Abstract
Free full text

Prospects for Minocycline Neuroprotection
Abstract
Minocycline is a clinically available antibiotic and anti-inflammatory drug that also demonstrates neuroprotective properties in a variety of experimental models of neurological diseases. There have thus far been more than 300 publications on minocycline neuroprotection, including a growing number of human studies. Our objective is to critically review the biological basis and translational potential of this action of minocycline on the nervous system.
The lack of robust treatment options available for neurodegenerative diseases, stroke, and central nervous system (CNS) trauma, all of which often involve both inflammation and apoptotic cell death, has led to an explosion of studies to reexamine the potential of established drugs for treatment of these conditions. Minocycline is a second-generation tetracycline derivative with a proven, safe clinical track record as an antibiotic and anti-inflammatory drug for treating acne and arthritis. Minocycline effectively crosses the blood-brain barrier and has demonstrated neuroprotective qualities in experimental models of CNS trauma, stroke, spinal cord injury, and neurodegenerative diseases including amyotrophic lateral sclerosis, Huntington disease, Parkinson disease, and multiple sclerosis. Thus, it is not surprising that several off-label minocycline clinical trials are under way for a variety of CNS diseases. The emerging success of some of these trials raises optimism that minocycline treatment may soon be translated into clinical practice for CNS diseases, whereas others (eg, in amyotrophic lateral sclerosis) have raised red flags. A thorough understanding of minocycline’s modes of action and the targeted cellular mechanisms is warranted, as are guidelines on safe and effective doses, routes of administration, and establishment of a therapeutic window. Although several lines of evidence point to a convergent action of minocycline in suppression of both apoptosis and CNS inflammation by preventing neural cell death and by inhibiting microglial activation, the exact molecular targets of minocycline have yet to be identified and characterized. This review provides an overview of the established mechanisms of action by which minocycline exerts its neuroprotective effects, summarizes results of animal studies in experimental models of neurological diseases, and discusses results of clinical trials.
MECHANISMS OF ACTION
Several mechanisms of action are thought to be responsible for minocycline’s neuroprotective effects; however, the primary target of this drug in the nervous system remains unknown. Recent studies have focused on the anti-inflammatory, anti-apoptotic, and antioxidant properties of minocycline. The main biological effects of minocycline, which are involved in the pathogenesis of several neurological disorders, include inhibition of microglial activation, attenuation of apoptosis, and suppression of reactive oxygen species production.
In experimental disease models, minocycline treatment suppresses microglial proliferation/activation as well as subsequent release of cytokines such as interleukins 1β and 6 and tumor necrosis factor α.1,2 In addition, it decreases CNS expression of chemokines and their receptors such as macrophage inflammatory protein 1α, interferon-inducible protein 10, chemokine receptor type 5, and chemokine (CXC motif) receptor 3 as well as suppressing the release of lipids and the activity of matrix metalloproteases, which disrupt the blood-brain barrier.1,2 Furthermore, minocycline inhibits T-cell migration into the CNS. The neuroprotection afforded by minocycline treatment of experimental disease models also appears to be related to p38 mitogen-activated protein kinase inhibition, which both preserves neurons and inhibits microglial activation.1,3
The antiapoptotic effects of minocycline are exerted, at least in part, on mitochondria. By reducing mitochondrial calcium overloading, minocycline stabilizes the mitochondrial membrane and inhibits release of cytochrome c and other apoptotic factors into the cytoplasm, thus resulting in decreased caspase activation and nuclear damage.4,5 Minocycline also exerts caspase-independent neuroprotective effects including upregulation of anti-apoptotic factor BCL-2.6
Minocycline possesses antioxidant properties in addition to its antibiotic, antiapoptotic, and anti-inflammatory properties. It directly scavenges free radicals and inhibits molecules such as cyclooxygenase 2, induced nitric oxide synthase, and nicotinamide adenine dinucleotide phosphate oxidase. The antioxidant activity of minocycline may be derived from its chemical structure or its modulating effects on enzymes such as nitric oxide synthase and lipoxygenases.
