Abstract
Free full text

Androgen receptor-independent function of FoxA1 in prostate cancer metastasis
Abstract
FoxA1 (FOXA1) is a pioneering transcription factor of the androgen receptor (AR) that is indispensible for the lineage-specific gene expression of the prostate. To date, there have been conflicting reports on the role of FoxA1 in prostate cancer progression and prognosis. With recent discoveries of recurrent FoxA1 mutations in human prostate tumors, comprehensive understanding of FoxA1 function has become very important. Here, through genomic analysis we reveal that FoxA1 regulates two distinct oncogenic processes via disparate mechanisms. FoxA1 induces cell growth requiring the AR pathway. On the other hand, FoxA1 inhibits cell motility and epithelial-to-mesenchymal transition (EMT) through AR-independent mechanism directly opposing the action of AR signaling. Using orthotopic mouse models we further show that FoxA1 inhibits prostate tumor metastasis in vivo. Concordant with these contradictory effects on tumor progression, FoxA1 expression is slightly up-regulated in localized prostate cancer wherein cell proliferation is the main feature, but is remarkably down-regulated when the disease progresses to metastatic stage for which cell motility and EMT are essential. Importantly, recently identified FoxA1 mutants have drastically attenuated ability in suppressing cell motility. Taken together, our findings illustrate an AR-independent function of FoxA1 as a metastasis inhibitor and provide a mechanism by which recurrent FoxA1 mutations contribute to prostate cancer progression.
INTRODUCTION
FoxA1 (FOXA1), also named HNF-3α, is a winged-helix transcription factor of the forkhead family. It plays essential roles in the epithelial differentiation and development of a number of organs including the pancreas, prostate and breast (1–6). For example, while FoxA1-knockout mice are developmentally lethal, conditional FoxA1 knockout in the mouse prostate results in severely altered ductal development that contains immature epithelial cells surrounded by abnormally thick stromal layers (7). Concordantly, in the adult prostate FoxA1 has also been tightly linked to the maintenance of the prostate epithelial phenotype and the expression of prostate-specific genes. This is mediated through its regulation of the Androgen Receptor (AR) transcriptional activities (8; 9). As a pioneering factor FoxA1 opens up compact chromatin to facilitate subsequent AR recruitment (4; 10–13). Genome-wide location analysis of prostate cancer cells have shown that FoxA1 pre-occupies lineage-specific enhancers even before androgen stimulation and co-occupies a majority of AR binding sites in androgen-treated cells. FoxA1 is thus indispensible for defining a prostatic AR program and is critical to prostate development, function, as well as malignant transformation.
Consistent with its being a pro-differentiation factor, functionally FoxA1 has been shown to inhibit epithelial-to-mesenchymal transition (EMT) in pancreatic cancer cells (14). Low FoxA1 level has been associated with high grade, late-stage tumors in bladder cancer (15). Similarly, FoxA1 exhibits tumor suppressive functions in breast cancer and high FoxA1 expression is correlated with favorable prognosis in ER-positive breast tumors (16). However, reports of FoxA1 function in prostate cancer (PCa) have been controversial. FoxA1 level has been associated with either good or bad clinical outcomes depending on the patient cohort. For example, some studies have shown that high FoxA1 expression is associated with worse prognosis probably by enhancing AR activity (17; 18), while other studies have demonstrated an association of decreased FoxA1 expression with castration-resistant, poor prognosis prostate tumors (19). Concordantly, functional studies have shown that FoxA1 increases cell proliferation through regulation of cell cycle genes, which may be in part modulated via its control of AR-mediated transcriptional regulation. FoxA1 has also been implicated in the EMT of prostate cancer cells (18; 20; 21). Comprehensive analysis of FoxA1 function however has not been attempted and whether or not FoxA1 can regulate PCa independently of AR is unclear.
Genomic studies have recently revealed recurrent FoxA1 gene mutations in human prostate tumors (22; 23). Through next-generation sequencing, 4 of 111 exomes were found to harbor non-silencing mutations to the FoxA1 gene including the M253K mutation. Similarly, in another study 5 FoxA1 mutations including F400I mutation are found in 3.4% of human prostate tumors (both localized and metastatic PCa) (22). Most mutations cluster on the C-terminal transactivation domain of the FoxA1 gene, likely disrupting its binding to DNA and attenuating its transactivation of target genes (23). How these mutations contribute to PCa progression and what are the key downstream targets, however, are yet to be understood.
In the present study, through genomic analysis we found that FoxA1 regulates two distinct oncogenic processes through respective manipulation of genes involved in cell cycle and cell motility. Combining functional assays with gene expression microarrays we demonstrate that FoxA1 induces cell growth but inhibits cell motility through AR-dependent and –opposing mechanisms, respectively. We then pinpoint a key downstream molecule involved in its anti-motility function and demonstrate the relevance of this regulation in vivo and in human specimens. We further provide evidence that recurrent FoxA1 mutations found in patients have attenuated anti-motility ability.
MATERIALS AND METHODS
Patient tissue specimens
Patient samples were requested from the Northwestern University Prostate Cancer Specialized Program of Research Excellence (SPORE) Tissue Core. All samples were collected with informed consent of the patients and prior Institutional Review Board approval. Prostate tissue was harvested according to standard operating procedures of the Prostate SPORE Tissue Bank as previously reported (24; 25). Briefly, tissue was snap frozen within 20–30 min after surgical removal and stored at −80°C.
Plasmids, siRNAs, and antibodies
Human FoxA1 cDNA was amplified by reverse transcription-PCR from LNCaP cells and cloned into the entry vector pCR8/GW/TOPO (Invitrogen). The pCR8-FoxA1 F400I and M252K mutants were generated using QuikChange® II Site-Directed Mutagenesis Kit (Agilent Technologies, Santa Clara, CA). Adenoviral construct expressing FoxA1 was generated by recombining pCR8-FoxA1 with pAD/CMV/V5 using LR Clonase II (Invitrogen). Control pAD/CMV/LACZ clones were obtained from Invitrogen. Lentiviral constructs were generated by LR recombination between pCR8-FoxA1 constructs and pLenti CMV/TO Puro DEST (Addgene plasmid 17293). The pGIPZ lentiviral control, FoxA1 and SLUG shRNAmir were purchased from Open Biosystems. The pLenti-CMV-puro-Luc lentiviral luciferase construct (Addgene plasmid 17477) was used to monitor tumor growth in living mice. FoxA1 siRNA (sense 5′-GAGAGAAAAAAUCAACAGC-3′; antisense 5′-GCUGUUGAUUUUUUCUCUC-3′) (26) and control Luciferase GL2 Duplex siRNA (D-001100-01-20) were synthesized by Dharmacon. The antibodies used in this study include anti-E-Cad (MAB3199) from Millipore, anti-FoxA1 (ab23738) and anti-GAPDH-HRP (ab9385) from Abcam, anti-SLUG (9585s) and anti-Vimentin(3932p) from Cell Signaling, and anti-Vimentin (sc-2620) from Santa Cruz.
