Abstract
Free full text

Poliovirus Receptor (CD155) Regulates a Step in Transendothelial Migration between PECAM and CD99
Abstract
The movement of leukocytes across endothelium [referred to as diapedesis or transendothelial migration (TEM)] is a critical step in the inflammatory process. Recently, it was demonstrated that treatment of endothelial cells and monocytes with antibodies against poliovirus receptor (PVR; CD155) and DNAX-associated molecule-1 (DNAM-1; CD226) arrested monocytes over endothelial junctions and prevented TEM, suggesting that these molecules are involved in diapedesis. However, nothing was known about the mechanism by which PVR and DNAM-1 work in TEM. Herein, we show that, similar to endothelial PECAM interacting with leukocyte PECAM, activation of endothelial PVR with anti-PVR antibodies or interaction with its ligand, DNAM-1, results in recruitment of the tyrosine phosphatase Shp-2, and this process is dependent on Src kinases. Furthermore, differential and sequential treatment with blocking antibodies directed against PVR, DNAM-1, PECAM, and CD99 showed that endothelial PVR and monocyte DNAM-1 interact at and regulate a step between those regulated by PECAM and CD99. Further studies demonstrate that PVR resides in the recently identified lateral border recycling compartment, similar to PECAM and CD99. These findings suggest that the localization of adhesion/signaling molecules to the lateral border recycling compartment and the recruitment of Shp-2 may be common mechanisms for the regulation of TEM by endothelial cells.
Transendothelial migration (TEM; alias diapedesis) is the putative committed step of the multistep process by which leukocytes move out of the bloodstream, across the endothelial cell layer, and into target tissue to combat infection and heal injuries.1,2 This makes it an attractive therapeutic target for pathological conditions resulting from dysregulated inflammation, such as autoimmune arthritis, atherosclerosis, and multiple sclerosis. TEM has been regulated in a quantitatively significant way by at least two proteins, PECAM and CD99, in several in vitro and in vivo systems.3–5 PECAM and CD99 are expressed on both leukocytes and endothelial cells, and have been shown to operate via homophilic interaction (ie, leukocyte PECAM with endothelial PECAM and leukocyte CD99 with endothelial CD99) in the TEM of neutrophils and monocytes in response to multiple stimuli.1 Disruption of PECAM or CD99 homophilic interactions using antibodies against the appropriate regions of their extracellular domains inhibits TEM both in vitro and in vivo.4–6
Recently, Reymond et al7 demonstrated a role for the molecules poliovirus receptor (PVR) and DNAX-associated molecule-1 (DNAM-1) in TEM, showing that monoclonal antibodies against either molecule could block the transmigration of isolated monocytes across a monolayer of cultured human umbilical vein endothelial cells (HUVECs). More recently, Manes and Pober8 used antibodies against PVR and DNAM-1 to block TEM of effector memory T cells. However, these studies provided no characterization of the mechanism of action or the relationship of these molecules to other components of the TEM machinery. PVR (CD155) is a transmembrane immunoglobulin superfamily member expressed on neurons, epithelium, endothelium, and monocytes/macrophages, which localizes to sites of cell-cell and cell-matrix adhesion.7,9–14 PVR has primarily been investigated for its role as the key receptor for poliovirus entry into cells, but has also been reported to be involved in cell adhesion and motility and cell-mediated cytotoxicity.15–20 DNAM-1 is a transmembrane immunoglobulin superfamily member expressed on natural killer cells, T cells, some B cells, platelets, monocytes, and, possibly, activated HUVECs.21–25 It has been associated with natural killer and CD8 T-cell cytotoxicity,21 T-cell development,22,25 platelet activation,22,26 and, more recently, monocyte TEM.7,8
In this study, we sought to characterize the exact role that PVR and DNAM-1 play in TEM and, perhaps, more important, the mechanisms through which they act. Herein, we show that, in a manner analogous to PECAM activation, the engagement of PVR on the endothelial cell leads to its phosphorylation by an Src-dependent mechanism, which leads to localized recruitment and specific binding of Shp-2. We also sought to investigate the timing of the PVR and DNAM-1 interaction during TEM in relation to the roles played by PECAM and CD99. Having previously described that PECAM and CD99 function sequentially in TEM,5 we describe herein that endothelial cell PVR and leukocyte DNAM-1 interact with each other at a step between those regulated by PECAM and CD99. We have also previously shown that PECAM and CD99 localize to the lateral border recycling compartment (LBRC) and that recycling of this compartment is essential to the proper functioning of PECAM and CD99 during TEM.3,27 Herein, we describe that PVR also localizes to the LBRC. Furthermore, only the subset of PVR molecules localized to the LBRC is relevant to TEM, analogous to data previously published for PECAM and CD99. Our findings demonstrate that the process of TEM is regulated at the molecular level by at least three pairs of molecular interactions functioning at three sequential steps, yet all involving the membrane platform of the LBRC.
Materials and Methods
All procedures involving human subjects and human materials were approved by the Institutional Review Board of Northwestern University Feinberg School of Medicine (Chicago, IL).
Antibodies and Proteins
This study used mouse IgG2a anti-human PECAM clone hec7,28 mouse IgG1 anti-human CD99 clone hec2,5 and mouse IgG2a anti-human VE-cadherin clone hec1,29 all produced in the laboratory via hybridoma methods. Rabbit polyclonal anti-human PECAM(177) was produced as previously described.4 Anti-PVR antibody (clone D171) was purchased from Neomarkers/LabVision (Fremont, CA). Anti–DNAM-1 antibody (clone DX11) was purchased from BD Biosciences (San Jose, CA). Anti–Shp-2 antibody (rabbit polyclonal, sc280) was purchased from Santa Cruz Biotechnology (Santa Cruz, CA). Anti-phosphotyrosine (4G10-platinum) and streptavidin–horseradish peroxidase (HRP) were purchased from Millipore (Billerica, MA). Non-specific mouse IgG and goat–anti-rabbit Dylight-549 were purchased from Jackson ImmunoResearch Laboratories (West Grove, PA). Anti-human PECAM clone hec7 conjugated to Alexa Fluor 488 was prepared using a kit purchased from Invitrogen (Carlsbad, CA). DNAM-1-Fcmut plasmid DNA was a generous gift from Drs. Christina Bottino and Claudia Cantoni (Istituto Giannina Gaslini, Genova, Italy)7; expressed protein was purified according to standard techniques previously described.30
Isolation and Culture of Endothelial Cells
HUVECs were isolated from human umbilical cords, as previously described.28,31 At their second passage, isolated HUVECs were cultured on either three-dimensional type I collagen matrices (PureCol; Inamed Biomaterials, Fremont, CA) or coverslip dishes (Mattek, Ashland, MA) and allowed to reach confluence. Cells grown on collagen gels were allowed to mature for 2 to 3 days after this point to ensure the formation of high-quality basement membranes. Primary human dermal microvascular endothelial cells (HMVECs) were purchased from Lonza Group Ltd (Basel, Switzerland) and cultured in the recommended media, also purchased from Lonza Group Ltd. PECAM, PVR, and CD99 were all expressed at intercellular junctions in HMVECs in a manner identical to HUVECs (data not shown). HMVECs were used at the second or third passage, and TEM assays involving HMVECs were performed in the same way as those described for HUVECs later.
Isolation of Primary Human Leukocytes
Leukocytes were harvested as previously described.31,32 Blood drawn from healthy volunteers was immediately mixed with 10 mmol/L final concentration of EDTA and an equal volume of HBSS (Mediatech Inc., Herndon, VA) and then layered over Ficoll-Paque density gradient medium (GE Healthcare Biosciences AB, Uppsala, Sweden) for the isolation of peripheral blood mononuclear cells (PBMCs). After centrifugation at 780 × g for 20 minutes, the upper plasma layer was collected into fresh tubes, and the PBMCs at the interface were harvested with a flame-polished glass pipette into a separate tube. The cells were diluted in HBSS, and both cells and platelet-poor plasma were centrifuged for 10 minutes at 360 × g. The cell pellet was resuspended in the spun plasma and centrifuged for 5 minutes at 235 × g. The resulting pellet was washed two to three times with HBSS via resuspension and centrifugation for 5 minutes at 235 × g. The final pellet was resuspended in M199 (Invitrogen) containing 0.1% human serum albumin (HSA; Grifols Biologicals Inc., Los Angeles, CA).
Flow Cytometry
Confluent HUVECs were removed from the tissue culture plate via light trypsinization. Leukocytes were isolated as previously described. Pilot studies established that these procedures did not affect the detection of the antigens examined herein. Cells in suspension were incubated with the appropriate primary antibody (including no primary and irrelevant control isotype-matched antibodies) at 10 μg/mL in PBS for 1 hour on ice. Cells were washed repeatedly with PBS, and then incubated with labeled secondary antibody (Alexa Fluor 488 goat anti-mouse; Molecular Probes, Invitrogen) at 1 μg/mL in PBS for 1 hour on ice. Cells were washed again, and then assayed on a BD FACSCalibur (BD Biosciences, San Jose, CA) instrument using BD CellQuest Pro Software version 5.1 (BD Biosciences).