Poly (ADP [adenosine diphosphate]–ribose) polymerase-1 (PARP-1) is activated by DNA damage and is critically involved in excitotoxicity, oxidative stress, and inflammation. Activation of PARP-1 leads to translocation of apoptosis-inducing factor from the mitochondria to the nucleus and finally promotes cell death and inflammation. Alano et al7 reported that minocycline and other tetracyclines inhibited PARP-1 at nanomolar concentrations, and minocycline protected neurons against PARP-1–mediated toxicity. Other minocycline effects include inhibition of mitochondrial peripheral benzodiazepine receptor (recently renamed 18 kDa translocator protein), increased phosphorylation, membranal insertion of glutamate receptor 1, and inhibition of mitogen activated protein kinases.
Minocycline treatment provides neuroprotection against excitotoxic insults in a number of experimental models. The neuroprotective effects of minocycline are evident after glutamate exposure, and oligodendrocyte survival is enhanced by minocycline treatment after lipopolysaccharide-induced inflammation; all likely occur as a result of decreased microglial activation.8 Overall, although it is still unclear which of these mechanisms plays the pivotal role in eliciting the neuroprotective properties of minocycline, the major targets for minocycline neuroprotection could lie within the complex signaling network linking mitochondria, oxidative stress, excitotoxicity, PARP-1, and apoptosis (Figure).
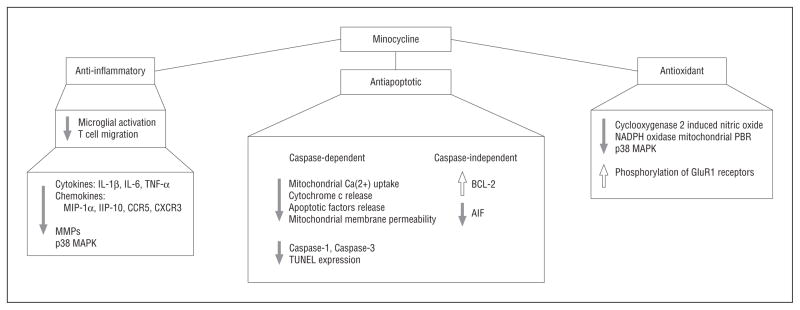
Signaling mechanisms involved in the neuroprotective actions of minocycline. See the text for detailed descriptions. AIFindicatesapoptosis-inducing factor; BCL-2, B-cell leukemia/lymphoma 2; CCR5, chemokine receptor type 5; CXCR3, chemokine (CXC motif ) receptor 3; GluR, glutamate receptor; IL, interleukin; IP-10, interferon-inducible protein 10; MAPK, mitogen-activated protein kinase; MIP-1α, macrophage inflammatory protein 1α; MMP, matrix metalloprotease; PBR, peripheral benzodiazepine receptor; TNF, tumor necrosis factor; TUNEL, terminal deoxynucleotidyl transferase dUTP (2′-deoxyuridine, 5′-triphosphate)–biotin nick-end labeling.
STUDIES IN EXPERIMENTAL MODELS OF HUMAN NEUROLOGICAL DISEASES
Ischemic Injury
Yrjanheikki et al9 first published the neuroprotective effects of minocycline on cerebral ischemia, prompting numerous subsequent evaluations of minocycline on experimental models of stroke, neurodegenerative diseases, and spinal cord injury (Table 1). Some of these studies indicated substantial neuroprotective effects, while in others, minocycline was ineffective or even deleterious. These studies varied widely in dosage, route of administration, timing of drug treatment, species, and experimental model used. Several studies that tested multiple doses of minocycline treatment after ischemia reported neuroprotection with relatively low doses but observed toxicity with high-dose minocycline treatment.6,10 Interestingly, minocycline treatment exacerbated injury in neonatal mouse models of stroke, whereas it attenuated injury in rats using a similar model and dosing paradigm.10,11 Furthermore, minocycline exerts different effects on different types of cells. One recent study found that, while low-dose minocycline was protective to neurons in both in vivo and in vitro experimental models of stroke, it provided no protection to astrocytes in the same models.6 Yet, other studies describe decreased ischemia-induced astrogliosis and reduced white matter damage after ischemic injury following minocycline treatment.12,26 In addition, though most of these publications addressed only the acute effects of minocycline treatment on ischemic injury, it will be important, from a translational point of view, to determine whether minocycline treatment confers long-term neuroprotection or simply delays the onset of cell death. One promising observation, in this respect, is that ischemia-induced behavioral deficits lessened after minocycline treatment.27
Table 1
