Abstract
Free full text

Future Directions in Malignant Hyperthermia Research and Patient Care
Abstract
Malignant hyperthermia (MH) is a complex pharmacogenetic disorder of muscle metabolism. To more closely examine the complexities of MH and other related muscle disorders, the Malignant Hyperthermia Association of the United States recently sponsored a scientific conference at which an interdisciplinary group of experts gathered to share new information and ideas. In this Special Article, we highlight key concepts and theories presented at the conference along with exciting new trends and challenges in MH research and patient care.
Introduction
Malignant hyperthermia (MH) is a pharmacogenetic disorder of muscle metabolism. Acute episodes of MH are usually precipitated by exposure to potent inhaled anesthetics and/or succinylcholine. At the cellular level, MH is characterized by abnormalities in excitation-contraction coupling and the control of myoplasmic calcium levels during muscle activation. In an effort to contribute to our understanding of these complex processes, the Malignant Hyperthermia Association of the United States recently sponsored a scientific conference (April, 2010). At the conference, an interdisciplinary group of experts including clinicians, geneticists involved in MH research and counseling, and basic scientists studying mechanisms of calcium regulation and dysregulation in normal and MH skeletal muscle were able to present and discuss new information and ideas as they relate to MH and other muscle disorders.
A broad range of topics was addressed, including: advances in understanding the pathophysiology of MH; genetic testing for MH susceptibility; the relationship between MH susceptibility and other muscle disorders; MH epidemiology; and future directions in clinical practice, educational initiatives, and MH-related research. (See Appendix for conference agenda, nothing speakers and topics.) In this Special Article, we have attempted to integrate key concepts and theories presented at the conference (Table 1) with those in the current literature. In doing so, we hoped to highlight both the trends and challenges in the field of MH research and patient care (Table 2).
Table 1
Key Concepts and Theories Presented at the Scientific Conference
|
MH (malignant hyperthermia); RyR1 (skeletal muscle ryanodine receptor); RyR1 (skeletal muscle ryanodine receptor gene); SOCE (Store-Operated Ca2+ Entry); ECCE (Excitation-Coupled Ca2+ Entry); CCD (Central Core Disease; SR (sarcoplasmic reticulum); SIFT (Sorting Intolerant from Tolerant); PolyPhen (Polymorphism Phenotyping); CHCT (caffeine-halothane contracture test); IVCT (in vitro contracture test); ACF (activated charcoal filter); MHS (MH-susceptible)
Table 2
Research Directions and Patient Care Issues: Selected Highlights
Basic Research | Patient Care |
---|---|
|
|
RyR1 (skeletal muscle ryanodine receptor gene); MH (malignant hyperthermia); CHCT (caffeine-halothane contracture test); IVCT (in vitro contracture test); MHS (MH-susceptible)
Malignant Hyperthermia Pathophysiology
Malignant hyperthermia (MH) has been described as a pharmacogenetic disorder of skeletal muscle involving dysregulated myoplasmic calcium (Ca2+), hypercontracture, and hypermetabolism in response to exposure to potent volatile anesthetics and depolarizing muscle relaxants. In some cases, the precipitating environmental factor may be exertional or heat stress (without pharmacologic trigger agents). Susceptibility to MH is transmitted in an autosomal dominant fashion with incomplete penetrance and variable expressivity. The skeletal muscle ryanodine receptor (RyR1) is the Ca2+ release channel within the sarcoplasmic reticulum (SR) membrane whose major known function is supplying the myoplasmic Ca2+ for contraction. It is widely accepted that the gene for this protein (RyR1) is the primary, though not exclusive site for mutations conferring susceptibility to MH, and that the aberrant channel plays a critical role in the development of this syndrome.1
Phenotypic Variability of RyR1 mutations
Thus far, more than 200 RyR1 variants2 associated with MH susceptibility and/or central core disease (CCD) have been identified, though only 30* of these have garnered sufficient evidence to be considered causal, and hence diagnostic, for MH susceptibility. The MH susceptibility mutations in RyR1 are reported to be present in only 50–70 percent of families that are susceptible to MH.3 Of the many RyR1 variants reported by Rosenberg et al.2, more than 150 are reported to be associated with MH, ~100 are reported to predispose to CCD, a rare myopathy that presents with slowly progressive weakness, and more than 20 appear to be associated with both MH and CCD. Furthermore, RyR1 mutations have also been associated with other myopathies such as multiminicore disease (MmD) and nemaline rod myopathy, though the linkage of these entities to RyR1 is far less certain than with CCD. Interestingly, mutations in RyR1 can produce a single disease state, such as MH susceptibility or CCD alone, or a combination of disease states, i.e., MH susceptibility and CCD together.4 The considerable degree of phenotypic variability associated with RyR1 mutations was a theme of this conference.
Regulation of Calcium Homeostasis
The regulation of intracellular Ca2+ is a focus for researchers attempting to better understand MH pathophysiology and the pathogenic implications of the different RyR1 phenotypes. In MH-susceptible (MHS) individuals, the release of Ca2+ through an abnormal RyR1 in the presence of volatile anesthetic activates an exaggerated increase in intramyoplasmic Ca2+ by an unknown mechanism. This increase in Ca2+ leads to muscle activation, often a prolonged state of muscle contracture, and more often the hypermetabolic response characteristic of MH.
Further understanding of MH pathophysiology may be provided by elucidating the mechanism of action of dantrolene, the direct-acting muscle relaxant that is the sole treatment for MH. Dantrolene has been shown to bind to RyR1 at a site distinct from the ryanodine binding site and to inhibit RyR1-dependent Ca2+ transients, thereby serving to decrease resting Ca2+ in the myoplasm, as well as decrease the Ca2+ transient after electrical excitation or exposure to caffeine or halothane.5–7 However, four decades after the discovery of dantrolene, the exact mechanism by which this compound exerts its pharmacological effects is still debated. Indeed, there is evidence that dantrolene may not have a direct effect on RyR1 channel properties per se8; rather, it may modulate Ca2+ levels indirectly by inhibiting processes which control Ca2+ entry into the myoplasm. Specifically, dantrolene may inhibit a RyR1-dependent variant of a process called Store-Operated Calcium Entry (SOCE), by which Ca2+ is brought into the cell through the plasma membrane from extracellular stores (see also Figure 1). Activation of SOCE occurs when the sustained opening of RyR1 channels depletes SR Ca2+ stores.9–13 Dantrolene appears to suppress that part of SOCE activity dependent upon RyR1.
