Abstract
Free full text

Bacterial biosynthesis and maturation of the didemnin anticancer agents
Abstract
The antineoplastic agent didemnin B from the Caribbean tunicate Trididemnum solidum was the first marine drug to be clinically tested in humans. Because of its limited supply and its complex cyclic depsipeptide structure, considerable challenges were encountered during didemnin B's development that continue to limit aplidine (dehydrodidemnin B), which is currently being evaluated in numerous clinical trials. Herein we show that the didemnins are bacterial products produced by the marine α-proteobacteria Tistrella mobilis and Tistrella bauzanensis via a unique post-assembly line maturation process. Complete genome sequence analysis of the 6,513,401 bp T. mobilis strain KA081020-065 with its five circular replicons revealed the putative didemnin biosynthetic gene cluster (did) on the 1,126,962 bp megaplasmid pTM3. The did locus encodes a 13-module hybrid nonribosomal peptide synthetase-polyketide synthase enzyme complex organized in a co-linear arrangement for the synthesis of the fatty acylglutamine ester derivatives didemnins X and Y rather than didemnin B as first anticipated. Imaging mass spectrometry of T. mobilis bacterial colonies captured the time-dependent extracellular conversion of the didemnin X and Y precursors to didemnin B in support of an unusual post-synthetase activation mechanism. Significantly, the discovery of the didemnin biosynthetic gene cluster may provide a long-term solution to the supply problem that presently hinders this group of marine natural products and pave the way for the genetic engineering of new didemnin congeners.
Introduction
Sessile marine invertebrates such as sponges and tunicates are intimately linked with microbes that are consumed as food and hosted for their protective chemistry. These marine animals are rich sources of structurally diverse collections of unique bioactive molecules that in some cases have been developed into drugs for the benefit of human health.1,2 A major challenge in the drug development of marine natural products, however, has historically been the difficulty in supplying sufficient material for pre-clinical and clinical evaluation due to natural limitations of the source organism.3 Marine products are frequently endowed with complex organic structures that complicate their total syntheses in large scale to meet the demands of a drug development program.4 Microbes are commonly suggested as the actual producers of many molecules originally isolated from invertebrate tissues, and thus microbial fermentation offers the promise of a renewable supply of important marine drug candidates.5 However, very few examples exist in which clinically relevant compounds originally extracted from marine invertebrates are produced by cultured microbes. Herein we show that the didemnin family of anticancer agents, including didemnin B as the first marine natural product clinically tested in humans,6 is produced by marine bacteria of the genus Tistrella through an unexpected biosynthetic pathway.
Invertebrate-derived natural products, especially those with complex polyketide and nonribosomal peptide structures, are generally proposed to be microbial in origin since the molecular basis governing their biosyntheses resides exclusively in microbes.7–9 Metagenomic studies have revealed the microbial production of noteworthy bioactive natural products from diverse marine invertebrates, including the pederin group of polyketides from sponges,10 the patellamides and ecteinascidin-743 (Yondelis) from tunicates,11,12 and the bryostatin macrolides from bryozoans.13 In each case, uncultivated bacterial symbionts are thought to be the natural producers. Free-living marine microbes are often not implicated in the synthesis of marine invertebrate natural products, although a field-collected marine cyanobacterium was shown to harbor the sponge macrolide swinholide A.14 As a consequence, molecular engineering approaches involving whole pathway expression are envisaged to produce complex molecules from marine invertebrate symbionts in laboratory microbial strains in order to develop a renewable supply of the compound and allow for the rational engineering of new chemical entities to probe structure-function relationships.15 To date, only the cyanobactin group of ribosomal peptides, which includes the patellamides, has been heterologously produced and engineered.16 Complex polyketides, nonribosomal peptides and hybrids thereof, on the other hand, present an unmet technical challenge due to their large biosynthetic gene clusters that often exceed 100 kb.