Immunofluorescence Microscopy
Confluent HUVECs were fixed in 2% paraformaldehyde for 20 minutes, washed three times with PBS, quenched with 75 mmol/L glycine for 15 minutes, and washed three more times with PBS. Blocking buffer (PBS + 5% ovalbumin; Sigma-Aldrich, St. Louis, MO) was added for 30 minutes at room temperature. Cells were then incubated with primary antibody at 10 μg/mL in blocking buffer for 1 hour at room temperature, washed extensively with PBS, and then incubated with secondary antibody (Alexa Fluor 488 goat anti-mouse) at 4 μg/mL in blocking buffer for 1 hour at room temperature, protected from light. For the visualization of Shp-2, cells were permeabilized for 4 minutes with 0.1% Triton X-100 (Sigma-Aldrich) before blocking. To detect PECAM, some samples were then incubated with Alexa Fluor 488–conjugated anti-PECAM antibodies (clone hec7) for 45 minutes and washed before visualization. Samples that were treated with polystyrene beads were visualized using an Ultraview VoX imaging system (Ultraview, Waltham, MA) equipped with a Yokogawa CSU-1 spinning disk (Yokogawa Electric Company, Tokyo, Japan). Images were acquired with a 488-nm wavelength laser through a 20× water immersion objective using Volocity software version 6.2.1 (Perkin Elmer, Waltham, MA). Monolayers and the samples with glass beads were imaged using a restoration workstation (Delta Vision 3D; Applied Precision, Issaquah, WA) equipped with an inverted microscope (model IX70; Olympus Tokyo, Japan) using a 60× oil objective.
Electron Microscopy
Antibodies recognizing PVR, PECAM, and VE-cadherin (clones D171, hec7, and hec1, respectively) were labeled with HRP using an EZ-Link Plus activated peroxidase kit (Thermo Fisher Scientific, Waltham, MA). Analysis of the labeling efficiency was determined using SDS-PAGE and showed that the ratio of HRPs per antibody was approximately 1:1. Antibodies were incubated with HUVECs grown on 13-mm-diameter cell culture–treated Thermanox coverslips (Thermo Fisher Scientific) at either 4°C or 37°C. After incubation for 30 minutes, the monolayers were chilled, washed three times with ice-cold PBS, and fixed for 10 minutes with 4% glutaraldehyde in 100 mmol/L cacodylate buffer, pH 7.4 (Electron Microscopy Sciences, Hatfield, PA). Monolayers were then washed three times with PBS and incubated with 10 mg/mL diaminobenzidine (Sigma-Aldrich) and 0.03% hydrogen peroxide in PBS for 20 minutes. Monolayers were then washed three times and fixed again as before. Samples were embedded according to standard microscopic methods. En face sections (70 nm thick) were examined using an Tecnai Spirit electron microscope (FEI Company, Hillsboro, OR).
Glass and Polystyrene Microsphere (Bead) Coating
All incubation steps for the bead coating were performed using an end-over-end rotator at room temperature, unless otherwise indicated. Glass beads (Polysciences, Warrington, PA), 3 to 10 μm, 2 mg per sample, were washed three times with 96% ethanol and silanized with 5% 3-aminopropyltriethoxysilane (Sigma-Aldrich) in 96% ethanol for 1 hour. The beads were then washed with PBS and resuspended in 3% glutaraldehyde in PBS and rotated for 2 hours. The beads were washed again and fractionated into separate tubes, and resuspended in 250 μL of 200 μg/mL of either bovine serum albumin (BSA) or antibodies against PVR (clone D171), PECAM (clone hec7), or non-specific mouse IgG and incubated for 1.5 hours. To help visualize the beads, this conjugation reaction was sometimes supplemented with 1 μg/mL of a fluorescently labeled irrelevant antibody. The beads were then washed and incubated with 10 mg/mL of BSA in PBS overnight at 4°C.
Amino-functionalized 3 μm polystyrene beads (Polysciences) were washed with PBS and resuspended in 1 mL of 8% glutaraldehyde in PBS for 2 hours. Beads were washed again, fractionated into separate tubes, and incubated with 300 μL of 300 μg/mL of either BSA or the indicated antibody for 2 hours. After washing, the beads were blocked with 10 mg/mL BSA for 1 hour. Conjugated beads (both glass and polystyrene) were stored at 4°C in blocking solution for up to 1 week without noticeable loss of efficacy. Beads were always washed with PBS immediately before use.
For the bead experiments, HUVEC monolayers were grown on fibronectin-coated coverslip dishes to confluence. The conjugated beads were diluted into preconditioned HUVEC media and allowed to settle and bind for either 10 minutes (glass beads) or 20 minutes (polystyrene beads). Monolayers were then washed with PBS and fixed with 4% paraformaldehyde, and the indicated proteins were visualized using immunofluorescence. All images within each experiment were captured under identical settings and processed identically using ImageJ software version 1.45s (NIH, Bethesda, MD) to preserve any differences in relative intensities.
Immunoprecipitation and Western Blot Analyses
HUVECs were grown to confluence on fibronectin-coated, 6-cm dishes. Monolayers were pretreated for 10 minutes with 5 μmol/L PP2 [4-amino-5-(4-chlorophenyl)-7-(t-butyl)pyrazolo(3,4-d)pyramidine] or inactive analogue PP3 [4-amino-7-phenylpyrazolo(3,4-d)pyramidine], both from EMD Chemicals (Darmstadt, Germany), diluted in conditioned media from a 5 mmol/L stock in dimethyl sulfoxide. At the start of the assay, media were replaced with conditioned media containing either PP2 or PP3 and 20 μg/mL of the indicated antibody. After 20 minutes of incubation at 37°C with 5% CO2, the media were removed, and the monolayers were chilled and washed with ice-cold PBS. To visualize PVR using Western blot analysis, the monolayers were then treated with 1 mg/mL Sulfo-NHS-LC-biotin (Thermo Fisher Scientific) in PBS on ice for 15 minutes, followed by quenching for 15 minutes with 50 mmol/L glycine in PBS. After washing with PBS, the cells were lysed in PBS containing 1% NP-40, 1 mmol/L orthovanadate, 1× protease inhibitor cocktail (P8340; Sigma-Aldrich), and 1 mmol/L phenylmethylsulfonyl fluoride (Sigma-Aldrich) for 10 minutes on ice. The lysates were collected and centrifuged at 18,000 × g at 4°C for 15 minutes in a microfuge. The supernatants were recovered and incubated with Protein A–agarose beads loaded with anti-PVR antibody (D171) for 2 hours at 4°C with end-over-end rotation. The bound beads were washed repeatedly with lysis buffer and resuspended in 2× Laemmli loading buffer with β-mercaptoethanol. The samples were then boiled for 5 minutes, and equivalent amounts were loaded onto a 10% polyacrylamide gel and resolved using SDS-PAGE. Proteins were transferred to PVDF and detected using standard Western blot analysis techniques. Blots were first probed for phosphotyrosine using 4G10-platinum and then stripped and reprobed to detect Shp-2, using rabbit-polyclonal anti–Shp-2, and PVR, using streptavidin-HRP.
TEM Assay
TEM assays were performed as previously described.31 Briefly, PBMCs (4 × 106 per mL) were mixed 1:1 with either medium alone or dilutions of antibodies to a final concentration of 20 μg/mL. These were then plated onto confluent HUVEC monolayers grown on hydrated type I collagen gels and allowed to migrate at 37°C in 5% CO2 for 1 hour. The monolayers were washed twice with 1 mmol/L EDTA (Sigma-Aldrich) in HBSS, twice with PBS supplemented with Ca2+ and Mg2+, and then fixed in 1% glutaraldehyde in 0.1 mol/L sodium cacodylate buffer (Electron Microscopy Sciences). Fixed samples were stained with modified Wright-Giemsa stain (Protocol Hema3; Fisher Diagnostics, Middletown, VA) and mounted onto glass slides for visualization. Imaging was performed with a Zeiss Ultraphot microscope with Nomarski optics and a SPOT Insight Color CCD (Diagnostic Instruments, Inc., Sterling Heights, MI). TEM was analyzed by manually counting at least 100 cells per collagen gel and noting their position relative to the endothelial monolayer (above or below, defining above as any monocyte having most of the cell body on or above the focal plane of the endothelial cell nuclei). For each figure describing TEM, samples were all assayed in the same experiments and were only separated into panels for clarity.
Monocyte-Side Function Studies
PBMCs were mixed with reagents, as previously described for the TEM assay. Before adding the PBMCs to the HUVECs, the PBMCs were incubated on ice for 15 minutes with the indicated antibody. The cells were then centrifuged at 235 × g for 5 minutes and washed three times with M199/0.1% HSA to remove unbound antibody. PBMCs were then resuspended in M199/0.1% HSA at 2 × 106 PBMCs/mL and added to the HUVECs for the TEM assay, as previously described, except that leukocytes were allowed to migrate for 30 minutes instead of 1 hour.
