Abstract
Free full text

Matrix metalloproteinases in liver injury, repair and fibrosis
Abstract
The liver is a large highly vascularized organ with a central function in metabolic homeostasis, detoxification, and immunity. Due to its roles, the liver is frequently exposed to various insults which can cause cell death and hepatic dysfunction. Alternatively, the liver has a remarkable ability to self-repair and regenerate after injury. Liver injury and regeneration have both been linked to complex extracellular matrix (ECM) related pathways. While normal degradation of ECM components is an important feature of tissue repair and remodeling, irregular ECM turnover contributes to a variety of liver diseases. Matrix metalloproteinases (MMPs) are the main enzymes implicated in ECM degradation. MMPs not only remodel the ECM, but also regulate immune responses. In this review, we highlight some of the MMP-attributed roles in acute and chronic liver injury and emphasize the need for further experimentation to better understand their functions during hepatic physiological conditions and disease progression.
Introduction
The liver is a vital organ, responsible for the metabolism of carbohydrates, proteins and lipids, removal of drugs and toxins from the blood, and regulation of immune responses [1]. The hepatic parenchyma is organized in lobules, which are repetitive functional units consisting of hepatocytes, endothelial cells, Kupffer cells, stellate cells, and bile duct cells [2]. Hepatocytes carry out most of the metabolic functions of the liver, and account for about 80% of the liver weight and for about 70% of all liver cells [3]. Hepatocytes, endothelial cells, as well as other liver cells, are each uniquely susceptible to a number of insults and take part in diverse clinically recognized syndromes of liver injury [4]. Alternatively, the liver has a remarkable regenerative potential as evidenced by the capability to regulate its growth and mass after hepatectomy and by its recovery after ischemic, toxic, or infectious acute liver injury [5,6]. The extracellular matrix (ECM), formed by the complex network of proteins and sugars surrounding cells in all solid tissues, is among the most important regulators of cellular and tissue functions in the body [7]. In addition to providing a physical scaffold and structural support for cells, ECM regulates various cellular functions, such as adhesion, migration, differentiation, proliferation, and survival. Cellular responses are context dependent, and dysregulation of ECM production and proteolysis is often associated with the development of liver pathology [8].
Matrix metalloproteinases (MMPs) are a family of over 24 zinc-dependent endopeptidases capable of degrading virtually any component of the ECM [9]. Since their initial discovery approximately 50 years ago, MMPs have emerged as essential mediators in defining how cells interact with their surrounding microenvironment [10]. MMPs have been categorized into five major groups according to their ECM substrate specificity: collagenases, gelatinases, membrane-type, stromelysins and matrilysins [11]. In addition to their recognized roles in ECM protein degradation and rearrangement, MMPs also act on non-ECM substrates, such as cytokines and chemokines, and have regulatory functions in inflammation and immunity [12]. MMPs are generally secreted into the extracellular environment, or tethered to the cell membranes, as inactive proenzymes [13]. The regulation of MMP activity is a tightly controlled process and it takes place at transcriptional, post-transcriptional, and at protein levels [14]. Dysregulation of MMP activity often results into tissue damage and functional alterations [15]. Tissue inhibitors of metalloproteinases (TIMPs) are a family of at least four identified physiological inhibitors (TIMP 1–4) capable of regulating proteolytic activities of MMPs in tissues [9,15]. TIMPs are secreted molecules that bind reversibly to MMPs in a 1:1 stoichiometric ratio. Alterations in MMP–TIMP balances have been linked to pathologies that require disruption of basement membranes, such as tumor invasion, angiogenesis, and wound healing [14,16]. However, the biology of MMPs is rather complex, since the same MMP can have opposing effects based upon the cell type or tissue in which it is expressed [17]. The choice of which MMPs to target for therapeutic purposes is still uncertain, even in fields like cancer, where MMPs have been extensively studied [18]. This article examines the role of MMPs and their TIMP natural occurring inhibitors in the development of both acute and chronic liver injury, and discusses the potential for MMP modulation in the prevention and treatment of liver diseases.