Didemnins (Fig. 1) are cyclic depsipeptides with remarkable antitumor, antiviral and immunosuppressive properties6 that were first discovered in the Caribbean tunicate Trididemnum solidum in 1981.17 The most potent analog didemnin B was the first marine natural product to enter clinical trials as an anticancer agent, yet ultimately failed to demonstrate effective antitumor activity while displaying cardiac and neuromuscular toxicities18,19 A closely related compound, dehydrodidemnin B (aplidine), was isolated from the Mediterranean tunicate Aplidium albicans and shown to be more potent and less toxic despites its minor structural change.20,21 Aplidine has since replaced didemnin B in clinical trials and is currently in multiple phase II and III trials for the treatment of various cancers.6 Due to their cyclic depsipeptide structures with highly modified amino acid residues, didemnins have long been suspected as microbial products assembled by a hybrid nonribosomal peptide synthetase–polyketide synthase (NRPS–PKS) enzymatic pathway.15 In this study, we report that the didemnins are not only the products of the α-proteobacterium Tistrella mobilis, which was also recently reported by Tsukimoto and co-workers,22 but also of Tistrella bauzanensis. More importantly, we show through the complete genome sequencing of T. mobilis and MALDI-imaging MS technique that didemnin B is biosynthesized by an unanticipated rare mechanism involving a post assembly activation of lipoglutamine congeners, didemnins X and Y.
Results and Discussion
Isolation of didemnins from Tistrella species
We isolated the α-proteobacterium T. mobilis KA081020-065 from the Red Sea and measured a very potent cytotoxicity against HeLa cells. Following a bioassay-guided fractionation of the ethyl acetate extract, we isolated two metabolites that based on high-resolution MS and NMR characterization and comparison with authentic standards, were identical to the cyclic depsipeptides didemnin B (Fig. S1) and nordidemnin B (Fig. S2) originally isolated from the Caribbean tunicate Trididemnum solidum.17 Since this result showed that the didemnins are bacterial products, we examined additional Tistrella strains to explore the extent of didemnin chemistry in this poorly characterized genus. To our surprise, all strains tested, including two Pacific Ocean T. mobilis isolates and a T. bauzanensis strain, also produced didemnin B and nordidemnin B at varying levels (Fig. 2). During the preparation of this manuscript, Tsukimoto and co-workers independently reported their isolation from a T. mobilis strain isolated from marine sediments in Japan.22 Based on these preliminary observations, the didemnins appear to be commonly associated with this recently described bacterial genus whose secondary metabolic potential has not yet been reported.23 To further explore the natural product biosynthetic capacity of Tistrella and to deduce the molecular basis governing didemnin assembly, we sequenced the genome of the Red Sea isolate T. mobilis KA081020-065.
Genome characteristics of T. moblis KA081020-065
The complete genome sequence of T. mobilis strain KA081020-065 totaling 6,513,401 bp was established by 454 pyrosequencing to reveal five replicons comprising a 3,919,492 bp circular chromosome and four circular plasmids ranging in size from 83,885 bp (pTM4) to 1,126,962 bp (pTM3) (Fig. 3). Its genome organization with three megaplasmids greater than 600 kb each is reminiscent of several other α-proteobacteria members such as the rice plant endophyte Azospirillum sp. B510 that harbors six plasmids, five of which in excess of 500 kb.24 General features of the T. mobilis genome, which has a high average G+C content of 68%, are summarized in Table S1.