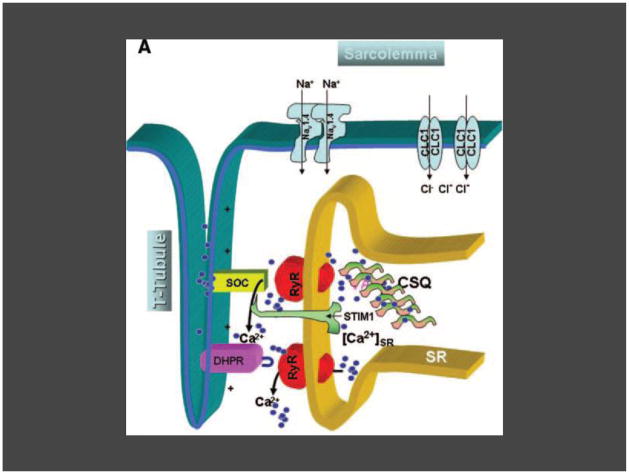
Depolarization of the sarcolemma is sensed by the DHPR (dihydropyridine receptor) in the T-tubule membrane. DHPR undergoes a conformational change and directly contacts RyR1 (the skeletal muscle ryanodine receptor) in the SR (sarcoplasmic reticulum) membrane, causing release of Ca2+ (primarily bound to CSQ [calsequestrin]) from SR stores (small blue circles). Ca2+ then becomes available for stimulation of the contractile apparatus (not shown). If the requirements for myoplasmic Ca2+ increase beyond that which can be provided by SR stores, SOCE (Store-Operated Ca2+ Entry) channels composed of Orai1 and likely subtypes of the TRP channel resident in the T-tubule membrane are activated by the SR membrane luminal Ca2+ sensor protein, STIM1, and allow more Ca2+ into the cell (SOCE). Alternatively, when muscle is highly stimulated, Ca2+ may enter the cell through the DHPR in a SR store-independent manner in the process termed excitation-coupled Ca2+ entry (ECCE). It is not yet known whether one or both processes are active during normal skeletal muscle physiology, or during pathophysiological processes such as malignant hyperthermia.
Figure and modified legend from the following citation- Parness J, Bandschapp O, Girard T. The myotonias and susceptibility to malignant hyperthermia. Anesth Analg 2009;109:1054- reprinted with permission (granted 3/8/11) from Wolters Kluwer Health.
In addition to SOCE, dantrolene also appears to affect other Ca2+ entry pathways such as excitation-coupled calcium entry, a store-independent Ca2+ entry pathway that is activated by repetitive or prolonged depolarization14 and SOICR (store overload induced calcium release), a process triggered by SR Ca2+ overload that leads to spontaneous release of SR Ca2+ into the cytoplasm.15 Investigators postulate that MH-causing mutations in RyR1 serve to lower the channel’s SOICR threshold, an effect increased by halothane and other volatile anesthetics. Dantrolene then appears to work by preventing the Ca2+ increase secondary to SOICR (as observed in isolated cell systems expressing mutant RyR1).15 These results suggest that dantrolene may act at multiple sites within the various physiological processes that underlie Ca2+ homeostasis in skeletal muscle, or that dantrolene works at a single site on RyR1 that affects coupling to all these different processes. Future research into the mechanism of action of dantrolene should lend insight into the regulation of skeletal muscle Ca2+ homeostasis, as well as the pathophysiology of MH and other skeletal muscle disorders.
Researchers studying the regulation of Ca2+ homeostasis and the role of RyR1 continue to propose and test different theories relating to the phenotypic variability observed in RyR1-related disorders. It appears that the rate of Ca2+ leak from the SR into the cytoplasm is one of the processes that may vary between MH phenotypes. It is theorized that RyR1 mutations causing a high leak rate may sufficiently deplete Ca2+ stores in the SR to produce a dearth of Ca2+ for sufficiently strong contractions during normal excitation-contraction coupling, thereby producing chronic myopathic weakness. How it would do this and not trigger replenishing of the SR Ca2+ store via SOCE is not clear. It is also postulated that at the same time these very same RyR1 mutations lower the threshold for activation of this channel by volatile anesthetics, allowing for the triggering of MH. Both of these phenomena in an affected individual would then account for both CCD and MH susceptibility in the same patient. In contrast, RyR1 mutations conferring a low leak rate might lower the threshold for triggering MH, but would not sufficiently deplete Ca2+ stores so as to cause a myopathy such as CCD. 4,16 Additionally, another mechanism that has been proposed to explain the phenotype of CCD alone is excitation-contraction (EC)-uncoupling, wherein activation of RyR1 by the DHPR (dihydropyridine receptor) is inefficient, and does not result in the typical degree of SR Ca2+ release and subsequent muscle contraction. EC-uncoupling thus results in the phenotypic muscle weakness and hypotonia observed in patients with CCD.16 Of note, the impact of RyR1 mutations on the bidirectional signaling between DHPR and RyR1 during EC coupling is also an active area of investigation by many research groups.17
As researchers piece together the underlying pathophysiology of these disorders, new approaches to treatment may be developed, including the use of drugs to alter Ca2+ release or leak, as well as the methodology to directly target specific gene mutations, such as with allele-specific gene silencing. This method uses siRNA (small interfering RNA), a class of double-stranded RNA molecules that interfere with the expression of a specific gene, to “target” or ‘silence’ the damaging mutant allele, while sparing the normal wild type allele.18 As a result, an increased proportion of wild type RyR1 is expressed, with an expected rescue of channel and muscle function. Allele-specific gene silencing is currently being studied as a potential means of decreasing the deleterious effects resulting from a damaging mutant allele in autosomal dominant disorders.
Histological Changes in Myopathic Muscle Cells
The regulation of Ca2+ release is also being studied with regard to its role in the development of histological changes observed in myopathic muscle cells. Zvaritch et al.19 have postulated that the random association of normal and mutant RyR1 subunits creates a heterogeneous group of RyR1 tetramers with a range of degrees of functionality, yielding variable responses of dysregulated Ca2+ release and muscle contraction. This is hypothesized to result in physical stresses among myofibrils, and tearing and shearing in focal areas. These areas expand to form minicores, cores, and nemaline rods. These core regions are the hallmark of CCD and are present in other myopathies such as MmD, reportedly (but not proven to be) associated with MH. Formation of these core regions has also been proposed to result from excessive SR Ca2+ leak, leading to the formation of reactive oxygen species and reactive nitrogen species.20 Accumulation of these species results in oxidative damage to the cell, disruption of mitochondria and the sarcotubular system, and the eventual degeneration of contractile machinery. Ca2+ stores are depleted, cores and rods are formed, and a myopathic state develops.21
The Link to Environmental Heat Stroke
In addition to RyR1-related myopathies, MH susceptibility may also share a common pathogenic mechanism with environmental heat stroke. Using mouse models of MH, investigators are actively studying the role of Ca2+ leak and oxidative stress in the development of environmental heat stroke.20 Mice with a specific knock-in MH mutation in RyR1 demonstrate muscle contractures, rhabdomyolysis and death in response to elevated temperatures, this in the absence of anesthetics. The proposed mechanism is similar to that described for core formation above, and involves excessive Ca2+ leak leading to the development of reactive nitrogen species. Subsequent S-nitrosylation of the mutant RyR1 channel occurs, leading to an increase in temperature sensitivity and the triggering of more Ca2+ release, resulting in prolonged muscle contracture and the hypermetabolic response typical of MH.