Extracellular matrix proteins and matrix-degrading proteases of normal liver
ECM proteins form distinct networks with tissue-specific variation in composition and architecture [19]. These macromolecular networks surround stromal cells and underlie endothelial and epithelial cells. In a normal liver, ECM comprises less than 3% of the relative area on a tissue section, and approximately 0.5% of the wet weight. [20] Collagen, fibronectin, laminin, proteoglycans, and matricellular proteins are among the most characterized ECM components in the normal liver. Liver ECM proteins are mostly detected in the Glisson’s capsule, portal tracks, central veins, and in the subendothelial space of Disse [20,21]. Collagen types I, III, IV, and V are the predominant collagens detected in the liver; collagen types I, III, and V are mostly interstitial ECM proteins in the portal and central regions, whereas collagen IV is highly detected in basement membranes [21]. Fibronectin is abundantly expressed in the hepatic ECM; it is detected in the subcapsular connective tissue, in septa, and portal areas, and it is the main ECM component in Disse’s space in normal livers [22]. The space of Disse is characterized by a low density basement membrane-like matrix, which sustains the differentiated stage of the surrounding cells and allows metabolic exchanges to take place between the blood flow and the microvilli of the hepatocytes [2,21]. Laminin similarly to collagen IV is a main component of the hepatic basement membranes [22].
ECM changes in the liver depend upon ECM synthesis and MMP-mediated ECM proteolytic degradation. Healthy adult livers have a moderate ECM turnover, which seems to correlate with the relatively small amounts of MMPs constitutively detected in those livers [23]. MMP-1, MMP-2, MMP-3, MMP-11, and MMP-13 are among the MMPs constitutively expressed in normal livers [24–27]. In addition to remodeling the ECM, MMPs can have other biological roles. MMP-2, a member of the gelatinase family which also includes MMP-9, is detected in the vascular areas of naïve livers (MMP-9 is virtually absent in naïve livers) [27]. Our unpublished studies (Kato & Coito) suggest that MMP-2 has an important role in the preservation of liver vascular homeostasis, through its participation in the TGF-β activation process. In addition to mediating TGF-β activation, MMP-2 is capable of modulating the activity of IL-1β, TNF- α, and MCP-3, through proteolytic cleavage [28,29]. MCP-3 is also efficiently cleaved by MMP-1 and MMP-3 [29]. MMP activity is controlled by TIMPs under physiological conditions. Low levels of TIMP-1 have been detected in the parenchyma of naïve murine livers [30]. It has been shown that exogenous TIMP-1 inhibits apoptosis of various cell types, including hepatic stellate cells and endothelial cells, which support the view that the basal expressions of TIMP-1 detected in naïve livers may contribute to the survival of liver cells [31,32]. TIMP-3 gene deletion results in spontaneous lymphocyte infiltration in naïve livers, suggesting a role in preserving liver homeostasis [33]. All together, MMPs and TIMPs seem to have important roles in the preservation of liver homeostasis. These roles should be taken into account when designing improved MMP-targeted therapies for hepatic diseases; therefore, more mechanistic studies are needed to further decipher the physiologic roles of MMPs and TIMPs in the liver.
Matrix metalloproteinases in liver injury
The liver is susceptible to various forms of injury which are frequently screened for in clinical practice. Regardless of cause, direct damage to liver cells and to their organelles can lead to the release of reactive oxygen and nitrogen (ROS, RNS) species and other pro-inflammatory mediators, which activate innate immune cells. In turn, activated Kupffer cells and infiltrating leukocytes can act to aggravate the initial liver injury [34]. A prominent feature of the hepatic response to injury is the very early appearance of newly synthesized ECM proteins, such as cellular EIIIA-fibronectin and tenascin-C [35–37]. In self-limited acute inflammation, the ECM changes are transient and the liver architecture is mostly restored, whereas in sustained injury, a persistent inflammatory response and increased accumulation of ECM progressively replace the liver parenchyma by scar fibrotic tissue [2]. Hepatic injury is frequently categorized into acute and chronic liver injury and MMPs have been linked to a number of acute and chronic liver disorders (summarized in Table 1).
