Abstract
Background
ELL-associated factor 2 (EAF2) is an androgen-regulated tumor suppressor in the prostate. However, the mechanisms underlying tumor suppressive function of EAF2 are still largely unknown. Identification of factors capable of modulating EAF2 function will help elucidate the mechanisms underlying EAF2 tumor suppressive function.Methods
Using eaf-1(the ortholog of EAF2) mutant C. elegans model, RNAi screen was used to identify factors on the basis of their knockdown to synergistically enhance the reduced fertility phenotype of the eaf-1 mutant C. elegans. In human cells, the interaction of EAF2 with FOXA1 and the effect of EAF2 on the FOXA1 protein levels were determined by co-immunoprecipitation and protein stability assay. The effect of EAF2 and/or FOXA1 knockdown on the expression of AR-target genes was determined by real-time RT-PCR and luciferase reporter assays. The effect of EAF2 and/or FOXA1 knockdown on LNCaP human prostate cancer cell proliferation and migration was tested using BrdU assay and transwell migration assay.Results
RNAi screen identified pha-4, the C. elegans ortholog of mammalian FOXA1, on the basis of its knockdown to synergistically enhance the reduced fertility phenotype of the eaf-1 mutant C. elegans causing sterility. EAF2 co-immunoprecipitated with FOXA1. EAF2 knockdown enhanced endogenous FOXA1 protein level, whereas transfected GFP-EAF2 down-regulated the FOXA1 protein. Also, EAF2 knockdown enhanced the expression of AR-target genes, cell proliferation, and migration in LNCaP cells. However, FOXA1 knockdown inhibited the effect of EAF2 knockdown on AR-target gene expression, cell proliferation, and migration in LNCaP cells, suggesting that FOXA1 can modulate EAF2 regulation of AR transcriptional activation, cell proliferation, and migration.Conclusions
These findings suggest that regulation of the AR signaling pathway, cell proliferation, and migration through FOXA1 represents an important mechanism of EAF2 suppression of prostate carcinogenesis.Free full text

FOXA1 modulates EAF2 regulation of AR transcriptional activity, cell proliferation and migration in prostate cancer cells
Abstract
BACKGROUND
ELL-associated factor 2 (EAF2) is an androgen-regulated tumor suppressor in the prostate. However, the mechanisms underlying tumor suppressive function of EAF2 are still largely unknown. Identification of factors capable of modulating EAF2 function will help elucidate the mechanisms underlying EAF2 tumor suppressive function.
METHODS
Using eaf-1(the ortholog of EAF2) mutant C. elegans model, RNAi screen was used to identify factors on the basis of their knockdown to synergistically enhance the reduced fertility phenotype of the eaf-1 mutant C. elegans. In human cells, the interaction of EAF2 with FOXA1 and the effect of EAF2 on the FOXA1 protein levels were determined by co-immunoprecipitation and protein stability assay. The effect of EAF2 and/or FOXA1 knockdown on the expression of AR-target genes was determined by real-time RT-PCR and luciferase reporter assays. The effect of EAF2 and/or FOXA1 knockdown on LNCaP human prostate cancer cell proliferation and migration was tested using BrdU assay and transwell migration assay.
RESULTS
RNAi screen identified pha-4, the C. elegans ortholog of mammalian FOXA1, on the basis of its knockdown to synergistically enhance the reduced fertility phenotype of the eaf-1 mutant C. elegans causing sterility. EAF2 co-immunoprecipitated with FOXA1. EAF2 knockdown enhanced endogenous FOXA1 protein level, whereas transfected GFP-EAF2 down-regulated the FOXA1 protein. Also, EAF2 knockdown enhanced the expression of AR-target genes, cell proliferation, and migration in LNCaP cells. However, FOXA1 knockdown inhibited the effect of EAF2 knockdown on AR-target gene expression, cell proliferation and migration in LNCaP cells, suggesting that FOXA1 can modulate EAF2 regulation of AR transcriptional activation, cell proliferation and migration.
CONCLUSIONS
These findings suggest that regulation of the AR signaling pathway, cell proliferation, and migration through FOXA1 represents an important mechanism of EAF2 suppression of prostate carcinogenesis.
INTRODUCTION
Prostate cancer is the most commonly diagnosed cancer and the second leading cause of cancer-related death in men in the United States (1). It is estimated that in 2014 there will be nearly 233,000 new cases of prostate cancer and nearly 29,480 men will die of the disease in the United States (http://www.cancer.gov/cancertopics/types/prostate). Although significant advances have been made in understanding the mechanisms of prostate carcinogenesis as well as improving its diagnosis and treatment, the disease is still a major cause of mortality and morbidity. Increased understanding of the mechanisms underlying the initiation and progression of prostate cancer remains a matter of urgency.
EAF2 (ELL-associated factor 2), encoded by an androgen induced gene (2), was identified as a tumor suppressor in our previous studies (3). EAF2 knockout mice developed lung adenocarcinoma, hepatocellular carcinoma, B-cell lymphoma and prostatic intraepithelial neoplasia (4). Consistent with its tumor suppressor function, EAF2 is down-regulated in prostate cancer compared to benign prostate tissue (3,5). Expression of EAF2 in xenograft prostate tumors markedly induced apoptosis and inhibited tumor growth in vivo (3). In recent years, several studies were conducted to elucidate the mechanisms underlying EAF2 tumor suppressive function. EAF2 deletion could induce up-regulation of RAS cascade genes and resulted in elevated p-ERK levels in the mouse prostate model (6). In addition, through binding to the different partners, EAF2 could also regulate the activity of several signaling pathways. EAF2 could bind to β-catenin to inhibit canonical Wnt/β-catenin signaling (7). Through binding to HIF-1α, EAF2 modulates the hypoxia-signaling pathway and thereby protects cells against hypoxia-induced cell death (8). Furthermore, EAF2 could inhibit angiogenesis through interaction with pVHL (9,10) and regulate the expression of TSP-1 through blocking p53 repression of the TSP-1 promoter (11). However, the mechanisms underlying tumor suppression function of EAF2 are still largely unknown. Identification of other factors capable of modulating EAF2 activity should help us gain more insight into the mechanisms of its action.