Results of Experimental Studies on Minocycline Treatment for Neurological Diseases
Animal Model | Dose and Route | Timing | Outcome | Source |
---|---|---|---|---|
Stroke | ||||
MCAO | 45 mg/kg IP on d 1, then 22.5 mg/kg for 2 d | 12 h prior to MCAO, twice daily for 3 d | Reduced infarct volume, inhibited microglial activation, reduced COX-2 expression | Yrjänheikki et al9 |
MCAO | 20 or 100 mg/kg IV | 60 min after stroke | Low dose improved behavioral function, reduced infarct volume, decreased apoptosis, upregulated BCL-2; high dose increased behavioral deficit, increased infarct volume, increased apoptosis, and suppressed BCL-2 expression | Matsukawa et al6 |
OGD | 0.001–100 μM in vitro | 1.5–12 h | Low dose increased neuron viability, decreased neuron death, increased BCL-2 expression; high dose decreased neuron and astrocyte viability, increased astrocyte death | Matsukawa et al6 |
Hypoxia-ischemia | 45 mg/kg, IP or SC | 12 h prior to hypoxia | Decreased infarct volume in rats, increased infarct volume in mice | Tsuji et al10 |
Hypoxia-ischemia | 45 mg/kg, SC, for 1 d, then 22.5 mg/kg SC for 2 d | Twice per d starting 12 h prior to hypoxia | Increased infarct volume in mice | Tsuji et al10 |
Hypoxia-ischemia | 135 mg/kg SC for 1 d, then 68 mg/kg for 2 d | Twice per d starting 12 h prior to hypoxia | Increased infarct volume in mice | Tsuji et al10 |
Hypoxia-ischemia | 45 mg/kg on d 1, then 22.5 mg/kg or 22.5 mg/kg on d 1, then 10 mg/kg IP | 1 week, starting 2 h after hypoxia | Decreased white matter injury, decreased microglial activation | Carty et al11 |
Hypoxia-ischemia | 50 mg/kg IP | Every 12 h for 4 d, starting immediately after hypoxia | Reduced white matter injury, reduced microglial activation | Lechpammer et al12 |
| ||||
Parkinson Disease | ||||
MPTP | 60, 90, or 120 mg/kg, oral gavage | 24 h after MPTP | Reduced neuron death, reduced microglial activation | Du et al13 |
Rotenone | 1, 5, 10 μM in vitro | 6 d | Decreased neuron death | Radad et al14 |
MPTP in monkey | 200 mg/kg, oral | 2 weeks during MPTP | Increased neuron death, worsened motor symptoms | Diguet et al15 |
MPTP | 45 or 60 mg/kg IP | Twice per d for 3 d | Increased neuron death, inhibited microglial activation | Yang et al16 |
Weaver mutant | 60 mg/kg, oral gavage | P10–P24 | Decreased neuron death | Peng et al17 |
| ||||
Huntington Disease | ||||
3-NP | 45 mg/kg IP | 5 d during 3-NP | Worsened behavioral function, increased neuron death | Diguet et al15 |
R6/2 mice | 5 mg/kg IP | Daily | Delayed disease progression, inhibited caspase expression, delayed mortality | Chen et al18 |
R6/2 mice | 1 or 10 mg/mL in drinking water | Daily | No change in behavioral function, no change in aggregate formation | Smith et al19 |
R6/2 mice | 5 mg/kg IP with CoQ10 | Daily | Improved behavioral function, decreased neuron damage, decreased aggregate formation | Stack et al20 |
Quinolinic acid injection | 50 mg/kg on d 1, 25 mg/kg after, IP | 7 or 21 d after injection | Decreased neuron death, decreased microglial activation | Ryu et al21 |
| ||||
Alzheimer Disease | ||||
Tg-SwDI mice | 50 mg/kg IP | Every other day for 28 d at 12 mo of age | Decreased microglial activation, decreased IL-6, improved behavioral function | Fan et al22 |
APP-tg mice | 55 mg/kg in chow | Daily for 3 mo | Improved cognitive performance, decreased microglial activation | Seabrook et al23 |
| ||||
Multiple Sclerosis | ||||
EAE | 45 mg/kg IP | For 14 d at symptom onset | Decreased clinical scores, decreased T-cell infiltration | Nikodemova et al24 |
| ||||
Amyotropic Lateral Sclerosis | ||||
SOD1 mice | 10 mg/kg IP | Daily starting at 5 wk of age | Delayed disease onset, prolonged survival | Zhu et al25 |
Abbreviations: 3-NP, nitropropionic acid; CoQ10, coenzyme Q10; COX-2, cyclooxygenase-2; EAE, experimental autoimmune encephalomyelitis; IL, interleukin; IP, intraperitoneal; IV, intravenous; MCAO, middle cerebral artery occlusion; MPTP, 1-methyl-4-phenyl-1,2,3,6-tetrahydropyridine; OGD, oxygen glucose deprivation; P, postnatal day; SC, subcutaneously; SOD1, superoxide dismutase 1.