Endothelial-Side Function Studies
HUVECs were treated with 20 μg/mL of the indicated antibody in M199/0.1% HSA and incubated at either 4°C or 37°C for 1 hour. Monolayers were then washed extensively with M199/0.1% HSA before the addition of PBMCs. TEM was then allowed to proceed as previously described, except that leukocytes were allowed to migrate for 30 minutes instead of 1 hour.
Sequential Blockade of TEM
This procedure was performed as previously described.5 Briefly, the TEM assay was performed exactly as previously described, except that at the end of the 1-hour incubation at 37°C, instead of washing and fixing cells, the plates were chilled for 30 minutes, washed several times with cold PBS, and new 20 μg/mL antibody dilutions in M199/0.1% HSA were added to the samples. Plates were then warmed, and TEM was allowed to proceed for an additional 2 hours (previously shown to be sufficient for recovery from the initial blockade5). TEM was then stopped by fixing the samples, and the migration was quantified as previously described.
Statistical Analysis
All experiments with quantitation were performed independently at least three times with at least three replicates for each sample within each experiment. For the transmigration assays, the values for the replicates were averaged together within each experiment. The average and SD of these averages are shown in the figures. P values for each experimental condition were calculated from the average values using the Student’s t-test for unpaired observations relative to the non-blocking or non-specific antibody controls. All P values noted by an asterisk were < 0.05, and most were < 0.01.
Results
PVR Stimulation Recruits Shp-2
In agreement with published data, we confirm that PVR is expressed on monocytes and endothelial cells, whereas DNAM-1 is expressed on monocytes but not on endothelial cells (Supplemental Figure S1A).7,21 In addition, PVR is localized to endothelial cell junctions in a manner similar to PECAM and CD99 (Supplemental Figure S1B). Both PECAM and PVR contain an immunoreceptor tyrosine–based inhibitory motif (ITIM), a domain that has been reported to be phosphorylated on ligand stimulation by Src family kinases. This phosphorylation leads to the recruitment of protein tyrosine phosphatases and is critical for the signal functions of the proteins that contain the ITIM. Indeed, tyrosine 686 of the ITIM of PECAM has been shown to be phosphorylated and to recruit the phosphatase, Shp-2.33–35 Although the role of phosphorylation of PVR in TEM is presently unknown, the phosphorylation of the ITIM of PVR and the recruitment of Shp-2 has been found to be crucial for the entry of poliovirus into cells.16,17 In light of these findings, we hypothesized that PVR phosphorylation and Shp-2 recruitment could be involved in TEM in a mechanism analogous to PECAM and sought to determine whether ligation of PVR recruits Shp-2 in primary endothelial cells. In HUVECs under resting conditions or on treatment with an irrelevant antibody, most of the Shp-2 is distributed diffusely throughout the cytosol, with only a small fraction found near intercellular junctions (Figure 1). On treatment with either anti-PVR or a DNAM-1-Fc chimera, Shp-2 showed a dramatic redistribution to the junctions (Figure 1).
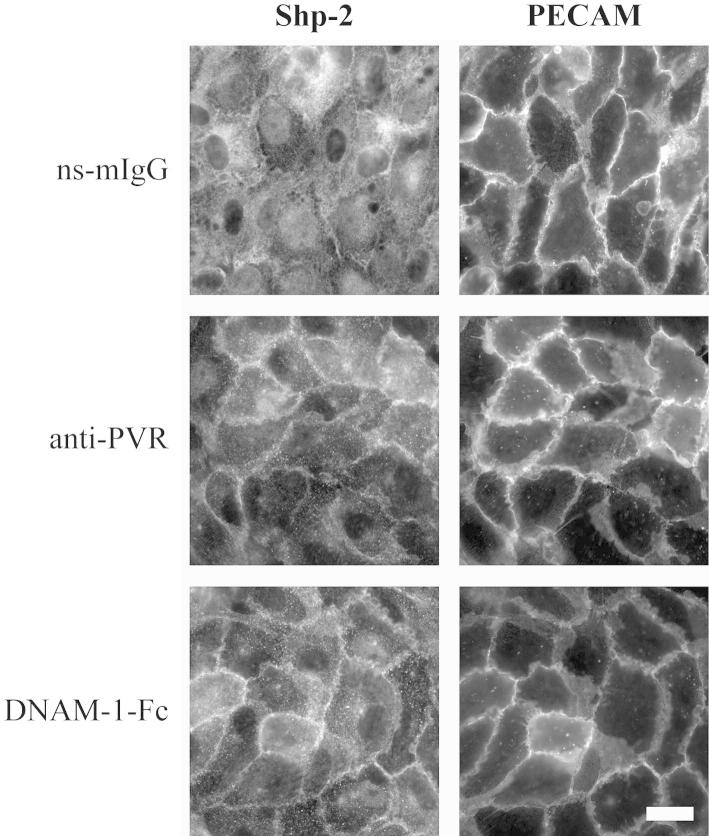
Shp-2 localizes to endothelial cell junctions on PVR stimulation. HUVEC monolayers were treated with 20 μg/mL non-specific mouse IgG (ns-mIgG), antibody against PVR, or DNAM-1-Fc chimera for 20 minutes at 37°C and fixed. PECAM and Shp-2 were visualized using fluorescent antibodies against the indicated protein. Data are representative of four independent experiments. Scale bar = 25 μm.
Shp-2 Recruitment to Coated Beads
Although this redistribution is striking, the stimulation of adhesion molecules that occurs during TEM is not global; instead, it is much more spatially restricted to the area in contact with the leukocyte. Our attempts to examine Shp-2 recruitment to migrating leukocytes were complicated by the presence of Shp-2 in the leukocyte. Also, because Shp-2 is known to be involved in other steps during TEM,33,36 we could not distinguish the PVR-specific recruitment from recruitment mediated by other receptors (data not shown). To investigate the specific Shp-2 recruitment after PVR stimulation, we stimulated a leukocyte using beads coated with appropriate ligands. HUVECs were treated with polystyrene beads that were conjugated with non-specific mouse IgG, DNAM-1-Fc chimera, or antibodies against PVR or PECAM. When HUVECs were incubated with beads coated with non-specific mouse IgG, only a few beads (approximately 10%) showed even a modest increase in the amount of Shp-2 in the adjacent cytoplasm (ie, an increase in fluorescence intensity of approximately 1.5 times that of the neighboring cytoplasm) (Figure 2). The incubation of HUVECs with beads conjugated to ligands known to stimulate PVR led to an increase in both the intensity of Shp-2 in the area surrounding the bead and the frequency of beads with enrichment (approximately 50%). Although several different incubation times were tested, the number of beads with enriched Shp-2 never reached 100%, a finding that likely indicates the variable amount of time required for the beads to settle and the transient nature of the Shp-2/PVR interaction. This enrichment was the result of specific Shp-2 recruitment and not a generic volume effect of increased cytosol around the bead because no enrichment of the housekeeping protein, glyceraldehyde-3-phosphate dehydrogenase, was observed around the bead (data not shown). Also, only minimal and rare enrichment was observed with beads coated with antibodies against major histocompatibility complex class I (data not shown), suggesting that the recruitment is not simply to a bead interacting with the endothelial cells. Stimulation of PECAM has also been shown to recruit Shp-2.33–35 As expected, anti-PECAM beads recruited Shp-2 in a manner similar to anti–PVR-coated beads (Figure 2) and also recruited PECAM, which could be detected using a different antibody against PECAM. For experiments with anti–PVR-coated beads, we were unable to check for the recruitment of PVR because of experimental limitations. As an added control, we also performed experiments using microspheres of a different material (glass) coated with antibodies or DNAM-1-Fc. As expected, Shp-2 was similarly recruited in a manner dependent on PVR activation, which shows that the recruitment is not an artifact of the bead composition or labeling (Figure 3).