ChIP and ChIP-PCR analysis
ChIP was carried out as described previously (25; 30; 31). All primers (listed in Supplementary Table S7) were designed using Primer 3 (http://frodo.wi.mit.edu/primer3/), synthesized by Integrated DNA Technologies, and utilized for SYBR Green based real-time PCR. ChIP-PCR enrichment of target loci was normalized to input DNA.
Gene expression array and data analysis
Total RNAs were isolated using TRIzol reagent (Invitrogen). The integrity of the RNA was monitored using Bioanalyzer 2100. Microarray profiling was performed using HumanHT-12 v 4.0 Expression BeadChip (Illumina). Bead-level data were preprocessed using GenomeStudio (Illumina), and the expression values were quantile-normalized using the beadarray package in Bioconductor. Differentially expressed genes were identified using a 2-fold cutoff. Gene Ontology (GO) terms enrichment was analyzed using GOrilla (http://cbl-gorilla.cs.technion.ac.il/) (27).
Cell proliferation, migration and invasion assay
Cell proliferation assay was carried out using the WST-1 kit according to the manufacturer’s instruction (Clontech). Briefly, cells were seeded in a 24-well plate at a density of 5000~20000 cells in 500 ul of complete culture medium and cultured in a CO2 incubator at 37 oC for 24~48 hours prior to assay. After adding 50 μl WST-1 reagents per well, cultures were incubated for 2 hours and the absorbance at a wavelength of 450 nm was determined using a microplate reader. Cell migration and invasions assays were carried out as previously reported (28) and described in detail in Supplementary Experimental Procedures.
Murine Orthotopic Xenograft Model
Mouse handling and experimental procedures were approved by the Center for Animal and Comparative Medicine in Northwestern University School of Medicine in accordance with the US National Institutes of Health Guidelines for the Care and Use of Laboratory Animals and the Animal Welfare Act. Athymic male nude mice (nu/nu; 6 weeks old) were purchased from the Jackson Laboratory. Orthotopic implantations were carried out as previously described (29). Briefly, a suspension of PC-3M control or FoxA1-expressing stable cells (2 × 105 cells in 50 μl of PBS) was injected into the mouse prostate exposed by surgery. To monitor tumor growth, mice were imaged weekly with the IVIS Spectrum In vivo imaging system (Caliper Life Sciences, Hopkinton, MA).
Accession numbers
The microarray data have been deposited in the National Center for Biotechnology Information Gene Expression Omnibus database under the accession number GSE37314.
RESULTS
Genomic analysis reveals FoxA1 regulation of two distinct oncogenic processes
In order to shed some light on the potential functions of FoxA1, we first attempted to gain a comprehensive profile of its downstream molecules and pathways. Using FoxA1-targeting shRNA, we generated LNCaP cells with stable FoxA1 knockdown (Fig. 1A). The cells were then profiled for global gene expression using microarrays. Analysis of triplicate experiments identified 405 genes that were decreased and 516 genes increased by FoxA1 knockdown (Fig. 1B–C). Using qRT-PCR, we confirmed a random set of 11 genes (Supplementary Fig. S1A). To gain some insights into the molecular pathways in which these FoxA1-regulated genes may be involved, we carried out GO analysis (27). Our data revealed that FoxA1-induced genes, i.e., down-regulated upon FoxA1 knockdown, strongly overlap with genes that regulate cell cycle process, DNA replication, and cell division (Fig. 1B, Supplementary Table S1). In good agreement with this, FoxA1-suppressed genes, on the other hand, are associated with cell apoptosis and death pathway genes (Fig. 1C, Supplementary Table S2). These findings suggest a role of FoxA1 in positively regulating cell growth, being consistent with its known function as a cofactor of AR, a critical mediator of PCa growth. Indeed, microarray analysis of gene expression in control and FoxA1-knockdown cells grown in un-stimulated and androgen-stimulated medium showed that androgen and FoxA1 synergistically induce a core set of cell cycle genes in PCa (Fig. 1D).
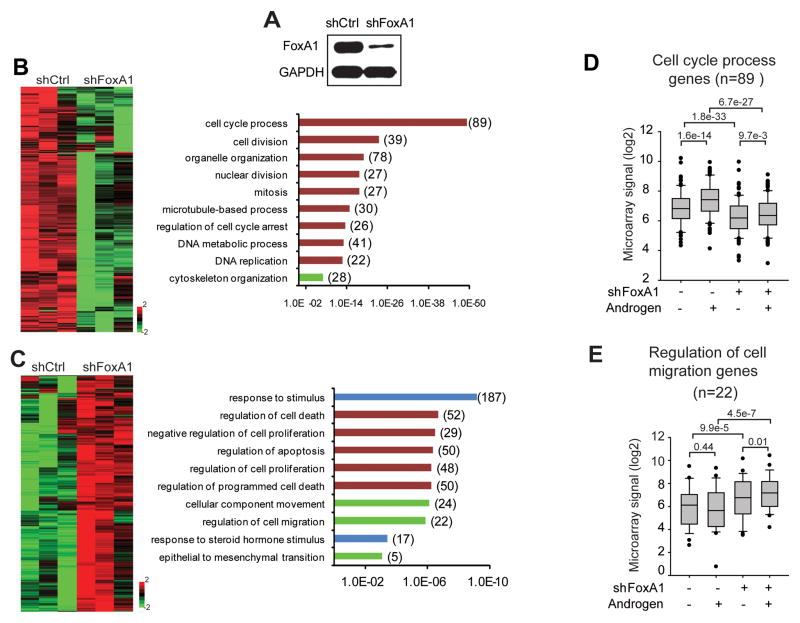
A, Western blot of FoxA1 protein in control and knockdown LNCaP cells. GAPDH was used as a loading control. B–C, Heatmap view and GO analysis of FoxA1-induced (B) and -repressed genes (C). Differentially expressed genes were identified through microarray analysis of control and FoxA1-knockdown LNCaP cells using a 2-fold cutoff and p-value threshold of 0.01. GO concepts involved in cell cycle are shown in red bars, cell motility in green and androgen response in blue. The P values and the number of genes in each GO category are indicated at the x-axis and next to the bar, respectively. D–E, LNCaP shFoxA1 and control cells were cultured in hormone-depleted medium or treated with 1 nM R1881 for 24 hours. Total RNA was isolated and analyzed by microarrays. Expression levels of FoxA1-induced cell cycles genes (D) and FoxA1-repressed cell migration genes (E) were determined.