Parkinson Disease
The effects of minocycline treatment on neurodegeneration in chemically induced Parkinson disease models have varied. While most studies found reduced microglial activation and neuronal cell death,13,14 others reported decreased microglial activation and enhanced neuron toxicity.15,16 In one genetic model, the Weaver mouse, minocycline treatment resulted in nigrostriatal neuroprotection.17
Huntington Disease
In a genetic model of Huntington disease (HD), the R6/2 mouse, one group reported that minocycline provided neuroprotection,15 another observed no clinical evidences of therapeutic efficacy,18 and a third reported that minocycline treatment was detrimental.19 Several studies investigated the effects of minocycline treatment combined with other therapies. Treatment with minocycline and pyruvate or coenzyme Q10 afforded significant neuroprotection in mice with HD,20,21 suggesting that minocycline may best be used as a combinatorial therapy.
Alzheimer Disease
While minocycline treatment produced mixed results in HD and Parkinson disease studies, it appears to be beneficial in experimental models of Alzheimer disease. Both in vivo and in vitro studies report suppressed microglial activation in Alzheimer disease models along with attenuated neuronal cell death, astrogliosis, and improved behavioral performance.22,23 Further studies are necessary to confirm that these beneficial effects lead to long-term neuroprotection.
Amyotrophic Lateral Sclerosis
Amyotrophic lateral sclerosis (ALS) is a neurodegenerative disease characterized by motor neuron death in the spinal cord, brainstem, and motor cortex. Mutations in superoxide dismutase 1 account for a proportion of cases of familial ALS, and mutant superoxide dismutase 1 transgenic mice provide a useful tool for treatment-testing paradigms. Minocycline treatment improved the symptoms of ALS in these mice via inhibition of cytochrome c release and caspase activation, thus delaying the onset of degeneration and muscle strength decline.25 Owing to the success of minocycline in these experimental trials, it quickly advanced to clinical trials but these yielded unexpectedly poor results (see “Clinical Studies” section).
Experimental Autoimmune Encephalomyelitis
Minocycline suppresses T-cell proliferation, migration, and cytokine production in an autoimmune animal model of multiple sclerosis, experimental autoimmune encephalomyelitis, and delays the onset and diminishes the severity of clinical neurological disability.24,28
Spinal Cord Injury
In animal models of spinal cord injury, minocycline treatment reduced microglial activation and astrogliosis and enhanced oligodendrocyte survival but reports of functional recovery were conflicting.2
CLINICAL STUDIES
The use of minocycline as an antibiotic as well as its excellent safety profile and penetration into the CNS spurred interest in using this drug to treat neurological diseases. Clinical trials are under way for the use of minocycline to treat several neurological diseases such as stroke, multiple sclerosis, spinal cord injury, Huntington disease, and Parkinson disease. Trials already completed have revealed both positive and negative effects (Table 2).