To identify potential functional partners of EAF2, we performed an RNAi screen using eaf-1(ortholog of EAF2 (12)) mutant C. elegans model. Through analyzing the difference of the effect on fertility between different treatment groups, we identified pha-4 as a synergistic enhancer of eaf-1’s effect on C. elegans fertility. In human, the FOXA family is the ortholog of C. elegans of pha-4 (13).
FOXA1, also known as HNF-3, is a member of the FOXA transcription factor family (14). Over the past years, FOXA1 has emerged as an important player in the progression of prostate cancer. FOXA1 could bind to compact chromatin to make the chromatin more accessible to AR to bind, functioning as a pioneer factor (15,16). Physically interacting with AR and thereby acting as a key member in AR transcription complex, FOXA1 can regulate the transcriptional activity of AR (16,17). Recent studies indicate that changes in FOXA1 expression level could result in redistribution of AR transcriptional program (18–20). Due to the important role of FOXA1 in regulation of the AR transcriptional program, some other factors, such as NKX3-1 or NF1, could modulate the AR transcriptional activity through interaction with FOXA1 (21,22).
In the present study, we identified the FOXA1 ortholog pha-4 as a functional partner of the EAF2 ortholog eaf-1 in the C. elegans model and subsequently showed that FOXA1 can physically interact with EAF2 and modulate EAF2 suppressed regulation of AR transcriptional activity, cell proliferation and migration in LNCaP human prostate cancer cells. Our work has uncovered a novel role for EAF2 in regulating the AR signaling pathway and suggested that the tumor suppressive function of EAF2 is in part mediated through FOXA1.
Materials and methods
Plasmids
CMV-Myc and pEGFP-N3 vectors were purchased from Clontech and pCMV-3Tag-1A from Agilent Technologies. Human EAF2 cDNA was amplified by PCR and cloned into the pEGFP-N3, pCMV-Myc and pCMV-3Tag-1A vectors, producing the plasmids of pEGFP-EAF2, Myc-EAF2 and Flag-EAF2. EAF2 deletion mutants were generated previously by PCR and cloned into the pCMV-Myc (23). pCMV-Renilla plasmid was obtained from Promega and pPSA6.1Luc, a PSA promoter-driven luciferase reporter (24), was a generous gift from Dr. Marianne Sadar, Ph.D. (BC Cancer Agency, British Columbia, Canada). The PSA promoter is 6.1kb, located from −6kb to +12bp (24). pCMV6-FOXA1 plasmid was purchased from Origene (RC20604); pEGFP-N3-FOXA1 was generated from pCMV6-FOXA1 by PCR and subcloned into pEGFP-N3 and untagged FOXA1 were also generated from pCMV6-FOXA1 by digestion and ligation.
C. elegans strain, RNAi screening and treatment
Wild-type N2 worms were obtained from the C. elegans Genetics Center, which is supported in part by NIH funding. ALF50 (eaf-1(tm3976)) and RNAi screening were described previously (12,25). The pha-4 RNAi clone was retrieved from the Ahringer RNAi library (26), and was validated by DNA sequencing. RNAi treatment and Nomarski imaging of C. elegans was performed as previously described (12). Briefly, adult worms were treated with hypochloride solution. Eggs were collected and subsequently seeded onto the RNAi plates. After several days, we analyzed the phenotype and fertility as described previously (12).
Cell culture and transfection
LNCaP prostate cancer cells and HEK 293 cells were obtained from ATCC and cultured in RPMI or DMEM (Hyclone) medium supplemented with 10% FBS and 5% antibiotics. For overexpression experiments, cells were transfected with plasmids using PolyJet In Vitro Transfection reagent (SignaGen Laboratories) according to the manufacturer’s instructions. Forty eight hours later, cells were harvested and prepared for subsequent assays. For knockdown experiments, cells were transfected with negative control siRNA or siRNA against FOXA1 and EAF2 using lipofectamine 2000 (Invitrogen). The amount of each siRNA was 100 pmol in each well of the 6-well plate. The control siRNA was used to complement the amount in single knockdown group. Twenty four hours after transfection, the cells were treated with 1 nM R1881 for an additional 48 hours and then used for further experiments. Negative control siRNA (NC1) and a custom siRNA against EAF2 or FOXA1 were ordered from IDT (Integrated DNA Technologies, USA). The sequences of siRNA were listed as follows: siEAF2.1 is siRNA pool against EAF2 purchased from Santa Cruz (sc-62251). siEAF2.2: AAGGTTCAACTCCACCAGTAA was purchased from Qiagen (SI000375627). siEAF2: AAACAGUUACUGGUGGAGUUGAACCUU and siFOXA1: UUGUUUGCUGUUGAUUUUUUCUCUCUU were designed by ourselves.