Interestingly, we found that FoxA1-inhibited genes are also enriched for genes that control cell motility and EMT (Fig. 1C). This new function is in great agreement with the role of FoxA1 as an epithelial pro-differentiation factor. To understand how this role of FoxA1 relates to AR function, we analyzed aforementioned microarray data and found that the loss of FoxA1 significantly induces a core set of cell migration genes regardless of the androgen context, while androgen does not have a significant impact on their expression (Fig. 1E). Taken together, genomic analysis revealed that FoxA1 may regulate two distinct oncogenic processes, namely cell proliferation and cell motility, through AR-dependent and –independent mechanisms, respectively.
To validate this key observation and also to preclude off-target effects and potential adaptive responses from shRNA-mediated stable knockdown, we used an independent siRNA that targets a different region of the FoxA1 gene to transiently deplete FoxA1 in LNCaP cells. Microarray expression profiling and GO analysis of siFoxA1-regulated genes revealed similar enrichment of cell cycle and cell motility genes (Supplementary Fig. S1B–C, Table S3–4). Moreover, this finding was further confirmed in 22RV1 cells, as an independent prostate cancer cell line model, through control and shFoxA1 knockdown (Supplementary Fig. S1D–E, Table S5–6). In concordance with the AR-dependent and –independent roles of FoxA1, analysis of FoxA1 and AR ChIP-Seq data revealed that less than 20% of FoxA1 binding sites are co-occupied by AR, leaving over 80% binding events mediating potentially AR-independent function (Supplementary Fig. S1F). Furthermore, integrative analysis of FoxA1 ChIP-Seq and microarray data revealed that over 60% of FoxA1-regulated genes harbor at least one FoxA1 binding event within 50kb of the gene, supporting direct FoxA1 transcriptional regulation (Supplementary Fig. S1G). Next, we attempted functional assays to affirm the two disparate roles of FoxA1 in prostate carcinogenesis.
FoxA1 increases cell proliferation through the AR
To assess the effect of FoxA1 on cell proliferation, we carried out cell growth assays in a panel of prostate cancer cell lines following ectopic FoxA1 overexpression or knockdown. In LNCaP cells, we found that FoxA1 knockdown dramatically reduced cell growth (Fig. 2A) while FoxA1 overexpression promoted cell growth (Supplementary Fig. S2A). To further confirm this we performed FoxA1 knockdown in 22RV1 cells. Consistently, cell growth assay manifested greatly reduced 22RV1 cell proliferation following FoxA1 knockdown (Fig. 2B). Next, we tried to understand whether this role of FoxA1 is dependent on the AR function. To test this, we analyzed two AR-negative prostate cancer cell lines, PC-3M and DU145. As these cell lines are known to express very low levels of endogenous FoxA1, we decided to overexpress FoxA1 in these cells. Interestingly, ectopic FoxA1 overexpression showed no effects on DU145 and PC-3M cell growth. This strongly suggests that FoxA1 regulation of cell growth is mediated by and dependent on the AR pathway.
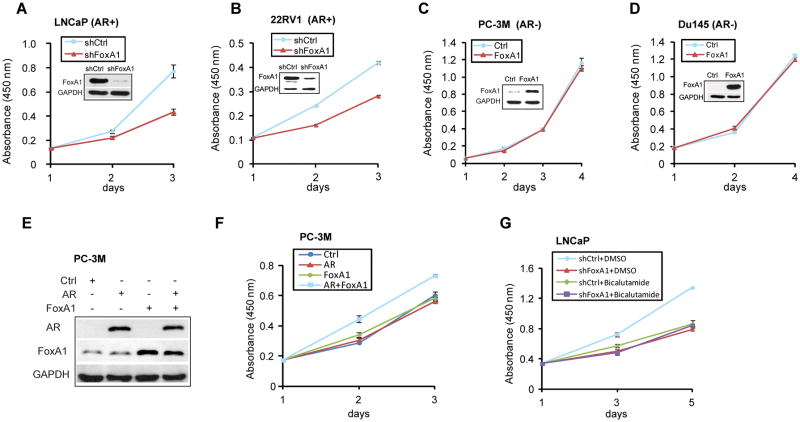
A–B, WST-1 cell growth assay of AR-positive LNCaP (A) and 22RV1 (B) control (shCtrl) and shRNA-treated (shFoxA1) cells. Data shown are the mean of independent replicates +/− SEM. FoxA1 protein knockdown was confirmed by western blot (inset). C–D, AR-negative PC-3M (C) and DU145 (D) cells were subjected to control and FoxA1 overexpression. Total protein was blotted (inset) and cell growth assayed. Data shown are the mean of independent replicates +/− SEM. E–F, PC-3M cells were transfected with control, FoxA1- or AR-expressing vector, or both and stable cell lines were established. AR and FoxA1 protein were determined by immunoblotting (E) and cell growth by WST-1 assay (F). G, Control (shCtrl) and FoxA1-knockdown (shFoxA1) LNCaP cells were treated with vehicle or 10nM anti-androgen bicalutamide. Total cell growth was measured by WST-1 assay.
We next examined whether the expression of AR might sensitize PC-3M to FoxA1 regulation. To do this, we overexpressed AR, FoxA1, or both in the PC-3M cells and carried out cell growth assays. Remarkably, we found that FoxA1 overexpression indeed significantly increased the growth of AR-expressing PC-3M cells but not that of the control cells (Fig. 2E–F and Supplementary Fig. S2B). QRT-PCR analysis confirmed induced expression of known AR target genes such as PSA and TMPRSS2 by ectopic AR and FoxA1 co-expression supporting the activation of AR signaling pathway (Supplementary Fig. S2C). On the other hand, we investigated whether the blockade of AR signaling in LNCaP cells might attenuate the ability of FoxA1 to regulate LNCaP cell growth. We used anti-androgen bicalutamide to block AR signaling in LNCaP cells, which led to reduced cell growth as expected. Importantly, in the presence of anti-androgen, FoxA1 knockdown no longer has a significant effect on LNCaP cell growth (Fig. 2G). Collectively, our data support that FoxA1 promotes PCa cell growth through the AR pathway and that this tumor-promoting role can be efficiently targeted by anti-androgens.