Table 2
Summary of Human Trials of Minocycline Treatment for Neurological Diseases
Disease | Dosage, mg/d | Route | Duration | Effect | Source |
---|---|---|---|---|---|
Acute stroke | 200 | Oral | 5 d | Lower NIHSS, lower mRS, increased BI | Lampl et al29 |
Spinal cord injury | 400 or 800 | Intravenous | 7 d | Improved ASIA, FIM, SCIM | Casha et al30 |
Multiple sclerosis | 200, with glatiramer acetate | Oral | 9 mo | Reduced gadolinium-enhancing lesions | Metz et al31 |
Parkinson disease | 200 | Oral | 18 mo | Passed the safety and efficacy trial | NINDS-NET-PD Investigators32 |
Huntington disease | 200 | Oral | 24 mo | Ameliorated psychiatric symptoms | Bonelli et al33 |
Huntington disease | 200 | Oral | 6 mo | No significant differences in UHDRS, AIMS, and MMSE | Thomas et al34 |
ALS | 200 | Oral | 9 mo | Declined ALSFRS-R | Gordon et al35 |
Abbreviations: AIMS, Abnormal Involuntary Movement Scale; ALS, amyotropic lateral sclerosis; ALSFRS-R, ALS functional rating scale; ASIA, American Spinal Injury Association; BI, Barthel Index; FIM, Functional Independence Measure; MMSE, Mini-Mental State Examination; mRS, modified Rankin Scale; NIHSS, National Institutes of Health Stroke Scale; SCIM, Spinal Cord Independence Measure; UHDRS, Unified Huntington Disease Rating Scale.
Brain Ischemia
A number of completed clinical studies reported beneficial effects of minocycline treatment without increased incidence of severe adverse events. In an open-label, evaluator-blinded study, oral administration of 200 mg/d of minocycline for 5 days 6 to 24 hours after acute CNS infarction caused no major complications, significantly decreased scores on the National Institutes of Health Stroke Scale and modified Rankin Scale, and increased Barthel Index scores in treated patients at days 7, 30, and 90 of follow-up. These observations suggest that the treatment of acute stroke with minocycline at this stage is associated with better clinical outcome.29 There are, however, several limitations to this study including the open-label design and small sample size. Future studies of minocycline administration after CNS infarction should include double-blind, controlled trials that use a broader window of treatment time (especially earlier) as well as oral and intravenous dosages.
Spinal Cord Injury
A small, phase 1/2 pilot study is under way to assess minocycline treatment in human patients after spinal cord injury. In this randomized, double-blind trial, patients receive 200 or 400 mg of minocycline intravenously twice daily for 7 days and are evaluated for 1 year. Results obtained thus far indicate that minocycline treatment enhances recovery, as shown by improved scores on the American Spinal Injury Association examination, Functional Independence Measure, Spinal Cord Independence Measure, London Handicap Score, and Short Form–36. These improvements were limited to patients with cervical spinal cord injury, whereas those with thoracic complete spinal cord injury showed no treatment effect.30 The sample size in this study is small, so conclusions of neuroprotection are preliminary.
Multiple Sclerosis
Several small clinical trials found minocycline treatment to be effective in reducing lesion size and occurrence rate in relapsing-remitting multiple sclerosis.36,37 More recently, a double-blind, placebo-controlled study was completed to evaluate the effects of minocycline combined with glatiramer acetate treatment in patients with relapsing-remitting multiple sclerosis. In this study, patients received 100 mg of minocycline twice daily combined with glatiramer acetate or glatiramer acetate/placebo for 9 months. The combination of drugs reduced the total number of gadolinium-enhancing lesions, the total number of new and enlarging lesions, and the total disease burden compared with glatiramer acetate/placebo treatment.31 Although the number of patients in this study was relatively small, it provides support for the use of minocycline as a combinatorial therapy for multiple sclerosis.
Parkinson Disease
In pilot clinical trials using minocycline treatment for Parkinson disease, 200 mg/d of minocycline was given for 18 months and patients were assessed for changes in the total Unified Parkinson Disease Rating Scale and a number of other measures to identify disability and symptoms.32 The patients treated with minocycline showed neither a decline in symptoms nor any increase in adverse events.