Indeed, the association of exertional heat illness and MH has been described in humans. There have been several case reports of individuals with histories of clinical MH who subsequently developed exertional rhabdomyolysis (ER) or exertional heat illness. In addition, as recently published by Capacchione et al.,22 a patient with a history of ER was reported to subsequently have experienced a clinical MH episode.23 This patient was later found to have several variants in the RyR1, CACNA1S (the alpha subunit of the DHPR), and CASQ1 (calsequestrin) genes, although the pathophysiological significance of these variants is unclear. Because these genes encode proteins with central roles in the process of excitation-contraction coupling and the regulation of intracellular Ca2+, the speculation is that perhaps variants from normal in these genes lead to enhanced sensitivity not only to the common triggers of MH (e.g., volatile anesthetics), but also to exercise and heat stress.
Future Research
Further study of the role of RyR1, CACNA1S, and CASQ1 (and the proteins they encode) in MH may be valuable because of the role their respective cardiac isoforms have in the development of CPVT (catecholaminergic polymorphic ventricular tachycardia). CPVT is an inherited syndrome manifest as sudden death related to mutations in the cardiac ryanodine receptor gene (RyR2). CPVT, like MH, occurs because of abnormal repetitive Ca2+ release from the SR, thereby causing cardiac arrhythmias.15,24,25 In fact, dantrolene has been shown to prevent CPVT in a knock-in mouse model, presumably by inhibiting Ca2+ leak through the cardiac RyR (RyR2). Dantrolene appears to correct the defective RyR2 interdomain interaction, illustrating again how studies of dantrolene’s mechanism of action may serve to elucidate the pathophysiology of an RyR-linked disorder.26
Oxidative damage and the regulation of Ca2+ release/Ca2+ leak thus appear to be important factors in the development of the histological changes apparent in the core myopathies, as well as in the physiological response observed in environmental heat stroke and MH. At the conference, current efforts to design new therapies for these disorders were discussed, including the evaluation of antioxidants and nitric oxide synthetase inhibitors, as well as the study of new ways to modulate RyR1 activity and Ca2+ homeostasis.
Genetics of MH
Identification of Gene Variants Causal for MH-Susceptibility – A Complex Matter
Very soon after the first description of the MH syndrome, investigators determined it was inherited in an autosomal dominant manner. With this observation, health care practitioners hoped it would be possible to identify MHS individuals by DNA analysis. However, it was not until 1990 that the primary gene locus for MH was identified as the RyR1 gene,27 and then several years after, a second genetic locus associated with MH was identified as CACNA1S, which encodes the α1 subunit of the DHPR.28 Subsequently, DNA testing for clinical use became available, but not until 2005, and then only for certain cases. During this time, mutations in RyR1 were also found to be causal for CCD and several other myopathies, while some cases of hypokalemic periodic paralysis were associated with mutations in DHPR.
Obtaining a diagnosis of MHS by analyzing the RyR1 and DHPR genes is both a difficult and time-consuming task. First, there is no single predominant mutation that is causal for MH in the human population. Second, RyR1 is a complex gene comprising 106 exons with a cDNA ~15,117kb,29 while CACNA1S spans 46 exons30 with a cDNA of ~6.16 kb28. Finally, RyR1 is characterized by allelic heterogeneity; that is, there are more than 200 RyR1 variants with potential functional significance in MH and/or CCD and possibly other diseases, as well as harmless (benign) variants (polymorphisms) found in more than 1% of the population.2 While some variants may ultimately be shown to be benign, not affecting amino acid sequence or function in the final protein, others may indeed be pathogenic as a result of replacement of generally conserved amino acids that lead to altered protein function. Sorting out the physiologic and clinical significance of these variants has been the focus of much of MH research over the past decade.
To determine whether a DNA variant is causally related to MH, several approaches are used. The variant must be characterized through genetic and functional studies, and meet certain requirements, as described by the European Malignant Hyperthermia Group†. First, the variant must be absent in at least 100 control patients. Second, the variant must be found in two unrelated families displaying clinical MH, and segregate with those who are MHS, either by clinical episode or by the caffeine-halothane contracture test (CHCT). Third, the DNA variant must represent a change in an amino acid that is ordinarily conserved across many species.
A widely accepted way to determine the significance of a DNA change or mutation is to incorporate the change into the gene for RyR1, and transfect that gene into a cell that does not normally express RyR1 (i.e., the human embryonic kidney or Chinese hamster ovary cell lines), or alternatively, to culture a myotube or immortalized lymphoblast from an affected individual. By quantitatively measuring Ca2+ release from the storage organelle in these cells after exposure to RyR1-agonists such as caffeine, or 4 chloro-m-cresol (4CmC), it is possible to determine whether the mutation causes enhanced Ca2+ flux, as found in cells from MHS individuals.1
More recently, proof of principle of pathogenicity of RyR1 mutations has been shown by incorporating an MH-causing RyR1 mutation into the genome of a mouse (a knock-in mouse) and demonstrating the development of MH in these animals in response to trigger agents.31 Such approaches are the most accurate in determining pathogenicity, but they are time-consuming and expensive. Thus, in order to assess which DNA variants are likely to be or to not be tolerated, various computational tools have been developed. One such tool is the SIFT, or Sorting Intolerant From Tolerant, program, which evaluates the significance of a particular DNA change (and thus amino acid substitution in a protein) based on its conservation in other cell systems. A prediction is then made regarding whether a specific amino acid substitution at a specific position in the protein will have a phenotypic effect.32 Another such tool, PolyPhen, (polymorphism phenotyping) evaluates whether an amino acid change is likely to be deleterious for a protein, based on its 3 dimensional structure and multiple alignments of homologous sequences.33
Applying these approaches to genetic testing for MH has resulted in the determination, as noted previously, that 30 DNA variants in RyR1 are indeed causal for MH, while three variants in DHPR have been shown to be causal for MH28,34. Overall about 30% of known MHS patients will demonstrate one of the known RyR1 causal mutations.35 However, up to 70% will demonstrate a DNA variant, whether it be a known causal mutation or a variant of unknown significance. Interpretation of genetic tests of MH susceptibility must be made carefully to differentiate those variants that are causal from those of unknown significance. It is therefore extremely important for both a trained genetic counselor and an MH expert to review the clinical findings along with the DNA results, in order to best assess the impact of these findings on the management of the patient and the diagnosis of MH susceptibility in their family.