FoxA1 inhibits cell invasion independently of AR
Next we investigated the potential function of FoxA1 in inhibiting cell motility and EMT as suggested by GO analysis (Fig. 1C). To do this, we monitored cell invasion through matrigel in Boyden chamber assay and cell migration using wound-healing assay. We first examined the effect of FoxA1 overexpression on the FoxA1-low but highly invasive DU145 cells. Remarkably, our data showed a drastic reduction in the number of invaded cells upon FoxA1 overexpression (Fig. 3A). In concordance with this, wound-healing assays over a time-course revealed substantially delayed cell migration in FoxA1-expressing DU145 cells (Fig. 3B). These inhibitory effects of FoxA1 on cell migration and invasion were also observed in another independent, FoxA1-low, cell line PC-3M (Fig. 3C–D and Supplementary Fig. S3A). Expression microarray profiling confirmed at the molecular level that FoxA1 overexpression inhibits genes involved in cell motility (Supplementary Fig. S3B–C). As both DU145 and PC-3M cells do not express AR, the anti-motility role of FoxA1 is thought independent of the AR. Next we examined how this function of FoxA1 relates to that of AR using LNCaP cells.
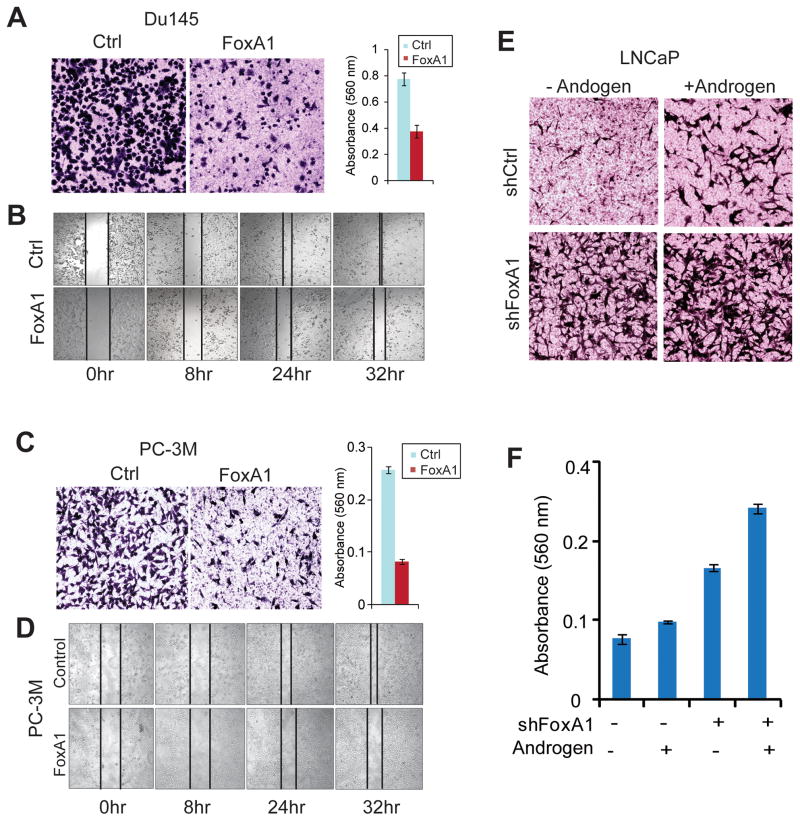
A–D, Ectopic FoxA1 overexpression decreased DU145 and PC-3M cell invasion (A, C) and migration (B, D). DU145 and PC-3M cells were infected with control and FoxA1-expressing lentivirus and subjected to antibiotic selection. Stable control and FoxA1-expressing cells were then analyzed using Boyden Chamber invasion assay for 24 hours. Invaded cells were quantified using colormetry with absorbance at 560nm (right panels of A and C). Cell migration was measured using wound-healing assay at a time-course after cell scratching. E–F, FoxA1 knockdown increases LNCaP cell invasion. Control and FoxA1-knockdown cells were established and grown in hormone-deprived or androgen-treated medium and assessed by Boyden Chamber Assay. Error Bars indicate the mean from three independent experiments +/− SEM.
Being concordant with aforementioned results, cell invasion assays demonstrated that FoxA1 knockdown in LNCaP cells resulted in dramatic increase in the number of invaded cells regardless of the AR activity, i.e., both in the presence and absence of androgen (Fig. 3E–F). Interestingly, androgen stimulation and thus AR signaling, in contrast to FoxA1, positively regulates cell invasion. In addition, FoxA1 loss and AR signaling demonstrated a synergistic effect in increasing cell invasion. Similar opposing roles of FoxA1 and AR on cell invasion were also observed in PC-3M cells with ectopic AR and/or FoxA1 overexpression (Supplementary Fig. S3D–E). Therefore, the anti-motility function of FoxA1 is independent of the AR pathway and in fact directly opposes that of AR. Since increased cell motility is closely associated with EMT and our GO analysis has revealed a link between FoxA1-repressed genes with EMT (Fig. 1C), we next sought to examine whether FoxA1 might inhibit EMT.
FoxA1 suppresses epithelial-to-mesenchymal transition
To delineate the potential role of FoxA1 in regulating EMT, we first measured representative EMT markers in a panel of FoxA1-positive and -negative prostate cancer cells. Immunoblot analysis revealed that E-cadherin (E-Cad) is highly expressed in FoxA1-positive PCa cells including LNCaP, whereas vimentin (VIM) is significantly up-regulated in the FoxA1-negative, highly aggressive cell lines such as PC-3M and DU145 cells (Fig. 4A). FoxA1 expression in these PCa cell line panel is positively associated with the epithelial marker but negatively associated with the mesenchymal marker, supporting its potential role in inhibiting EMT. To investigate this, we examined the morphologic changes of cells with FoxA1 dys-regulation. We found that FoxA1 knockdown LNCaP cells appeared more dispersed and demonstrated an astrocyte-like, fusiform, or fibroblastic phenotype (Fig. 4B). Concomitant with this mesenchymal phenotypic change, there is a remarkable increase in VIM expression (Fig. 4C–D, Supplementary Fig. S4). We next investigated whether FoxA1 overexpression is able to reverse the highly invasive PC-3M cells that have a spindle-shaped fibroblast-like appearance typical of mesenchymal cells. Analysis of the cell morphology revealed an evident shift to a more rounded epithelial-like phenotype, indicating reversal of EMT by FoxA1 overexpression (Fig. 4E). In addition, FoxA1-expressing cells tend to grow in clusters, a phenotype characteristic of epithelial cells that maintain complete cell-to-cell contact. Consistent with these phenotypic changes, the cell contact protein E-Cad is remarkably up-regulated upon FoxA1 overexpression (Fig. 4F–G). With the role of FoxA1 in cell motility and EMT established, we next attempted to pinpoint the critical downstream genes or molecular pathways that mediate this newly identified function of FoxA1 in cell motility and EMT.
