Abstract
Free full text

Inflammation-activated Protein Kinases as Targets for Drug Development
Abstract
Given the prevalence and debilitating nature of chronic inflammatory diseases, there is a never-ending quest to identify novel targets for the rational development of antiinflammatory drugs. The major signaling pathway that controls inflammation-associated gene expression is the one which leads to activation of transcription factor nuclear factor-κB. Therefore, inhibitors of the kinase responsible for nuclear factor-κB activation, IκB kinase, are expected to have potent antiinflammatory activity. Indeed, our results with cell type−specific inactivation of the β-catalytic subunit of IκB kinase are by and large consistent with this assertion. In addition to IκB kinase and nuclear factor-κB, the expression of certain proinflammatory cytokines, such as interleukin-1 and tumor necrosis factor-α, is dependent on mitogen-activated protein kinases. Therefore, considerable attention has also been given to mitogen-activated protein kinases as likely targets for the development of novel antiinflammatory therapeutics. Preliminary preclinical data suggest that inhibitors that target all these pathways exhibit antiinflammatory activity. This review focuses on the possible mechanisms through which such inhibitors may interfere with inflammation and some of the complications that may be associated with their use.
Chronic inflammatory diseases, such as rheumatoid arthritis, psoriatic arthritis, inflammatory bowel disease, and chronic obstructive pulmonary disease, are highly debilitating diseases that affect a large segment of our population. It has become apparent that even metabolic diseases, such as type 2 diabetes, and certain cardiovascular diseases, for instance, atherosclerosis, should also be considered inflammatory in nature and origin. Thus, inflammation seems to be at the root of almost all chronic diseases (cancer notwithstanding), and huge efforts and resources are dedicated to the development of antiinflammatory drugs. However, because of its very nature—a chronic disorder that in its initial and even more advanced stages is frequently not life-threatening—chronic inflammation presents a challenge for therapeutic intervention, because one needs to develop drugs that are efficacious, relatively free of side effects, and can be used effectively for a long time. Therefore, there is a never-ending quest for new targets for the development of antiinflammatory drugs that one hopes will be highly specific, potent, and free of side effects. The logical identification of such targets depends on better understanding of the signaling pathways involved in the initiation and maintenance of inflammation and the availability of target validation technology, such as targeted mutagenesis in mice and gene silencing mediated by small inhibitory RNA.
ROLE OF NUCLEAR FACTOR-κB ACTIVATION IN INFLAMMATION
Considerable evidence indicates that the primary signaling pathway involved in the initiation and amplification of inflammatory responses is the one that leads to activation of nuclear factor (NF)-κB transcription factors (1, 2). Although many different receptors can lead to activation (i.e., nuclear translocation) of NF-κB, they all rely on two major signaling pathways, known as the classic (or canonical) and the alternative NF-κB signaling pathways (Figure 1) (3, 4). The first pathway primarily affects diverse NF-κB dimers, such as the most common one, the RelA (p65)–p50 heterodimer, through phosphorylation-induced proteolysis of the kinase responsible for NF-κB activation, IκB kinase (IKK). The alternative pathway affects only the activation of RelB–p52 dimers, through phosphorylation-induced processing of the NF-κB2 (p100) precursor protein (5). Ample evidence suggests that it is the classic pathway, which relies on the IKKβ catalytic subunit and IKKγ regulatory subunit of the IKK complex, that is most important for the initiation and propagation of inflammatory responses (3, 6). The alternative pathway, by contrast, is most important for secondary lymphoid organ development and adaptive immunity (4).
ROLE OF MITOGEN-ACTIVATED PROTEIN KINASE ACTIVATION IN INFLAMMATION
Despite its central role, it is unlikely that the mere activation of NF-κB is sufficient for transcriptional activation or induction of any single NF-κB target gene that is involved in the initiation of inflammatory responses. For most promoters that have been critically analyzed, for instance, the IFN-β promoter (7), NF-κB requires assistance from other sequence-specific transcription factors. Often the activity of such transcription factors, for instance, members of the activator protein (AP)-1 family (8), is dependent on their phosphorylation by mitogen-activated protein kinases (MAPKs) or MAPK-activated kinases. A classic MAPK cascade is composed of an MAPK, the kinase that activates the MAPK through phosphorylation on serine and tyrosine residues (called a MAPK kinase, MKK, MAPKK, or MAP2K), and the kinase that activates the MKK (called a MKK kinase, MEKK, MAPKKK, or MAP3K; Figure 2) (9). The MAP3Ks are activated through a variety of mechanisms, most of which are not entirely clear, in response to engagement of cell surface receptors. Thus, the MAP3Ks provide the stimulus specificity, whereas the MAPKs carry out the effector functions of each cascade, either though direct phosphorylation of effector proteins or via the activation of subordinate kinases, the MAPK-activated kinases.