Table 1
Matrix metalloproteinases in liver injury.
Matrix Metalloproteinase | Liver pathology |
---|---|
MMP-1 (collagenase-1) | • ECM degradation in hepatic fibrosis [76]. |
MMP-2 (gelatinase-A) | • Expressed in liver IRI [24,27]. |
• Preserved vascular homeostasis in liver IRI. [Kato & Coito, unpublished studies] | |
• Expressed in human fibrotic livers [92]. | |
• Absence exacerbated liver fibrosis [85]. | |
• Suppressed collagen type I expression [84]. | |
MMP-3 (stromelysin-1) | • Expressed in normothermic ischemia injury [48]. |
• Expressed in hepatocellular carcinoma [93]. | |
MMP-7 (matrilysin-1) | • Upregulated in liver IRI. [Baber & Coito, unpublished studies] |
• Expressed in biliary atresia fibrosis [94]. | |
• Associated to colorectal carcinoma liver metastasis [95]. | |
MMP-8 (collagenase-2) | • Upregulated in liver IRI. [Duarte & Coito, unpublished studies] |
• Overexpression reduced fibrosis [96]. | |
• Expressed in cholestatic injury [75]. | |
• Promoted leukocyte infiltration in TNF-induced acute hepatitis [60]. | |
MMP-9 (gelatinase-B) | • Expressed by leukocytes in liver IRI [24]. |
• Expressed in normothermic ischemia injury [48]. | |
• Mediated leukocyte infiltration in liver IRI [27]. | |
• Promoted PECAM-1 proteolytic breakdown in liver IRI [97]. | |
• Hepatocyte apoptosis in liver IRI [51]. | |
• Activated myeloperoxidase in liver IRI [27]. | |
• Impaired liver regeneration after liver IRI [97]. | |
• Favored hepatic regeneration after hepatectomy [65]. | |
• Mediated acute small-for-size graft injury [49]. | |
• Facilitated brain extravasation and edema in fulminant hepatic failure [98,99]. | |
• Promoted hepatic stellate cell apoptosis [100]. | |
• Expressed in hepatocellular carcinoma [101–104]. | |
• Associated to neutrophil infiltration in monocratiline-induced acute liver injury [56]. | |
MMP-10 (stromelysin-2) | • Upregulated in liver IRI.[Duarte & Coito, unpublished studies] |
• Promoted hepatic repair and regeneration after partial hepatectomy and bile duct ligation [105]. | |
• Expressed in hepatocellular carcinoma [93]. | |
MMP-11 (stromelysin-3) | • Expressed in normothermic ischemia injury [48]. |
MMP-12 (metalloelastase) | • Expressed in normothermic ischemia injury [48]. |
• Degraded elastin in hepatic fibrosis [106]. | |
• Promoted inflammation and IL-13 induced hepatic fibrosis [107]. | |
MMP-13 (collagenase-3) | • Expressed in acute liver injury [108]. |
• Accelerated liver fibrogenesis [79]. | |
• Promoted recovery from liver fibrosis [77,78,109]. | |
MMP-14(MT1-MMP) | • Associated to liver recruitment in liver IRI [52]. |
• Upregulated after partial hepatectomy [66]. | |
• Expressed in highly invasive hepatocellular carcinoma [110]. | |
• Expressed in liver fibrosis and linked to its resolution [92,111]. | |
MMP-15(MT2-MMP) | • Downregulated after partial hepatectomy [66]. |
MMP-16(MT3-MMP) | • Expressed in hepatitis [112]. |
• Expressed in cirrhosis and hepatocellular carcinoma [112]. | |
MMP-19 (enamelysin) | • Promoted TGF-p signaling in development of liver fibrosis [87]. |
MMP-24 (MT5-MMP) | • Expressed after partial hepatectomy [66]. |
Acute liver injury
Acute liver injury is a common clinical occurrence stemming from a variety of different etiologies such as drugs, toxins, ischemia/reperfusion events, bile acids, and pathogens. Acute cellular damage mediated by oxidative stress elicits an immune response that, when uncontrolled, can lead to significant cell death [34]. If extensive cell death is not adequately balanced by liver regenerative activity, acute liver injury may progress into acute liver failure (ALF) [38]. ALF has a high mortality rate and is one of the most frequent causes for emergency orthotopic liver transplantation (OLT) [39].