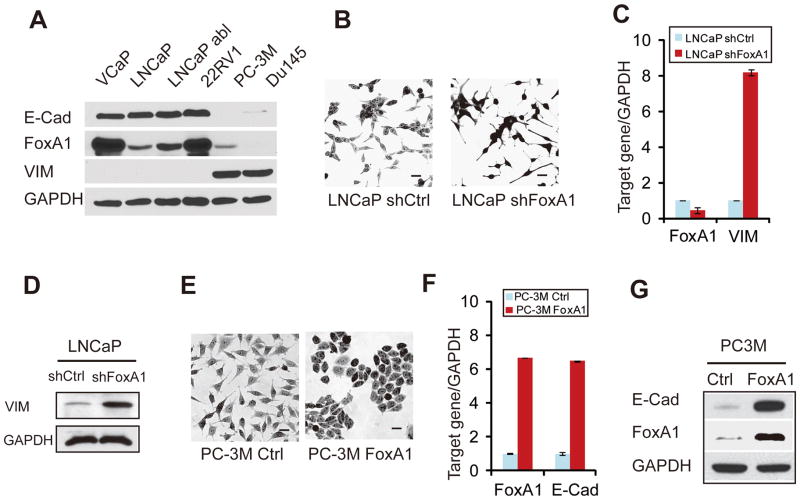
A, Western blot analysis of E-Cad, FoxA1 and VIM in a panel of PCa cell lines. B, Phase-contrast microscopy images of control vs. FoxA1-knockdown LNCaP cells. Scale bars represent 50 μm. C, qRT-PCR showing increased VIM expression in FoxA1-knockdown LNCaP cells. D. Western blot of VIM protein following FoxA1 knockdown. LNCaP cell lysates were first immunoprecipitated with a mouse anti-VIM antibody and then detected using a rabbit anti-VIM antibody. Total cell lysate (bottom) was immunoblotted by anti-GAPDH as a loading control. E, Phase-contrast microscopy images of control vs. PC-3M cells with ectopic FoxA1 overexpression. Scale bars represent 50 μm. F–G, Induced levels of E-Cad transcript and protein in FoxA1-expressing PC-3M cells.
SLUG is a critical mediator of the anti-motility function of FoxA1
We hypothesized that FoxA1 may directly regulate the expression of a critical mediator of EMT. To identify such genes, we performed integrative analysis of FoxA1 ChIP-Seq data and expression microarray data that compares control and FoxA1-knockdown LNCaP cells (Supplementary Fig. S1G). We found SLUG, a transcription factor essential for EMT (16), as a candidate direct target of FoxA1. ChIP-Seq revealed a strong FoxA1 binding event within the intragenic region of the SLUG gene. This binding is markedly reduced upon FoxA1 knockdown (Fig. 5A). Using primers that flank this binding site, we confirmed using ChIP-PCR that FoxA1 indeed binds to this intragenic enhancer (Fig. 5B) and that this binding is reduced in FoxA1-knockdown cells (Fig. 5C). This binding event was further validated in an additional cell line model VCaP cells (Supplementary Fig. S5A). We next investigated whether FoxA1 indeed regulates SLUG transcription.
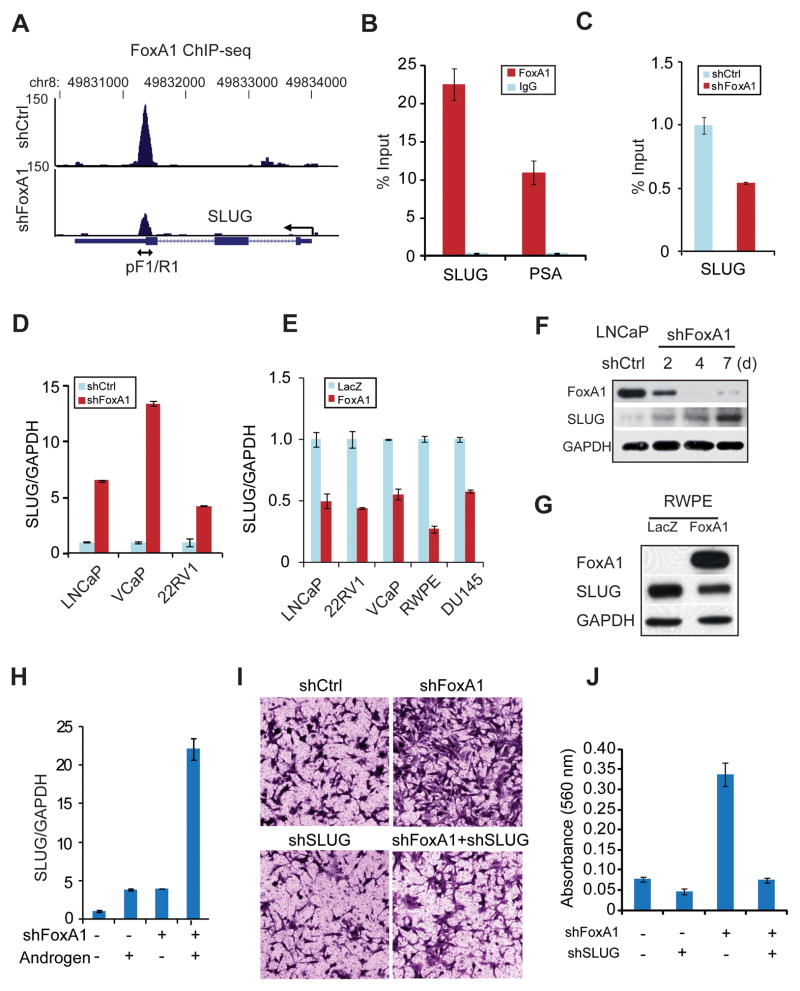
A, FoxA1 ChIP-Seq in the control and FoxA1-knockdown LNCaP cells around the SLUG gene. A major binding event locates at a downstream enhancer within the intragenic region, around which the primers SLUG pF1 and pR1 were designed for ChIP-PCR in B-C and Supplementary Figure S5A. B. FoxA1 ChIP-PCR in LNCaP cells. IgG was used as a control antibody and PSA as a positive control gene. C. FoxA1 binding was validated by ChIP-PCR in control and FoxA1-knockdown LNCaP cell. D, LNCaP, VCaP and 22RV1 cells with stable FoxA1 knockdown were established. SLUG gene expression was assessed by qRT-PCR. E, qRT-PCR analysis of SLUG gene expression in LNCaP, 22RV1, VCaP, RWPE and DU145 cells with control or FoxA1 overexpression. F, LNCaP cells were transfected with control- or FoxA1-targeting shRNA lentivirus and selected for stable cells. Cell lysates were collected over a 7-day period and total protein was immunoblotted. G, Protein levels of FoxA1 and SLUG in control vs. FoxA1-expressing RWPE cells. H, qRT-PCR analysis of SLUG gene expression in control and FoxA1-knockdown LNCaP cells grown in the presence or absence of androgen. I–J, SLUG knockdown reversed shFoxA1-induced cell invasion. Cell invasion was assessed by Boyden Chamber Assay (I) and quantified using colorimetry (absorbance at 560nm) (J).