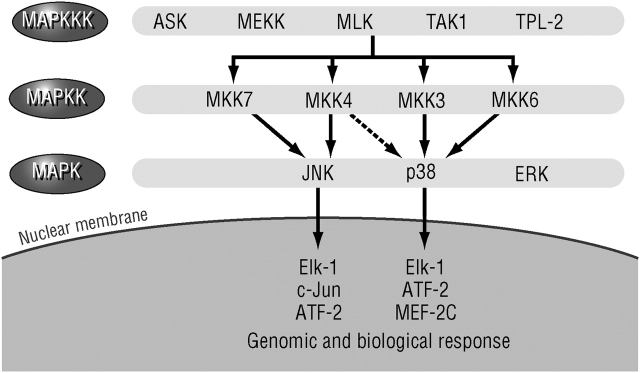
Mitogen-activated protein kinase (MAPK) signaling pathways. ASK = apoptosis signal–regulating kinase; ATF = activating transcription factor; Elk-1 = ETS-like gene-1; ERK = extracellular signal–regulated kinase; JNK = Jun-N terminal kinase; MAPK = mitogen-activated protein kinase; MAPKK = MAPK kinase; MAPKKK = MAPK kinase kinase; MEF-2C = myocyte-specific enhancer factor 2C; MEKK = MAPK/ERK kinase kinase; MKK = MAPK kinase; MLK = mixed lineage kinase; TAK = TGF-β1-associated kinase; TPL = tumor progression locus-2 (MAPKKK-8).
Several distinct MAPK cascades have been identified in mammals, including humans. The three most common are the extracellular signal–regulated kinase (ERK), the Jun-N terminal kinase (JNK), and the p38 MAPK cascades. Each cascade leads to activation of several closely related MAPK enzymes, for instance, ERK1 and ERK2 or JNK1, JNK2, and JNK3, which can be activated by two different MKKs, such as MAPK/ERK kinase (MEK)1 and MEK2 for the ERK cascade and MKK4 and MKK7 for the JNKs (9). The MKKs, however, can be activated by a myriad of MAP3Ks. Gene disruption experiments reviewed by Chang and Karin (9) indicate that each of the MAPK cascades has a distinct function, although a given stimulus, for example, tumor necrosis factor (TNF)-α or interleukin (IL)-1, can activate to variable extents all three MAPK cascades. Gene disruption experiments also reveal that the response specificity (i.e., the type of stimulus that activates any given cascade) is determined by the MAP3K (10).
Although quite a few cell surface receptors can lead to the activation of IKK and MAPK, those that are most relevant to inflammation are the type 1 TNF receptor (TNFR1), the IL-1 receptor (IL-1R), and Toll-like receptors (TLRs). TNFR1 transmits its signals through the recruitment of adaptor proteins called the Fas-associated death domain protein and the TNF receptor−associated death domain protein (11). Whereas the Fas-associated death domain protein is important for activation of caspase-8 and the induction of programmed cell death, the TNF receptor−associated death domain protein is required for most other TNFR1-mediated functions, also including induction of programmed cell death. The TNF receptor−associated death domain protein (TRADD) mediates Fas-associated death domain protein (FADD) recruitment and the recruitment of receptor-interacting protein-1 (RIP-1) and related protein kinases and the signal-transducing proteins TNF receptor−associated factor 2 (TRAF2) and TRAF5, all of which participate in the activation of IKK and MAPKs (12, 13). The function of TRAF2 and TRAF5 is somewhat redundant, and both of them need to be deleted for complete inhibition of TNF-α−induced MAPK and IKK activation (14). Another member of the TRAF family, TRAF6, plays a critical role in IL-1R and TLR signaling (15). Both IL-1R and the TLRs belong to the same superfamily of Toll and IL-1 receptor (TIR) domain–containing proteins (16). These receptors signal through recruitment of TIR domain–containing adaptor proteins, such as MyD88, TIRAP/MAL (TIR domain–containing activator protein/MyD88 adaptor-like), TRIF (TIR domain–containing adaptor inducing interferon-β), and TRAM (TRIF-related adaptor molecule). The adaptors then lead to recruitment and activation of complexes between TRAF6 and IL-1 receptor−associated kinase. In all three cases (TNFR1, IL-1R, and TLRs), it is the TRAF proteins that are responsible for activation of the MAPK and IKK effector pathways (11). The mechanisms by which the TRAFs lead to MAPK and IKK activation are still a matter of debate and are not discussed here.
