Abstract
Free full text

Mechanisms and Modulators of Temperature Regulation
Local thermal control of the human cutaneous circulation
Abstract
The level of skin blood flow is subject to both reflex thermoregulatory control and influences from the direct effects of warming and cooling the skin. The effects of local changes in temperature are capable of maximally vasoconstricting or vasodilating the skin. They are brought about by a combination of mechanisms involving endothelial, adrenergic, and sensory systems. Local warming initiates a transient vasodilation through an axon reflex, succeeded by a plateau phase due largely to nitric oxide. Both phases are supported by sympathetic transmitters. The plateau phase is followed by the die-away phenomenon, a slow reversal of the vasodilation that is dependent on intact sympathetic vasoconstrictor nerves. The vasoconstriction with local skin cooling is brought about, in part, by a postsynaptic upregulation of α2c-adrenoceptors and, in part, by inhibition of the nitric oxide system at at least two points. There is also an early vasodilator response to local cooling, dependent on the rate of cooling. The mechanism for that transient vasodilation is not known, but it is inhibited by intact sympathetic vasoconstrictor nerve function and by intact sensory nerve function.
the blood flow to the cutaneous circulation has a tremendous range, from nearly zero in extreme cold to a total for the entire body surface of ~7 l/min when body temperatures are high. This wide range is even more impressive when the blood flow is expressed per unit volume of skin. Maximal skin blood flow, for example, can reach an estimated 300–400 ml·min−1·100 g skin−1 when reflex, local, and/or pharmacological vasodilator stimuli fully relax cutaneous vascular smooth muscle (25, 40, 63).
Reflexes controlling skin blood flow have the capacity to use nearly this entire range. Body cooling will raise sympathetic vasoconstrictor activity directed to skin through reflexes initiated by low skin temperature and potentially low internal temperature. Body heating inhibits the tonic activity of the vasoconstrictor nerves through reflexes initiated by elevated internal and skin temperatures. As body heating increases in duration and internal temperature increases significantly, a sympathetic cholinergic active vasodilator system is activated in nonglabrous skin. That system has the potential to bring skin blood flow to maximal levels in those areas under conditions of “tolerable” heat stress (40, 65, 76). Those reflex mechanisms are reviewed by Charkoudian in this series (9).
Direct heating or cooling of the skin also has the capacity to fully vasodilate or fully vasoconstrict the skin, and how such local heating and cooling causes those responses is the subject of this review. Although studies of direct warming and cooling are generally done separately, at least some mechanisms for those responses are shared. We will keep with the usual format of separating these stimuli and the associated mechanisms for the vasomotor responses. Most of the mechanistic development recently has been done in humans and in nonglabrous (hairy) skin, typically in the forearm, and that is the focus for this review.
LOCAL WARMING
The understanding of the nature of the local thermal control of the cutaneous circulation traces from the classical studies by Barcroft and Edholm in the 1940's (5), in which cooling and warming forearm skin was carried out over a wide range of temperatures. The findings from those studies included the observations of a nonlinear relationship of forearm blood flow to local skin temperature, such that increments in blood flow between 20 and 35°C were relatively small, becoming much more dramatic above 37°C, and reaching maximal or near maximal values at ~42°C, the limit of heating in those studies. They also noted that prolonged heating at a constant elevated temperature caused an initial vasodilation, succeeded by a return toward baseline, the “die away” phenomenon (5). These observations serve as the basis for later mechanistic studies into how direct skin cooling causes vasoconstriction and how direct skin warming causes vasodilation.
Direct warming of the skin causes a vasodilation dependent on the degree and the speed of the local warming (5, 29, 58). When local temperature is rapidly increased to 42°C and maintained at that level thereafter, skin blood flow in that region typically increases to its maximum (39, 76). That observation, in itself, is useful in providing a characteristic for that region of skin, because it describes the circulation to that area when cutaneous vascular smooth muscle is fully relaxed and, therefore, is independent of the subtle differences in vasomotor activity that exist at submaximal levels of vasodilation. Because it is a characteristic, local warming to 42°C is often used as means of normalization of the blood flow or vascular conductance responses during an experiment, enabling comparison among blood flow measurement sites and among subjects or subject groups (46). This use is especially pertinent when laser Doppler flowmetry is used to monitor skin blood flow, because the high spatial discretion of that method tends to show significant site-to-site differences in baseline blood flow and in the increases in absolute blood flow for a given stimulus (38, 41). Normalization to maximum vasodilation is much like the use of maximal oxygen consumption as a normalizing characteristic for the cardiovascular responses to dynamic exercise (65). A caveat to the use of heating to 42°C to maximally vasodilate skin is that interventions in the nitric oxide (NO) system may prevent full relaxation of vascular smooth muscle because of its involvement as one of the vasodilating mechanisms (see below).
Submaximal local skin warming produces a response pattern characterized by an early, transient peak in skin blood flow, followed by a prolonged plateau phase and, ultimately, by a return in blood flow toward preheating levels (5, 29, 48, 58). As discussed below, the NO system, adrenergic nerves, and sensory nerves each have a role in achieving that response pattern. Figure 1 shows the conceptual outline of the mechanisms of the vasodilator response to local skin warming as they are currently understood.