To examine whether FoxA1 regulates SLUG gene expression, we performed FoxA1 knockdown in a panel of FoxA1-expressing PCa cell lines including LNCaP, VCaP, and 22RV1 (Supplementary Fig. S5B). QRT-PCR analysis revealed that SLUG transcript level is remarkably increased following FoxA1 knockdown, suggesting that FoxA1 inhibits SLUG expression (Fig. 5D). On the other hand, ectopic FoxA1 overexpression in a wide-range of PCa cell lines robustly suppressed SLUG gene expression regardless of the AR context (Fig. 5E, Supplementary Fig. S5C). To validate this regulation at the protein level, we carried out immunoblot analysis of LNCaP cells that have been selected for stable FoxA1 knockdown over a time-course. Our data confirmed a gradual reduction of FoxA1 protein following colony selection of knockdown cells. Importantly, SLUG protein expression steadily increased following the decrease of FoxA1 (Fig. 5F). Being consistent with this, ectopic FoxA1 overexpression, on the contrary, significantly reduced SLUG protein level (Fig. 5G).
Therefore, FoxA1 directly binds to the SLUG enhancer to inhibit its expression and this transcriptional regulation is AR-independent. In fact, we found that androgen treatment stimulates SLUG expression, demonstrating a role that is directly opposite to that of FoxA1 (Fig. 5H). FoxA1 loss and AR signaling showed a synergistic effect in drastically up-regulating SLUG expression for more than 20 fold. Similarly, we confirmed in PC-3M and DU135 cells that FoxA1 and AR overexpression regulate SLUG in opposite direction, while both increase AR-induced genes such as PSA and TMPRSS2 (Supplementary Fig. S5D–E & S2C–D). These results are consistent with our earlier observation showing their distinct functions in cell invasion but similar roles in cell growth. These data strongly suggest that SLUG may be a critical mediator of FoxA1 regulation of cell motility. To assess this, we used shRNA to block SLUG up-regulation in FoxA1-knockdown LNCaP cells (Supplementary Fig. S5F–G). Cell invasion assays revealed that while FoxA1 knockdown dramatically induced the invasiveness of LNCaP cells, this increase was blocked by SLUG knockdown (Fig. 5I–J). It is thus clear that FoxA1 plays an important anti-motility role that is mediated by its inhibition of SLUG expression. As cell migration and invasion in vitro is tightly associated with tumor metastasis in vivo, we next investigated whether FoxA1 might inhibit xenograft prostate tumor metastasis in mice.
FoxA1 inhibits prostate cancer metastasis
As we have demonstrated that FoxA1 inhibits cell motility and SLUG expression in a diverse prostate cell lines regardless of the AR status, we chose to examine this role in vivo using one representative cell line. PC-3M cell line was selected as it is AR- (thus ideal for studying AR-independent function of FoxA1) and that its orthotopic implantation has been proven a valuable metastatic model for human prostate cancer (32). In order to trace tumors in live animals, a luciferase reporter was transfected into PC-3M cells through lentiviral infection followed by antibiotic selection of stable clones. These luciferase-expressing control and FoxA1-epxressing cells were then inoculated into the mice prostate and monitored through live mice imaging using an IVIS system (Fig. 6A). We observed xenograft tumor formation in both control and FoxA1 mice at approximately 2 weeks after implantation. There were no significant differences in tumor size between the two groups for up to 4 weeks after implantation. These findings suggest that FoxA1 overexpression does not affect PC-3M cell growth in vivo. The mice with FoxA1-expressing cells eventually developed significantly smaller regional tumors at 5 weeks after implantation (Fig. 6B). These differences in tumor size, however, are most likely due to the merge of regionally metastatic tumors in the control mice, as at 5 weeks post-inoculation the control mice had massive metastasis and had to be euthanized. Strikingly, the mice with FoxA1-expressing cells had significantly less tumor metastasis (Fig. 6C). Out of the 5 mice, only 1 mouse showed distant metastasis, while the control group had 100% metastasis. Having demonstrated the role of FoxA1 in inhibiting cell motility in vitro and xenograft tumor metastasis in vivo, we next examined its expression in human PCa.
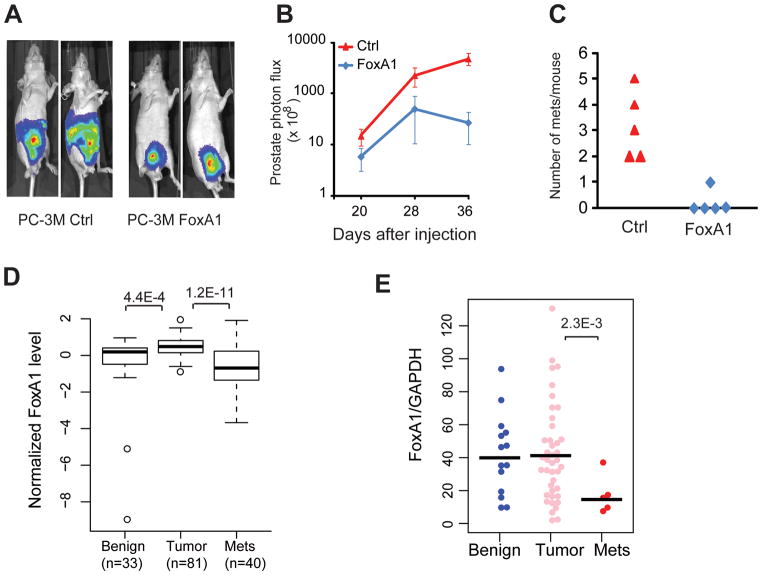
A–C, FoxA1 overexpression inhibits orthotopic tumor growth and metastasis in mice. Nude mice were injected orthotopically with luciferase-labeled control and FoxA1-expressing PC-3M cells. Xenogen images of two representative mice per group are shown in A. Tumor growth (B) and metastasis (C) were monitored through living mice imaging using an IVIS Spectrum Bioluminescence System. D, FoxA1 mRNA level in a microarray dataset profiling 33 benign prostate, 81 localized prostate tumor and 40 metastatic PCa (22). Significant P-values are shown. E, qRT-PCR analysis of FoxA1 mRNA in a panel of human prostate tissues. Significant P-value was shown.