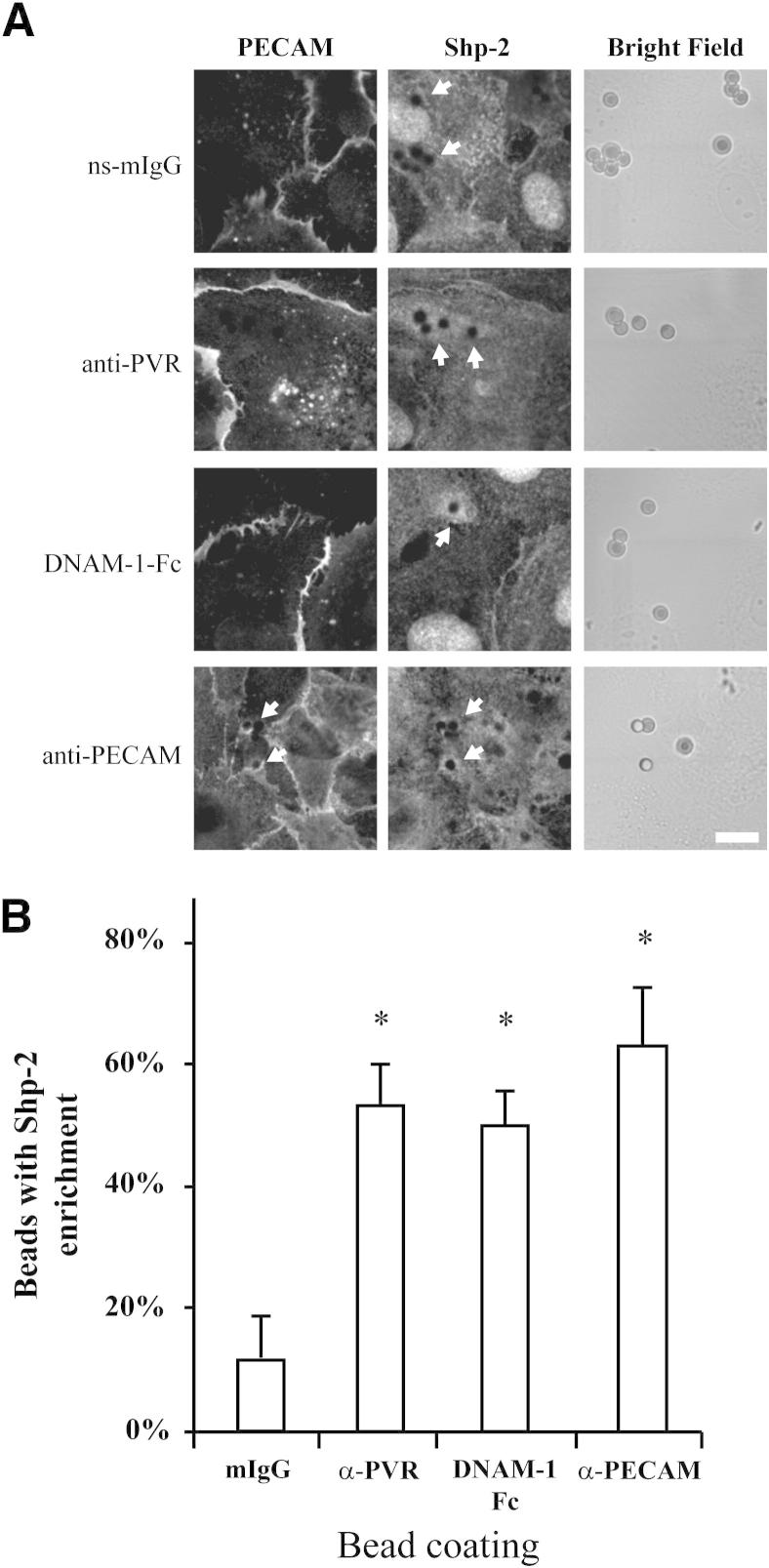
Shp-2 recruitment to coated beads. A: HUVEC monolayers were exposed to polystyrene beads coated with non-specific mouse IgG (ns-mIgG), DNAM-1-Fc chimera, or antibodies against PVR or PECAM for 20 minutes and fixed. Shp-2 and PECAM were visualized using immunofluorescence, as indicated. Beads for the corresponding images were visualized using bright field microscopy. Arrows, beads with Shp-2 recruitment. Shp-2 recruitment around beads was variable, depending on its position on the cell engaged and the length of time the bead had bound. Scale bar = 10 μm. B: The number of beads showing enrichment of Shp-2 fluorescence, as seen in A, was scored from five experiments in which approximately 50 beads were scored at random for each sample. Enrichment was defined as those beads with at least a 1.5-fold increase in intensity in the area immediately surrounding the bead of that compared with the surrounding cytosol. Data shown are the mean and SD from five independent experiments. *P < 0.05, determined using the Student’s t-test for unpaired observations.
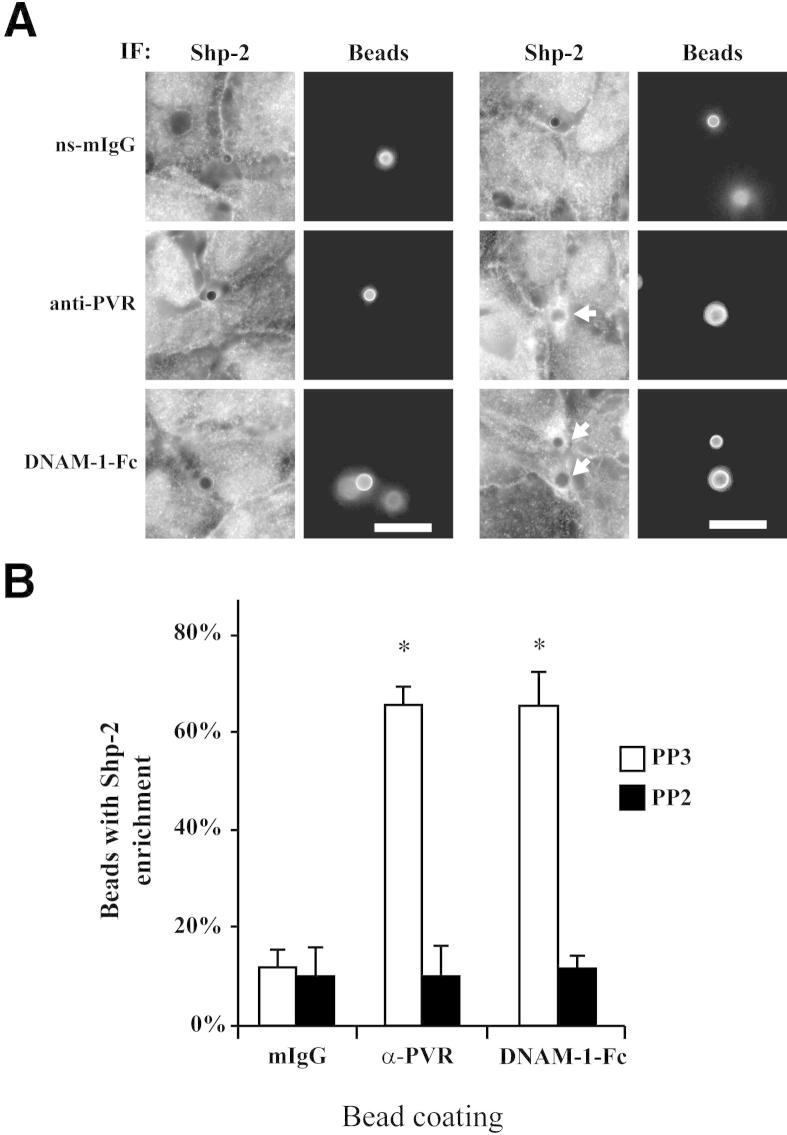
Shp-2 recruitment is dependent on Src kinase. A: Shp-2 recruitment to coated glass beads was visualized, as in Figure 2, in the presence of 5 μmol/L Src kinase inhibitor, PP2, or 5 μmol/L inactive inhibitor analogue, PP3. Beads were coated with the indicated proteins in addition to a small amount of fluorescently labeled non-specific antibodies to aid in their visualization using immunofluorescence (IF). Arrows, beads with Shp-2 recruitment. Data are representative of three independent experiments. Scale bars: 10 μm. B: The number of beads with enrichment was determined, as in Figure 2B. Data shown are the mean and SD from three independent experiments. *P < 0.01. ns-mIgG, non-specific mouse IgG.
Experiments performed in fibroblasts showed that Shp-2 is recruited to PVR after Src-mediated phosphorylation of Y398 in the ITIM motif of PVR.17 To see if Shp-2 recruitment in our system is also dependent on Src kinases, we treated HUVECs with the Src family inhibitor, PP2. After treatment with PP2, Shp-2 recruitment to anti-PVR or DNAM-1-Fc–coated beads (Figure 3) was reduced to baseline levels. Treatment with the inactive analogue, PP3, had no effect, confirming that Src family kinases play a role in Shp-2 recruitment (Figure 3).
Shp-2 Interacts with Phosphorylated PVR
The experiments with coated beads show that the recruitment of Shp-2 is dependent on PVR stimulation and phosphorylation by Src kinases. However, it is possible that Shp-2 is being targeted to some other protein instead of directly interacting with PVR. To further examine this, we immunoprecipitated PVR from HUVECs after treatment with antibodies against PVR, DNAM-1-Fc, or non-specific mouse IgG. Commercially available anti-PVR antibodies do not work well in Western blot analyses; thus, to detect PVR, we chilled the monolayers and biotinylated all surface proteins with a membrane-impermeant reagent after the antibody stimulation but before cell lysis. PVR could then be immunoprecipitated with anti-PVR antibodies and detected on the blot using streptavidin-HRP (Figure 4). Treatment of HUVECs with either anti-PVR antibodies or DNAM-1-Fc induced a significant increase in the amount of phosphorylated PVR. On the same blots, we could detect the co-immunoprecipitation of Shp-2 with phosphorylated PVR. Both the phosphorylation of PVR and its interaction with Shp-2 were inhibited by the Src kinase inhibitor, PP2, further indicating that Shp-2 binds directly to PVR in an Src-mediated, phosphorylation-dependent manner.