In recent years, there has been considerable interest in unveiling the roles of MMPs in acute liver injury, including in settings of hepatic ischemia and reperfusion injury (IRI). Liver IRI is a prominent feature in various clinical settings that span from surgical procedures to hepatic pathologies, where blood flow to the liver is partially or completely impeded. In human liver transplantation, IRI is a major determinant of postoperative allograft dysfunction and morbidity; it also increases the risk of acute and chronic rejections [40]. Recruitment of inflammatory leukocytes is a hallmark of hepatic IR-mediated damage; however, the process of leukocyte recruitment to sites of inflammatory stimulation in the liver is still poorly understood [41]. In general, leukocytes circulate continuously in the blood, and their migration across endothelial or ECM barriers to inflamed tissues is a complex process dependent on the coordination of cellular adhesion-release steps and focal matrix degradation mechanisms. [41] The liver is a venous-driven vascular bed with slow flow rates and it may require distinct cascades of adhesive/degradation events compared with other organs with higher flow rates and different ECM profiles. Fibronectin is a large glycoprotein with a recognized central role in cellular adhesion and migration, and it is likely an important ECM protein involved in leukocyte adhesion during liver IRI [35]. The expression of cellular (EIIIA+) fibronectin by liver sinusoidal endothelial cells is an early feature after injury [37], and it precedes leukocyte recruitment in hepatic IRI [35]. Moreover, the blockade of fibronectin interactions with its most characterized α4β1 and α5β1 integrin receptors lowers leukocyte recruitment and ameliorates liver IRI in experimental organ transplantation [35,42]. Tenascin-C, similarly to cellular fibronectin, is not normally detected in adult livers, and it is likely a prominent damage-associated molecular pattern (DAMP) molecule within the ECM [43]. Tenascin-C is expressed in damage livers and its gene deletion results in reduced leukocyte recruitment and cytokine expression after hepatic reperfusion [36]. Among different MMPs, MMP-9 is emerging as an important mediator of leukocyte traffic in hepatic IRI [41]. MMP-9 is an inducible gelatinase expressed by leukocytes in acutely damaged livers [27]. MMP-9 is responsible for the turnover and degradation of several ECM proteins, including fibronectin and type IV collagen [44]. In addition to promoting ECM breakdown, MMP-9 may also increase vascular permeability by cleaving PECAM-1 and/or other endothelial junctional proteins during liver reperfusion [45]. In human orthotopic liver transplantation, MMP-9 has been detected in the serum of patients minutes after reperfusion [46], and it remained elevated for several days after transplantation [47]. In rat livers, MMP-9 has been shown by our laboratory to be upregulated after 6 h following OLT [35], and by others after 3 h of IRI [48]. MMP-9 deficiency and anti-MMP-9 antibody therapy lower the infiltration of Ly-6G and Mac-1 leukocytes in mouse lean and steatotic livers after the IR-insult [27,45]. Further, MMP-9 inhibition has been shown to be beneficial in a model of small-for-size liver graft IRI [49]. On the other hand, TIMP-1 inhibition results in increased levels of MMP-9 activity and extensive leukocyte recruitment in severely damaged livers post-IRI [30]. MMP activity can be upregulated by nitric oxide (NO)-mediated S-nitrosylation [50]. The inhibition of nitric oxide synthase (iNOS)-derived NO production hampers MMP-9 activity as well as leukocyte recruitment in liver IRI [51].