Diagnostic Testing
For more than 40 years, the primary laboratory diagnostic test for MH continues to be the CHCT in North America. In Europe, the diagnostic test is known as the in vitro contracture test. The two tests use the same general approach of exposure of freshly biopsied skeletal muscle to caffeine and halothane, but they differ slightly in methodology and interpretation.36
After the introduction of DNA analysis, researchers began to compare findings of the contracture tests and DNA changes in the two genes that are associated with MH. In general the agreement is excellent, though there are reports of discordance.37–39 Such cases suggest there are as yet unknown factors responsible for the clinical expressivity of MH. Two approaches have been followed since then, first to refine the analysis of the contracture test in relation to the DNA test, and the second to further characterize the molecular genetics of MH in order to be able to apply DNA analysis as the primary test for MH susceptibility. In addition, Ginz et al. have recently described a new approach to resolving the differences between the contracture test and the genetic test results; the area under the curve of the contracture response to halothane or caffeine is analyzed, rather than the peak contracture response alone. By this approach, the sensitivity and specificity of predicting MH mutations has been reportedly improved to 93% and 95% respectively.40
Challenges Remain
Although much progress has been made in clarifying the molecular genetics of MH, there are still many challenges to overcome. At issue is the need to confirm a patient’s phenotype before DNA analysis is performed, because genetic testing undertaken in patients or family members with questionable clinical episodes is generally unproductive. There are several methods used to assist with phenotype confirmation; one tool is the Clinical Grading Scale, a scoring system relating the clinical signs of MH to the likelihood of an MH event.41 A second method is to investigate family history in detail for clinical MH crises expressed over several generations. Ultimately, in the absence of a definitive clinical event, the most useful evaluation tool in confirming phenotype is the CHCT in North America and the in vitro contracture test in Europe.42
Adding to the complexity of analyzing DNA variants is the observation that DNA mutation profiles (and possibly the functional effect of specific variants) may differ between human populations. For example, Sambuughin et al. have reported that a specific RyR1 variant associated with MH susceptibility in the Caucasian population may be a neutral polymorphic change in individuals of African descent.43 On the other hand, in some cases, the search for disease-causing mutations may be simplified because certain mutations are more prevalent in specific populations. For example, the G2434R mutation appears to be the most common in the United Kingdom, followed by T2206M and then G341R; whereas in Germany, the R163C, R614C, T2206M, G2434R and R2454H mutations are common.29 Such population-based variations are valuable for rapid screening of those at risk and their family members.
As noted previously, the penetrance and expression of the MH clinical phenotype appears to be quite variable among individuals who harbor RyR1 variants. A recent study by Carpenter et al.44 suggests that the severity of the MH phenotype may be correlated with the type of RyR1 variant, and mutations within highly conserved regions of the gene may, in fact, be associated with a more severe MH phenotype. It is also possible that there are other factors not related to DNA sequence changes (i.e., epigenetic factors), which may affect the expression of RyR1 variants. For example, Robinson et al.45 recently examined whether epigenetic allele silencing of RyR1 mutations was a contributing factor to the variable penetrance of MH susceptibility.
Although these investigators concluded that allele silencing of RyR1 was not likely to play a role in the inheritance of MH susceptibility, they did not discount the possibility that allelic silencing may be a contributing factor with respect to other genetic loci. Furthermore, reports of MHS patients and families who harbor more than one RyR1 variant39,43,46 lead us to question how the clinical expression of MH may be altered by the presence of more than one RyR1 variant. Is there a synergistic effect of these variants, leading to the preferential expression of MH susceptibility in certain individuals? Certainly, the discrepancy between the incidence estimates of MH events (discussed later in this article) and the much higher prevalence estimates of RyR1 variants within certain populations (1:2000 individuals by Monnier et al. 47 and 1:3,000 individuals by Ibarra et al.48) lends further support to unknown, possibly epigenetic, factors at work.
As we enter the era of molecular medicine, rapid progress is being made in DNA analysis. It is noteworthy that DNA sequencing costs have declined 15,000-fold over the past decade and are likely to decline further in the next decade. In the very near future it will be economically possible to sequence the entire coding region of a gene and then the entire genome through use of such techniques as exome sequencing. This will lead to the detection of many more DNA variants whose significance will need to be determined, and, if phenotype information is available, this should also greatly enhance our understanding of the relationship between genotype and phenotype. In the case of MH susceptibility, it is hoped that such techniques will lead to the identification of other DNA changes that account for cases of phenotypic MH without either RyR1 or DHPR mutations.
Genetic testing for MH susceptibility has certainly led to greater appreciation of the clinical presentation of MH. However, we are far from the goal of using genetic testing alone for determining whether a specific individual is or is not at risk for developing a clinical episode of MH.
Malignant Hyperthermia Epidemiology
Conference speakers presented data on the incidence of MH, the mortality and morbidity from MH events, the prevalence of MH susceptibility, and the demographics of patients experiencing MH events.
The existence of the MH clinical grading scale defining MH events and MH susceptibility41 and the international classification of disease (ICD) code for MH due to anesthetics were fundamental to recent progress. The MH clinical grading scale has facilitated analysis of cases reported to the North American Malignant Hyperthermia Registry and comparison of cases from diverse sources. The MH ICD code has permitted analysis of cause-of-death-files from the National Vital Statistics System49 and administrative databases such as the Nationwide Inpatient Sample50, the Healthcare Cost and Utilization Project State Inpatient Database51 and the Kids’ Inpatient Database.
Brady et al. have estimated the MH prevalence rate for the years 2001–2005 at 1.1/100,000 New York State surgical discharges with probable anesthetic exposures.51 However, as cited previously, Monnier et al. and Ibarra et al.’s analyses of individuals with homozygous or compound heterozygous mutations yielded an estimated MH susceptibility prevalence as high as 1 in 2,000–3,000 in the French and Japanese populations.47,48 These different prevalence estimates are a product of the fact that not all people are exposed to anesthetics and MH has variable expressivity.
Recent mortality estimates have been disparate partially as a function of the characteristics of the database used for analysis. Li et al. recently estimated the risk of MH-related mortality at 0.0082/100,000 United States surgical inpatients encompassing approximately 1% of all anesthesia-related deaths for the years 1999–2005.49 In contrast, Rosero et al.50 estimated the risk of MH-related mortality at 0.1310/100,000 United States inpatients. Li et al’s lower estimate of MH-related mortality is more likely than Rosero et al’s since the latter study used a less rigorously collected administrative database and it did not isolate medical from surgical patients.
While MH mortality rates are low, the rate of complications in acute MH episodes is much higher. Larach et al. found a 34.8% morbidity rate in 181 cases reported to the North American MH Registry between 1987 and 2006. Frequently reported complications included: change in consciousness level/coma, cardiac dysfunction, pulmonary edema, renal dysfunction, disseminated intravascular coagulation and hepatic dysfunction.52 The high complication rate emphasizes the need to continue to educate practitioners on the most effective way to diagnose and treat MH events and to study whether these efforts subsequently decrease the morbidity rate.