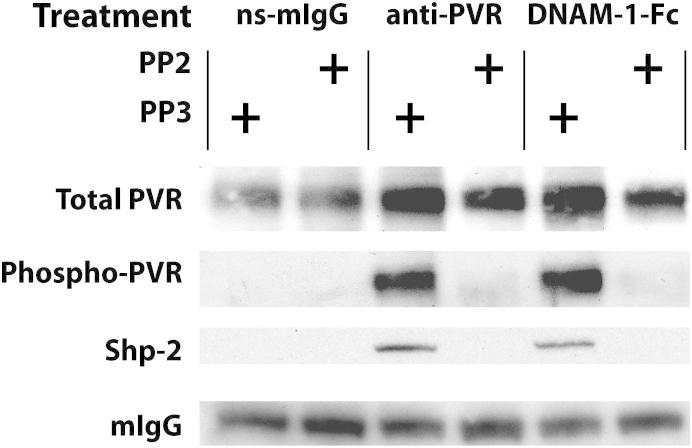
Shp-2 interaction with PVR is dependent on Src kinase. HUVEC monolayers were treated with non-specific mouse IgG (ns-mIgG), antibody against PVR, or DNAM-1-Fc chimera in the presence of Src kinase inhibitor, PP2, or the inactive inhibitor analogue, PP3, as indicated. Monolayers were then chilled, labeled with biotin, and lysed. PVR was immunoprecipitated and visualized using streptavidin conjugated to HRP. Coprecipitated proteins were visualized using corresponding antibodies, as detailed in Materials and Methods. Data are representative of three independent experiments.
PVR and DNAM-1 Function in TEM at a Step in between PECAM and CD99
To investigate the possible connection between PVR, PECAM, and CD99, we investigated the timing of the roles of these proteins during TEM. Toward this end, we used an in vitro assay that is distinct from the one used by Reymond et al.7 Thus, it was important to confirm the role of these molecules in our standard TEM assay using HUVECs grown on hydrated collagen gel matrices. As described in Materials and Methods, PBMCs were incubated on confluent HUVEC monolayers for 1 hour at 37°C in the continuous presence of the indicated monoclonal antibodies. During this time, monocytes settle on and cross the endothelial monolayer unless the antibody disrupts a critical protein-protein interaction required for TEM. In samples incubated with a non-blocking antibody (anti–VE-cadherin), transmigration proceeded uninhibited to a maximal 80%. Confirming previous reports, transmigration was significantly reduced in the presence of antibodies against PECAM and CD99,3,5,7,37 and also by antibodies against PVR and DNAM-17 (Supplemental Figure S2A). Approximately 20% of the monocytes in these samples were able to overcome the antibody blockade, as has been reported for PECAM,27,30,37 CD99,5,38 and PVR and DNAM-1.7,8 The inhibition of TEM was not significantly altered when the samples were incubated simultaneously with any combination of two antibodies (Supplemental Figure S2B). None of the antibody treatments had a significant effect on the total number of leukocytes able to adhere to the monolayers (data not shown), suggesting that these observations are due solely to the ability of these antibodies to block TEM.
To dissect the order of the interactions that facilitate diapedesis, we exploited the fact that antibody blockade of TEM is reversible if the blocking antibody is washed away and samples are given additional time (>1 hour) to undergo transmigration.4,5 If a new antibody is added during this later incubation, TEM will only continue to be blocked if the leukocytes have not already passed the step mediated by the second targeted molecule.5 Thus, by performing two sequential rounds of treatment with different antibody combinations, we can determine the order in which the different proteins function during TEM. As seen in Figure 5A, the initial antibody treatments that effectively blocked TEM (Supplemental Figure S2B) could be reversed by washing out the antibody and allowing TEM to proceed for an additional 2 hours. When the same antibody was added back for the second incubation, TEM remained blocked, demonstrating that the monocytes do not overcome the blockade solely by prolonged incubation (Figure 5B).
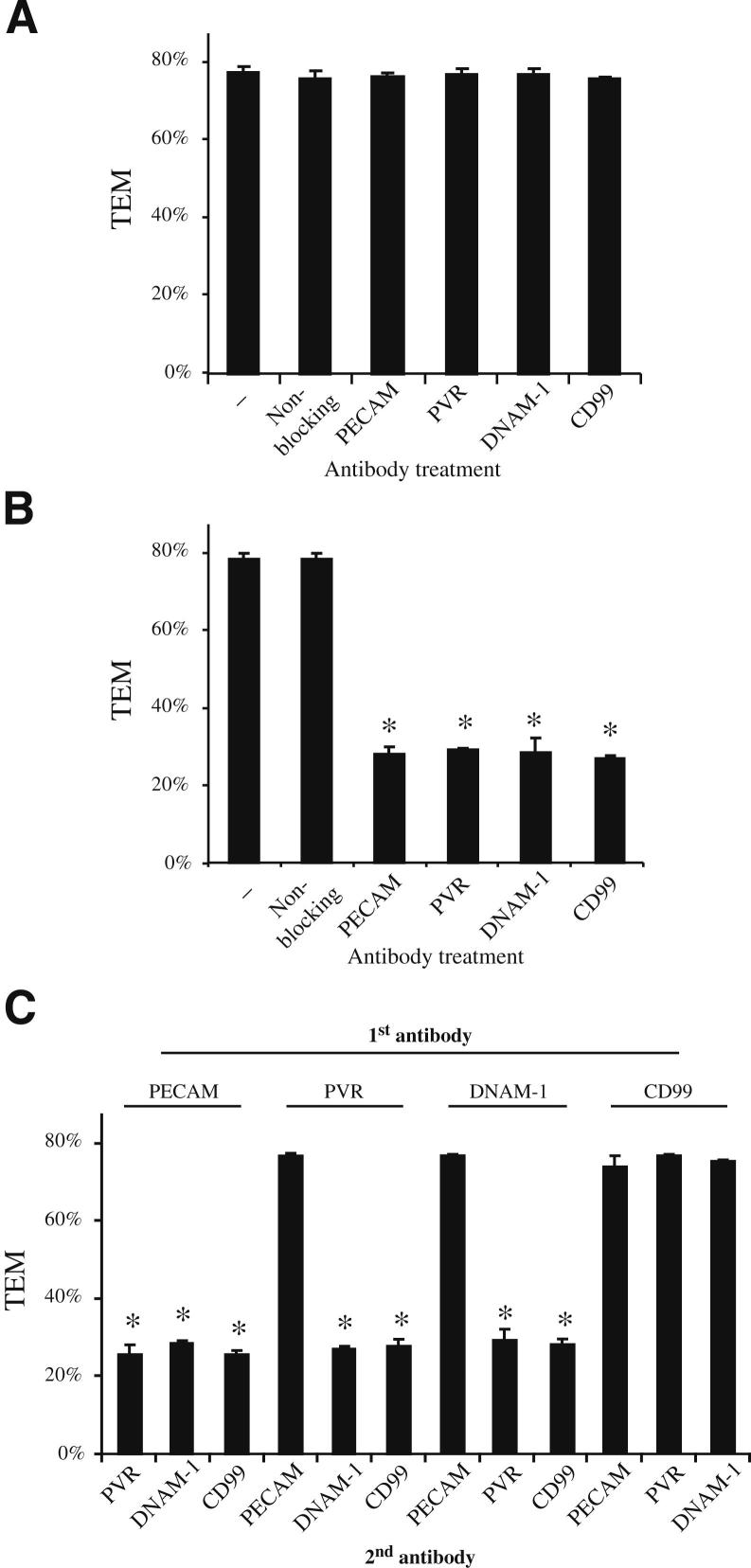
Sequential blockade of TEM. The TEM of PBMCs through HUVEC monolayers was assayed in the absence or continuous presence of antibodies, as described in Materials and Methods for sequential antibody incubations. Samples were initially incubated with antibodies against the indicated molecules, as described for the standard TEM assay. Samples were then chilled, washed extensively, warmed, and subjected to a second incubation in the presence of antibodies (A), the same antibody used for the initial incubation (B), or an antibody against a different protein (C). After the second incubation, the samples were fixed and the TEM was quantified. Data represent the mean of at least four replicates from at least three different experiments. All of the samples for A–C were performed in parallel but are shown separately for clarity. Error bars represent SDs. *P < 0.05, determined using the Student’s t-test for unpaired observations.