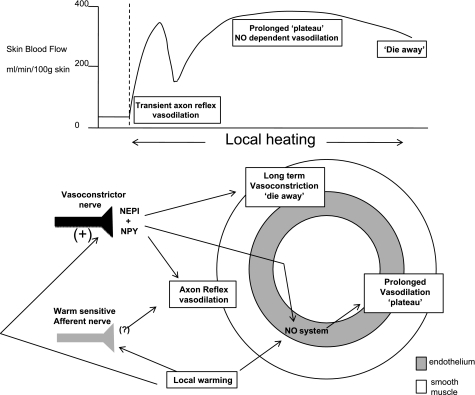
Cutaneous vascular response to rapid local skin warming. Top: pattern of the blood flow response to local warming, including an early transient vasodilation (axon reflex), a more sustained plateau vasodilation, and a later die-away phenomenon. Bottom: our current understanding of the mechanisms leading to that pattern, including roles for the endothelium and nitric oxide (NO) generation, sympathetic transmitters and cotransmitters, and warm-sensitive afferents. NEPI, norepinephrine; NPY, neuropeptide Y.
NO
A locally synthesized vasodilator substance in essentially all tissues is NO. Could local heating be a stimulus for NO formation and vasodilation in the skin? It is clear that NO is an important element in neurogenic vasodilation in the skin (44, 66). Results from a series of studies clearly demonstrate NO to be importantly involved in the vasodilator response to direct warming of the skin. This demonstration was made through the use of the placement of a microdialysis fiber, providing for the delivery of NG-nitro-l-arginine methyl ester (l-NAME) (48, 58) an antagonist to NO synthase (NOS), although not isoform specific. This approach has the value of limiting the antagonist delivery to a small area of skin, while avoiding systemic effects and providing a continuous delivery, such that the washout or duration of action of the inhibitor is not an issue. This drug delivery approach, when combined with laser Doppler flowmetry as the method for monitoring skin blood flow, enables monitoring specific to the site of drug treatment and at a nearby control site at the same time and, therefore, the same thermoregulatory background.
Application of l-NAME after the skin had already been vasodilated by local warming to 40°C caused a prompt reversal of that vasodilation, averaging a fall of nearly 50% (48). The original vasodilation was restored by application of sodium nitroprusside (48). The conclusion that NO is importantly involved in the cutaneous vasodilator response to local skin warming was also gained from a study by Minson and colleagues (58), who applied l-NAME (microdialysis) before or during a standard local heating protocol. Again, the vasodilator response after 30 min was inhibited by ~50% by the NOS antagonist. The earlier initial peak, characteristic of the response to local warming, was also reduced.
These findings gained further verification from Gooding et al. (24), who also found that the microdialysis of l-NAME reduced the vasodilator response to local skin warming. The level of local heating, 42–43°C, is usually sufficient to cause maximal vasodilation (39, 76). In this case, there was an average reduction in maximum skin blood flow of ~28% when l-NAME was applied (24).
An interesting element of the above study by Gooding et al. (24) was the test of whether the phosphodiesterase inhibitor sildenafil (oral), which prevents the breakdown of cyclic GMP, might enhance the vasodilator response to local warming. The peak response to a local temperature of 42°C was not significantly affected, which is the expectation, if the skin is already maximally vasodilated by the direct warming to 42°C. However, the sildenafil treatment extended the vasodilation during the recovery phase from local heating, an observation in keeping with stimulation of NOS enzyme(s) by the elevated local temperature.
The above studies did not identify which of the NOS isoforms is important in mediating that response. Studies by Kellogg et al. (43, 49, 50) and Stewart at al. (73), designed to find that answer, came to quite different conclusions, however. Stewart et al. (73) applied the neuronal NOS (nNOS) antagonist Nω-nitro-l-arginine-2,4-l-diaminobutyric amide, as well as the nonspecific NOS antagonist nitro-l-arginine to a second site, then heated the areas to 42°C, and compared those results in calf skin blood flow to the vasodilation seen earlier at the same sites, before NOS antagonism. The different antagonists inhibited the response to local warming equally, suggesting an important role for nNOS in the vasodilator response to local heating (73).
The latter conclusion was not confirmed by a series of studies by Kellogg et al. (43, 49, 50). The specific nNOS inhibitor 7-nitroindazole had no significant or measureable effect on the vasodilator response to local skin warming, but was shown to reduce significantly the reflex vasodilation to whole body heating, consistent with the effects of nonspecific NOS antagonism on active vasodilation seen earlier (44, 66). When Kellogg and colleagues (49) followed with a similar protocol, they found that the endothelial NOS (eNOS)/inducible NOS inhibitor NG-amino-l-arginine had no significant effect on the reflex vasodilator response to heat stress, but reduced by over 50% the increase in skin blood flow attending local heating. These data are strongly supportive of the conclusion that the NOS isoform important for the cutaneous vasodilator response to local skin warming is eNOS, but that it does not play any obvious role in active reflex cutaneous vasodilation.
In the third of this series, Kellogg et al. (43) used a different nNOS inhibitor, Nω-propyl-l-arginine, alone and in combination with eNOS inhibition by ng-amino-l-arginine. The results were consistent with the above conclusion that nNOS is important in reflex cutaneous vasodilator responses to whole body heating, but not in the vasodilation accompanying local skin warming, whereas eNOS is involved in the response to local heating, but not in the reflex response to whole body heating. Why these internally consistent results differ from those of Stewart et al. (73) is not at all clear. Differences between responses in forearm skin (49) and leg skin (73) are possible. Differences in the heating protocols cannot be ruled out: repeat heating of a single site before and during NOS inhibition (73) vs. separate control and NOS antagonized sites (49). Finally, the in vivo efficacy and specificity of the NOS antagonists has to be considered before a final conclusion can be drawn. Until specific agonists are available for in vivo testing of dosing efficacy and antagonist specificity, this question will remain open.