SUITABILITY OF THE IKK AND MAPK CASCADES AS DRUG TARGETS
IKK
The IKK complex contains two catalytic subunits, IKKα and IKKβ, and a regulatory subunit, IKKγ/NEMO (NF-κB essential modulator) (17). Targeted mutagenesis in mice indicates that IKKβ is required for NF-κB activation in response to proinflammatory stimuli, such as TNF-α, IL-1, and LPS (18–20). IKKα, on the other hand, is not essential for NF-κB activation by these stimuli (21) and is instead required for activation of the alternative NF-κB pathway, which as explained previously is based on induced processing of NF-κB2 (p100) (5). Using mice harboring a conditional Ikkβ “floxed” allele, we were able to inactivate and delete IKKβ in specific cell types and analyze its contribution to various inflammatory responses. Using this approach we found that deletion of IKKβ in intestinal epithelial cells (enterocytes) prevented the onset of multiple organ failure in response to gut ischemia–reperfusion (6). However, the deletion of IKKβ in enterocytes also greatly increased their susceptibility to various apoptosis-inducing stimuli (22, 23). Because the apoptosis of enterocytes disrupts the barrier function of the intestinal mucosa, the deletion of IKKβ in these cells increased the colonic inflammation caused by oral administration of dextran sulfate salt, which is generated by invasion of enteric bacteria into the lamina propria (23). We also found that deletion of IKKβ in myeloid cells (macrophages and neutrophils) or in all hematopoietic cells inhibited the production of inflammatory mediators in response to LPS (20) and reduced the severity of dextran sulfate salt-induced colitis. (24) At the same time, this deletion greatly increased the susceptibility of macrophages to LPS-induced apoptosis (20) and, as a result, increased the secretion of mature IL-1β, the processing of which depends on activation of caspase-1 (F. R. Greten and M. Karin, unpublished data). Deletion of IKKβ in hematopoietic cells also prevented inflammation-induced bone loss caused by injection of LPS into the joints of mice (25).
Inflammation-induced bone loss is also prevented by a peptide inhibitor that disrupts the interaction between IKKβ and IKKγ (26). We extensively analyzed how the deletion of IKKβ in hematopoietic cells prevents inflammation-induced bone loss and identified two relevant mechanisms. First, the loss of IKKβ in bone marrow progenitors prevents terminal osteoclast differentiation. Second, macrophages and osteoclast precursors that are IKKβ deficient become highly susceptible to TNF-α−induced apoptosis, resulting in the elimination of activated osteoclasts (25). When TNF-α−induced apoptosis was prevented by deleting the gene for TNFR1, the inhibitory effect of the IKKβ deletion on inflammation-induced bone loss was considerably reduced.
The ability of NF-κB to suppress apoptosis, especially in response to TNF-α, is well established (27). As discussed above, this can result in a beneficial effect in the case of inflammation-induced bone loss caused by localized exposure to LPS. However, at the same time, by increasing the susceptibility of macrophages to LPS- or TNF-α−induced apoptosis and thereby increasing the release of mature IL-1β, the deletion of IKKβ in hematopoietic cells increases the acute inflammatory response (septic shock) caused by systemic exposure to LPS.
Of course, in real life situations, that is, in a patient treated with an IKKβ inhibitor, the final outcome may depend on the extent of the IKKβ inhibition that is required for prevention of inflammation and the nature of the inflammatory disorder being treated. Small-molecule inhibitors of IKKβ have been developed, but at this point there is only a limited amount of information regarding their efficacy and safety in experimental models of inflammation (28). So far, such inhibitors have been shown to be effective in mouse models of short-term collagen-induced arthritis and dextran sulfate salt (DSS)–induced colitis (28).