Huntington Disease
An initial open-label study found no obvious toxicity and observed improvements in motor function in minocycline-treated patients with HD based on their Unified HD Rating Scale and Mini-Mental State Examination after 6 months of treatment.33 In a 24-month follow-up, these authors reported stabilization of motor function and amelioration of psychiatric symptoms.38 The study included 14 patients, with only 11 included in the final analyses, making it difficult to draw firm conclusions. An 8-week, double-blind, randomized, placebo-controlled study by the Huntington Study Group in which patients with HD were treated with the same dose (100 or 200 mg/d) as the Bonelli group indicated that, although minocycline was well tolerated, it had no effect on Unified HD Rating Scale scores and actually worsened scores on the Stroop Interference test.39 Another small pilot study reported similar findings of good tolerability but lack of motor improvement on the Unified HD Rating Scale after 6 months of minocycline treatment.34
Amyotrophic Lateral Sclerosis
In a completed phase III clinical trial, patients with ALS received minocycline at up to 400 mg/d for 9 months; they were evaluated on the revised ALS functional rating scale and for forced vital capacity, manual muscle testing, quality of life, survival, and drug safety. Patients treated with minocycline showed faster deterioration than the placebo group according to the ALS functional rating scale and trended toward a faster decline in forced vital capacity and manual muscle testing scores. There was also a nonsignificant increase in mortality in the minocycline treatment group as well as gastrointestinal and neurological adverse events. Despite the negative effect of minocycline treatment on disease progression and adverse events, minocycline-treated patients described similar quality-of-life scores to the placebo patients.35 The authors of this study concluded that minocycline is not a viable treatment for ALS and warned against its use to treat other neurodegenerative diseases. Among possible reasons for this disappointing result are (1) that an inadequately low dosage schedule was used and (2) that the dosage of minocycline, by increasing glutamate receptor 1 phosphorylation and membrane insertion, promoted glutamate toxicity and motor neuron loss.40
SUMMARY OF AVAILABLE CLINICAL TRIALS DATA
While the clinical trials mentioned reveal both beneficial and deleterious results of minocycline treatment, all hope is not lost regarding its use as a future therapeutic. More studies are needed to confirm the appropriate timing and dosage, which may vary by disease. Some researchers speculate that doses of 100 to 200 mg/d, typically used in current clinical trials, may be ideal for humans to alleviate symptoms without toxic consequences. Others believe that this dose is too low, given that the typical dose for animal studies ranges from 10 to 100 mg/kg/d, which would theoretically equal approximately 3 to 7 g/d for humans. The dose of 200 mg/d provided improvement for acute stroke, yet had no effect on Parkinson disease or patients with HD. Thus, the possibility remains that increasing the dose of minocycline would improve its efficacy. In addition, minocycline treatment could be combined with other drug treatments in hopes of enhancing the beneficial effects without increasing the risk of harmful adverse effects seen with high doses of minocycline treatment. This therapeutic strategy has already proved useful in preliminary studies of multiple sclerosis and may be advantageous in a number of other diseases as well.
CONCLUSIONS
The practical utility of the neuroprotective effects of minocycline, though based on a substantial body of promising in vitro and animal studies, continues to be a matter of intense debate, with contradictory evidence ranging from neuroprotection to the exacerbation of toxicity in various experimental models and human trials. Studies to date have clearly established that minocycline exerts neuroprotective effects but have also shown that the actions of this drug are complex, and that its administration can, under certain circumstances, also have neurotoxic consequences. More studies, both clinical and preclinical, and both combinatorial and sequential strategies, should be carried out to ascertain the therapeutic window and the indications for which minocycline could be useful, effective, and safe.
Acknowledgments
Funding/Support: This study was supported in part by grants from the National Multiple Sclerosis Society, Roche Foundation for Anemia Research, and Feldstein Medical Foundation (Dr Deng); the Shriners Hospitals for Children (Drs Deng and Pleasure); and by grants R01 NS059043, R01 ES015988 (Dr Deng), and R01 NS025044 (Dr Pleasure) from the National Institutes of Health.
Footnotes
Financial Disclosure: None reported.