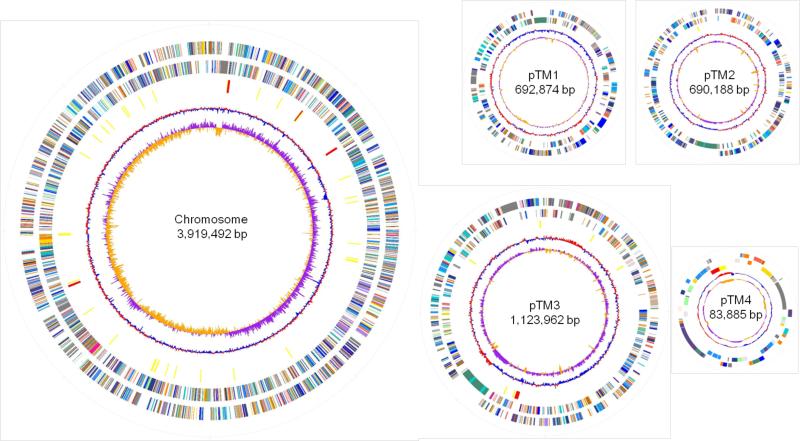
Genome maps of T. mobilis KA081020-065. From the outside inward, the first and second circles show predicted coding regions transcribed on the forward and reverse strand, respectively, colored by functional categories according to COG classification. The third circle shows RNAs (yellow for tRNA and red for rRNA). The fourth and fifth circles show G+C content and GC skew (G-C)/(G+C) calculated using a 5 kb window (purple for values >1, orange for values <1), respectively.
Based on the chemical structure of the didemnin depsipeptides that contains the two ketide-extended amino acid residues isostatine (Ist) and α-(α-hydroxyisovaleryl)-propionic acid (Hip),17 we hypothesized their biosynthesis by a modular hybrid nonribosomal peptide synthetase-polyketide synthase (NRPS-PKS) pathway reminiscent of nostopeptolide biosynthesis.25 Bioinformatic analysis of the Tistrella genome revealed just one large NRPS-PKS biosynthetic gene cluster (did) located on pTM3 (TMO_c0602–c0611) that was consistent with the proposed biosynthesis of the didemnin family of cyclic depsipeptides. We detected only one other multimodular NRPS-based gene cluster in the genome, a 34,665 bp locus on pTM2 (TMO_b0323–b0325), which rather encodes a putative eight-residue peptide product inconsistent with didemnin assembly (Fig. S3).
Bioinformatic analysis of the didemnin biosynthetic gene cluster reveals an unexpected post-assembly processing mechanism
In silico analysis of the putative didemnin biosynthetic gene cluster (did) revealed eight NRPS and two PKS-encoding genes spanning from didA to didJ (Fig. S4), comprising 13 modules in total (Fig. 4). Based on the enzymatic logic of thiotemplate-mediated assembly line biosynthesis, the projected product of the DidB–J megasynthetase perfectly correlates with the co-linear synthesis of didemnin B. The DidB adenylation (A) domain A3 is predicted to load pyruvate and reduce it in cis via the adjacent ketoreductase (KR) domain to give lactate bound to the DidB thiolation (T) domain in a reaction reminiscent to that proposed in valinomycin assembly.26 Further processing by the monomodular DidC and the trimodular DidD NRPSs are next proposed to assemble the Pro-DMeLeu-Thr-Ile tetrapeptide fragment. The N-methylated leucine residue is the lone d-amino acid in the didemnin B molecule, which correlates nicely with the epimerase (E) and methyltransferase (MT) domains that reside in the Leu-specific module 5 of DidD. Malonate extension of the DidD product by the PKS DidE, which lacks an integrated acyltransferase (AT) domain reminiscent of trans-AT PKSs,27 yields the ketide-extended β-hydroxy γ-amino acid residue Ist. Analysis of the genes bordering didA–J and elsewhere in the T. mobilis genome, however, did not reveal a discrete AT from trans-AT systems, suggesting that the didemnin polyketide synthase may co-opt the fatty acid synthase AT FabD as reported recently for the biosynthesis of FK228.28