Focal matrix degradation during leukocyte extravasation is likely regulated by a complex interplay of proteolytic cascades. MT1-MMP/MMP-14, which is expressed by infiltrating macrophages in damaged livers, facilitates their migration through FN coated membranes, suggesting that MT1-MMP/MMP-14 may act as an amplifier in the recruitment of macrophages in hepatic IRI [52]. MMP-2, MMP-3, MMP-8, MMP-10, MMP-12, and MMP-13 are among other significantly upregulated MMPs during hepatic IRI, but very little is still known about their specific functions in the progression of acute liver damage [27,48]. (Duarte & Coito, unpublished studies) MMP mediated proteolysis may not only facilitate leukocyte migration, but may also lead to detachment of parenchyma cells from ECM resulting in apoptosis, a phenomenon called “anoikis” [53]. In this regard, hepatocyte apoptosis is significantly reduced in the absence of MMP-9 after hepatic IRI [51]. Fig. 1 illustrates MMP-dependent liver damage after the IR-insult.
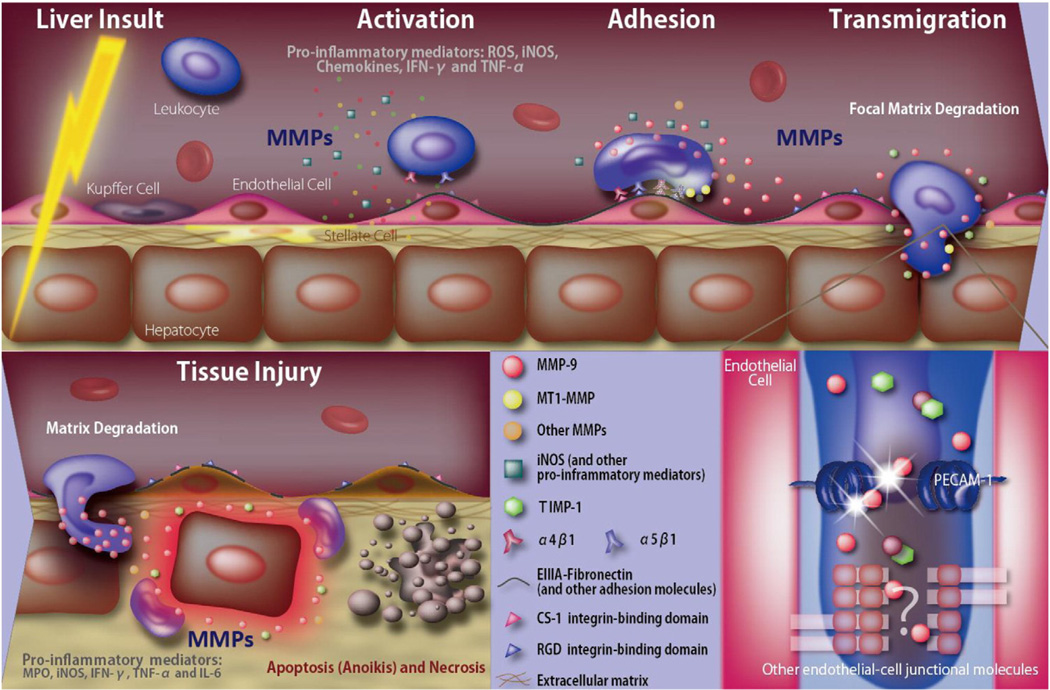
Schematic model for MMP-dependent liver damage after the IR-insult. The IR-insult to the liver results in MMP-dependentand independent release of ROS, cytokines, and other pro-inflammatory mediators, which activate innate immune cells and upregulate the expression of liver vascular adhesion molecules. Interactions between activated integrins (e.g. α4β1 and α5β1) expressed on leukocytes and newly synthesized adhesion molecules promote binding of leukocytes to the liver endothelium. MMPs, particularly leukocyte-derived MMP-9 (in the presence of low levels of TIMP-1), facilitate focal matrix degradation and leukocyte extravasation across vascular barriers. Besides leukocyte recruitment, MMP-mediated loss of the vascular endothelial cell barrier integrity interferes with the liver’s capability to regenerate after hepatic IRI. Additionally, MMPs contribute to tissue injury by promoting parenchyma cell detachment from ECM, resulting in apoptosis/anoikis of parenchyma cells. Pro-inflammatory factors produced during the acute phase of IRI, such as TNF-α, and iNOS-derived NO, further stimulate the production/activation of MMPs, which in turn modulate the activity of some of these factors through proteolytic cleavage; thus, providing a possible feedback loop that would amplify and sustain the inflammatory environment.