In the same study, Larach et al. also reported the demographic characteristics of 286 patients experiencing an MH event. They were predominantly male (75%) and young (median age of 22.0 years with 45% ≤ 19 years). Twenty-nine percent of patients had a muscular body build and 7% had a history of increased muscle tone. Before experiencing their MH event, 75% of these patients had undergone at least one general anesthetic and 51% had undergone 2 or more general anesthetics in which no signs of MH were observed.52 The experience of multiple prior unremarkable anesthetics illustrates the difficulty in determining the prevalence of MH susceptibility in the general population without an inexpensive, noninvasive MH diagnostic test. Furthermore, in a presentation based on a Kids’ Inpatient Database study for the years 2000, 2003, and 2006, Li et al. found that children with MH were 69% male and had co-morbid muscle ligament disease, muscular dystrophies, and strabismus.‡ Larach et al’s and Brady et al’s studies confirm earlier epidemiologic studies by Britt et al.53, Ording54, and Mauritz et al.55 which reported that this autosomal dominantly inherited disease with variable penetrance is expressed predominantly in the young and in males. Further molecular biologic and biochemical studies of the effects of age and gender on Ca2+ regulation and channel function are needed to elucidate the reasons for MH preferential expression.
Conference participants agreed that the success of future epidemiologic studies will depend upon continued reporting of adverse anesthetic events to the North American MH Registry, the creation of a separate ICD code for MH susceptibility history, and evaluation of current MH ICD coding accuracy by reviewing medical records at multiple institutions. Furthermore, future epidemiologic studies should focus on defining the clinical factors responsible for precipitating MH expression, including determining whether these factors behave differently with varying MH genotypes.
MH Susceptibility and Relationship to other Muscle Disorders
Assessment of Risk
The risk of MH or MH-like reactions in patients with known muscular disorders is not easy to determine. When such patients are scheduled for surgical procedures, this question becomes acute. Avoiding inhaled anesthetics may not make a general anesthetic safer because it is difficult to predict clinical perioperative events based on knowledge of the pathophysiology of the muscular disease. There have been many case reports and even small series that have discussed patients with myotonias, muscular dystrophies, core myopathies, and enzyme defects who developed one or more of the following symptoms: rigidity, increased temperature, arrhythmia, rhabdomyolysis, and hyperkalemia, during or after anesthesia. These reports were reviewed at the 2008 symposium sponsored by the Society for Pediatric Anesthesia and Malignant Hyperthermia Association of the United States (MHAUS), and subsequently published in a special series of articles.56–60 It is important to note that these reports are based on patients with the disease who had complications, not on the complete population with that disease; therefore, the incidence of serious postanesthetic complications in myopathic patients with these muscle diseases remains unknown.
RyR1-Related Myopathies - Closely Linked to MH Susceptibility
There is general agreement that certain rare myopathies, presenting with varying degrees of muscle weakness as well as differing clinical, genetic, and histologic findings are closely linked to MH susceptibility; specifically, individuals affected with King-Denborough syndrome, CCD or MmD are considered to be MHS.56,57 While a syndrome is defined by gross features of clinical presentation, myopathies such as CCD or MmD have been defined on the basis of histologic changes. Nevertheless, both syndromes and histologic findings may be associated with a variety of defects in genes and proteins. Current literature suggests that RyR1 variants are found in the majority of CCD cases. RyR1 variants are also associated with MmD, although the predominant form of MmD is most frequently associated with mutations in the selenoprotein N1 gene.57 In King-Denborough syndrome, inheritance is unclear and most cases appear to be sporadic; however, RyR1 mutations have been associated with this syndrome.56 It is thought that individuals who harbor a RyR1 variant may be at higher risk of MH than those with other gene variants; however, little has been published about anesthetic risk with regard to genotype in these disorders. Nonetheless, drugs that have caused MH are strongly contraindicated in individuals with either King-Denborough Syndrome, CCD or MmD.56,57,61
Where the Risk for MH-Susceptibility is Unclear
Other muscle disorders in which the risk of MH-like reactions is less clear include those caused by enzyme deficiencies such as carnitine palmitoyltransferase type II (CPTII) deficiency, McArdle’s disease and Myoadenylate Deaminase Deficiency; as well as those caused by abnormalities in other proteins, such as in Duchenne Muscular Dystophy (DMD) and Becker Dystrophy (BD), and those in which the etiology is unknown such as Asymptomatic HyperCKemia.
Enzyme deficiencies compromise the energy supply of muscle. When muscle affected by enzyme deficiency is exercised, rhabdomyolysis (increase of the muscular form of serum creatine kinase more than 5 times the upper limit of normal) often occurs. In dystrophinopathies such as DMD and BD, there is continual muscle breakdown, rhabdomyolysis, and muscle regeneration until the muscle becomes fibrotic. While no causative RyR1 mutation has been identified in these dystrophic diseases, there may be alteration of normal RyR1 channel function contributing to abnormal Ca2+ homeostasis. In fact, investigators studying the mechanism of altered Ca2+ homeostasis in a mouse model of DMD, recently reported that RyR1 Ca2+ release channels are leaky (due to hypernitrosylation of the channel protein) in dystrophic muscle, thus contributing to muscle weakness and damage.62 Thus, for many reasons, individuals with these enzyme deficiencies or dystrophinopathies may be at an increased risk of rhabdomyolysis during and after anesthesia.
There is debate on recommendations regarding anesthetics in all of these conditions, myopathies, enzyme deficiencies and myotonias. Succinylcholine should be avoided because it causes depolarization of the muscle and some degree of increased resting tension in the process of inducing neuromuscular block.63 Muscle metabolism in normal muscle increases as repolarization occurs after removal of succinylcholine.64 Thus, succinylcholine causes muscle injury in people with normal muscle and significant rhabdomyolysis in myopathic patients.65 Furthermore, there have been reports of hyperkalemic cardiac arrest in patients with subclinical myopathies.66 In contrast, inhaled anesthetics less frequently produce clinically significant increases in rhabdomyolysis in patients with dystrophinopathy or muscle enzyme deficiency.
Evaluating Rhabdomyolysis
The need for a comprehensive evaluation of unexplained episodes of rhabdomyolysis was emphasized during the conference (Figure 2). The cause of rhabdomyolysis should be diagnosed because it has significant implications for relatives of the patient as well as for the medical management of the index case. All potential enzyme defects in muscle which may mimic one or another symptom of MH must be considered before jumping to a diagnosis of MH susceptibility. Evaluation of family history, the presence of other medical conditions, and a complete medical and neurological examination should guide the physician in ordering diagnostic tests. The neurologist may detect signs of occult myopathy, such as a preclinical dystrophinopathy, which were not detected by other physicians. The Exercise Intolerance Panel (DNA tests which screen for common mutations in the myophosphorylase [PYGM], myoadenylate deaminase [AMPD1], and carnitine palmitoyltransferase [CPTII] genes) and other enzyme analyses where deficiencies may underlie other possible metabolic myopathies may be useful. Genetic testing is available for many structural myopathies. If these assays are not diagnostic, it is recommended that a muscle biopsy and myoglobinuria panel be performed to analyze muscle tissue for the presence of other metabolic diseases which cannot be identified using only blood samples (e.g., mitochondrial myopathy). In addition, the bioassay diagnostic for MH susceptibility, the CHCT, may be completed as well. Viable muscle is needed to perform a valid CHCT. If MH susceptibility is diagnosed by CHCT, examination of RyR1 may facilitate evaluation of MH status in relatives. As noted at the conference, one must be careful because the validity of a positive CHCT in a patient with an existing myopathy is questionable.