Venturini et al. (82) found that activations of nNOS and inducible NOS were highly temperature sensitive in vitro, approximately doubling activity between 30 and 42°C. On the other hand, eNOS showed a much smaller sensitivity to temperature in the conversion of arginine to citrulline. That evidence, by itself, would suggest eNOS not to be a temperature-dependent source of NO in heat-induced vasodilation. That conclusion awaits in vivo demonstration of these in vitro findings. It is also of some importance to consider that temperature changes might affect the NO system at sites other than the enzyme, per se (32) (see LOCAL COOLING), and that the effects of temperature on skin blood flow in local warming may be more complex than simple NOS enzyme kinetics. For example, a real possibility that brings together much of the foregoing is that thermal sensitivity resides downstream from the NOS enzyme, such that tonic production of NO provides the substrate for subsequent thermally sensitive steps. In that case, the reduction of heat-induced vasodilation by pharmacological inhibition of eNOS would not be due to a thermal sensitivity of the enzyme, but because the availability of NO to downstream thermally sensitive steps was limited. This would explain observations of a limit to heat-induced vasodilation from eNOS inhibition, but a lack of thermal sensitivity of the enzyme (49, 82).
The major role for NO in the cutaneous vasodilator response to local heating is clearly its direct effects on smooth muscle activity. However, a series of important studies from the Crandall laboratory has shown there also to be a sympathoinhibitory role for NO (17, 67, 68, 84). Local skin warming inhibits the vasoconstrictor response to exogenous norepinephrine and to sympathetic vasoconstrictor activity (17, 67, 68). If the NOS systems are blocked, that inhibitory effect of local warming on adrenergic function is no longer seen, strongly indicating it is the NO component of local heating that is sympathoinhibitory (84).
Sensory Nerves
Afferents in the skin sensitive to the tissue temperature are implicated in the vasodilator response to local heating. Studies from several laboratories using very different approaches led to the conclusion that thermally sensitive afferents in the skin were important participants in the vasodilator response to local skin warming (54, 58, 72).
Magerl and Treede (54) briefly warmed skin at different rates and monitored a vasodilation that was rapid in onset, spread to adjacent areas up to 30 mm distant, and required a stimulus intensity consistent with the involvement of warm-sensitive nociceptors. Less intense warming, in the range of activation of warm receptors (30–35°C), did not evoke this axon reflex.
Minson et al. (58) applied a standard local heating protocol involving rapid warming of forearm skin to 42°C. They noted an early, transient vasodilator response, followed by a partial recovery and then a sustained plateau as local skin warming was sustained. Topical local anesthetic cream applied before locally heating the area caused the early, transient vasodilator response to be reduced by >50%. This reduction is likely because the treatment anesthetized thermally sensitive afferents in the dermis, as sympathetic vasoconstrictor fibers do not appear to be affected by this method of anesthetization of cutaneous nerves (42).
Evidence featuring sensory nerve involvement in the cutaneous vasodilator response to direct skin warming also comes from a study by Stephens et al. (72), in which topical capsaicin was used to compare the vasodilator effects of chemical stimulation of thermally sensitive afferents to that from their physical stimulation by direct heating. Several relevant observations came from that study. First, there was no perception of heat when sites with or without capsaicin treatment were cooled to 29°C or below. Skin blood flow was low, both at control and at capsaicin treated sites. Second, during slow local warming, local temperatures reached a threshold at which heat was first perceived; at that instant, substantial vasodilation occurred. This correspondence between the sensation of heat and the onset of vasodilation occurred at both treated and untreated sites, but at markedly different temperatures between sites (3–6°C lower at the capsaicin-treated sites, depending on its concentration). Third, the vasodilator response correlated more closely to the perception of temperature than to the actual temperature. These findings point to an important involvement of sensory nerves in the response (72). Together, these studies strongly support the conclusion that the early transient vasodilator response to local skin warming is mediated largely through warm receptors or warm sensitive nociceptors acting in an axon reflex, with spinal reflexes being ruled out by the lack of influence of proximal nerve blockade (58). It is likely that CGRP and/or substance P is important in this role of afferents in the response to local skin warming (83); however, the specific tests for that possibility have not yet been accomplished.
The axon reflex with local skin warming has some temporal and functional crossover with the NO involvement. The initial vasodilation is transient, but is modestly reduced or delayed by NOS inhibition (30, 36, 58), whereas the subsequent plateau phase is reduced by NOS inhibition, but the cutaneous vasodilation of that phase was unaffected by topical anesthetic.