By using this assay, we have already shown that PECAM and CD99 act sequentially in TEM.5 As previously shown and reconfirmed herein, blocking PECAM during the first incubation and CD99 during the second incubation result in an effective block of TEM that is comparable to continuous treatment with either antibody treatment alone (Figure 5C). Conversely, blocking CD99 first and PECAM second resulted in no blockade, consistent with PECAM acting upstream of CD99.5
To determine where PVR and DNAM-1 function in TEM relative to PECAM and CD99, we performed a similar analysis using sequential incubations of several combinations of antibodies against PVR and DNAM-1, in addition to PECAM and CD99 (Figure 5C). In all of the samples in which the initial incubation was with anti-PECAM antibodies (Figure 5C), TEM was significantly reduced, suggesting that PVR, DNAM-1, and CD99 all function in TEM at steps later than the step at which PECAM functions. This was confirmed by the observation that TEM was not blocked in any sample (including those treated with anti–DNAM-1 and anti-PVR) in which anti-PECAM antibodies were added only during the second incubation. The opposite findings were obtained when the initial incubations were with antibodies against CD99 (Figure 5C), indicating that CD99 functions after PECAM, PVR, and DNAM-1. These data place PVR and DNAM-1 function in between the activities of PECAM and CD99. Interestingly, regardless of their order, treatment with antibodies against PVR and DNAM-1 produced the same blockade, an observation that is consistent with PVR and DNAM-1 interacting at the same step in TEM. To confirm that these findings are not unique to large-vessel endothelial cells (HUVECs), we repeated the sequential TEM using primary HMVECs. As expected, PECAM, PVR/DNAM-1, and CD99 all acted in the same order as in HUVECs, further confirming the sequential nature of these molecules during transmigration (Supplemental Figure S3). When taken together, these data support the model that PVR interacts with DNAM-1 during TEM at a step between the one controlled by PECAM and the one controlled by CD99.
Only the PVR on the Endothelial Cells Is Functionally Active in TEM
Although PVR is clearly involved in diapedesis (Figure 5 and Supplemental Figure S2), the expression of PVR on both HUVECs and monocytes (Supplemental Figure S1) raises the question of which cell type bears the PVR that is relevant to TEM. To distinguish between these different populations of PVR, we pre-incubated each cell type with the indicated antibodies individually. The cells were then washed extensively, and TEM assayed as before, except that the incubation was shortened to 30 minutes, conditions that were previously shown to maintain the initial blockade in the absence of excess antibody in solution4,5 (Figure 6A). Consistent with our prior observations, pre-incubation of either HUVECs or PBMCs with antibodies against PECAM or CD99 significantly reduced TEM.4,5 TEM was also inhibited by pre-incubating HUVECs with antibodies against PVR or treating PBMCs with antibodies against DNAM-1. On the other hand, no blockade was seen when HUVECs were incubated with antibodies against DNAM-1 or when PBMCs were pre-incubated with antibodies against PVR.
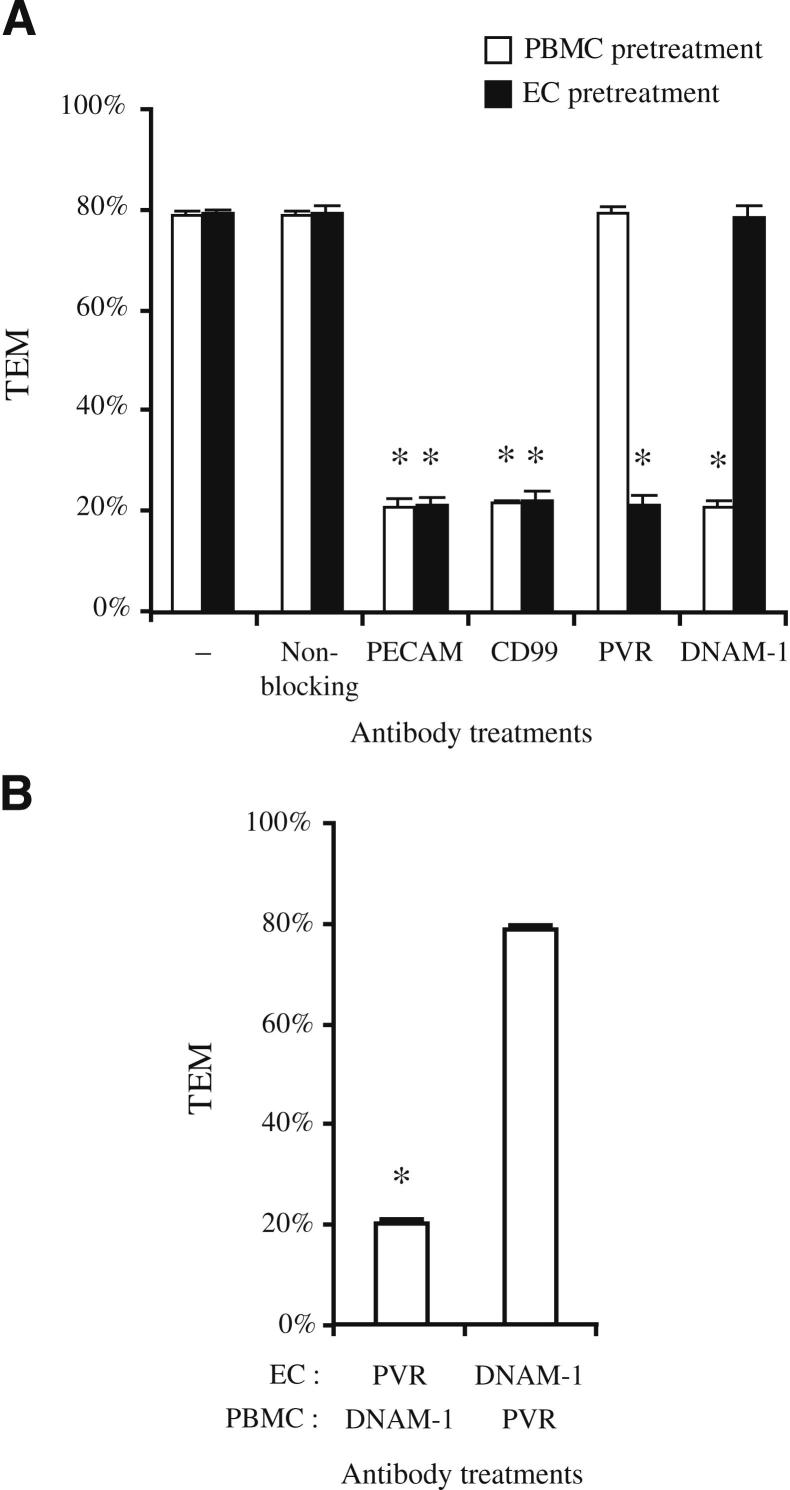
Cell type–specific blockade of TEM. A: HUVECs or PBMCs were pre-incubated with antibodies against the indicated molecules before being washed and used in the standard TEM assay (as described in Materials and Methods). B: TEM was assayed in samples in which HUVECs (treated at 37°C) and PBMCs (treated at 4°C) were differentially treated with either antibodies against PVR or DNAM-1, as indicated; both cell types were washed extensively before the TEM assay. The data shown in A and B are from the same experiments; they are separated into two graphs for clarity. Data represent the mean of at least three replicates from at least three independent experiments. Error bars represent SDs. *P < 0.05, determined using the Student’s t-test for unpaired observations.
As an additional control, we incubated monocytes and endothelial cells that had each been differentially, but simultaneously, treated with antibodies against PVR and DNAM-1 and washed before assaying TEM (Figure 6B). As expected, TEM was not inhibited when monocytes were pretreated with anti-PVR antibody and endothelial cells were pretreated with anti–DNAM-1. However, TEM was inhibited by blockade of monocyte DNAM-1 and endothelial cell PVR, and the blockade was no better than that observed when either protein was blocked individually, further supporting a direct functional interaction during TEM between endothelial cell PVR and monocyte DNAM-1.
PVR Is in the LBRC
PECAM and CD99 have both been shown to reside in the LBRC. Because PVR acts between these two, we reasoned that a subpopulation of PVR might also reside in the LBRC. It has been shown that PECAM in the LBRC is sequestered from extracellular reagents during treatment at 4°C, and it is this protected pool of PECAM that functions in TEM.5 To determine whether PVR is similarly sequestered, HUVECs were pretreated with anti-PVR antibody at either 4°C or 37°C and rinsed extensively before the addition of PBMCs. In contrast to treatment at 37°C, TEM was not inhibited when HUVECs were pretreated at 4°C with antibodies against PVR (Figure 7A), suggesting that the functional pool of PVR also resides in the LBRC in a manner analogous to PECAM and CD99.