Association of Heat- and Exercise-Induced Rhabdomyolysis with MH Susceptibility
As mentioned earlier, the link between heat and exercise-induced rhabdomyolysis and susceptibility to MH is an active area of investigation at both the mechanistic level in animal models, as well as at the clinical case level.20,23 It is difficult to make a strong statement regarding the incidence of ER and heat-related illness (EHI) in MHS humans because data are limited, and there are many causes of ER and EHI which are not related to MH susceptibility.23 Interestingly, new techniques discussed at this conference, such as heat tolerance testing used in the military, may prove to be useful tools for evaluating heat tolerance in MHS individuals.67,68 Because ER and EHI can occur in normal people, a more extensive investigation of potential genetic risk factors for these disorders is usually delayed until a second episode of ER occurs.
Risk Factors for Metabolic Muscle Disease
A more comprehensive testing strategy may help clinicians uncover the causes of previously unexplained myopathic symptoms. In addition, new research suggests that in the future, we may also be able to predict an individual’s predisposition for metabolic muscle disease based on genetic risk factors. For example, investigations into the development of myopathies in patients taking statin medications (to lower cholesterol) suggest that underlying genetic variants may play a role in determining whether a myopathy develops. In fact, Vladutiu et al. reported that in clinical trial patients taking statins, those who developed a myopathy were more likely to be carriers of CPTII deficiency and myophosphorylase deficiency (McArdle’s disease) when compared to those patients who did not develop a myopathy.69,70 In addition, a recently published study examining the pathologic effect of statins suggests that a preexisting impairment in Ca2+ homeostasis, such as in MHS muscle, confers an increased risk for statin-induced myopathy, since it appears that statins may have a pathologic effect on intracellular Ca2+ handling.71 Furthermore, an MH-like syndrome, without documentation of increased carbon dioxide production, was reported to occur in a child heterozygous for a mutation in the CPTII gene after exposure to succinylcholine and halothane. This raises the possibility that the presence of a pathogenic mutation in an apparently normal CPTII heterozygote may be a significant risk factor upon exposure to drugs capable of producing rhabdomyolysis.60 It will be interesting to follow future research efforts aimed at identifying genetic risk factors for metabolic muscle disease.
In addition, there has been described a MH-like syndrome with rhabdomyolysis in patients with recent onset of type-1 diabetes.72 Clearly, when speaking of a syndrome such as MH that presents without pathognomonic signs, assigning pathophysiological causality with certainty is fraught with difficulty, and lends itself to incorrect diagnostics. Hence, there is a need for a comprehensive approach to diagnostics, and a continued intensive research program designed to identify the various potential causes of MH and MH-like syndromes.
Future Directions in Patient Safety
Anesthesia Workstations for the MHS Patient
As anesthesia machines have evolved into complex anesthesia workstations, it has become more challenging to prepare a “clean machine” for MHS patients and to safely ventilate patients’ lungs using workstations during acute MH crises. As reviewed by Kim and Nemergut73, the problem is two-fold. First, the ventilator is now part of the internal gas delivery system. Second, larger amounts of volatile-absorbent plastic and rubber materials are incorporated into the anesthesia workstation. Thus, even when the vaporizers are turned off, the workstation may continue to deliver a significant dose of potent inhaled anesthetics fueling an on-going MH event or triggering a new event in MHS individuals.
In recognition of these developments, MHAUS has suggested that anesthesiologists contact manufacturers for workstation volatile anesthetic washout procedures. Manufacturers responded by suggesting that anesthesiologists contact MHAUS. To break this cycle, future research, carefully coordinated with manufacturers, is critical. A suggested approach is the following: (1) determine the minimum concentration of anesthetic gas required to trigger a MH crisis in MHS swine; (2) evaluate the safety and efficacy of activated charcoal filters (ACF) placed on the inspiratory limb of the breathing circuit to scrub volatile anesthetics from various workstations; (3) examine each major type of workstation and determine a safe and effective method for preparing each of them for use in MH patients. These issues should be addressed by conducting both in vitro and in vivo experiments with MHS swine, the best large animal model for MH events.74
Preliminary in vitro work in this area by Jantzen and Kleemann75 and Gunter et al.76 has demonstrated the utility of an ACF placed in the inspiratory circuit for decreasing inspiratory inhaled anesthetic concentrations. Recently, Birgenheier et al.77 have also demonstrated the utility of Food and Drug Administration-approved ACFs placed in both the inspiratory and expiratory limbs of contaminated Apollo and Aestiva workstations after their anesthesia circuits and rebreathing bags were changed. The ACFs served to decrease volatile anesthetic vapor concentrations to less than 5 ppm in less than 2 minutes and maintain these low concentrations for 60 to 90 minutes.
While awaiting these future studies, a safer method for managing MHS patients may be to use the FDA-approved ACF with a clean circuit and rebreathing bag and taped off anesthetic vaporizers, while administering high gas flows. It is important to remember, however, that the filters need to be changed every 90 minutes. In the opinion of two authors (MGL and BWB) of this manuscript, for patients experiencing an acute MH event, the filter use should probably be limited to 60 minutes only; the acute MH patient should then be placed on an uncontaminated ventilator with subsequent sedation/anesthetic provided IV. In the chaos of treating an MH event, changing machines after an hour may be preferable to changing the filters every hour.
Temperature Monitoring
Another patient safety issue is temperature monitoring. Larach et al. advocated for electronic monitoring of core temperature in all anesthetics expected to last 30 minutes. Reasons for this recommendation include the following: temperature abnormalities may be an early MH sign; higher maximum temperatures significantly increase the likelihood of MH complications including disseminated intravascular coagulation; and skin liquid crystal temperature probes do not trend accurately during MH events.52 Participants noted that safe and effective nasal electronic temperature probes should be manufactured to substitute for current “repurposed” and inaccurate surface temperature probes.