Sympathetic Contribution
Although it is intuitive to assign sympathetic nerve function to extrinsic reflex control of vascular resistance, the evidence strongly supports some involvement in the local thermal control of skin blood flow during skin warming. Furthermore, recent findings show a role for sympathetic adrenergic nerves in support of the vasodilator response, also counterintuitive to our general appreciation of sympathetic adrenergic function. Charkoudian et al. (10) studied the responses to local skin warming in subjects who had undergone surgical sympathectomy 0.5–5 yr earlier. They observed that the transient peak, characteristic of the early response to local skin warming, was absent in the patient group, whereas they did not find any alteration in the later plateau phase. These results indicate a role for sympathetic function in that axon reflex portion of the response, or that the later NO-dependent phase began earlier, obscuring the pattern normally attending the axon reflex (10). The latter possibility suggests an inhibitory effect of sympathetic activity on NO-dependent vasodilation.
More acute pharmacological abolition of sympathetic function has a somewhat different effect on the pattern and extent of the vasodilator response to local skin warming. Houghton et al. (36) found the axon reflex to be abolished by local treatment with the antiadrenergic drug bretylium. The principal action of bretylium is to act presynaptically on adrenergic nerves to prevent the release of norepinephrine and any cotransmitters (26, 47, 71). Although this latter observation was somewhat surprising, given the usual vasoconstrictor role for norepinephrine, it is in keeping with studies showing norepinephrine to have a sensitizing effect on the pain threshold to skin heating (15) and to generate axon reflex vasodilation mediated through α1-receptors (14). Application of norepinephrine directly to skin causes a vasoconstriction at the site, but a vasodilation in its periphery (14, 16). That vasodilation is blocked by topical anesthesia (14), verifying its neurogenic nature. This scenario has been questioned, however, by the observation that norepinephrine administered locally by microdialysis does not cause axon reflex vasodilation (89), but does do so if the norepinephrine is administered by iontophoresis (16). These methodological questions, while important, do not negate the effect of bretylium on abolition of the axon reflex to slow local heating (30, 36).
That local antiadrenergic treatment has an inhibitory effect on the axon reflex response to local skin warming was further demonstrated by the combination of intradermal α- and β-adrenoceptor antagonists or the neuropeptide Y (NPY) receptor antagonist, which delayed the axon reflex to higher local temperatures during local warming. When those agents were combined, the axon reflex portion of the response to local heating was eliminated. However, the axon reflex can be restored in the presence of sympathetic blockade if the local warming is rapid (29). Thus the sympathetics can support or modify the axon reflex, but are not required for that response.
A role for sympathetic vasoconstrictor nerves in the cutaneous vasodilator responses to direct skin heating extends both to intermediate and to longer term durations of local heating (29, 30). When local heating was extended to the NO-dependent plateau period, blockade of transmitter release from cutaneous vasoconstrictor nerve terminals limited the extent of vasodilation by ~50% (29). This limitation to vasodilation in this series was the same as seen when NOS was blocked. It was of particular interest that the addition of NOS inhibition to sympathetic blockade did not further limit the extent of vasodilation, suggesting the actions of norepinephrine and/or NPY were somehow through NOS production. This possibility is conceivable because there are α2- and NPY Y1-receptors on endothelial cells, and binding to their agonists stimulates eNOS to produce NO (2, 13, 51, 81, 88).
To discover whether local warming stimulates adrenergic transmitter release, Hodges et al. (29) held body skin temperature at 34°C, a neutral temperature chosen to minimize tonic vasoconstrictor nerve activity (40). That this was successful is shown by the failure of bretylium delivered by microdialysis to cause a vasodilation in thermoneutral skin, demonstrating a lack of tonic transmitter release under those conditions. Nevertheless, bretylium still caused a reduced blood flow response to local heating, indicating the presence of transmitter and implying that its release is stimulated by the local warming (29, 30).
As local heating is prolonged to beyond 60 min, the cutaneous vasodilator response is seen to wane significantly in the “die-away” phenomenon (5). Treatment with bretylium abolished the die away, such that blood flow at treated sites was remarkably constant during that phase of local heating. Blood flow at those sites was less than that at control sites in the early portion of the plateau phase of local heating, becoming equal as blood flow at the control sites underwent the die-away phenomenon (29). This comparison suggests that either the role for norepinephrine and/or NPY is reversed to one of vasoconstriction in these later stages of local heating, or that the release of transmitter stimulated by local heating (or subsequent steps in the process) is waning, and the vasodilator support by adrenergic transmitters in the earlier period of the plateau phase is being withdrawn. In either case, the findings make it clear that the die-away phenomenon is dependent on adrenergic function.
Other Contributors
The above development implicates the NOS system(s), sensory nerves, and sympathetic nerves as contributors to heat-induced cutaneous vasodilation, with the latter two mechanisms having at least some of their effects through NO. It is likely that CGRP and substance P are involved in at least the axon reflex portion of the response, although that expectation has not been directly tested. Histamine does not appear to play an important role, according to several studies (24, 85). Although histamine is a cutaneous vasodilator, the urticaria associated with histamine is not typically seen with local warming. Also, histamine and heat-induced vasodilation appear to involve different afferent fibers (80).
Prostaglandins have also been dismissed as contributors to heat-induced vasodilation (23). Oral aspirin sufficient to block vasodilation from an anodal current (18) was without effect on the vasodilation from direct warming of the skin to 41°C (23). The only caveat to that conclusion is the observation that mild pain with local heating can induce a NO-independent cutaneous vasodilation (49), and, in that context, nociceptor stimulation of the formation of vasodilating prostaglandins or histamine might contribute to that response. However, it would be more accurate to characterize such vasodilation as pain mediated rather than a response to local heating per se.