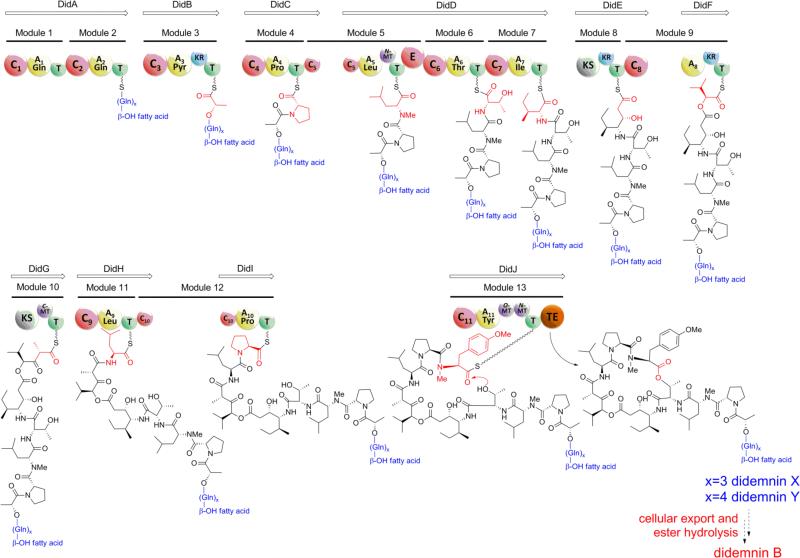
Proposed biosynthetic pathway of didemnins and domain organization of the biosynthetic did gene cluster in T. mobilis KA081020-065. Domain notation: C, condensation; A, adenylation (substrate included); E, epimerase; KR, ketoreductase; KS, b-ketoacyl synthase; MT, methyltransferase; T, thiolation; TE, thioesterase.
Subsequent activation of 2-oxoisovaleric acid by the DidF A domain, whose sequence is similar to the N-terminal HetE A domain (43% identity at amino acid level) that is also responsible for its selection in hectochlorin biosynthesis,29 sets up the incorporation of the α-hydroxy acid 2-hydroxyisovaleric acid and a second round of malonate extension by the DidG PKS. The DidG MT domain putatively incorporates the α-methyl group in the Hip residue. Further chain elongation of the DidG product by the sequential addition of the residues Leu, Pro, and Me2Tyr via the monomodular DidH–J NRPSs completes the assembly of the linear didemnin B molecule. The C-terminal thioesterase (TE) domain of DidJ is hypothesized to offload didemnin B as the cyclic depsipeptide product. In the case of co-produced nordidemnin B, the DidD A7 domain is anticipated to exhibit substrate flexibility toward aliphatic residues to also activate and incorporate Val. [Hysp]didemnin B30 is an additionally characterized didemnin B derivative from T. mobilis extract (Fig. S5) which further indicates substrate promiscuity in DidF domain A9 for α-hydroxy acids 2-hydroxyisovaleric acid (didemnin B) and 2-hydroxy-3-methylvaleric acid ([Hysp]didemnin B. While the biosynthetic model described above is clearly consistent with the observed didemnin B and nordidemnin B chemistry of T. mobilis, this proposal ignores the DidA bimodular NRPS that should function as the priming synthetase prior to DidB catalysis. Inspection of both DidA A domains revealed their preference for Gln. Furthermore, sequence analysis of the DidA N-terminal condensation (C) domain suggested its enzymatic role in the fatty acylation of the first amino acid residue to yield a lipopeptide product (Fig. 4) consistent with other important nonribosomal peptides such as surfactin.31 Among the natural didemnin congeners previously identified from the tunicate T. solidum, only didemnins X and Y have both Gln residues and a lipid component (Fig. 1).32 Interestingly, although these two didemnin derivatives contain Gln residues, they each have more than two residues as predicted by the bimodular DidA if each module were to function just once in peptide elongation. We thus explored the possibility that the immediate product of the didemnin biosynthetic pathway is not (nor)didemnin B, but rather an acylglutamine ester such as (nor)didemnin X or Y in which the ester side chain facilitates the cellular export of the cyclic depsipeptide from the Gram-negative bacterium for extracellular unmasking of the didemnin B byproduct.