Co-immunoprecipitation and Western blot
For exogenous co-immunoprecipitation, HEK 293 cells were transfected with indicated plasmids, cultured for 48 hours after transfection, and then lysed in RIPA lysis buffer (50mM Tris-HCL, 1% NP-40, 150mM NaCl, 1mM EDTA, 0.25% sodium deoxycholate, pH 7.8). After preclear with protein A/G plus-agarose beads (sc-2003, Santa Cruz) for 2 hours, cell lysates were added to anti-GFP, Flag or Myc antibody-conjugated agarose beads (Sigma) and rotate at 4°C for 4h. The beads were washed using lysis buffer 5 times. Immunoprecipitates and whole cell lysate (WCL) were boiled with SDS sample loading buffer for five minutes, and then subjected to Western blot analysis.
The endogenous co-immunoprecipitation was performed using nuclear extracts. After cell lysis using NE-PER Nuclear and Cytoplasmic Extraction Reagents (Thermo Scientific), 5 mg nuclear extracts were incubated with anti-FOXA1 antibody (ab-23738, abcam) or anti-IgG (Santa Cruz) as a negative control at 4°C overnight, followed by addition of protein A/G plus-agarose beads for 2 hours. The beads were washed 5 times using NP40 lysis buffer (25 mM Tris-HCL, 1% NP-40, 150 mM NaCL, 5% Glycerol, 1 mM EDTA, pH 7.4) and then boiled with SDS sample buffer for five minutes. All the samples were separated by SDS-PAGE and analyzed by Western blot.
Real-time PCR
Total RNA was extracted from LNCaP cells using TRIzol reagent (Invitrogen) according to manufacturer’s protocol. The RNA reverse transcription was carried out using a first-strand cDNA synthesis kit (Promega). Real-time PCR was performed using SYBR green mix (Thermo Scientific). The expression of indicated genes was normalized with respect to the GAPDH mRNA level. The sequences of primers used were listed below. FOXA1 forward: 5′-GAAGATGGAAGGGCATGAAA-3′, reverse: 5′-GCCTGAGTTCATGTTGCTGA-3′, EAF2 forward: AGGTGAACAGGTGACCATAACTCTGC, reverse: GGGAGTCCTGGCTGAATTCCACATTT, PSA forward: AGGCCTTCCCTGTACACCAA, reverse: GTCTTGGCCTGGTCATTTCC, TMPRSS2 forward: CTGCCAAGGTGCTTCTCATT, reverse: CTGTCACCCTGGCAAGAATC, AR forward: TGGATGGATAGCTACTCCGG, reverse: CCCAGAAGCTTCATCTCCAC, GAPDH forward: CGACCACTTTGTCAAGCTCA, reverse: AGGGGAGATTCAGTGTGGTG.
Luciferase reporter assays
LNCaP cells were plated into 12-well plates and maintained in phenol red-free RPMI containing 10% charcoal-dextran treated FBS. Twenty four hours after transfection with siEAF2 and/or siFOXA1, cells were transfected with pGL-PSA and pCMV-Renilla as an internal control. Twenty four hours later, cells were treated with or without 1 nM R1881 for another 24 hours and then harvested and lysed for luciferase assay. Luciferase activity was determined by the dual-luciferase reporter assay system (Promega). pGL-PSA luciferase activities were determined by normalization of the firefly luciferase activity with Renilla luciferase activity.
BrdU incorporation assay
Cells seeded on coverslips (Fisher, Pittsburgh, PA, US) were transfected with siEAF2 and/or siFOXA1 and then treated with R1881 at 1 nM for 48 hours. The cells were subsequently cultured in the presence of 10 μM BrdU for 6 hours and then fixed with Carnoy’s fixative (3 volume methanol and 1 volume glacial acetic acid) for 20 min at −20°C. After treatment with 2M HCl and wash with 0.1M Boric acid, cells were incubated with 3% hydrogen peroxide (H2O2) for 10 min at room temperature, followed by blocking with 10% goat serum for 1 hour. Cells were then incubated with anti-BrdU antibody (B2531, sigma) overnight at 4 °C and CY3 labeled goat anti-mouse secondary antibody (A10521, Life technologies) for 1 h at 37 °C. The nucleus was stained with SYTOX Green (S7020, Life technologies). Images were acquired using a fluorescence microscope (Nikon TE2000-U). The cells were counted one by one using Photoshop CS5 counting tool (Adobe, San Jose, California, USA) and the percentage of BrdU-positive cells was calculated using (BrdU-positive cell number/total cell number) × 100%.
Transwell Migration Assay
Cell migration assay was performed in a transwell chamber (24-well, 8μm pore size; Corning). LNCaP cells were transfected with siRNA at 100 pmol each as indicated in a 6-well plate for 48h, collected with trypsin treatment, and then placed in the top chamber with 200 μl RPMI containing 0.2% BSA (1 × 105 cells). Six hundred μl RPMI containing 10% FBS and 1 nM R1881 was placed in the bottom chamber as chemoattractants. After 36 hours, cells on the top surface of the chamber membrane (nonmigrated) were removed by gentle scraping with a cotton swab. The cells at the lower surface of chamber membranes (migrated) were stained with 0.1% crystal violet. The number of migrated cells in five random high power fields (400X) per membrane was counted under microscope.
Colony formation assay
LNCaP cells were plated into 10cm dishes. When the cells reached 80% confluence, cells were transfected with 0.83 μg pEGFP-N3, 4.17 μg CMV-HA, 4.17 μg CMV-HA-EAF2 and/or 0.83 μg pEGFP-FOXA1-N3 as indicated using PolyJet In Vitro Transfection reagent (SignaGen, Gaithersburg, MD, USA). Twenty four hours after transfection each 10 cm dish was split into three 10 cm dishes. 48 hours after transfection, 1mg/mL G418 was added to the medium to start selection. The medium should be replaced with fresh medium containing G418 every 3–4 days. After 3 weeks, the colonies were stained with a 0.5% crystal violet solution. The colonies were counted using the Adobe Photoshop CS5 counting tool (Adobe, San Jose, California, USA).