Author Contributions: Drs Plane and Shen had full access to all of the materials in the study and take responsibility for the integrity of the data and the accuracy of the data analysis. Study concept and design: Plane, Shen, Pleasure, and Deng. Acquisition of data: Plane, Shen, Pleasure, and Deng. Analysis and interpretation of data: Plane, Shen, Pleasure, and Deng. Drafting of the manuscript: Plane, Shen, Pleasure, and Deng. Critical revision of the manuscript for important intellectual content: Plane, Shen, Pleasure, and Deng. Statistical analysis: Pleasure. Obtained funding: Plane, Shen, Pleasure, and Deng. Administrative, technical, and material support: Plane, Shen, Pleasure, and Deng. Study supervision: Pleasure and Deng.
References
Full text links
Read article at publisher's site: https://doi.org/10.1001/archneurol.2010.191
Read article for free, from open access legal sources, via Unpaywall:
https://jamanetwork.com/journals/jamaneurology/articlepdf/801762/nnr05012_1442_1448.pdf
Subscription required at archneur.ama-assn.org
http://archneur.ama-assn.org/cgi/reprint/67/12/1442.pdf
Subscription required at archneur.ama-assn.org
http://archneur.ama-assn.org/cgi/content/full/67/12/1442
Free to read at archneur.ama-assn.org
http://archneur.ama-assn.org/cgi/content/abstract/67/12/1442
Citations & impact
Impact metrics
Citations of article over time
Alternative metrics
Smart citations by scite.ai
Explore citation contexts and check if this article has been
supported or disputed.
https://scite.ai/reports/10.1001/archneurol.2010.191
Article citations
Minocycline Acts as a Neuroprotective Agent Against Tramadol-Induced Neurodegeneration: Behavioral and Molecular Evidence.
Int J Prev Med, 15:47, 28 Sep 2024
Cited by: 0 articles | PMID: 39539580 | PMCID: PMC11559692
Alcohol, HMGB1, and Innate Immune Signaling in the Brain.
Alcohol Res, 44(1):04, 08 Aug 2024
Cited by: 0 articles | PMID: 39135668 | PMCID: PMC11318841
Review Free full text in Europe PMC
Emerging perspectives on precision therapy for Parkinson's disease: multidimensional evidence leading to a new breakthrough in personalized medicine.
Front Aging Neurosci, 16:1417515, 04 Jul 2024
Cited by: 0 articles | PMID: 39026991 | PMCID: PMC11254646
Review Free full text in Europe PMC
Cool the Inflamed Brain: A Novel Anti-inflammatory Strategy for the Treatment of Major Depressive Disorder.
Curr Neuropharmacol, 22(5):810-842, 01 Jan 2024
Cited by: 1 article | PMID: 37559243 | PMCID: PMC10845090
Review Free full text in Europe PMC
From Animal Models to Clinical Trials: The Potential of Antimicrobials in Multiple Sclerosis Treatment.
Biomedicines, 11(11):3069, 16 Nov 2023
Cited by: 1 article | PMID: 38002068
Review
Go to all (159) article citations
Similar Articles
To arrive at the top five similar articles we use a word-weighted algorithm to compare words from the Title and Abstract of each citation.
The potential of minocycline for neuroprotection in human neurologic disease.
Clin Neuropharmacol, 27(6):293-298, 01 Nov 2004
Cited by: 83 articles | PMID: 15613934
Review
Rise and fall of minocycline in neuroprotection: need to promote publication of negative results.
Exp Neurol, 189(1):1-4, 01 Sep 2004
Cited by: 59 articles | PMID: 15296829
Antibiotics acting as neuroprotectants via mechanisms independent of their anti-infective activities.
Neuropharmacology, 73:174-182, 04 Jun 2013
Cited by: 11 articles | PMID: 23748053
Review
Minocycline: far beyond an antibiotic.
Br J Pharmacol, 169(2):337-352, 01 May 2013
Cited by: 459 articles | PMID: 23441623 | PMCID: PMC3651660
Review Free full text in Europe PMC
Funding
Funders who supported this work.
NIEHS NIH HHS (1)
Grant ID: R01 ES015988
NINDS NIH HHS (3)
Grant ID: R01 NS025044
Grant ID: R01 NS059043
Grant ID: R01 NS025044-26