Drug induced acute liver injury (DILI) accounts for more than 50% of acute liver failure cases in the United States [54]. The pathological features of DILI are acute hepatocellular and endothelial cell death, cholestasis, and rapid recruitment of inflammatory leukocytes [54]. MMPs have been associated with microcirculatory dysfunctions and hepatocellular damage in acetaminophen-induced liver injury, one of the most studied models of DILI. It has been shown that treatment of livers subject to acetaminophen injury with a gelatinase inhibitor improves hepatic microcirculation and reduces the number of erythrocytes in the space of Disse [55]. In livers undergoing monocrotaline-induced acute injury, upregulation of MMP-9 correlates with the proteolysis of heparan sulfate and collagen IV and with increased neutrophil infiltration [56]. Moreover, suppression of MMP-9 activity attenuates microcirculatory obstruction in monocrotaline-induced acute liver injury [57,58]. High levels of TNF-α produced by macrophages is a feature of ALF [59]. Administration of batimastat (BB-94) in a murine model of TNF/GaIN induced ALF reduces leukocyte infiltration and protects livers from parenchymal apoptosis [59]. It has also been shown that MMP-8 inhibition, via reducing CXCL-5 chemokine activation, has a protective role in TNF/GaIN induced ALF [60].
In addition to hepatocyte cell death, impaired liver regeneration/repair is a common feature in acute liver failure. The balanced integration of a variety of cellular processes, such as metabolism, cell cycle regulation, angiogenesis, inflammation, cell adhesion, recruitment, and senescence, is important to assure liver regeneration [61]. While it is conceivable that ECM and MMPs can impact the majority of these processes, we are still far from understanding their roles during hepatic regeneration. Most studies in liver regeneration have been performed in murine models of partial hepatectomy; however, in humans, liver regeneration occurs most frequently after liver injury by ischemia or by other insults, such as toxins, viral infections, and immune-mediated injuries [62]. It is important to mention that hepatectomy models do not allow the investigation of the interaction of signals coming from the inflammatory cells and their expressed growth factors and cytokines [63]. Moreover, the origin of the cells that replace missing hepatocytes differs after partial hepatectomy and parenchymal cell necrosis [64]. Hence, it is somewhat expected that MMP-dependent mechanisms of liver regeneration may vary with the nature and extent of tissue injury. MMP-9 deficient mice show a delayed regenerative response after 70% hepatectomy [65], and TIMP-1 gene deletion increases the MMP-dependent release of hepatocyte growth factor (HGF) from ECM and accelerates regeneration after major liver resection [66]. On the other hand, TIMP-1 deficiency increases the MMP-mediated shedding of c-met tyrosine kinase (cMet), the HGF receptor, in MMP-9 + leukocyte-rich livers [30], and MMP-9 gene deletion enhances the hepatocyte progression into S phase and mitosis after liver IRI [45]. Intact sinusoidal endothelial cells are necessary to stimulate the initial burst of hepatocyte proliferation [67], and therefore, it is reasonable to postulate that MMP-mediated loss of the vascular endothelial cell barrier integrity interferes with the liver’s capability to regenerate after hepatic IRI [45]. Findings that physiologic doses of certain proinflammatory mediators, such as IL-6 and TNF-a induce liver regeneration, while their overexpressions inhibit the cell cycle progression [68], provide another example that roles and mechanisms cannot be generalized in liver regeneration. Interestingly, excessive protease activity in TIMP-3 deficient livers hampers their regeneration after hepatectomy; TIMP-3 gene deletion results in TNF-α increased activity and hepatocyte cell death after liver resection [69]. The mechanisms by which MMPs affect liver regeneration are likely multifactorial, and further experimentation is needed to better understand these mechanisms, particularly in clinical relevant models of liver injury.