Statistical analysis
Data are presented as mean ± SEM. Statistical analyses were performed with Student’s t-test. Differences were considered statistically significant at p<0.05. *, **, and *** denote p<0.05, p<0.01, and p<0.001, respectively, when compared to the negative control group.
RESULTS
Identification of pha-4 as an eaf-1 synergic enhancer
In C. elegans, eaf-1, the ortholog of human EAF2, plays important roles in fertility, survival and cuticle morphogenesis (12). To explore the mechanisms of eaf-1 action, we conducted a targeted RNAi screen in C. elegans using RNAi libraries consisting of transcription factors, phosphatases, and chromatin-modifying factors, to identify genes which could modulate the effect of eaf-1 mutation on C. elegans fertility (25). The screen led to the identification of 5 hits, among which pha-4 is the C. elegans ortholog of human FOXA family (25). As shown in Figure 1, the eaf-1 mutation alone can reduce the F1 progeny produced, as does treatment with pha-4 RNAi alone, indicating these genes are important for the embryo survival. However, when eaf-1 mutants were treated with pha-4 RNAi, these worms became sterile. The degeneration and atrophy of the embryos were more severe in eaf-1 mutants treated with pha-4 RNAi when compared to either eaf-1 mutant or pha-4 RNAi treated wild-type worms (Figure 1B). These results suggested that in C. elegans, eaf-1 and pha-4 have a synergistic effect in the control of embryo development and growth. As a member of FOXA family, FOXA1 is a master gene governing steroid hormone signaling and also a key cofactor of AR in human prostate cancer (15). Since orthologs often, though not always, retain the same functions in evolution and interactions of the proteins are likely conserved across different species, there may be also functional interactions between EAF2 and FOXA1, the human orthologs of eaf-1 and pha-4, respectively.
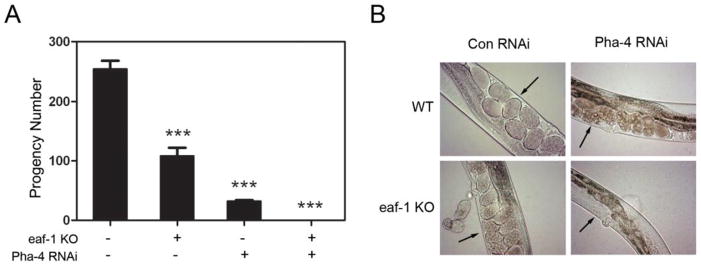
(A) The eaf-1(tm3976) mutation or pha-4 RNAi reduced the F1 progeny numbers. However, the combination of eaf-1(tm3976) mutation and pha-4 RNAi resulted in the worm sterility. (B) Developing worm embryos were present in the wild-type control, eaf-1(tm3976) knockout mutant, or pha-4 RNAi treated worms, but not in the eaf-1(tm3976) knockout mutant treated with pha-4 RNAi. Arrows point to the developing embryos. *** indicates p < 0.001.
EaF2 co-immunoprecipitates with FOXA1
To explore the association between EAF2 and FOXA1, we first performed coimmunoprecipitation to determine whether the two proteins interact. HEK 293 cells were transfected with GFP-FOXA1 and Flag-EAF2 separately or in combination. GFP-FOXA1 and FLAG-EAF2 were precipitated using the anti-GFP and anti-Flag antibody-conjugated agarose beads, respectively. As shown in Figure 2A, precipitation of GFP-FOXA1 pulled down FLAG-EAF2, and vice versa, indicating that EAF2 and FOXA1 could interact with each other. Furthermore, we performed coimmunoprecipitation of endogenous FOXA1 and EAF2 using anti-FOXA1 antibody in LNCaP cells. Consistent with the results of transfected proteins, endogenous EAF2 could be pulled down using anti-FOXA1 antibody (Figure 2B). These results suggested EAF2 and FOXA1 could interact with each other.
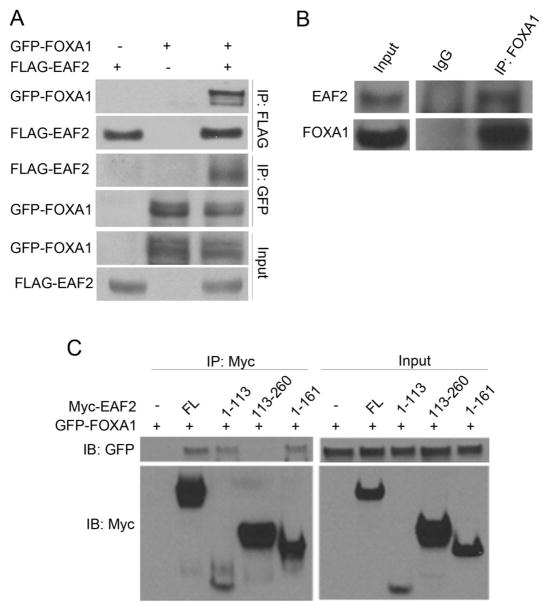
(A) 2.5 μg FLAG-EAF2 and 2.5 μg GFP-FOXA1 were transfected into HEK 293 cells separately or in combination, and then whole cell lysates were prepared for co-immunoprecipitation with anti-GFP or anti-FLAG antibody-conjugated agarose beads. FLAG-EAF2 and GFP-FOXA1 could be pulled down by each other. (B) Co-immunoprecipitation of endogenous EAF2 and FOXA1 was performed using nuclear extracts prepared from LNCaP cells with rabbit anti-FOXA1 antibody or control rabbit IgG. Co-precipitated EAF2 was detected by Western blot using a monoclonal mouse anti-EAF2 antibody. (C) 2.5 μg Myc-tagged full-length EAF2, EAF2 deletion mutants 5 μg Myc-EAF2 (amino acids (aa) 1-113), 5 μg Myc-EAF2 (aa 1-161), or 2.5 μg Myc-EAF2 (aa 113-260) were co-transfected with 2.5 μg GFP-FOXA1 into HEK 293 cells. Co-immunoprecipitation was performed with anti-Myc antibody and GFP-FOXA1 was detected with anti-GFP antibody.