Physiological and Pathophysiological Modifiers of the Response to Local Warming
Understanding the mechanisms for the cutaneous vasodilator response to local heating encourages the application of that knowledge to situations in which vasomotor control might be altered [see the review by Minson (57) in this series]. Indeed, any abnormalities in the response to local heating might be traceable to specific processes and offer a better mechanistic understanding of the altered response. Furthermore, when a particular pathology affects a known control system, that knowledge can be used to probe the mechanisms of control, as has been the case for the neurogenic active vasodilator system (7, 45).
One example is the use of maximal skin blood flow from local heating to 42°C as a way of estimating structural differences and/or their resolution. For example, maximal skin blood flow is reduced in hypertension (8). Because this characteristic is, by definition, the resistance when there is no contractile activity in vascular smooth muscle, the reduction signals a probable thickening of the media (22). It would be interesting to follow changes in maximal skin blood flow with different antihypertensive therapies as a way to monitor which was the most efficacious in reversing that altered vascular architecture. Of course, caution must be exercised in this respect for cases in which NOS function is compromised, because local warming requires a fully functional NOS system to exert its full vasodilator potential. This would potentially include hypertension (33), diabetes (see below), and aging (see below).
Maximal skin blood flow is reduced with age (55, 64). There are also functional changes at submaximal levels with advancing age (35, 59, 79). These are covered in detail elsewhere in this series (see Ref. 34). Suffice it to note here that, with respect to the vasomotor effects of local warming, cutaneous vasodilation is less in older subjects, and that appears to reflect an impaired NO signaling, consistent with endothelial dysfunction (35, 59). It appears that the sensory element of locally induced vasodilation is also reduced (60), perhaps another manifestation of impaired endothelial function. Thus it appears that both the initial sensory and later NO-dependent phases of the response to local warming are impaired by aging, although these deficits may be both through the NO system.
One caveat here is that the use of laser Doppler flowmetry in assessing cutaneous vascular responses typically refers those responses to a maximal value (see Ref. 46), because the spatial discretion of the method for the measurement of skin blood flow limits the ability to make estimates of absolute levels of skin blood flow (see Refs. 38, 41). This is usually not a problem, but the fact that maximal skin blood flow is reduced by aging (55, 64) means that analysis of differences in heat-induced vasodilation requires recognition of that characteristic lowering of the ceiling. The lower maximal levels of skin blood flow indicate that age-dependent structural changes in the cutaneous circulation also are part of the overall difference with younger subjects.
Charkoudian et al. (11) investigated the role of female reproductive hormone background on the vasodilator response to local skin warming, finding a small but significant enhancement of the vasodilator response when estrogen and progesterone were elevated, compared with the low-hormone phase of the menstrual cycle. This enhancement may be stimulated by estrogen acting at one or more of the mechanisms above, in particular NOS (27), but that possibility has not been directly tested.
Local heating has also been used to evaluate cutaneous vascular function in type 2 diabetes mellitus, a group that is generally regarded to have disrupted endothelial function (69, 70, 75). Relatively healthy subjects with diabetes mellitus did not show an altered axon reflex portion of the vasodilator response to skin warming (75), although that is likely to become degraded as the duration and severity of the diabetes increases. More prolonged, direct local heating to 42–45°C was found to cause significantly less vasodilation in patients with diabetes mellitus and associated peripheral vascular disease. Because local heating to this extent usually causes maximal cutaneous vasodilation (76), it is not clear whether this effect of diabetes is through its effects on endothelial dysfunction or through structural changes, although it appears that the relative contribution of NO to vasodilation in diabetes is similar to that in healthy control subjects, in the face of a reduced vasodilation in absolute terms (69). The above provides a potential site to probe for the degree of vascular dysfunction in this disease and also a monitor for changes in vascular function as a result of disease progression or therapy. This is of major importance in this epidemic disease, because vascular dysfunction in skin is the reason for loss of limbs in diabetes, and it is primarily cutaneous vascular lesions that are an early signal and often the primary tissue at risk.
There is also good evidence that the plateau phase of the response to prolonged local skin heating is impaired in a subgroup of patients with orthostatic hypotension, although the axon reflex is apparently not affected (56). NOS inhibition converts the responses of healthy controls and that of the patient group to very similar patterns, consistent with endothelial dysfunction as an explanation for the limited response in the patient group (56, 73). Angiotensin II receptor blockade also normalizes the response to local heating in this patient group (74), suggesting a role for angiotensin II in the endothelial dysfunction seen with low-flow postural tachycardia syndrome.
LOCAL COOLING
Direct cooling of the skin has the expected vasoconstrictor influence, and the mechanisms underlying that intuition have gained clarity recently. The important elements appear to be an inhibition of the NO system at more than one point, an involvement of vasoconstrictor function through postsynaptic upregulation of α2c-receptors and a role for cold-sensitive afferents. Figure 2 outlines the control elements of the cutaneous vascular response to local cooling as they are currently understood.