Conversion of didemnins X and Y to didemnin B captured by imaging mass spectrometry
Repeated attempts were unsuccessful to isolate additional didemnin congeners by altering the culture medium in order to evaluate the activation hypothesis. We thus turned to MALDI-TOF MS to analyze the bacterial culture extracts, whereupon we successfully detected low abundance masses consistent with didemnins X and Y in addition to two novel derivatives, nordidemnins X and Y (Fig. S6). We confirmed their structures by MSn analysis and high resolution Fourier-transform ion cyclotron resonance MS as reported in the Supporting Information (Fig. S7). The production of didemnins X and Y not only suggests that they are the fully elaborated biosynthetic product of the didemnin synthetase but also indicates that the DidA bimodular NRPS functions iteratively to install the three to four Gln residues in didemnins X and Y, respectively (Fig. 4).
In order to explore the biosynthetic connection between these molecules, we next used MALDI-imaging MS33,34 to study the temporal and spatial distribution of the didemnin compounds. We interrogated T. mobilis colonies over a time course ranging from 6 hours to 6 days (Fig. 5), which strikingly revealed that didemnins X and Y are produced and excreted from the colony during the early growth phase of the bacterium peaking at 24 hours. Concomitant with their disappearance by day 4 was the emergence of didemnin B as the primary didemnin metabolite that steadily increased over time. This time-lag difference between didemnins X and Y and didemnin B was similarly observed in the nordidemnin series (Fig. 5) and is consistent with the proposed model in which the lipoglutamine side chain facilitates the cellular export of the mature didemnin molecule for extracellular processing.
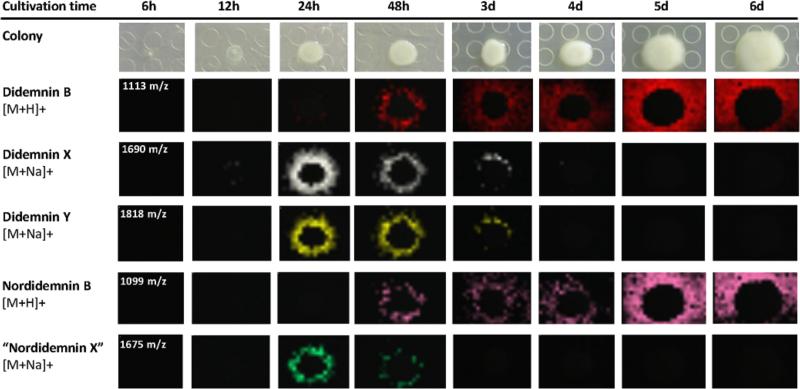
Time-course production of didemnins by T. mobilis KA081020-065 detected by MALDI-imaging mass spectrometry.
We next set out to determine whether the maturation of didemnins X and Y involved a secreted factor. To this end, we performed a hydrolysis assay of purified didemnin precursors and filtered growth media cultured with and without the bacterium. The assay clearly showed that didemnin X/Y turnover to didemnin B was dependent on the addition of a protein extract from a 1 day T. mobilis culture supernatant (Fig. S8). While studies are ongoing to identify the secreted hydrolytic factor involved in the peptide activation mechanism, sequence analysis of the open reading frames adjacent to the didA–J operon revealed a number of genes encoding membrane-associated transport proteins (orf3, orf6) and hydrolytic enzymes (orf2, orf14) (Fig. S4), further supporting the export-hydrolysis model. Recently an activation mechanism was established in the nonribosomal peptide synthesis of the antibiotic xenocoumacin from the γ-proteobacterium Xenorhabdus nematophila in which a precursor molecule is processed by a membrane-bound and d-Asn-specific peptidase.35 Although the characterized pathway in xenocoumacin processing parallels the proposed pathway for didemnin maturation, the did cluster does not harbor a homolog of the xenocoumacin ABC transporter-transpeptidase XcnG. In addition, the maturation of xenocoumacin takes place in the periplasmic space rather than the extracellular space for didemnins, which may suggest an alternative mechanism in T. mobilis.
