sci 359: 7–16 [Europe PMC free article] [Abstract] [Google Scholar]
- Prasanth SG, Prasanth KV, Stillman B (2002) Orc6 involved in DNA replication, chromosome segregation, and cytokinesis. Science 297: 1026–1031 [Abstract] [Google Scholar]
- Rieder CL, Cole RW, Khodjakov A, Sluder G (1995) The checkpoint delaying anaphase in response to chromosome monoorientation is mediated by an inhibitory signal produced by unattached kinetochores. J Cell Biol 130: 941–948 [Europe PMC free article] [Abstract] [Google Scholar]
- Ritzi M, Baack M, Musahl C, Romanowski P, Laskey RA, Knippers R (1998) Human minichromosome maintenance proteins and human origin recognition complex 2 protein on chromatin. J Biol Chem 273: 24543–24549 [Abstract] [Google Scholar]
- Romanowski P, Madine MA, Rowles A, Blow JJ, Laskey RA (1996) The Xenopus origin recognition complex is essential for DNA replication and MCM binding to chromatin. Curr Biol 6: 1416–1425 [Abstract] [Google Scholar]
- Rowles A, Tada S, Blow JJ (1999) Changes in association of the Xenopus origin recognition complex with chromatin on licensing of replication origins. J Cell Sci 112: 2011–2018 [Europe PMC free article] [Abstract] [Google Scholar]
- Shareef MM, Badugu R, Kellum R (2003) HP1/ORC complex and heterochromatin assembly. Genetica 117: 127–134 [Abstract] [Google Scholar]
- Sherratt DJ (2003) Bacterial chromosome dynamics. Science 301: 780–785 [Abstract] [Google Scholar]
- Shimada K, Pasero P, Gasser SM (2002) ORC and the intra-S-phase checkpoint: a threshold regulates Rad53p activation in S phase. Genes Dev 16: 3236–3252 [Europe PMC free article] [Abstract] [Google Scholar]
- Sun WH, Coleman TR, DePamphilis ML (2002) Cell cycle-dependent regulation of the association between origin recognition proteins and somatic cell chromatin. EMBO J 21: 1437–1446 [Europe PMC free article] [Abstract] [Google Scholar]
- Tatsumi Y, Ohta S, Kimura H, Tsurimoto T, Obuse C (2003) The ORC1 cycle in human cells: I. Cell cycle-regulated oscillation of human ORC1. J Biol Chem 278: 41528–41534 [Abstract] [Google Scholar]
- Triolo T, Sternglanz R (1996) Role of interactions between the origin recognition complex and SIR1 in transcriptional silencing. Nature 381: 251–253 [Abstract] [Google Scholar]
- Tye BK (1999) MCM proteins in DNA replication. Annu Rev Biochem 68: 649–686 [Abstract] [Google Scholar]
- Vashee S, Simancek P, Challberg MD, Kelly TJ (2001) Assembly of the human origin recognition complex. J Biol Chem 276: 26666–26673 [Abstract] [Google Scholar]
- Weinreich M, Liang C, Stillman B (1999) The Cdc6p nucleotide-binding motif is required for loading mcm proteins onto chromatin. Proc Natl Acad Sci USA 96: 441–446 [Europe PMC free article] [Abstract] [Google Scholar]
- Yang ZY, Guo J, Li N, Qian M, Wang SN, Zhu XL (2003) Mitosin/CENP-F is a conserved kinetochore protein subjected to cytoplasmic dynein-mediated poleward transport. Cell Res 13: 275–283 [Abstract] [Google Scholar]
Articles from The EMBO Journal are provided here courtesy of Nature Publishing Group
Full text links
Read article at publisher's site: https://doi.org/10.1038/sj.emboj.7600255
Read article for free, from open access legal sources, via Unpaywall:
http://emboj.embopress.org/content/23/13/2651.full.pdf
Citations & impact
Impact metrics
Citations of article over time
Alternative metrics
Smart citations by scite.ai
Explore citation contexts and check if this article has been
supported or disputed.
https://scite.ai/reports/10.1038/sj.emboj.7600255
Article citations
ORC1 enhances repressive epigenetic modifications on HIV-1 LTR to promote HIV-1 latency.
J Virol, 98(8):e0003524, 31 Jul 2024
Cited by: 0 articles | PMID: 39082875
In silico functional, structural and pathogenicity analysis of missense single nucleotide polymorphisms in human MCM6 gene.
Sci Rep, 14(1):11607, 21 May 2024
Cited by: 0 articles | PMID: 38773180 | PMCID: PMC11109216
Mode of SUV420H2 heterochromatin localization through multiple HP1 binding motifs in the heterochromatic targeting module.
Genes Cells, 29(5):361-379, 25 Feb 2024
Cited by: 1 article | PMID: 38403935
Chromatin's Influence on Pre-Replication Complex Assembly and Function.
Biology (Basel), 13(3):152, 27 Feb 2024
Cited by: 0 articles | PMID: 38534422 | PMCID: PMC10968542
Review Free full text in Europe PMC
The nucleolar protein GNL3 prevents resection of stalled replication forks.
EMBO Rep, 24(12):e57585, 15 Nov 2023
Cited by: 1 article | PMID: 37965896 | PMCID: PMC10702807
Go to all (189) article citations
Other citations
Data
Data behind the article
This data has been text mined from the article, or deposited into data resources.
BioStudies: supplemental material and supporting data
Similar Articles
To arrive at the top five similar articles we use a word-weighted algorithm to compare words from the Title and Abstract of each citation.
Human origin recognition complex is essential for HP1 binding to chromatin and heterochromatin organization.
Proc Natl Acad Sci U S A, 107(34):15093-15098, 05 Aug 2010
Cited by: 95 articles | PMID: 20689044 | PMCID: PMC2930523
Cell cycle behavior of human HP1 subtypes: distinct molecular domains of HP1 are required for their centromeric localization during interphase and metaphase.
J Cell Sci, 116(pt 16):3327-3338, 02 Jul 2003
Cited by: 97 articles | PMID: 12840071
A CAF-1 dependent pool of HP1 during heterochromatin duplication.
EMBO J, 23(17):3516-3526, 12 Aug 2004
Cited by: 131 articles | PMID: 15306854 | PMCID: PMC516634
Replication of heterochromatin: insights into mechanisms of epigenetic inheritance.
Chromosoma, 114(6):389-402, 15 Nov 2005
Cited by: 37 articles | PMID: 16220346
Review
Funding
Funders who supported this work.
NCI NIH HHS (2)
Grant ID: P01 CA013106
Grant ID: CA13106