Subsequently, we used EAF2 deletion mutants to further determine which part of EAF2 protein is required for the interaction with FOXA1. In Figure 2C, GFP-FOXA1 can be co-precipitated by Myc-EAF2 (full-length), Myc-EAF2 (amino acids (aa) 1-113) and Myc-EAF2 (aa 1-161) using anti-Myc antibody conjugated to agarose beads. However when Myc-EAF2 (aa 113-260) was precipitated, there was no GFP-FOXA1 detected. The results indicated that the N-terminal portion (aa 1-113) of EAF2 is responsible for the interaction of FOXA1 and EAF2 (Figure 2C).
EAF2 decreases the protein levels of FOXA1
Since EAF2 and FOXA1 could interact with each other, we tested if EAF2 could affect the protein levels of FOXA1. We first knocked down EAF2 protein in LNCaP cells using two different specific siRNAs and found that depletion of EAF2 led to an increase in the endogenous FOXA1 protein level (Figure 3A). To test whether this increase occurred at the protein or the mRNA level, we employed real-time PCR and detected no difference in FOXA1 mRNA level when LNCaP cells were treated with siRNA specific to EAF2 or control siRNA (Figure 3B). This indicted that EAF2 can modulate FOXA1 protein level. To further confirm this, we co-transfected FOXA1-expressing plasmids with increasing amounts of GFP-EAF2 plasmids in HEK 293 cells and found that as the amount of GFP-EAF2 plasmid increased, the protein levels of FOXA1 decreased. These observations suggest that EAF2 can negatively affect FOXA1 protein stability in LNCaP prostate cancer cells.
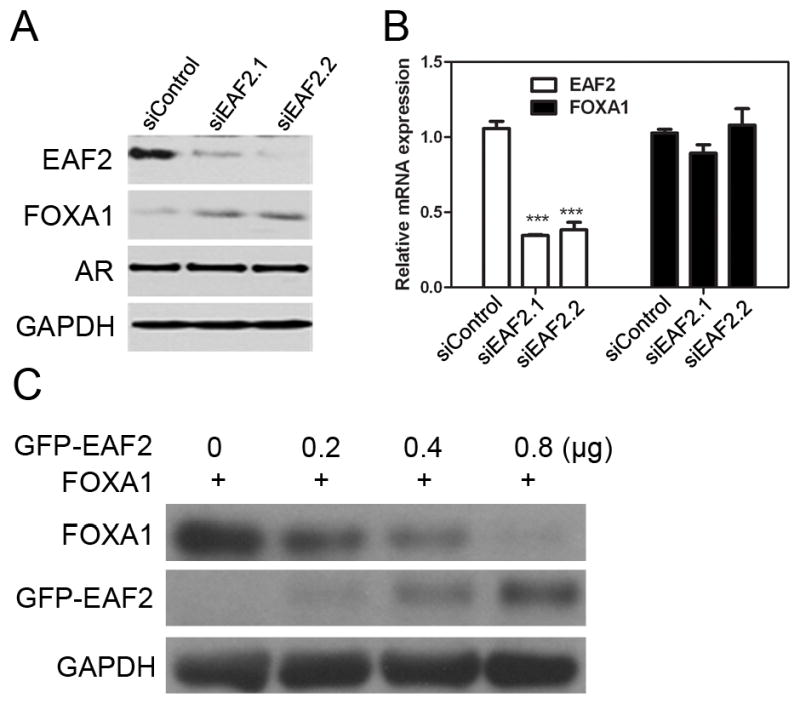
(A) Two different siRNAs targeting EAF2 were used separately at 100 pmol each to knockdown EAF2 expression in LNCaP cells. Endogenous protein levels of FOXA1, AR and EAF2 were detected by Western blot using anti-FOXA1 anti-AR, and anti-EAF2 antibodies. The protein level of FOXA1 increased upon EAF2 knockdown. (B) Total RNA was isolated from LNCaP cells after knockdown of EAF2. Relative mRNA levels of FOXA1 normalized against GAPDH were determined using real-time PCR. (C) HEK293 cells were co-transfected with 0.2 μg FOXA1 and increasing amounts of GFP-EAF2. Expression levels of FOXA1and EAF2 were determined by Western blot. GAPDH protein was detected as a loading control.