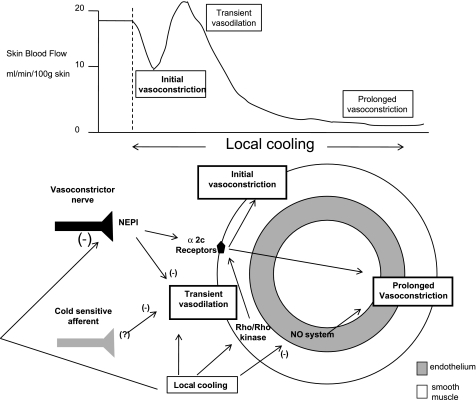
Cutaneous vascular response to rapid local skin cooling. Top: pattern of the response in skin blood flow to local cooling, including a transient early vasodilation (made more obvious when cooling is rapid or sensory, or adrenergic nerve function is blocked locally), followed by a sustained vasoconstriction when the cooling is prolonged. Bottom: mechanisms and structures important in generating that pattern, including inhibition of the endothelial NO synthase system, an upregulation of α2c-receptors, sympathetic vasoconstrictor nerves, and cold-sensitive afferents.
NO Function
Whereas local skin warming has much of its effect through stimulation of the NO system, either through effects on NOS or some other point downstream from the enzyme (see above), local cooling has a significant portion of its vasoconstrictive effect through inhibition of the NO system (32, 87). Blockade of NOS with l-NAME reduced basal skin blood flow by removing tonic NO production (87). Subsequent local cooling reduced skin blood flow at both untreated and NOS-inhibited sites, but to a lesser extent at the latter sites. Importantly, the final levels of cutaneous vascular conductance and skin blood flow were the same at the two sites: local cooling had acted to inhibit the NO system at control sites, as l-NAME had already done at the other sites. Most of the remaining vasoconstriction was due to adrenergic function (see below).
Hodges et al. (32) followed the above observation with a similar study in which slow local cooling was applied to areas of skin previously treated with bretylium to remove adrenergic function. Cooling had essentially the same effect on skin blood flow as did NOS inhibition with l-NAME. Addition of NO to an l-NAME site with exogenous nitroprusside was used to restore skin blood flow to its original baseline. Local cooling at that stage caused a significant reduction in skin blood flow at that site, but had no effect at l-NAME-treated sites without NO supplementation. The interpretation of the above is that local cooling has a significant portion of its vasoconstrictor effects through inhibition of the NO system, but the evidence favors an effect on points beyond NO synthesis, because sites without functional NOS, but with supplemental NO, showed a cold-induced vasoconstriction. The evidence also favors inhibition of NOS, per se, because local cooling or pharmacological NOS inhibition causes a greater vasoconstriction than does local cooling of the NOS-blocked/NO-supplemented site. Specific antagonists to the different NOS isozymes have not been applied to the responses to local cooling.
Adrenergic Function
Adrenergic function is a second major component of the cutaneous vasoconstrictor response to local skin cooling (31, 32). The story has taken a few turns, but now it appears that the major alteration in adrenergic function with cooling takes place postsynaptically. According to the current evidence, local cooling stimulates the mobilization of α2c-receptors from the Golgi apparatus to the vascular smooth muscle plasma membrane (3, 4, 37). This causes an apparent increase in α2 sensitivity, but this is due to an increased receptor number and not to an effect of cold on individual receptor sensitivity (12). That mobilization is brought into play by the Rho-Rho kinase system (3), which, in turn, is stimulated by mitochondrial reactive oxygen species (4). Recently, a cyclic AMP/Rapl/JNK signaling pathway in stimulating α2c gene transcription has been implicated as a part of this adrenergic portion of the response to local cooling (19). It is not clear whether cooling augments the activity of this transcription pathway, however.
The above synthesis derives from studies involving many species, cell types, and experimental approaches at the cellular/molecular level. This analysis of vascular function is made more engaging from observations on intact skin of healthy humans in which an α2-antagonist, applied by iontophoresis to the dorsal aspect of the finger, was shown to inhibit cold-induced vasoconstriction (20, 53). More recently, when the Rho-Rho kinase inhibitor fasudil was administered by intradermal microdialysis to forearm skin, the vasoconstrictor response to local cooling was significantly delayed and reduced (77). That the scenario developed from in vitro studies applies to nonglabrous skin in intact humans also gained support from Yamazaki (86), who found that antioxidant supplementation with intradermal ascorbate reduced the vasoconstrictor response to local cooling. This is consistent with there being an important role for reactive oxygen species in local cooling-induced vasoconstriction (4). The inhibitory effect of intradermal ascorbate disappeared when cooling was applied to skin, with adrenergic receptors blocked (86), also consistent with the above in vitro findings.
The phases of the cutaneous vasomotor responses to local cooling do not have the same convenient demarcations as does the pattern of the response to local warming (58). Nevertheless, the findings with fasudil indicate the initial portion of the vasoconstriction to be due to the Rho-Rho kinase system, presumably working through α2c-receptor mobilization, whereas the later phase probably involves both activation of adrenoceptors and inhibition of NOS. Indeed, when both NO and adrenergic systems are blocked by l-NAME and bretylium, respectively, there is no vasoconstriction to local cooling of 10°C or so (32, 87).