Additional Preparation for MH Needed at Free-Standing Health Care Facilities
While all hospital-based and free-standing anesthetizing facilities, such as ambulatory surgery and office-based surgery centers, should be prepared to diagnose and treat an infrequently occurring MH event, the more thinly resourced free-standing facilities will find advanced preparation even more essential. Meeting participants recommended that free-standing facilities should: conduct MH drills, check for the ready availability of 36 vials of dantrolene§, plan to obtain critical laboratory studies, and develop transfer plans** for stabilized patients to appropriate hospital facilities. Advance preparations are required to ensure the survival of these critically ill patients. If a free-standing facility does not use volatile anesthetics and does not depend on succinylcholine as an airway rescue drug, then such preparation is unnecessary, assuming that myopathic patients are not treated at that facility. Multiple MHAUS Hotline reports of MH deaths occurring after anesthetics delivered in outpatient surgery facilities highlight the importance of adequate preparation for MH crises.
Communication
Finally, patient safety mandates good communication and transparency between primary care providers and their consultants, physicians and their patients, and MHS individuals and their families.†† Clear communication coupled with education saves lives. Furthermore, continued collaboration between MH researchers and clinicians will advance the science of MH and inform educational efforts to improve the care of those who are MHS or who have related disorders.
Conclusion
In this article, we have attempted to provide the reader with an overview of the current trends in MH-related research and patient care, as highlighted at the Scientific Conference. While it was not possible to go into great detail about each topic, we hoped to communicate the need for further evaluation in such areas as:
the physiology and regulation of Ca2+ homeostasis and the impact of temperature on such processes;
factors involved in the predisposition of an individual to triggerable myopathies;
genotype – phenotype relationships, and the reasons behind incomplete penetrance with regard to the clinical expressivity of MH.
Continued progress in our understanding of these subjects will no doubt translate into the development of new methods to diagnose, treat, and care for patients with MH and related disorders.
Acknowledgments
Funding: Funding for this conference, on which this report is based, was, in part, from an R13 Grant (1 R13AR05422-01 from the National Institute of Arthritis and Musculoskeletal and Skin Diseases (NIAMS), including co-funding from the Office of Rare Disease Research (ORDR). Funding was also generously contributed by the Dept. of Anesthesiology, UPMC, Mercy Hospital.
Information for LWW regarding depositing manuscript into PubMed Central: This research was funded by National Institutes of Health grant number 1 R13AR05422-01.
We would like to thank Dr. Sheila Muldoon for reviewing and providing suggestions to improve this manuscript; we would also like to thank Dr. John Capacchione for his valuable input.
Appendix I
AGENDA Day 1- Friday, April 23, 2010
Topic | Speaker(s) |
---|---|
Welcome and Introductions | Henry Rosenberg, MD, President, MHAUS Andrew Herlich, MD, UPMC and John P. Williams, M.D., UPMC |
Pathophysiology of MH Overview and Recent Advances in Understanding | Session Chairperson: Jerome Parness, MD, PhD, UPMC |
Introduction to MH Pathophysiology; fresh approaches to understanding MH at cellular level; dantrolene & mechanism of action | Jerome Parness, MD, PhD, UPMC |
Lessons from a Mouse Model of Malignant Hyperthermia: Mechanisms and New Interventions | Susan Hamilton, PhD, Baylor College of Medicine (Keynote Speaker) |
Mechanistic Models for the Cause of MH and for Core Formation in CCD | David MacLennan, PhD University of Toronto |
New Frontiers in Personalized Medicine: RyR1 Allele-Specific Gene Silencing in Mouse Models of MH and CCD | Robert T. Dirksen, PhD University of Rochester |
Changes in DHPR kinetics induced by MH-related conformational changes in RyR1 | Paul Allen, MD, PhD Brigham and Women’s |
Genetics of MH: Results and Future Directions | Chairperson: Sheila Muldoon, MD, USUHS |
Genetic testing for MH susceptibility in Europe; role of new diagnostic testing strategies in evaluating MH risk | Henrick Rueffert, MD University of Leipzig, Germany |
Reassessment of the operating characteristics of IVCT given genetic RyR1 results; update on the EMHG Reference: Improve on Phenotyping | Thierry Girard, MD University Hospital of Basel, Switzerland |
Results of genetic testing (RyR1 gene findings) in basic research laboratories | Khishge Sambuughin, PhD, USUHS |
Assessment of RyR1 gene variants for Pathogenicity: Theory and Examples | Jeffrey Kant, MD, PhD UPMC |
Results of genetic testing (RyR1 gene findings) in CLIA-approved clinical laboratories | Jim Weber, PhD Prevention Genetics |
RyR1 (skeletal muscle ryanodine receptor gene); MH (malignant hyperthermia); CHCT (caffeine-halothane contracture test); IVCT (in vitro contracture test); MHS (MH-susceptible); central core disease (CCD)
Topic | Speaker(s) |
---|---|
Welcome, Comment on Day 1 | Sheila Muldoon, MD, USUHS |
Relationship between MH-susceptibility and other muscle conditions/disorders | Chairperson: Barbara Brandom, MD, UPMC |
Review of 2008 SPA Meeting Recommendations (anesthetic considerations in MH-associated diseases) | Barbara Brandom, MD UPMC |
Creatine Kinase and Heat Tolerance in Mice and Men | Patricia Deuster, PhD, MPH USUHS |
Confounding Cases: Is this MH and How Would You Provide Anesthesia? | John Capacchione, MD USUHS |
Genetic susceptibility to statin-induced myopathy | Georgirene Vladutiu, PhD SUNY at Buffalo |
MH Epidemiology | Chairperson: Henry Rosenberg, President, MHAUS |
Introduction to Epidemiology of MH; role of MH Hotline and North American MH Registry (NAMHR) databases as research and educational tools; implications and limitations of epidemiologic studies, Epidemiology Slide Handout | Henry Rosenberg President, MHAUS |
MH Comorbidities | Joanne E. Brady, SM, Columbia University |
What the Registry has Taught Us: MH Presentation, Treatment, and Outcome; Is it Time for Revisions to MHAUS Clinical Care Recommendations? | Marilyn Larach, MD Penn State College of Medicine |
Future Directions/Challenges | Chairperson: Henry Rosenberg, MD, President, MHAUS |
Changing Recommendations for Anesthesia Machine Preparation | David Rymer, MD UPMC |
Need for building the NAMHR database as source for future clinical trials; Recent reporting trends; Current contributions from the MH Diagnostic | Barbara Brandom, MD UPMC |
Welcome, Comment on Day 1 | Sheila Muldoon, MD, USUHS |
Centers | |
Patient Safety First | Dale Micalizzi Board of Directors, MHAUS |
Closing Remarks: Future directions for MH – research and non-invasive diagnostic testing; Future Directions for MHAUS – patient safety initiatives (ambulatory surgery issues, educational initiatives [simulation]); advice for MHS individuals. | Henry Rosenberg, MD MHAUS |
Footnotes
Conflict of Interest: See Disclosures at the end of the article
Disclaimer
The views expressed in this article do not necessarily reflect the official policies of MHAUS or of the Department of Health and Human Services; nor does mention of trade names, commercial practices, or organizations imply endorsement by Malignant Hyperthermia Association of the United States or the United States Government.