ERK
Compared with the MAPK cascades leading to p38 MAPK and JNK activation, there is relatively little information about the role of the ERK cascade in inflammation (Table 1). However, one publication indicates that the MAP3K Tpl2/Cot is responsible for ERK activation in response to bacterial endotoxin (LPS) in macrophages (29). The knockout of Tpl2 abolished ERK activation by LPS, an agonist of TLR4 (16) and, of most importance, inhibited the induction of TNF-α release (29). Further investigation revealed that the inhibition of ERK activation or the deletion of Tpl2 prevented the transport of TNF-α mRNA from the nucleus to the cytoplasm but had no effect on transcription of the Tnfα gene or the stabilization of TNF-α mRNA (29). Although the exact substrate for ERK that controls the nuclear export of TNF-α mRNA remains to be identified, these interesting findings suggest that inhibition of ERK activity may offer a unique strategy for inhibition of TNF-α production. Because TNF-α is a major mediator of chronic inflammation, inhibition of ERK represents a novel means of therapeutic intervention.
TABLE 1.
Mitogen-activated protein kinase signaling and its role in acute inflammation (regulation of tumor necrosis factor-α synthesis)
MAPK | ERK | JNK | p38 |
---|---|---|---|
MKK | MEK1/2 | MKK4/7 | MKK3/6 |
Inhibitor | PDO98059 | SP600025 | SB202190 |
Role in acute inflammation | Transport of TNF-α mRNA from nucleus to cytoplasm | Increased Tnfα gene transcription | Stabilization and translation of TNF-α mRNA |
Definition of abbreviations: ERK = extracellular signal-regulated kinase; JNK = Jun-N terminal kinase; MAPK = mitogen-activated protein kinase; MKK = MAPK kinase; TNF = tumor necrosis factor.
No direct ERK inhibitors have been reported to date, but several inhibitors have been described that interfere with the activity of the MKKs that act upstream of the ERKs and downstream of the relevant MAP3Ks, MEK1 and MEK2. These compounds, including PD098059 (30) and U0126 (31), are effective inhibitors of ERK activation. In light of the results discussed above, it would be worthwhile to test their effectiveness in animal models of chronic and acute inflammation. More recently it was found that the activation of Tpl2 and the subsequent activation of ERK in LPS-treated macrophages are dependent on the activation of IKKβ (32). It was observed that activation of Tpl2 depends on its dissociation from p105, the precursor for the NF-κB subunit p50 (32). As the processing of p105 to p50 is IKKβ dependent, IKKβ inhibitors can also inhibit the activation of Tpl2 and the subsequent activation of ERK by LPS. It would, therefore, be of interest to evaluate which of the therapeutic effects of IKK inhibitors in the mouse model of collagen-induced arthritis (33) are due to inhibition of NF-κB activation and which are due to inhibition of ERK activation.
JNK
Compared with ERK, JNK activity is more strongly induced in response to proinflammatory stimuli, and there is preliminary evidence that inhibition of JNK activity can retard or even prevent tissue damage in animal models of rheumatoid arthritis (34).
The JNKs were first identified by their ability to phosphorylate and, thereby, activate the transcriptional potential of c-Jun, a critical component of the AP-1 transcription factor (35). However, it is quite obvious that in addition to c-Jun, the JNKs can stimulate the activity of other transcription factors (36, 37) and other target proteins (10, 38). Although not as critical as NF-κB, AP-1 and related transcription factors, such as activating transcription factor-2 (the activity of which is also stimulated by JNK-mediated phosphorylation), play an important role in the inflammatory response through their ability to contribute to the transcriptional activation of important cytokine genes, such as those that encode TNF-α and IFN-β (Table 1) (8). In addition, AP-1 activity is required for the induction of matrix-degrading enzymes, such as collagenase (39). Besides being involved in the induction of TNF-α gene expression, AP-1 activity is induced by TNF-α (40), and JNK activity is required for this response (34).
Several different JNK inhibitors have been identified through high-throughput screening, and at least one of them, SP6000125, has been tested in a rat model of rheumatoid arthritis, where it reduced not only inflammation (paw swelling) but also tissue damage (34). The reduction in tissue damage was attributed to inhibition of collagenase and stromalysin expression, an effect that correlates with reduced c-Jun N-terminal phosphorylation and TNF-α−induced AP-1 activity (34). Similar findings were obtained with mice deficient in JNK1, the major JNK isoform responsible for c-Jun phosphorylation and AP-1 activation (34), thereby validating the results obtained with the low molecular weight JNK inhibitor. Interestingly, JNK1-deficient mice are also resistant to obesity-induced insulin resistance, a metabolic disorder thought to be caused by low-grade inflammation that is elicited by fat deposition (41). Whereas NF-κB activation confers resistance to apoptosis (25), the sustained activation of JNK, which is seen on inhibition of NF-κB activity, contributes to the apoptotic response (42, 43). Thus, JNK inhibition may alleviate one of the adverse side effects that could be associated with the use of IKKβ inhibitors. Indeed, reduced JNK activity was shown to protect mice from fulminant liver failure, a condition that accompanies severe liver inflammation and is exacerbated by IKKβ inhibition (43).