One puzzling aspect of the adrenergic role in the vasoconstriction with local skin cooling begins with the assumption that there must be some norepinephrine release to stimulate the α2c-receptors newly displaced to the plasma membrane. As indicated above, low temperature inhibits the formation and release of norepinephrine (6), making it likely that there is less norepinephrine in the subsynaptic cleft to bind to the adrenergic receptors. It is possible that there is still sufficient release such that the increase in postsynaptic α2c-adrenoceptor number more than compensates for any reduced transmitter release. However, studies involving anesthesia of the antebrachial cutaneous nerve of the forearm do not present a picture consistent with the pre- and postsynaptic pharmacological blockade of adrenergic function (62). In particular, such anesthesia of the nerve was not associated with an inhibited vasoconstrictor response to local cooling, nor with the transient vasodilation at the beginning of local cooling (62). Similarly, elevated body skin temperature to reduce or eliminate tonic vasoconstrictor activity (29) was without measureable effect on the vasoconstrictor response to local cooling (62). As developed above, blockade of norepinephrine release or adrenergic receptors inhibit the vasoconstrictor response and reveal the early transient vasodilation (42, 62, 87). Do these results mean that local cooling does, indeed, stimulate transmitter release from adrenergic nerves, perhaps through an axon reflex (42)? A study to directly test that possibility showed that cold-sensitive afferents are not responsible for stimulating norepinephrine release from sympathetic nerve terminals, because topical anesthetic did not affect the steady-state response to local cooling, whereas that response was significantly reduced by blockade of transmitter release with bretylium (31). Alternatively, do the results indicate a direct effect of cold on α2c activity, even without transmitter present? Those questions delay any ultimate synthesis of the adrenergic component of local cooling-induced vasoconstriction.
Other Contributors
The fact that the combination of adrenergic nerve blockade and NOS inhibition eliminated the longer term vasoconstrictor response to local cooling is not reason to dismiss the possibility of other contributors to the response. In particular, when adrenergic function is blocked pre- or postsynaptically, or topical local anesthesia is administered locally, cooling is often accompanied by a marked transient vasodilation, made even more obvious if the local cooling is rapid (31, 42, 62, 87). The origin of this transient vasodilation is unknown. It is not via adrenergic function. Their removal reveals the vasodilator response to local cooling. However, blockade of NPY Y1-receptors does not reveal this transient vasodilation early in cooling, indicating that norepinephrine and not that cotransmitter is responsible (42). It is not via NO function, as nonspecific NOS inhibition in the presence of adrenergic blockade does not affect the transient vasodilation (87). It is attractive to consider an axon-mediated reflex as the source for this response, but it is also revealed by topical anesthesia, rather than inhibited by such treatment (42). This latter observation indicates an involvement of sensory nerves in the responses to local cooling, although that role may be limited to suppression of this transient vasodilation at the beginning of cooling. It is a bit puzzling that removal of either adrenergic or sensory nerve function reveals this unexplained vasodilation at the beginning of local cooling.
In summary, local cooling has a rate-dependent initial vasoconstriction with major involvement of adrenergic nerves, which act to cause a pronounced vasoconstriction and inhibit a background vasodilation of uncertain origin. The adrenergic component appears to involve a translocation of α2c-receptors from the Golgi to the plasma membrane, stimulated by mitochondrial-derived reactive oxygen species and supported by a cAMP system. As cooling proceeds, the NO system is inhibited, in part at the level of the NOS enzyme and in part at steps downstream from the production of NO.
Physiological and Pathophysiological Modifiers of the Response to Local Cooling
Pathology or background alterations in normal physiological function can serve to affect the cutaneous vasomotor responses to local skin cooling (see Ref. 57). For example, Raynaud's phenomenon is a prominent example of cold-induced vasoconstriction gone wrong (21). Inflammation or chronic physical stress may mobilize α2c-receptors in a manner similar to the normal response to local cooling, but the additional stimulus for receptor mobilization from local cooling may lead to exaggerated and prolonged vasoconstriction (52). If this proves to be the case in Raynaud's phenomenon, selective α2c or Rho-Rho kinase inhibition may serve as appropriate therapeutic agents (see Ref. 21).
Charkoudian et al. (11) tested whether background changes in estrogen and progesterone might influence the vasoconstrictor response to local cooling; vasodilator responses to local skin warming are slightly enhanced by the elevated estrogen and progesterone of the luteal phase. An exaggerated vasoconstrictor response to cooling might serve as a partial explanation for the greater incidence of Raynaud's phenomenon in women. However, stepwise local cooling from 32 to 20°C showed no difference in the vasoconstrictor response between the low- and high-hormone phases of oral contraceptive use (11).
Pérgola et al. (61) found that local skin cooling had an inhibitory effect on the reflex active vasodilator response to rising internal temperature. This was most likely through the adrenergic effects of local cooling, because that reduced sensitivity was reversed by bretylium pretreatment. Local cooling also suppresses the sensitivity of the reflex vasoconstrictor response to whole body cooling (1, 28). This effect appears to be more one of the reduced baseline rather than through an effect on the reflex, because restoration of blood flow with isoproterenol at locally cooled sites also restored the reflex vasoconstrictor response to body cooling (28).
Aging, covered in more detail in this series by Holowatz and Kenney (34), is not associated with an altered response to local skin cooling (79). However, the mechanisms for arriving at the same reductions in skin blood flow may, indeed, change with age, with the observation that blockade of Rho kinase appeared to have a more marked inhibitory effect on cold-induced vasoconstriction in older compared with younger subjects (78). These observations suggest enhanced Rho-Rho kinase function compensates for the expected reduction in adrenergic function with aging.