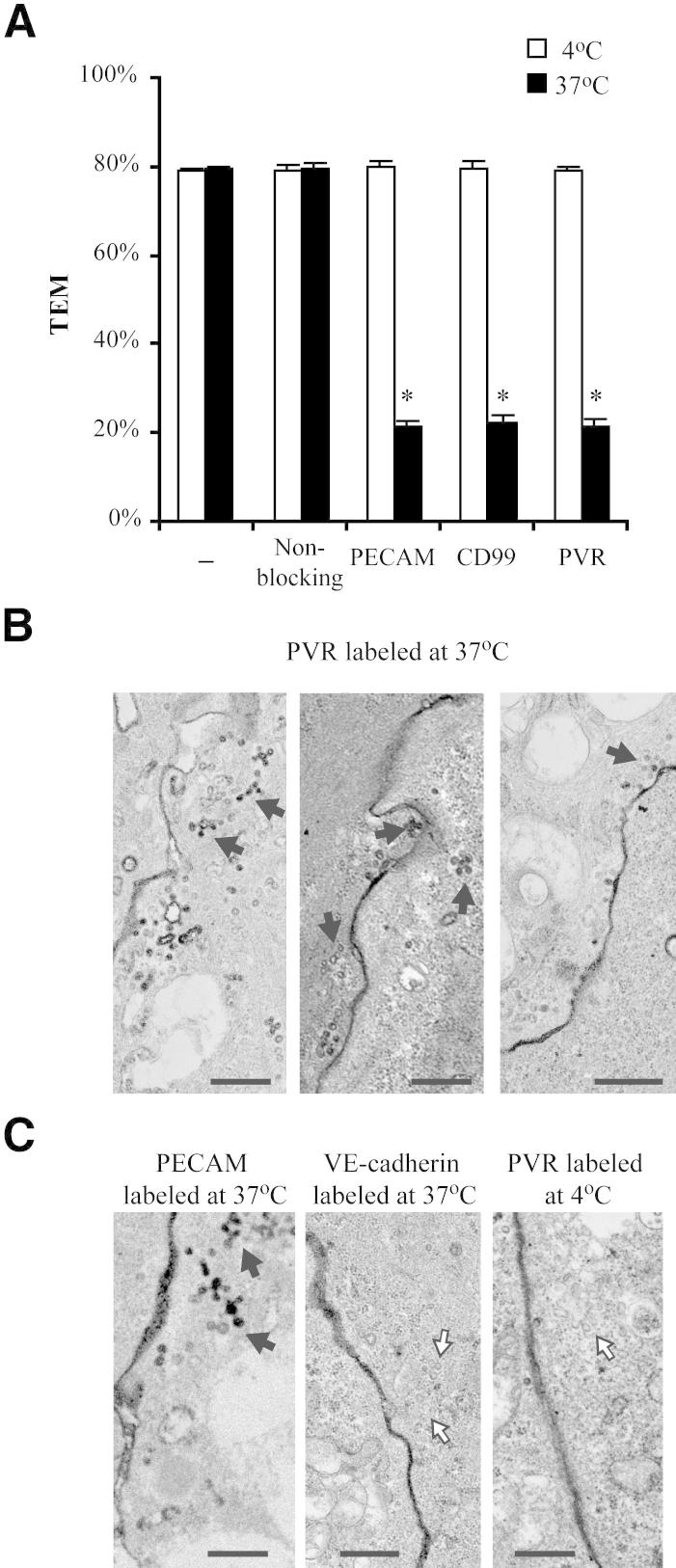
LBRC localization of PVR. A: The standard TEM assay was performed as described in Materials and Methods, except that HUVECs were pretreated for 45 minutes with antibodies against the indicated molecules at 4°C or 37°C. Monolayers were then washed at 4°C to remove unbound antibodies before adding PBMCs and continuing with the standard assay. B and C: HUVEC monolayers treated with HRP-conjugated antibodies, as indicated at either 4°C or 37°C, were processed for immuno-EM, as described in Materials and Methods. Black arrows, LBRC-interconnected vesicles visualized by diaminobenzidene staining; white arrows, likely unstained LBRC vesicles. Scale bars: 500 nm (B and C).
To examine this more directly, we analyzed the subcellular distribution of PVR using immunoelectron microscopy. Images obtained from HUVECs labeled at 37°C with HRP-conjugated anti-PVR antibody (Figure 7B) show the unique interconnected tubulovesicular structures that are characteristic of the LBRC, morphologically indistinguishable from staining with anti-PECAM antibodies (Figure 7C) and in contrast to staining for VE-cadherin, a junctional protein that is known to be excluded from the LBRC.39 In addition, incubating HUVECs with anti-PVR antibodies at 4°C only labeled the junctions and not the LBRC (Figure 7C). Under these conditions, unlabeled LBRC was visible, further demonstrating the exclusion of the antibody from this compartment at 4°C and indicating that the lack of LBRC staining is not a sampling issue (Figure 7C). This temperature-sensitive differential labeling has only been reported for other confirmed LBRC proteins and further demonstrates that PVR is also in the LBRC.
Discussion
Leukocyte extravasation involves sequential steps of tethering, rolling, activation, adhesion, locomotion, and diapedesis, each involving the interaction of different molecules on the leukocyte and endothelial surfaces.40,41 The process of diapedesis, in which the leukocyte migrates between two tightly apposed endothelial cells into the site of inflammation, has been shown to involve the sequential roles of two separate molecules, PECAM and CD99, each separately interacting in a homophilic manner between the leukocyte and endothelial cell.5 Herein, we confirm that the molecules PVR and DNAM-1 also act in diapedesis, and that they act together at the same step. Prior studies have shown that these molecules form a ligand-receptor pair,7,8,11,16 a feature in contrast to the homophilic intercellular interactions of PECAM and CD99. Our new studies contribute several novel findings: i) similar to PECAM, endothelial PVR engagement and subsequent phosphorylation recruit Shp-2 to the site of interaction; ii) the process of TEM can be molecularly dissected into at least three steps; iii) the interaction of leukocyte DNAM-1 with endothelial cell PVR regulates an intermediate step (between those controlled by PECAM and CD99) for monocyte TEM; iv) such as PECAM and CD99, PVR resides in the LBRC; and v) it is the fraction of PVR molecules that reside in the LBRC that is critical for TEM. A potential common theme emerges from this work that the LBRC may be a shared membrane platform on which the molecules that regulate TEM are arrayed to perform their functions. For at least PECAM and PVR, this common function also involves recruiting Shp-2.
Acquiring these data depended on the reversible blockade of specific required protein-protein interactions. Anti-PECAM, anti-CD99, and anti-PVR arrest TEM for as long as they are present. Once excess antibody is removed, however, processing of bound antibody on the endothelial cell and leukocyte ensues and concentrations decrease lower than the critical level for blockade of cognate molecules within approximately 1 hour, allowing TEM to resume. If a different blocking antibody is added during this time, however, that antibody is capable of binding its ligand and potentially halting the recovery of TEM. In this way, PECAM, CD99, and PVR could all be blocked separately and in a particular order. When one molecule (eg, PECAM) functions upstream of another (eg, PVR), blockade of the upstream molecule can be lifted and replaced with blockade of the downstream molecule, resulting in retained inhibition of TEM. If blocked in the reverse order, however, TEM is not inhibited when the block of the downstream molecule is lifted because the leukocyte has already migrated past the step that is regulated by the upstream molecule. In addition, sequential treatments using PVR and DNAM-1 antibodies inhibited TEM, regardless of the order of the treatments (Figure 5C), a finding that is only consistent with DNAM-1 and PVR functioning at the same step in TEM.
The LBRC is a subjunctional reticulum composed of interconnected 50-nm vesicle-like structures that are contiguous with the plasma membrane, distinct from caveolae, and not accessible to extracellular antibodies at 4°C.3 Data presented herein show that PVR localizes to the LBRC, similar to PECAM and CD99 (Figure 7). Based on the similar morphological characteristics of the PVR-containing structures and the PECAM-containing LBRC (Figure 7B) and the similar 4°C protection of PVR, PECAM, and CD99 (Figure 6A), it is likely that PVR colocalizes in the LBRC with PECAM and CD99, rather than being segregated into distinct subsections. This is further supported by the finding that PVR functions a step between PECAM and CD99 (Figure 5C).
We have previously shown that this compartment recycles to surround migrating leukocytes, and we hypothesize that this targeted movement of the LBRC could serve to deliver unligated adhesion molecules for critical interactions and signals to promote TEM and provide additional membrane surface area to accommodate the migrating leukocyte, which would be particularly required for transcellular TEM.3,39 Thus, the LBRC could function as both a delivery mechanism and a storage compartment for proteins that facilitate TEM. Together, these data support a model in which the functionally active pools of PECAM, PVR, and CD99 are all localized to the LBRC so that they may be targeted to the transmigrating leukocyte together.
Shp-2 has been shown to interact with PVR in other systems.16,17 Herein, we show that the two interact in a stimulus-dependent, Src kinase–dependent mechanism in primary endothelial cells. Data from fibroblasts suggest that this interaction occurs through phosphorylation of the ITIM domain of PVR.17 Interestingly, Shp-2 has also been shown to bind to the phosphorylated ITIM motif of PECAM.33–35 The respective roles of PECAM and PVR in this process, and the exact role of Shp-2, are areas of ongoing research. We hypothesized that PECAM stimulation could somehow affect the timing or amount of Shp-2 that interacts with PVR, perhaps by priming PVR to accept Shp-2. However, no significant change was observed in the kinetics or amount of Shp-2 association with PVR on prior or continuous stimulation of PECAM (data not shown). This could suggest that PVR and PECAM each require Shp-2 separately for their individual downstream signaling. Alternatively, it is possible that the recruitment of Shp-2 by PECAM, PVR, and/or other junctional proteins could serve to increase Shp-2 concentration in the area of transmigration to a critical threshold that, by itself, could be an important signal. Perhaps Shp-2 is recruited to the junction to turn off several of the signaling cascades initiated by leukocyte interaction. Indeed, the entire diapedesis process is initiated and completed within a few minutes. During this time, several receptors and signaling molecules are rapidly activated.2,42 It is likely that the endothelial cell would need to rapidly down-regulate or turn off these activated receptors and signals, possibly to preserve junctional integrity or to reset the mechanism for additional rounds of TEM.