We have examined how many of the LPS-inducible genes in macrophages are JNK dependent. Whereas most LPS-inducible genes are IKKβ dependent, only a small percentage of them are sensitive to inhibition of JNK (M. G. Ruocco and J. M. Park, unpublished results). Of importance, however, the JNK-dependent genes include TNF-α and several other members of the TNF family, such as Fas ligand, an important death-inducing cytokine (M. G. Ruocco and J. M. Park, unpublished results).
p38 MAPK
p38 MAPK was first identified as an IL-1–activated kinase (44) and as an LPS-activated kinase (45). Therefore, from the beginning it was assumed that it plays an important role in inflammation. Indeed, early support for the critical role of p38 in inflammation was derived from studies that identified p38 as a critical target for a group of novel antiinflammatory drugs, a prototype of which is SB202190 (46).
It is important to note that the p38 inhibitors are potent inhibitors of LPS-mediated TNF-α production by macrophages (46). However, the mechanism through which p38 contributes to TNF-α production is not entirely clear. It is almost certain that p38 activity is not required for transcriptional activation of the Tnfα gene. Yet, because TNF-α is an important and central mediator of inflammation, its synthesis is subject to intricate control. In addition to transcriptional activation, the mRNA for TNF-α, which is inherently short-lived in nonstimulated cells, becomes stabilized in response to cell stimulation, but p38 activity does not appear to be required for this process either. In addition, p38 does not seem to be involved in the transport of TNF-α mRNA from the nucleus to the cytoplasm, a process that depends on ERK activity instead. Most likely, p38 activity is required for initiation of TNF-α mRNA translation (Table 1) (46). However, the mechanism through which p38 contributes to activation of TNF-α mRNA translation is unknown. Nevertheless, the ability of p38 inhibitors to block TNF-α synthesis can be exploited in the treatment of inflammatory diseases, and p38 inhibitors have been shown to inhibit the development of rheumatoid arthritis in small-animal models (47). It is not entirely clear, however, whether the therapeutic effect of such inhibitors is solely dependent on inhibition of TNF-α production or whether p38 also contributes to other important processes. For instance, we have found that inhibition of p38 activity prevents the transcriptional activation of both the Il-1α and Il-1β genes, encoding the two different forms of IL-1, in LPS-stimulated macrophages (48). As discussed below, just like IKKβ, p38α is required for maintaining the viability of activated macrophages (20, 48).
PITFALLS AND BENEFITS OF TARGETING THE IKK AND MAPK CASCADES
In addition to their roles in expression of cytokines and other genes involved in inflammation and tissue remodeling, the IKK and MAPK cascades have other important biological functions, which can either complicate the outcome of their inhibition or generate additional benefits. Of most importance, these signaling cascades are involved in the control of cell survival, especially in macrophages, which are central regulators and effectors of inflammatory responses. Inhibition of either IKKβ or p38 activities greatly increases the susceptibility of macrophages to the induction of apoptosis in response to activation of TLR4 (20, 48). Although induction of macrophage apoptosis can accelerate the resolution of inflammation in some cases, as in that of inflammation-induced bone loss, we found that massive macrophage apoptosis caused by systemic exposure to LPS can strongly potentiate inflammation because it leads to the processing and release of mature IL-1β, a major inflammatory mediator (F. R. Greten and coworkers, unpublished data). It is well established that the processing of the IL-1β precursor requires the activation of caspase-1 and that without caspase-1 activation, no mature IL-1β can be released (49–51). As caspase-1 activation is linked to apoptosis, any condition that increases macrophage apoptosis, including p38 inhibition, potentiates IL-1β release. Such problems, however, if encountered, can be addressed through the use of IL-1 inhibitors, such as IL-1Ra.