This report was previously presented, in part, at the MHAUS Scientific Conference, April, 2010, which was the subject of an article in Anesthesiology News (Brief Editorial) and an article in the ASA NEWSLETTER.
Reprints will not be available from the authors.
*See www.emhg.org for a listing of RyR1 mutations deemed causative for MH susceptibility by the European Malignant Hyperthermia Group (last accessed 3/11/11).
†See www.emhg.org for a listing of criteria to be used in genetic testing of MH susceptibility (last accessed 3/11/11).
‡Li G, Brady JE, Rosenberg H, and Sun LS. Excess comorbidities associated with malignant hyperthermia in children. Presented at the International Anesthesia Research Society’s Annual Meeting, Honolulu, Hawaii, March 20–23, 2010.
§25% of 229 MH events required more than 36 vials of dantrolene for treatment. (Larach et al., 2010)
**See www.mhaus.org for guidance for ambulatory surgical centers with regard to the development of emergent MH transfer plans for suspected MH patients (last accessed 3/11/11).
††16 of 248 patients experiencing an MH event had an MH family history (Larach et al., 2010).
DISCLOSURES
Name: Sharon J. Hirshey Dirksen, PhD
Contribution: This author helped write the manuscript.
Conflicts: Sharon J. Hirshey Dirksen reported no conflicts of interest.
Attestation: Sharon J. Hirshey Dirksen approved the final manuscript.
Name: Marilyn Green Larach, MD, FAAP
Contribution: This author helped write the manuscript.
Conflicts: Marilyn Green Larach received an honorarium from MHAUS to help develop Transfer Guidelines for MH patients in ambulatory surgery centers.
Attestation: Marilyn Green Larach approved the final manuscript.
Name: Henry Rosenberg, MD
Contribution: This author helped write the manuscript.
Conflicts: Henry Rosenberg reported no conflicts of interest.
Attestation: Henry Rosenberg approved the final manuscript.
Name: Barbara W. Brandom, MD
Contribution: This author helped write the manuscript.
Conflicts: Barbara Brandom reported no conflicts of interest.
Attestation: Barbara Brandom approved the final manuscript.
Name: Jerome Parness, MD, PhD
Contribution: This author helped write the manuscript.
Conflicts: Jerome Parness reported no conflicts of interest.
Attestation: Jerome Parness approved the final manuscript.
Name: Robert Scott Lang, MD
Contribution: This author helped write the manuscript
Conflicts: Robert Scott Lang reported no conflicts of interest
Attestation: Robert Scott Lang approved the final manuscript
Name: Meera Gangadharan, MD
Contribution: This author helped write the manuscript.
Conflicts: Meera Gangadharan reported no conflicts of interest.
Attestation: Meera Gangadharan approved the final manuscript.
Name: Tyler Pezalski, MD
Contribution: This author helped write the manuscript.
Conflicts: Tyler Pezalski reported no conflicts of interest.
Attestation: Tyler Pezalski approved the final manuscript.
Contributor Information
Sharon J. Hirshey Dirksen, Malignant Hyperthermia Association of the United States, Pittsford, NY.
Marilyn Green Larach, The North American Malignant Hyperthermia Registry of MHAUS.
Henry Rosenberg, St. Barnabas Medical Center, Livingston, New Jersey.
Barbara W. Brandom, Department of Anesthesiology, Children’s Hospital of Pittsburgh, University of Pennsylvania Medical Center, Pittsburgh, Pennsylvania.
Jerome Parness, Department of Anesthesiology, Children’s Hospital of Pittsburgh, University of Pennsylvania Medical Center, Pittsburgh, Pennsylvania.
Robert Scott Lang, University of Pennsylvania Medical Center, Pittsburgh, Pennsylvania.
Meera Gangadharan, University of Texas Medical Branch at Galveston, Texas.
Tyler Pezalski, Uniformed Services University of the Health Sciences, Washington, DC.
References
Full text links
Read article at publisher's site: https://doi.org/10.1213/ane.0b013e318222af2e
Read article for free, from open access legal sources, via Unpaywall:
https://journals.lww.com/anesthesia-analgesia/fulltext/2011/11000/future_directions_in_malignant_hyperthermia.28.aspx
Citations & impact
Impact metrics
Article citations
Association of malignant hyperthermia and exertional heat illness in young athletes: An analysis of awareness among clinical and athletic first responders.
Saudi J Anaesth, 18(2):224-230, 14 Mar 2024
Cited by: 0 articles | PMID: 38654868 | PMCID: PMC11033909
Pediatric Hyperglycemic Hyperosmolar Syndrome: A Comprehensive Approach to Diagnosis, Management, and Complications Utilizing Novel Summarizing Acronyms.
Children (Basel), 10(11):1773, 31 Oct 2023
Cited by: 1 article | PMID: 38002864
Review
An Assessment of Penetrance and Clinical Expression of Malignant Hyperthermia in Individuals Carrying Diagnostic Ryanodine Receptor 1 Gene Mutations.
Anesthesiology, 131(5):983-991, 01 Nov 2019
Cited by: 13 articles | PMID: 31206373 | PMCID: PMC9912949
Malignant Hyperthermia.
Mo Med, 116(2):154-159, 01 Mar 2019
Cited by: 8 articles | PMID: 31040503 | PMCID: PMC6461318
Review Free full text in Europe PMC
Nitric Oxide-induced Activation of the Type 1 Ryanodine Receptor Is Critical for Epileptic Seizure-induced Neuronal Cell Death.
EBioMedicine, 11:253-261, 13 Aug 2016
Cited by: 21 articles | PMID: 27544065 | PMCID: PMC5049986
Go to all (16) article citations
Similar Articles
To arrive at the top five similar articles we use a word-weighted algorithm to compare words from the Title and Abstract of each citation.
Malignant hyperthermia and the clinical significance of type-1 ryanodine receptor gene (RYR1) variants: proceedings of the 2013 MHAUS Scientific Conference.
Can J Anaesth, 61(11):1040-1049, 05 Sep 2014
Cited by: 20 articles | PMID: 25189431 | PMCID: PMC4276369
[Malignant hyperthermia--diagnostics, treatment and anaesthetic management].
Anasthesiol Intensivmed Notfallmed Schmerzther, 44(11-12):758-63; quiz 764, 16 Nov 2009
Cited by: 10 articles | PMID: 19918708
Review
[Anesthetic malignant hyperthermia: what's new in 2012?].
Ann Fr Anesth Reanim, 32(1):e43-7, 03 Jan 2013
Cited by: 0 articles | PMID: 23290613
Review
[Malignant hyperthermia. The ugly].
Anaesthesist, 56(9):923-929, 01 Sep 2007
Cited by: 2 articles | PMID: 17565473
Review
Funding
Funders who supported this work.
NIAMS NIH HHS (2)
Grant ID: 1 R13AR05422-01
Grant ID: R13 AR059422-01