We first examined FoxA1 expression in a recent microarray dataset profiling over 150 human PCa tissues (22). Interestingly, we found that FoxA1 is marginally significantly (P = 4.4E-4) up-regulated in localized PCa when compared to benign prostate samples (Fig. 6D). Remarkably, FoxA1 is highly significantly (P = 1.2E-11) down-regulated in metastatic PCa relative to both benign and localized PCa. This interesting pattern of stage-dependent dys-regulation of FoxA1 is validated in another independent microarray dataset with a large sample size (Supplementary Fig. S6A) (33; 34). To further corroborate this finding, we carried out qRT-PCR and western blot analysis of FoxA1 expression in a panel of benign adjacent prostate, localized and metastatic PCa tissues. Our results confirmed that FoxA1 is indeed slightly up-regulated in localized PCa relative to benign tissues, but is dramatically down-regulated in metastatic PCa (Fig. 6E, Supplementary Fig. S6B–C). This disease stage-dependent expression pattern of FoxA1 precisely reflects the dual roles of FoxA1 in positively regulating cell proliferation, a cellular process that dominates primary tumors, but in negatively regulating cell motility and EMT, a process essential to tumor metastasis. We next examined in this context the functionalities of recurrent FoxA1 mutations recently reported in human PCa.
Mutant FoxA1 failed to inhibit SLUG expression and cell invasion
To investigate how FoxA1 mutations contribute to PCa progression, we generated two representative FoxA1 mutants, F400I and M253K that contain a point mutation in the transactivation and the forkhead domain, respectively. Lentivirus carrying wildtype or mutant FoxA1 genes were used to infect DU145 cells to generate stable cell lines that express either wildtype or mutant FoxA1. QRT-PCR using primers that amplify the wildtype regions confirmed FoxA1 overexpression in all three stable cell lines (Fig. 7A), while immunoblot analysis confirmed ectopic FoxA1 protein overexpression (Fig. 7B).
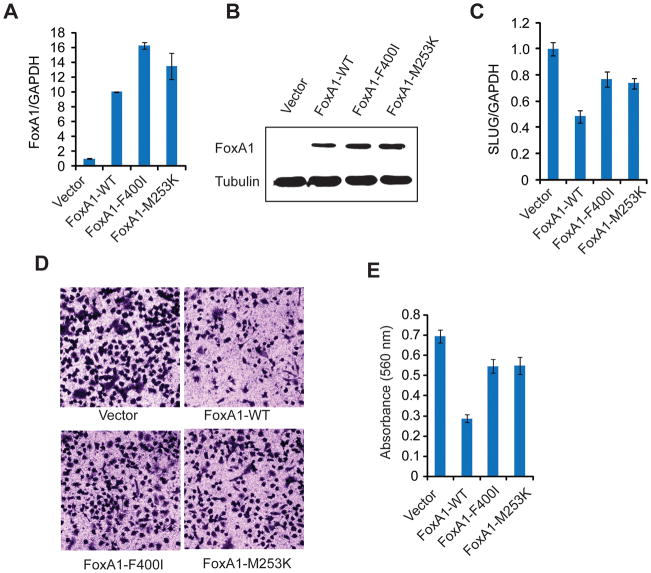
A–B, Wild type (WT) FoxA1, FoxA1 F400I and FoxA1 M253K were stably expressed in DU145 cells. Stable cells were generated using a different lentivirus construct or empty vector (as a control). QRT-PCR(A) and western blotting (B) confirmed FoxA1 expression. C, Wild type FoxA1 overexpression significantly inhibited SLUG expression. FoxA1 mutations resulted in significantly recovered SLUG expression. D–E, Cell invasion was assessed by Boyden Chamber Assay (D) and quantified using colorimetry (absorbance at 560nm) (E).
With the stable cell lines established, we next examined the potential impact of the mutations on FoxA1 function. Using qRT-PCR, we demonstrated that the ability of the mutant FoxA1 to inhibit SLUG gene expression is substantially attenuated (Fig. 6C). With similar levels of overexpression, the wildtype FoxA1 suppressed SLUG expression to a level significantly lower than that by the mutants. In concordance with this, cell invasion assays revealed that while wildtype FoxA1 substantially inhibited DU145 cell invasion, the mutants only showed moderate inhibitory effects (Fig. 7D–E). Mechanistically, this attenuated function may be due to reduced binding of FoxA1 mutants on the SLUG gene enhancer as supported by ChIP-PCR, despite their unaffected localization to the nuclei (Supplementary Fig. S7A–B). Therefore, one mechanism by which recurrent FoxA1 mutations contribute to PCa progression is through tempering its ability to inhibit SLUG gene expression and thus cell motility.
DISCUSSION
Understanding of FoxA1 function in PCa to date is largely limited to its regulation of AR activities. In this study, we revealed that, in addition to regulating cell cycle, FoxA1 also controls the expression of genes involved in cell motility and EMT. Importantly, this function of FoxA1 is distinct from that of AR and is independent of AR activation. Depending on the cellular context, either oncogenic or tumor suppressive function of FoxA1 may dominate. Indeed, using two large tumor expression profiling dataset we revealed that, during prostate cancer progression from benign tissue to localized PCa, and then to metastatic PCa, FoxA1 expression is firstly up-regulated (in localized PCa), and then dramatically down-regulated (in metastatic PCa). The initial up-regulation of FoxA1 may have been critical for original malignant cells to rapidly proliferate. However, the loss of FoxA1 at late-stage disease may be associated with tumor metastasis. Consistently, our xenograft studies showed FoxA1 inhibition of PCa tumor metastasis in vivo. Our discovery of this new anti-motility function of FoxA1 nicely reconciles reported discrepancy in studies associating FoxA1 expression with both good and bad prognosis. However, it needs to be noted that for these findings to be fully validated, future studies need to be done to determine FoxA1 protein level in larger cohorts of benign, cancerous and metastatic PCa tissues by western blot and/or tissue microarray analysis. Such analysis needs to be carefully carried out and interpreted considering AR status and disease stages, due to the dual roles of FoxA1 in inducing cell growth but inhibiting cell motility. It will also be useful in future studies to further dissect out the effects of FoxA1 on tumor growth and metastasis in vivo using AR+ cell lines with tetracycline-inducible FoxA1 knockdown.
Our findings of FoxA1 in inhibiting cell motility and EMT in cancer are also consistent with the role of FoxA1 during development in promoting cell differentiation. However, it is important to note that PCa progression is a complex process regulated by a large number of tumor suppressors and oncogenes. FoxA1, being one of them, may not be the whole story. For example, AR overexpression accounts for over 30% of metastatic PCa wherein FoxA1 might not be down-regulated. However, PCa cell lines that are driven by FoxA1 loss or mutation, if could be identified, will be very useful models for further characterization of the functions of wildtype and mutant FoxA1 and for the development of targeted therapy.