As mentioned previously, in contrast to IKKβ and p38, JNK activation can promote apoptosis (43). Therefore, inhibition of JNK, in addition to inhibition of inflammation, may be used in conjunction with IKK or p38 inhibition.
CONCLUSIONS
Preliminary preclinical data suggest that inhibitors that target IKKβ and the JNK and p38 MAPK cascades exhibit antiinflammatory activity. In addition, inhibition of ERK is a promising means of reducing production of TNF-α, although no direct inhibitors have been identified. However, it is difficult to extrapolate from gene knockout studies, in which the activity of a given kinase is completely abolished, to drug therapy, where a partial inhibition may be sufficient to achieve the desired therapeutic effect. Further experimentation with actual IKKβ, JNK, and p38 inhibitors is needed.
Notes
Supported by grants from the National Institutes of Health and the American Cancer Society.
Conflict of Interest Statement: M.K. has been a member of the scientific advisory board of Celgene Pharmaceutical, a company with an interest in developing various kinase inhibitors in inflammation, from which he has received $10,000/yr. M.K. is also a stockholder of Celgene.
References
Articles from Proceedings of the American Thoracic Society are provided here courtesy of American Thoracic Society
Full text links
Read article at publisher's site: https://doi.org/10.1513/pats.200504-034sr
Read article for free, from open access legal sources, via Unpaywall:
https://europepmc.org/articles/pmc2713329?pdf=render
Citations & impact
Impact metrics
Citations of article over time
Alternative metrics
Smart citations by scite.ai
Explore citation contexts and check if this article has been
supported or disputed.
https://scite.ai/reports/10.1513/pats.200504-034sr
Article citations
Interactions between Dietary Antioxidants, Dietary Fiber and the Gut Microbiome: Their Putative Role in Inflammation and Cancer.
Int J Mol Sci, 25(15):8250, 28 Jul 2024
Cited by: 0 articles | PMID: 39125822 | PMCID: PMC11311432
Review Free full text in Europe PMC
Transcription Factors in Prostate Cancer: Insights for Disease Development and Diagnostic and Therapeutic Approaches.
Genes (Basel), 15(4):450, 02 Apr 2024
Cited by: 1 article | PMID: 38674385 | PMCID: PMC11050257
Review Free full text in Europe PMC
Resveratrol improves the prognosis of rats after spinal cord injury by inhibiting mitogen-activated protein kinases signaling pathway.
Sci Rep, 13(1):19723, 13 Nov 2023
Cited by: 1 article | PMID: 37957210 | PMCID: PMC10643657
Cholesterol dependent cytolysins and the brain: Revealing a potential therapeutic avenue for bacterial meningitis.
AIMS Microbiol, 9(4):647-667, 21 Aug 2023
Cited by: 1 article | PMID: 38173970 | PMCID: PMC10758573
Review Free full text in Europe PMC
Diagnosis of T-cell-mediated kidney rejection by biopsy-based proteomic biomarkers and machine learning.
Front Immunol, 14:1090373, 06 Feb 2023
Cited by: 2 articles | PMID: 36814924 | PMCID: PMC9939643
Go to all (83) article citations
Data
Data behind the article
This data has been text mined from the article, or deposited into data resources.
BioStudies: supplemental material and supporting data
Nucleotide Sequences
- (1 citation) ENA - PDO98059
Similar Articles
To arrive at the top five similar articles we use a word-weighted algorithm to compare words from the Title and Abstract of each citation.
Mitogen activated protein kinases as targets for development of novel anti-inflammatory drugs.
Ann Rheum Dis, 63 Suppl 2:ii62-ii64, 01 Nov 2004
Cited by: 32 articles | PMID: 15479874 | PMCID: PMC1766783
Review Free full text in Europe PMC
Tanshinone IIA inhibits LPS-induced NF-kappaB activation in RAW 264.7 cells: possible involvement of the NIK-IKK, ERK1/2, p38 and JNK pathways.
Eur J Pharmacol, 542(1-3):1-7, 06 May 2006
Cited by: 114 articles | PMID: 16797002
Mitogen-activated protein kinase and nuclear factor kappaB together regulate interleukin-17-induced nitric oxide production in human osteoarthritic chondrocytes: possible role of transactivating factor mitogen-activated protein kinase-activated proten kinase (MAPKAPK).
Arthritis Rheum, 42(11):2399-2409, 01 Nov 1999
Cited by: 105 articles | PMID: 10555036