Chronic liver injury
Chronic liver disease is a major cause of high morbidity and mortality worldwide [2]. Chronic hepatic damage is the result of out of control wound-healing responses from the liver to various stimuli, which include viral infections, alcohol and non-alcoholic steatohepatitis (NASH) [2]. If the insult is sustained, the liver parenchyma is replaced by fibrotic scar tissue, a process in which ECM and MMPs have recognized key functions.
Chronic inflammation and repair cause an excessive accumulation of ECM components, such as fibronectin, collagens, and proteoglycans, which are major players in the formation of scar tissue. On the other hand, MMPs and TIMPs are the main regulators of ECM turnover in hepatic fibrosis [70]. The roles of MMPs and TIMPs in hepatic fibrosis have been fairly studied in the carbon tetrachloride (CCI4)-injection and the bile duct ligation (BDL) murine models [70–73]. Hepatic stellate cells, which express ECM components, MMPs and TIMPs in different timeframes are thought to play central roles in the development of hepatic fibrosis [23]. More recently, it has been suggested that hepatocyte-derived MMPs are also important mediators of ECM turnover and that the MMP-cell source is likely important in determining the final fibrotic phenotype [74]. MMP-1, MMP-8 and MMP-13 seem to be among the candidates for an anti-fibrotic role, since their overexpression has been associated to significantly reduced liver fibrosis and enhanced hepatocyte proliferation [75–77]. MMP-13 up-regulation in the liver has been correlated with the change from normal to abnormal matrix turnover in the CCI4 injury model [74]. Moreover, MMP-13 gene deletion results in a retarded resolution of CCI4-induced fibrosis [78]. However, it has also been reported that MMP-13 mediates the initial inflammation and contributes to accelerating fibrogenesis in cholestatic livers [79]. MMP-9 and MMP-12 have recently been identified in a CD11 Bhi F4/80int Ly-6Clo macrophage subset associated with resolution of fibrosis [80]. MMP-9 expression has been detected in the early stages of hepatic fibrogenesis and it may release/activate TGF-β, a major pro-fibrotic cytokine, from ECM reservoirs [81–83]. Additionally, MMP-9 may promote hepatic stellate cell apoptosis in the presence of low levels of TIMP-1 [82]. Interestingly, it has been reported that MMP-2 participates in the resolution of liver fibrosis by suppressing collagen type I expression instead of its degradation [84–86]. MMP-19 has also been linked to hepatic fibrosis; MMP-19 deficient mice show impaired TGF-β signaling and reduced liver fibrosis in the CCI4 murine model [87]. The rate and patterns of matrix degradation can be changed by the balance between TIMP and MMPs [73]. In addition to over-expressing MMPs, there is growing evidence supporting a TIMP scavenging approach to increase the ECM degrading potential in hepatic fibrosis [73]. TIMP-1 and TIMP-2 are expressed in high levels in murine fibrotic livers after CCI4 administration and BDL [88]. TIMP-1 overexpression hinders the clearance of fibrotic matrix leading to extensive accumulation of interstitial ECM [89,90]. Moreover, treatment of fibrotic murine livers with modified synthetic siRNA targeting TIMP-2 reduces fibrosis by decreasing HSC activation and collagen accumulation [91]. These observations support the view that therapies aimed at overexpressing selective MMPs and reducing excessive TIMP levels may ameliorate hepatic fibrosis.