General Summary
Although it is not surprising, intuitively, that direct warming of the skin would cause vasodilation and direct cooling would cause vasoconstriction, the actual mechanisms behind those responses are not so obvious, and some are actually counterintuitive. Both local warming and local cooling involve the NOS system in a way consistent with its activation by warming and its inhibition by cooling, although, in the case of cooling, it is not just an effect via the NOS enzyme(s). Both local cooling and local warming involve the adrenergic system, as well, but here heating and cooling do not operate by having directionally opposite effects on that system. Cooling appears to suppress transmitter release from adrenergic nerve endings, but to increase the number of subsynaptic α-receptors for a net vasoconstrictor effect. Norepinephrine and NPY also act to suppress a transient vasodilation of unknown origin, but stimulated by local cooling. Local warming appears to stimulate the release of norepinephrine and NPY. Oddly, these traditional vasoconstrictors support vasodilator actions. They support the axon reflex vasodilation early in local skin warming and the later plateau phase. However, they are also necessary for the die-away that occurs with longer term local warming. This last effect reflects a vasoconstrictor role. Both local warming and local cooling achieve part of their vasomotor effects through warm- and cold-sensitive afferents, respectively. The warm-sensitive nociceptors give rise to a transient vasodilation. The cold-sensitive afferents also apparently have an early transient effect, in this case to inhibit a vasodilation that would otherwise occur at the onset of local cooling. That transient vasodilation is also inhibited by the presence of a functional NOS system. Thus there are parallels in the mechanisms, giving rise to cold-induced vasoconstriction and warm-induced vasodilation, but those parallels are not simply opposite effects dependent on the direction of the temperature changes. This indicates that the separate analysis of the mechanisms behind the vasomotor effects of local cooling and local warming is not arbitrary, but is required by those differences in control. Finally, alterations in one or more of these mechanisms can be seen in normal physiological variations and can be altered in pathophysiological settings, raising the possibility of use of local temperature changes in diagnosis or as a probe in further understanding of the mechanisms involved.
GRANTS
Work from J. M. Johnson's laboratory was funded by National Heart, Lung, and Blood Institute Grant HL-059166 and from D. L. Kellogg's laboratory by Grant HL-65599.
DISCLOSURES
No conflicts of interest, financial or otherwise, are declared by the author(s).
ACKNOWLEDGMENTS
The authors gratefully acknowledge the contributions to this area of investigation by our many colleagues in this truly global team effort.
REFERENCES
Articles from Journal of Applied Physiology are provided here courtesy of American Physiological Society
Full text links
Read article at publisher's site: https://doi.org/10.1152/japplphysiol.00407.2010
Read article for free, from open access legal sources, via Unpaywall:
https://europepmc.org/articles/pmc2963328
Free to read at intl-jap.physiology.org
http://intl-jap.physiology.org/cgi/content/abstract/109/4/1229
Free after 12 months at intl-jap.physiology.org
http://intl-jap.physiology.org/cgi/content/full/109/4/1229
Citations & impact
Impact metrics
Article citations
A wirelessly programmable, skin-integrated thermo-haptic stimulator system for virtual reality.
Proc Natl Acad Sci U S A, 121(22):e2404007121, 20 May 2024
Cited by: 1 article | PMID: 38768347
Recent Technologies for Transcutaneous Oxygen and Carbon Dioxide Monitoring.
Diagnostics (Basel), 14(8):785, 09 Apr 2024
Cited by: 1 article | PMID: 38667431 | PMCID: PMC11049249
Review Free full text in Europe PMC
Observations of cold-induced vasodilation in persons with spinal cord injuries.
Spinal Cord, 62(4):170-177, 22 Feb 2024
Cited by: 0 articles | PMID: 38388759 | PMCID: PMC11003866
A century of exercise physiology: concepts that ignited the study of human thermoregulation. Part 2: physiological measurements.
Eur J Appl Physiol, 123(12):2587-2685, 05 Oct 2023
Cited by: 0 articles | PMID: 37796291
Review
A century of exercise physiology: concepts that ignited the study of human thermoregulation. Part 3: Heat and cold tolerance during exercise.
Eur J Appl Physiol, 124(1):1-145, 05 Oct 2023
Cited by: 0 articles | PMID: 37796292
Review
Go to all (119) article citations
Other citations
Similar Articles
To arrive at the top five similar articles we use a word-weighted algorithm to compare words from the Title and Abstract of each citation.
Mechanisms and modifiers of reflex induced cutaneous vasodilation and vasoconstriction in humans.
J Appl Physiol (1985), 109(4):1221-1228, 06 May 2010
Cited by: 156 articles | PMID: 20448028 | PMCID: PMC2963327
Review Free full text in Europe PMC
Skin blood flow in adult human thermoregulation: how it works, when it does not, and why.
Mayo Clin Proc, 78(5):603-612, 01 May 2003
Cited by: 393 articles | PMID: 12744548
Review
In vivo mechanisms of cutaneous vasodilation and vasoconstriction in humans during thermoregulatory challenges.
J Appl Physiol (1985), 100(5):1709-1718, 01 May 2006
Cited by: 204 articles | PMID: 16614368
Review
The sympathetic release test: a test used to assess thermoregulation and autonomic control of blood flow.
Adv Physiol Educ, 38(1):87-92, 01 Mar 2014
Cited by: 11 articles | PMID: 24585475
Funding
Funders who supported this work.
NHLBI NIH HHS (2)
Grant ID: HL-65599
Grant ID: HL-059166