FOXA1 modulates EAF2 repression of AR-dependent transcriptional activity
Given the important role of FOXA1 in AR transcriptional activation in prostate cancer, the co-immunoprecipitation between EAF2 and FOXA1 raised the possibility that EAF2 regulates AR transcriptional activity via FOXA1. To test this, we examined the effects of knocking down EAF2 and FOXA1 individually or in combination on the gene expression of two well-known AR target genes, prostate specific antigen (PSA) and the TMPRSS2-ETS fusion genes (27,28), by real-time PCR in LNCaP cells. Figure 4D&E showed that knockdown of EAF2 resulted in an increase in the mRNA levels of PSA and TMRPASS. To test whether this suppression function was mediated by FOXA1, we tested whether EAF2 knockdown can also up-regulated the expression of PSA and TMRPSS2 when FOXA1 was knocked down at the same time. As shown in Figure 4D&E, knockdown of FOXA1 alone or both FOXA1 and EAF2 caused similar down-regulation of PSA and TMRPASS mRNA levels. Interestingly, FOXA1 knockdown also caused a decrease in the mRNA level of EAF2 (Figure 4A). This can be explained by the fact that EAF2 is an androgen-induced gene. To rule out the possibility that EAF2 regulates these AR target genes through changing expression levels of AR, we tested the mRNA and protein levels of AR and found no significant differences between the groups (Figure 4A&C). These results suggested that EAF2 suppression of AR transcriptional activity is mediated through FOXA1.
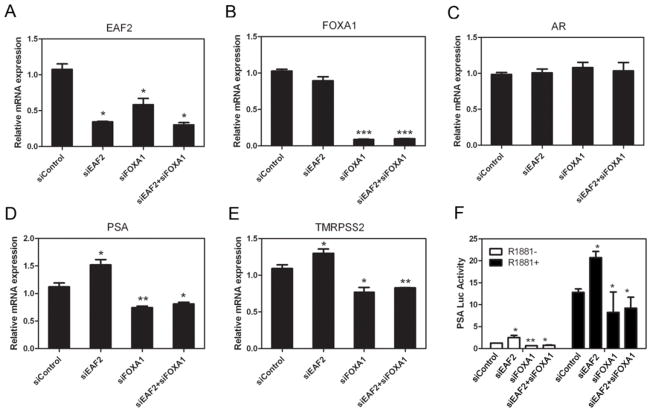
LNCaP cells were cultured in the presence of indicated siRNAs for 24 hours and then treated with 1 nM R1881 for 48 h. Subsequently, total RNA was extracted and mRNA levels of EAF2 (A), FOXA1 (B), AR (C), PSA (D) and TMPRSS2 (E) were determined by real-time PCR. (F) LNCaP cells were transfected with siRNA targeting the indicated proteins followed by transfection with 0.5 μg PSA-promoter driven fire-fly luciferase vector and 0.05 μg pCMV-Renilla in the presence or absence of the androgen analog R1881 (1 nM). Luciferase assays were performed as described in the Materials and Methods.
To further demonstrate EAF2 repression of AR transcriptional activity via FOXA1, we performed luciferase assays using a PSA promoter-driven luciferase reporter. As expected, knockdown of EAF2 alone enhanced the transcriptional activity of the PSA promoter. In contrast, knockdown of EAF2 had no significant effect on the PSA promoter activity when FOXA1 was knocked down concurrently. Knockdown of FOXA1 alone and knockdown of both EAF2 and FOXA1 caused similar down-regulation of the PSA promoter activity (Figure 4F). These results further suggested FOXA1 mediates EAF2 repression of AR transcriptional activity.
FOXA1 modulates EAF2 suppression of LNCaP cell proliferation and migration
It is well established that AR is required for prostate cancer cell proliferation (29,30). Thus, we considered the possibility that EAF2 could inhibit prostate cancer cell proliferation in part due to its suppression of AR transcriptional activity via FOXA1. To explore this possibility, we evaluated cell proliferation after knockdown of EAF2 and FOXA1 separately and in combination. LNCaP cells were transfected with siRNA targeting EAF2 or FOXA1 separately or in combination and then subjected to a BrdU proliferation assay. As can be seen in Figure 5, knockdown of EAF2 promoted while knockdown of FOXA1 inhibited BrdU incorporation in LNCaP cells (p < 0.05). When the two proteins were depleted simultaneously, the number of BrdU positive cells was slightly higher, with no statistical significance, when compare with FOXA1 knockdown alone (Figure 5A, B). These results indicated that the suppression effect of EAF2 on prostate cancer cells proliferation is in part mediated by the interaction of EAF2 and FOXA1. The effect of EAF2 and/or FOXA1 transfection on colony formation in LNCaP cells (Figure 6) is consistent with the results of knockdown of EAF2 and/or FOXA1 on cell proliferation (Figure 5A & C). As expected, EAF2 transfection inhibited while FOXA1 transfection enhanced LNCaP colony formation as compared to the empty vector control. Importantly, EAF2 transfection blocked the FOXA1 enhancement of LNCaP colony formation (Figure 6), also suggesting the capability of EAF2 to inhibit FOXA1 function.
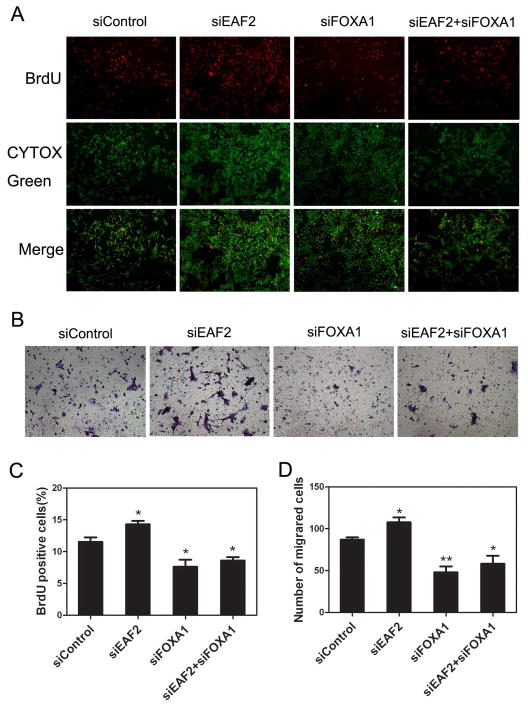
(A) LNCaP cells were transfected with specific siRNAs as indicated. Twenty four hours after transfection, LNCaP cells were cultured in the presence of 1 nM R1881 for 48 h, followed by incubation with BrdU for 6 h for BrdU assay. Images shown are representative pictures of different treatment groups. (B) LNCaP cells were transfected with siRNA at 100 pmol each as indicated in a 6-well plate for 48h, and then were harvested by trypsin treatment and placed in the top chamber. After 36 hours, the cells at the lower surface of chamber membranes (migrated) were stained and counted. Images shown are representative pictures of different treatment groups. (C) Quantitative analysis of BrdU-positive cells. (D) Quantitative analysis of migrated cells.