Conclusion
The discovery of the bacterial synthesis of the didemnins raises the question about their origin in the Caribbean tunicate T. solidum where they were first characterized over 30 years ago by Rinehart and coworkers.17 Based on the findings reported here, we suspect that the tunicate-derived didemnins are similarly bacterial in origin. A recent metagenomic profile of the tropical Pacific tunicate Lissoclinum patella revealed that the distinctive patellamide ribosomal peptides and the antitumor polyketide patellazole are biosynthetically linked to different bacteria within the tunicate microbiome.36 Similarly, the approved drug Yondelis (ET-743) from the tropical tunicate Ecteinascidia turbinata was recently shown to be bacterial in origin.12 With the T. mobilis genomic information now in hand, molecular methods could be used to examine didemnin-associated tunicates for the presence of Tistrella bacteria or other microbes that carry the did genes. The finding that the didemnin biosynthetic gene cluster resides on a plasmid is also intriguing since other bacteria may acquire the metabolic pathway by horizontal gene transfer. The did-containing megaplasmid pTM3 contains several essential elements required for conjugation, including a relaxase (TMO_c0026) needed for DNA transfer. The bacterial chromosome additionally contains a trb operon which encodes the components of a bacterial mating apparatus, (TMO_2187–2198), another important component in Gram-negative bacterial conjugation.37
With a bacterial production of the didemnins now in place, the supply of these structurally complex and potent anticancer agents may no longer be limiting. While clinical trials with didemnin B have been suspended, phase II trials with aplidine (dehydrodidemnin B; plitidepsin) from the Mediterranean tunicate Aplidium albicans are actively underway.38 Presently, multi-step total synthesis is employed to produce aplidine for the clinic due to its limited natural supply.6, 39 Biotechnology now may offer an alternative solution. The T. mobilis DidB tetradomain gene product is putatively responsible for the selection and attachment of pyruvate, its ketoreduction to lactate, and its ensuing condensation with the DidA fatty acylglutamine residue (Fig. 4). Gene inactivation of the didB KR domain would maintain the oxidation state of the pyruvate unit and thereby prevent the esterification reaction, effectively switching the didemnin B biosynthetic pathway to directly produce aplidine. While seemingly straightforward once a genetics system is established in Tristella, production levels may be significantly impacted since the activation and export mechanism that governs didemnin B synthesis from the lipopeptides didemnins X and Y may no longer be effective in such a mutant for aplidine. We are actively pursuing answers to the detailed mechanism of didemnin B biosynthesis and whether we can reengineer T. mobilis to directly produce aplidine. A provisional patent has also been filed on the didemnin biosynthetic gene cluster (U.S. provisional application number 61/537,416).
Experimental Section
Strain, Fermentation and Isolation of didemnin compounds
Tistrella mobilis KA081020-065 was isolated from seawater collected from the Red Sea during a 2009 research cruise. Its crude extract showed remarkable cytotoxicity on HeLa cells in a bioactive compound screening of Red Sea bacteria. T. mobilis KA081020-065 was grown in GYP medium [10 g glucose, 4 g yeast extract, 2 g peptone, and 17 g sea salts per l deionized water] using 50-liter stirred fermenters at 25 °C. After 72 hours, ethyl acetate was added to the culture to extract the metabolites. The crude extract was fractionated by reversed phase C18 liquid chromatography and eluted with increasing amounts of methanol in water. The active fraction was further purified by semi-preparative reversed phase (RP) HPLC with 63% MeCN in water to give didemnin B and nordidemnin B at 0.2 mg l-1 and 0.1 mg l-1, respectively. For MSn and FTMS analysis, crude extracts were separated by RP-HPLC (10-100% MeCN in 0.1% trifluoroacetic acid (TFA), 40 min gradient). For hydrolysis assay, didemnin X and Y were purified from crude extract by Sephadex LH20 (GE Healthcare) gel filtration with MeOH as a mobile phase and RP-HPLC (25-75% MeCN in 0.1% TFA, 60 min gradient).