Concluding remarks
Hepatic acute and chronic injuries are important causes of liver failure and MMPs along with their respective TIMP physiological inhibitors are promising drug-targets for these pathological conditions. MMP-9 is emerging as an important mediator of leukocyte recruitment and target in acute liver injury, whereas a TIMP scavenging approach to reduce excessive TIMP-1 or TIMP-2 expressions is solidifying as an area of interest in hepatic fibrosis. However, we must recognize that the specific roles of MMPs and TIMPs during hepatic physiological conditions and disease progression are still far from being understood. In addition to their traditional ECM remodeling functions, MMPs have the ability to process a wide range of non-ECM subtracts, modifying their activity and bioavailability. Therefore, we believe that more comprehensive studies of the functions of MMPs and TIMPs are needed to provide new insights into the biology of liver damage and regeneration and to contribute in the development of more ‘tailored’ organ-based/disease-specific (OBDS) therapies against acute and chronic liver disorders.
Acknowledgments
This work was supported in part by the National Institutes of Health (NIH), National Institute of Allergy and Infectious Diseases (NIAID) R01AI057832 grant. The authors thank Francisco William Braga for proof reading this article.
References
Citations & impact
Impact metrics
Article citations
Characterization of regeneration initiating cells during Xenopus laevis tail regeneration.
Genome Biol, 25(1):251, 01 Oct 2024
Cited by: 1 article | PMID: 39350302 | PMCID: PMC11443866
Cancer Metastases to the Liver: Mechanisms of Tumor Cell Colonization.
Pharmaceuticals (Basel), 17(9):1251, 23 Sep 2024
Cited by: 0 articles | PMID: 39338413 | PMCID: PMC11434846
Review Free full text in Europe PMC
N6-methyladenosine (m6A) RNA modification in fibrosis and collagen-related diseases.
Clin Epigenetics, 16(1):127, 12 Sep 2024
Cited by: 1 article | PMID: 39261973 | PMCID: PMC11391634
Review Free full text in Europe PMC
Purinergic Signaling in Non-Parenchymal Liver Cells.
Int J Mol Sci, 25(17):9447, 30 Aug 2024
Cited by: 0 articles | PMID: 39273394 | PMCID: PMC11394727
Review Free full text in Europe PMC
Fibrosis and Hepatocarcinogenesis: Role of Gene-Environment Interactions in Liver Disease Progression.
Int J Mol Sci, 25(16):8641, 08 Aug 2024
Cited by: 1 article | PMID: 39201329 | PMCID: PMC11354981
Review Free full text in Europe PMC
Go to all (209) article citations
Similar Articles
To arrive at the top five similar articles we use a word-weighted algorithm to compare words from the Title and Abstract of each citation.
Role of matrix metalloproteinases in cholestasis and hepatic ischemia/reperfusion injury: A review.
World J Gastroenterol, 21(42):12114-12124, 01 Nov 2015
Cited by: 17 articles | PMID: 26576096 | PMCID: PMC4641129
Review Free full text in Europe PMC
Connective tissue remodeling: cross-talk between endothelins and matrix metalloproteinases.
Curr Vasc Pharmacol, 3(4):369-379, 01 Oct 2005
Cited by: 33 articles | PMID: 16248781
Review
The role of matrix metalloproteinase inhibitors in ischemia-reperfusion injury in the liver.
Curr Pharm Des, 12(23):2923-2934, 01 Jan 2006
Cited by: 25 articles | PMID: 16918422
Review
Extracellular matrix degradation and the role of hepatic stellate cells.
Semin Liver Dis, 21(3):373-384, 01 Aug 2001
Cited by: 290 articles | PMID: 11586466
Review
Funding
Funders who supported this work.
NIAID NIH HHS (2)
Grant ID: R01 AI057832
Grant ID: R01AI057832
National Institute of Allergy and Infectious Diseases (NIAID) (1)
Grant ID: R01AI057832