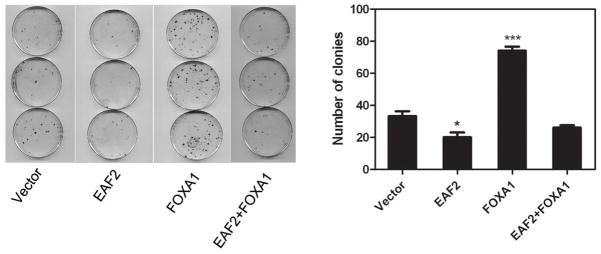
Effect of EAF2 and/or FOXA1 transfection on LNCaP cell colony formation. Left panel shows the colonies formed by LNCaP cells transfected with indicated expression vectors. Right panel shows the quantitative analysis of the colonies of the left panel. Transfection and quantitative analysis of colonies were conducted as described in Materials and Methods.
It was reported that FOXA1 expression is associated with the development of metastatic prostate cancer (31,32). To test whether EAF2 can affect prostate cancer cell migration through FOXA1, we performed transwell migration assays using LNCaP cells transfected with siRNAs as described above. The results showed that knockdown of EAF2 could stimulate LNCaP cell migration when compared with the control group (Figure 5B&D). In contrast, knockdown of FOXA1 inhibited LNCaP cell migration (Figure 5B&D). In the presence of both siEAF2 and siFOXA1, the inhibition of cell migration was slightly less but not statistically significant when compared to the FOXA1 knockdown group (Figure 5B, D). LNCaP proliferation rate is slow, with doubling time of ~60 hours (33). The same number of cells was used in the transwell migration assay, and the assay was completed within 36 hours, significantly less than the doubling time of LNCaP. Thus, the contribution of proliferation to the results in Figure 5B and 5D should be very limited. Our results suggested that EAF2 suppression of LNCaP cell migration is in part mediated through FOXA1.
DISCUSSION
The present study demonstrated that EAF2 interacts with FOXA1 physically and functionally to modulate androgen signaling, cell proliferation and migration in human prostate cancer LNCaP cell line. These findings suggested that an important mechanism of EAF2 action is mediated through its interaction with FOXA1.
Our studies suggest that EAF2 interaction with FOXA1 is conserved evolutionarily, from C. elegans to human. Knockdown of FOXA1 in human LNCaP cells and its ortholog in C. elegans had significant effects on the phenotypes caused by knockdown of EAF2 in human cells or mutation of its ortholog in C. elegans. The binding of FOXA1 to the N-terminal region of EAF2 is consistent with a prediction of a conserved role of EAF2-FOXA1 interaction in different organisms because the most conserved sequence in EAF family proteins is the N-terminus. Also, the N-terminal region of EAF2 is responsible for the growth suppression and pro-apoptotic activity of EAF2 (23). The binding of FOXA1 to this important region of EAF2 suggests that this interaction may mediate part of EAF2 suppression of prostate cancer cell proliferation.
The induction of FOXA1 protein levels, but not FOXA1 mRNA levels, by EAF2 knockdown suggested that EAF2 expression can reduce the stability of the FOXA1 protein. This finding was supported by the observation that the protein levels of transfected FOXA1 decreased as GFP-EAF2 plasmid and protein levels increased. FOXA1 plays an important role in modulation of AR-targeted gene expression, cell proliferation and motility. The increased FOXA1 protein level in LNCaP cells upon EAF2 knockdown is likely to contribute to the elevated expression of AR-targeted genes, proliferation, and motility. As expected, EAF2 knockdown increased the expression of AR-targeted genes, PSA, and TMRPSS2, and enhanced cell proliferation and invasion. As a control, knockdown of FOXA1 inhibited the expression of AR-targeted genes and cell proliferation and migration. The knockdown of both EAF2 and FOXA1 exhibited a slight, but not statistically significant, increase in AR-targeted gene expression, cell proliferation and migration when compared to the knockdown of FOXA1 alone. These data suggest that the tumor-suppressive activity of EAF2 is in part mediated through interaction with FOXA1. This interpretation is consistent with our previous findings that EAF2 can interact with multiple binding partners including ELL family proteins, pVHL, and p53 (9,11,23). These proteins are known to play important roles in cellular signaling involved in tumorigenesis and they can also mediate EAF2 action. Thus, FOXA1 appears to mediate part of the mechanisms of EAF2 action.
The ability of EAF2 to decrease the level of FOXA1 protein provides a potential negative feedback mechanism in androgen signaling. Other investigators have previously reported the regulation of AR by its target gene products via a positive or negative feedback loop to modulate AR signaling in prostate cancer (22,34). NKX3.1, for instance, a downstream target of AR, can in turn collaborate with AR and FOXA1 to control the AR transcriptional network in prostate cancer (22). Androgen induction of EAF2 could downregulate the FOXA1 protein level, which will subsequently limit the activity of AR. Thus, the EAF2-FOXA1 interaction provides another mechanism to prevent overactivation of androgen signaling and to maintain prostate homeostasis.