MS sample preparation and MS analysis of didemnins
For MALDI-TOF MS analysis, extract or peptide samples were dissolved in MeOH, mixed 1:1 with saturated Universal MALDI Matrix and spotted on a Bruker MSP96 anchor plate. MALDI-TOF MS analysis was performed on a Microflex Bruker Daltonics mass spectrometer outfitted with Compass 1.3 software suite. For MSn and FTMS analysis, HPLC-purified samples were redissolved in 50 μl MeOH/water/formic acid (50:49:1) and injected by a nanomate-electrospray ionization robot (Advion) for consecutive electrospray into the MS inlet of a LTQ 6.4T Fourier transform ion cyclotron resonance (FT-ICR) mass spectrometer (Thermo Finnigan). MS and MSn data were acquired in the positive ion mode. FTMS data were acquired in 400–2,000 m/z scans. Selected peptide mass signals were manually isolated and fragmented by CID. MSn data were collected either in ion trap or FT detection mode. All data were analyzed using QualBrowser, which is part of the Xcalibur LTQ-FT software package (Thermo Fisher).
Genome sequencing, annotation, and analysis
We used a massively parallel pyrosequencing technology (Roche 454 GS FLX)40 to sequence the T. mobilis KA081020-065 genome. We obtained 315,496 reads with an average length of 334 bp, which provided a 16.2-fold coverage of the 6.4 Mb genome. From these reads, we assembled 112 contigs (>500 bp) using the Newbler software of the 454 suite package. To enhance sequence quality and to construct scaffolds, we sequenced a paired-end Illumina library of an additional 2,625,640 bp with average length of 115 bp, which were then mapped to the genome sequence. All contig relationships within scaffolds were then validated by PCR, while the relationships among scaffolds were determined by multiplex PCR.41 Gaps were filled by sequencing PCR products. The final sequence assembly was carried out using the phred/phrap/consed package (http://www.genome.washington.edu) in which we resequenced the low sequence quality regions. The final error rate of the genome sequence was 0.28 per 100,000 bases. The sequences of the five replicons were deposited in the GenBank database as accession numbers CP003236–CP003240.
Putative protein-coding sequences (CDS) were determined by combining the prediction results of the Glimmer 3.0242 and Z-Curve programs.43 Functional annotations of CDS were performed by searching the KEGG and the NCBI non-redundant protein databases. tRNA genes were predicted with tRNAScan-SE (v1.23).44 Protein domain predictions and COG assignments45 were performed by RPS-BLAST using the NCBI CDD library.46
In silico analysis of the didemnin biosynthetic gene cluster and other NRPSs in T. mobilis KA081020-065
The putative roles of the proteins in the didemnin gene cluster were assigned using protein–protein BLAST and Pfam analyses. NRPS A domain specificities were predicted using the online program NRPSpredictor2 and antiSMASH.47–49
Time-course study of didemnin production in T. mobilis by MALDI-imaging MS
One μl of fresh overnight T. mobilis culture was spotted onto GYP agar plates and allowed to grow for 6 hours to 6 days. Colonies with different growing times were transferred to Bruker MSP 96 anchor plates and analyzed concurrently using the IMS technique as previously described.33,50
Didemnin precursor hydrolysis assay
50 μg HPLC-purified didemnin X and Y were each dissolved in 5 μl water and mixed with (a) 45 μl sterile GYP medium (negative control) or (b) 45 μl protein filtrate (10 kDa cutoff, washed 2x with GYP medium) of 1 ml of a 1 d T. mobilis KA081020-065 liquid culture (28 °C) and incubated for 24 h at 25 °C. Hydrolysis samples were taken and spotted on a MALDI-TOF MS plate for subsequent MS analysis at time points 5 min, 1 h, 12 h and 24 h.
Acknowledgment
We thank Z. Shao for providing the Pacific Ocean Tistrella strains and N. Ziemert, J. Yang and P. Lai for assistance with data analysis. Financial support was provided by King Abdullah University of Science and Technology (SA-C0040/UK-C0016 to P.Y.Q.), China Ocean Mineral Resources Research and Development Association (DY125-15-T-02 to P.Y.Q.), and the National Institutes of Health (GM085770 to B.S.M., CA044848 to W.F., GM086283 and S10RR029121 to P.C.D.).