One interesting observation of this study is the apparent paradox regarding how the D171 antibody (anti-PVR) can simultaneously stimulate PVR and block transmigration. Evidence from other studies suggests that antibody-mediated blockades/treatments often have this sort of result (ie, activation of the molecule but disruption of its function). Indeed, anti-PECAM antibody had the same effect (Figure 25,6). Although it is tempting to speculate that the only relevant result of PVR activation is the recruitment of Shp-2, in reality the process is likely to be much more complex. Indeed, the observation that the D171 antibody is able to recruit Shp-2 but still blocks TEM (a finding observed in part by other groups7,8) suggests that the recruitment of Shp-2 is not the sole function of PVR. This disconnect could be the result of altered spatiotemporal regulation of the target molecule. In other words, the molecule is stimulated, but not at the right time or location to elicit the desired outcome. Extrapolation of these findings to our study strongly suggests that D171 is activating PVR, leading to phosphorylation and Shp-2 recruitment; however, because this stimulation does not occur in the precise order and timing required for transmigration, the whole TEM process is disrupted and the leukocytes cannot migrate efficiently. Furthermore, this antibody treatment also blocks the interaction of PVR with its obligate binding partner, DNAM-1, thus preventing the reciprocal activation of DNAM-1 on the leukocyte, which could likely be an equally important signaling event during TEM. Indeed, this sort of antibody-mediated disruption, including Src-mediated phosphorylation and Shp-2 recruitment, has been reported for anti-PECAM antibody treatment, and additional data suggest that this is the mechanism by which antibody treatments can be used to disrupt extravasation at several different steps both in vivo and in vitro.
This elucidation of the mechanism of PVR and DNAM-1 function during TEM adds to the increasing information about the growing number of molecules involved in the process of diapedesis. One might envision that the relative roles of the different receptors and the different signaling mechanisms may vary based on the context (ie, the site of inflammation and the nature of the stimulus) and, in fact, data already exist to suggest such differential regulation of TEM.43–46 Alternately, these mechanisms may introduce a system of potential redundancy that would ensure that an evolutionarily essential process continues, even in the face of partial inhibition. Thus, understanding how the various molecules can potentially mediate TEM function will be essential to effective treatment. Ultimately, the complete understanding of these mechanisms will be essential to facilitate therapeutic intervention into hypoinflammatory and hyperinflammatory conditions in highly specific ways, without global disruption of immune function.
Acknowledgments
We thank Clifford D. Carpenter and Ron Liebman for assistance with experiments; Drs. Oliver Florey, Gong Feng, Fei Han, and Sumana Sanyal for insightful discussions; and Begum Kutay for thoughtful review of the manuscript.
Footnotes
Supported by NIH grant F32 AI084454 (D.P.S.), NIH Medical Scientist Training Program grant GM07739 (M.A.S.), and NIH grants R01 HL046849 and R37 HL064774 (W.A.M.).
Current address of M.A.S., Department of Pathology, Brigham and Women’s Hospital, Boston, Massachusetts.
Supplemental Data
PVR and DNAM-1 expression and localization. A: The indicated cell types were isolated and assayed for their expression of PVR (left panel) and DNAM-1 (right panel) using flow cytometry, as described in Materials and Methods. Thin lines represent samples labeled with an irrelevant isotype control; thick lines, samples labeled with antibodies against PVR or DNAM-1, as indicated. B: Immunofluorescence shows junctional localization of PECAM, PVR, and CD99. HUVECs were grown and stained with antibodies against the indicated targets, as described in Materials and Methods. Scale bar = 20 μm.
TEM blockade controls. TEM was assayed using HUVECs, as described in Materials and Methods, in the presence of monoclonal antibodies against PECAM, CD99, PVR, and DNAM-1, non-blocking monoclonal antibody against VE-cadherin, or DNAM-1-Fc chimera (A), or pairs of these (B); each reagent was used at a concentration of 20 μg/mL, with the overall concentration held at 40 μg/mL, adjusted with non-blocking antibody, as needed. The data shown in A and B are from the same experiments; they are separated into two graphs for clarity. Data shown are the mean percentage TEM of at least three different experiments with at least three replicates for each variable tested. Error bars represent SDs. All treatments blocked TEM significantly compared with no treatment or non-blocking antibody control. *P < 0.05, determined using the Student’s t-test for unpaired observations, relative to non-blocking antibody control.
Sequential blockade in HMVECs. The TEM of PBMCs through HMVEC monolayers was assayed in the absence or continuous presence of antibodies, as described in Materials and Methods for sequential antibody incubations. Samples were initially incubated with antibodies against the indicated molecules, as described for the standard TEM assay. Samples were then chilled, washed extensively, warmed, and subjected to a second incubation in the presence of an antibody against a different protein. After the second incubation, the samples were fixed and TEM was quantified. Data represent the mean of at least three replicates from at least three different experiments. Error bars represent SDs. *P < 0.05, determined using the Student’s t-test for unpaired observations, relative to non-blocking antibody control.
References
Articles from The American Journal of Pathology are provided here courtesy of American Society for Investigative Pathology
Full text links
Read article at publisher's site: https://doi.org/10.1016/j.ajpath.2012.11.037
Read article for free, from open access legal sources, via Unpaywall:
http://ajp.amjpathol.org/article/S0002944013000059/pdf
Citations & impact
Impact metrics
Citations of article over time
Alternative metrics
Smart citations by scite.ai
Explore citation contexts and check if this article has been
supported or disputed.
https://scite.ai/reports/10.1016/j.ajpath.2012.11.037
Article citations
An Intriguing Structural Modification in Neutrophil Migration Across Blood Vessels to Inflammatory Sites: Progress in the Core Mechanisms.
Cell Biochem Biophys, 82(1):67-75, 14 Nov 2023
Cited by: 3 articles | PMID: 37962751
Review
CDK4/6 inhibitors and the pRB-E2F1 axis suppress PVR and PD-L1 expression in triple-negative breast cancer.
Oncogenesis, 12(1):29, 26 May 2023
Cited by: 3 articles | PMID: 37230983 | PMCID: PMC10213015
Nectins and Nectin-like molecules drive vascular development and barrier function.
Angiogenesis, 26(3):349-362, 03 Mar 2023
Cited by: 8 articles | PMID: 36867287
Review
Nectin Family Ligands Trigger Immune Effector Functions in Health and Autoimmunity.
Biology (Basel), 12(3):452, 15 Mar 2023
Cited by: 3 articles | PMID: 36979144 | PMCID: PMC10045777
Review Free full text in Europe PMC
CD155 promotes radioresistance and malignancy of esophageal cancer by regulating Hippo-YAP pathway.
Discov Oncol, 13(1):53, 29 Jun 2022
Cited by: 3 articles | PMID: 35768666 | PMCID: PMC9243211
Go to all (50) article citations
Data
Data behind the article
This data has been text mined from the article, or deposited into data resources.
BioStudies: supplemental material and supporting data
Similar Articles
To arrive at the top five similar articles we use a word-weighted algorithm to compare words from the Title and Abstract of each citation.
DNAM-1 and PVR regulate monocyte migration through endothelial junctions.
J Exp Med, 199(10):1331-1341, 10 May 2004
Cited by: 180 articles | PMID: 15136589 | PMCID: PMC2211807
4D intravital microscopy uncovers critical strain differences for the roles of PECAM and CD99 in leukocyte diapedesis.
Am J Physiol Heart Circ Physiol, 311(3):H621-32, 15 Jul 2016
Cited by: 13 articles | PMID: 27422987 | PMCID: PMC5142183
Rapid remodeling of tight junctions during paracellular diapedesis in a human model of the blood-brain barrier.
J Immunol, 193(5):2427-2437, 25 Jul 2014
Cited by: 57 articles | PMID: 25063869 | PMCID: PMC4138548
Localized signals that regulate transendothelial migration.
Curr Opin Immunol, 38:24-29, 14 Nov 2015
Cited by: 32 articles | PMID: 26584476 | PMCID: PMC4715928
Review Free full text in Europe PMC
Funding
Funders who supported this work.
NHLBI NIH HHS (2)
Grant ID: R01 HL046849
Grant ID: R37 HL064774
NIAID NIH HHS (1)
Grant ID: F32 AI084454
NIGMS NIH HHS (2)
Grant ID: T32 GM007739
Grant ID: GM07739