Our studies also suggest that C. elegans is an excellent model to study the mechanism of EAF2 action. The modulators of EAF2 ortholog in C. elegans are likely to retain its functional role in mammalian cells. Importantly, functional modulators of EAF2 identified from the C. elegans model can be tested in mammalian cells including prostate cancer cells. This research strategy provides a powerful approach to dissect the mechanisms of EAF2 action in prostate carcinogenesis.
We would like to point out that there are discrepancies in the results of functional studies of FOXA1 in prostate cancer cells. In some studies, FOXA1 can increase AR target gene expression (16), promote prostate cancer cell proliferation (19,35) and invasion and migration (31), which is consistent with our results. In other studies, FOXA1 seems to decrease AR target gene expression levels (20), inhibit cell proliferation (20), invasion and migration (35). These discrepancies may reflect the differences in cell line models and/or experimental conditions.
In summary, our findings reveal a novel regulatory network between EAF2, FOXA1 and AR. As an androgen-induced gene, EAF2 can interact with FOXA1 and in turn to modulate AR, functioning as a co-repressor to repress AR transcriptional activity. This represents an AR signaling feedback loop that may play an important role maintaining prostate homeostasis. Moreover, EAF2 can inhibit prostate cells proliferation and migration in part via FOXA1. However, the mechanism of EAF2 modulation of FOXA1 protein level remains unknown and will need to be investigated in future studies.
Acknowledgments
We are grateful to Dr. Shohei Mitani (National Bioresource Project) for providing eaf-1 (tm3976) C. elegans mutants and Dr. Marianne Sadar from British Columbia Cancer Agency for providing pPSA6.1Luc reporter plasmid. We would like to thank Dr. Laura E. Pascal for critical reading and editing. This research was supported in part by NIH grant R01 CA186780 to ZW and National Science Foundation of China (NSFC) Project 81130046 to JZ. Anne L. Keener was a recipient of DOD pre-doctoral fellowship W81XWH-11-1-0316.
References
Full text links
Read article at publisher's site: https://doi.org/10.1002/pros.22982
Read article for free, from open access legal sources, via Unpaywall:
https://europepmc.org/articles/pmc4424106?pdf=render
Citations & impact
Impact metrics
Citations of article over time
Alternative metrics
Smart citations by scite.ai
Explore citation contexts and check if this article has been
supported or disputed.
https://scite.ai/reports/10.1002/pros.22982
Article citations
EAF2 Downregulation Recruits Tumor-associated Macrophages in Prostate Cancer through Upregulation of MIF.
Biol Proced Online, 26(1):21, 05 Jul 2024
Cited by: 0 articles | PMID: 38969982 | PMCID: PMC11225222
Role of sex steroids in colorectal cancer: pathomechanisms and medical applications.
Am J Cancer Res, 14(7):3200-3221, 15 Jul 2024
Cited by: 0 articles | PMID: 39113870 | PMCID: PMC11301278
Review Free full text in Europe PMC
Mechanism of ELL-associated factor 2 and vasohibin 1 regulating invasion, migration, and angiogenesis in colorectal cancer.
World J Gastroenterol, 29(24):3770-3792, 01 Jun 2023
Cited by: 2 articles | PMID: 37426316 | PMCID: PMC10324531
ELL associated factor 2 is a potential diagnostic and prognostic indicator: evidence from the in silico and in vitro experiments.
Am J Cancer Res, 13(6):2572-2587, 15 Jun 2023
Cited by: 0 articles | PMID: 37424805 | PMCID: PMC10326572
Overexpression of ELL-associated factor 2 suppresses invasion, migration, and angiogenesis in colorectal cancer.
World J Gastrointest Oncol, 14(10):1949-1967, 01 Oct 2022
Cited by: 3 articles | PMID: 36310706 | PMCID: PMC9611430
Go to all (19) article citations
Data
Similar Articles
To arrive at the top five similar articles we use a word-weighted algorithm to compare words from the Title and Abstract of each citation.
Identification of a genetic interaction between the tumor suppressor EAF2 and the retinoblastoma protein (Rb) signaling pathway in C. elegans and prostate cancer cells.
Biochem Biophys Res Commun, 447(2):292-298, 12 Apr 2014
Cited by: 5 articles | PMID: 24727455 | PMCID: PMC4038965
Androgen receptor-independent function of FoxA1 in prostate cancer metastasis.
Cancer Res, 73(12):3725-3736, 28 Mar 2013
Cited by: 97 articles | PMID: 23539448 | PMCID: PMC3686855
Nfib Regulates Transcriptional Networks That Control the Development of Prostatic Hyperplasia.
Endocrinology, 157(3):1094-1109, 17 Dec 2015
Cited by: 18 articles | PMID: 26677878 | PMCID: PMC4769366
Pioneer of prostate cancer: past, present and the future of FOXA1.
Protein Cell, 12(1):29-38, 18 Sep 2020
Cited by: 68 articles | PMID: 32946061 | PMCID: PMC7815845
Review Free full text in Europe PMC
Funding
Funders who supported this work.
NCI NIH HHS (1)
Grant ID: R01 CA186780
NIA NIH HHS (1)
Grant ID: R01 AG044768
NIEHS NIH HHS (1)
Grant ID: R01 ES017761
National Institutes of Health (1)
Grant ID: CA186780
National Natural Science Foundation of China (1)
Grant ID: 81130046
U.S. Department of Defense (1)
Grant ID: W81XWH-11-1-0316