Footnotes
Author Contribution Y.X and R.D.K contributed the same to the work.
Supporting Information
Copies of the 1H NMR, annotation of MSn of the didemnins and hydrolysis experiment of didemnin X/Y are included. This material is available free of charge via the Internet at http://pubs.acs.org.
References
Full text links
Read article at publisher's site: https://doi.org/10.1021/ja301735a
Read article for free, from open access legal sources, via Unpaywall:
https://europepmc.org/articles/pmc3401512?pdf=render
Citations & impact
Impact metrics
Citations of article over time
Smart citations by scite.ai
Explore citation contexts and check if this article has been
supported or disputed.
https://scite.ai/reports/10.1021/ja301735a
Article citations
Plant peptides - redefining an area of ribosomally synthesized and post-translationally modified peptides.
Nat Prod Rep, 41(7):1020-1059, 17 Jul 2024
Cited by: 1 article | PMID: 38411572 | PMCID: PMC11253845
Review Free full text in Europe PMC
Small molecule in situ resin capture provides a compound first approach to natural product discovery.
Nat Commun, 15(1):5230, 19 Jun 2024
Cited by: 1 article | PMID: 38898025 | PMCID: PMC11187115
Metallo-protease Peptidase M84 from Bacillusaltitudinis induces ROS-dependent apoptosis in ovarian cancer cells by targeting PAR-1.
iScience, 27(6):109828, 26 Apr 2024
Cited by: 0 articles | PMID: 38799586 | PMCID: PMC11126781
Structure and Biosynthesis of Hectoramide B, a Linear Depsipeptide from Marine Cyanobacterium Moorena producens JHB Discovered via Coculture with Candida albicans.
ACS Chem Biol, 19(3):619-628, 08 Feb 2024
Cited by: 0 articles | PMID: 38330248 | PMCID: PMC10949194
Metabolic insights from mass spectrometry imaging of biofilms: A perspective from model microorganisms.
Methods, 224:21-34, 29 Jan 2024
Cited by: 0 articles | PMID: 38295894 | PMCID: PMC11149699
Review Free full text in Europe PMC
Go to all (84) article citations
Data
Data behind the article
This data has been text mined from the article, or deposited into data resources.
BioStudies: supplemental material and supporting data
Nucleotide Sequences (3)
- (4 citations) ENA - KA081020
- (1 citation) ENA - CP003236
- (1 citation) ENA - CP003240
Similar Articles
To arrive at the top five similar articles we use a word-weighted algorithm to compare words from the Title and Abstract of each citation.
Exploring the Biosynthetic Potential of <i>Tistrella</i> Species for Producing Didemnin Antitumor Agents.
ACS Chem Biol, 19(10):2176-2185, 23 Sep 2024
Cited by: 0 articles | PMID: 39312286
A Survey of Didemnin Depsipeptide Production in Tistrella.
Mar Drugs, 21(2):56, 17 Jan 2023
Cited by: 0 articles | PMID: 36827097 | PMCID: PMC9964501
Bacterial production of the tunicate-derived antitumor cyclic depsipeptide didemnin B.
J Nat Prod, 74(11):2329-2331, 28 Oct 2011
Cited by: 55 articles | PMID: 22035372
Natural products as probes of cell biology: 20 years of didemnin research.
Med Res Rev, 22(2):102-145, 01 Mar 2002
Cited by: 47 articles | PMID: 11857636
Review
Funding
Funders who supported this work.
NCI NIH HHS (3)
Grant ID: CA044848
Grant ID: R37 CA044848
Grant ID: R01 CA044848
NCRR NIH HHS (2)
Grant ID: S10RR029121
Grant ID: S10 RR029121
NIGMS NIH HHS (5)
Grant ID: R01 GM085770-04
Grant ID: GM086283
Grant ID: R01 GM085770
Grant ID: R01 GM086283
Grant ID: GM085770