We pinpoint SLUG as key downstream gene that is critical for the new role of FoxA1 in inhibiting cell motility. Using FoxA1 overexpression and knockdown assays we provide strong evidence that FoxA1 inhibits SLUG gene expression at both the mRNA and protein levels. ChIP-Seq data revealed multiple FoxA1 binding events within the regulatory elements around SLUG, which was further confirmed by ChIP-PCR, suggesting direct regulation. However, as SLUG is a tightly regulated gene, it is very likely that additional pathways may exist and that SLUG may also be indirectly regulated by FoxA1. On the other hand, although previous studies have largely focused on FoxA1 as a transcriptional activator and a cofactor of the AR, recent studies have begun to show that AR can act directly as a transcriptional repressor (31; 36). Moreover, FoxA1 itself has been shown to function as a transcriptional repressor that is directly recruited to the RPRM promoter, interacts with HDAC7, and is necessary for estrogen-mediated repression of RPRM (37). Future studies are warranted to further examine the mechanism by which FoxA1 directly inhibits target genes such as SLUG. Moreover, our study showed that the loss of FoxA1 works in synergy with AR in inducing SLUG expression and increasing cell motility. This suggests that PCa with FoxA1 loss and AR gain may be especially dangerous. This is further supported by a recent study reporting SLUG also acting as an AR co-factor that further enhances AR signaling (38).
In this study we demonstrate evidence that while the wildtype FoxA1 drastically inhibits cell invasion, this tumor suppressive effect is greatly impaired by FoxA1 mutations that were found in patients. By contrast, no significant differences were observed in terms of their ability in regulating cell growth as recently reported (22). In addition, we show that the effect of FoxA1 in promoting cell growth is largely dependent on the AR and can be efficiently targeted by anti-androgens, which are standard first-line treatment for PCa. Therefore, instead of designing additional approaches to target FoxA1-mediated cell growth, it may be more beneficial to design therapeutic approaches to restore its favorable effect in suppressing metastasis, in combination with anti-androgen treatment. In addition, we showed SLUG as a key mediator of FoxA1 regulation of cell motility, thus providing a potential avenue for targeting the molecular subtype of prostate cancers that harbor FoxA1 mutation or loss.
In summary, this study represents the beginning of research endeavors to separate the intrinsic roles of FoxA1 from its secondary effects mediated via the AR. This study is the first to clearly demonstrate an important anti-motility function of FoxA1 in PCa that is independent of the AR. These results lead to further discovery of a critical mechanism by which recurrent FoxA1 mutations contribute to PCa progression. It is plausible that other pathways may also be involved, which will be interesting lines for future studies. In particular, it would be intriguing to determine in future studies whether the DNA binding profiles of the mutant FoxA1 are different from that of the wildtype and how they might alter the AR pathway. Taken together, our study provides a mechanistic link between recurrent FoxA1 mutations and PCa. By pinpointing the key molecular pathways involved, our findings offer unique opportunities for novel therapeutics to treat the molecular subtype of prostate cancers that harbor FoxA1 mutation or loss.
Acknowledgments
This work was supported by funding from the NIH P50CA090386 pilot project (to J.Y.), U54CA143869 pilot project (to J.Y.), K99/R00CA129565 (to J.Y.), the U.S. Department of Defense W81XWH-09-1-0193(to J.Y.), and the Research Scholar Award RSG-12-085-01 (to J.Y.) from the American Cancer Society.
Footnotes
Disclosure of Potential Conflicts of Interest
No potential conflicts of interest were disclosed.
References
Full text links
Read article at publisher's site: https://doi.org/10.1158/0008-5472.can-12-3468
Read article for free, from open access legal sources, via Unpaywall:
https://cancerres.aacrjournals.org/content/canres/73/12/3725.full.pdf
Citations & impact
Impact metrics
Citations of article over time
Alternative metrics
Article citations
FOXA1, induced by RC48, regulates HER2 transcription to enhance the tumorigenic capacity of lung cancer through PI3K/AKT pathway.
J Cancer, 15(18):5863-5875, 16 Sep 2024
Cited by: 0 articles | PMID: 39440051 | PMCID: PMC11493013
Polygenic risk score predicting susceptibility and outcome of benign prostatic hyperplasia in the Han Chinese.
Hum Genomics, 18(1):49, 22 May 2024
Cited by: 0 articles | PMID: 38778357 | PMCID: PMC11110300
Clinically-observed FOXA1 mutations upregulate SEMA3C through transcriptional derepression in prostate cancer.
Sci Rep, 14(1):7082, 25 Mar 2024
Cited by: 1 article | PMID: 38528115 | PMCID: PMC10963789
Transcription factors direct epigenetic reprogramming at specific loci in human cancers.
Front Genet, 14:1234515, 09 Oct 2023
Cited by: 0 articles | PMID: 37876590 | PMCID: PMC10591108
Development of gemcitabine-modified miRNA mimics as cancer therapeutics for pancreatic ductal adenocarcinoma.
Mol Ther Oncol, 32(1):200769, 26 Jan 2024
Cited by: 1 article | PMID: 38596306 | PMCID: PMC10869788
Go to all (97) article citations
Data
Data behind the article
This data has been text mined from the article, or deposited into data resources.
BioStudies: supplemental material and supporting data
GEO - Gene Expression Omnibus
- (1 citation) GEO - GSE37314
Similar Articles
To arrive at the top five similar articles we use a word-weighted algorithm to compare words from the Title and Abstract of each citation.
FOXA1 regulates androgen receptor variant activity in models of castrate-resistant prostate cancer.
Oncotarget, 6(30):29782-29794, 01 Oct 2015
Cited by: 28 articles | PMID: 26336819 | PMCID: PMC4745762
Pioneer of prostate cancer: past, present and the future of FOXA1.
Protein Cell, 12(1):29-38, 18 Sep 2020
Cited by: 68 articles | PMID: 32946061 | PMCID: PMC7815845
Review Free full text in Europe PMC
FOXA1 modulates EAF2 regulation of AR transcriptional activity, cell proliferation, and migration in prostate cancer cells.
Prostate, 75(9):976-987, 23 Mar 2015
Cited by: 19 articles | PMID: 25808853 | PMCID: PMC4424106
FOXA1 promotes tumor progression in prostate cancer via the insulin-like growth factor binding protein 3 pathway.
PLoS One, 7(8):e42456, 03 Aug 2012
Cited by: 43 articles | PMID: 22879989 | PMCID: PMC3411739
Funding
Funders who supported this work.
NCI NIH HHS (6)
Grant ID: P50 CA090386
Grant ID: K99/R00CA129565
Grant ID: P50CA090386
Grant ID: U54 CA143869
Grant ID: R00 CA129565
Grant ID: U54CA143869