Abstract
Free full text

Fatty acid synthase is preferentially degraded by autophagy upon nitrogen starvation in yeast
Associated Data
Significance
Starvation-induced protein degradation by autophagy is considered to be nonselective. This study provides evidence, however, that upon nitrogen starvation fatty acid synthase is selectively degraded by autophagy. Our results suggest that fatty acid synthase degradation by the autophagic pathway is essential to maintain cell homeostasis under starvation.
Abstract
Autophagy, an evolutionarily conserved intracellular catabolic process, leads to the degradation of cytosolic proteins and organelles in the vacuole/lysosome. Different forms of selective autophagy have recently been described. Starvation-induced protein degradation, however, is considered to be nonselective. Here we describe a novel interaction between autophagy-related protein 8 (Atg8) and fatty acid synthase (FAS), a pivotal enzymatic complex responsible for the entire synthesis of C16- and C18-fatty acids in yeast. We show that although FAS possesses housekeeping functions, under starvation conditions it is delivered to the vacuole for degradation by autophagy in a Vac8- and Atg24-dependent manner. We also provide evidence that FAS degradation is essential for survival under nitrogen deprivation. Our results imply that during nitrogen starvation specific proteins are preferentially recruited into autophagosomes
Autophagy is a major cellular catabolic pathway in eukaryotes, responsible for the degradation of organelles and large protein aggregates. This process is induced under various stress conditions, such as amino acid starvation, hypoxia, and oxidative stress (1, 2).
In yeast (Saccharomyces cerevisiae), nitrogen starvation is known to induce macroautophagy (hereafter termed autophagy), which is essential mainly for supplementing amino acids needed for protein synthesis (3). Autophagy has long been considered to be a nonselective process except for the recruitment of ribosomes into autophagosomes, which was suggested to be selective under conditions of nitrogen starvation (4). Selective autophagy is thought to play a role mainly in cell homeostasis, and it uses the core autophagy genes and varying sets of proteins for different cargos (5). Autophagy-related protein 8 (Atg8), a key autophagic factor essential for autophagosome biogenesis, also plays a major role in selective autophagy by recruiting cargo proteins into the autophagosome (6).
Fatty acid synthase (FAS) is a large (2.6 MDa), essential enzymatic complex responsible for the entire synthesis of C16- and C18-fatty acids (7). FAS is composed of two subunits, Fas1 (Fasβ) and Fas2 (Fasα), which are arranged in an α6β6 macromolecular complex. Deletion of one of the FAS subunits has been shown to result in degradation of the other (8). Whereas the unassembled Fas2 subunit is short-lived and degrades in the proteasome, the unassembled Fas1, once induced, is degraded by autophagy. Moreover, the FAS complex is known to be delivered to the vacuole under potassium acetate starvation (8).
Here we investigated the role of autophagy in the degradation of the FAS complex. We report a physical interaction between Atg8 and FAS, and show that autophagy preferentially degrades FAS and decreases its activity during nitrogen starvation. We suggest that the selective degradation of FAS under nitrogen starvation requires the participation of Atg24 and Vac8, two factors that each play a role in selective autophagy (9–12). Finally, we show that FAS degradation is important for cell viability. We propose that selective degradation of proteins under nitrogen starvation occurs to maintain cell homeostasis.
Results
FAS Is Preferentially Degraded by Autophagy Under Nitrogen Starvation.
We set out to characterize the role of autophagy in FAS degradation and to assess the physiological role of this process.
To study the degradation of FAS, we used yeast strains in which either FAS1 or FAS2 is fused to GFP under its endogenous promoter. FAS-GFP degradation was monitored by the GFP-processing assay in which, upon delivery of GFP-tagged proteins to the vacuole, the proteins are degraded while GFP remains relatively stable, enabling delivery of the tagged protein to the vacuole to be assessed (13). Induction of autophagy by nitrogen starvation resulted in degradation of FAS in the vacuole, as indicated by the accumulation of free GFP and by the inhibition in FAS processing upon PMSF treatment (Fig. S1). Using immunoblot analysis we observed that upon nitrogen starvation, both Fas1-GFP and Fas2-GFP were rapidly degraded in the vacuole, as indicated by a decrease in the protein levels and by the accumulation of free GFP in the vacuoles (Fig. 1A). Shifting these strains from nitrogen starvation to rich medium resulted in an increase in FAS levels and the disappearance of free GFP (Fig. 1A). This assay enabled us to determine that the half-life of GFP in the vacuole is ~2 h. Fas1 and Fas2 degradation rates were equivalent, supporting the notion that the FAS complex is degraded as a whole (Fig. 1B) and that the process is rapid compared with that of the nonselective autophagic substrate phosphoglycerate kinase 1 (Pgk1) (Fig. 1C). Consistent with a previous report (8), FAS was delivered to the vacuole under potassium acetate starvation as well, but its degradation rate was significantly lower (Fig. 1C). Microscopic visualization of Fas1-GFP and Fas2-GFP revealed that in WT cells the FAS complex shifts upon nitrogen starvation from the cytosol to the vacuole (Figs. 1D and and2B).2B). FAS-GFP in pep4 S. cerevisiae strains accumulated in FM4-64+ vesicles inside the vacuole (presumably autophagic bodies) under nitrogen starvation, further supporting FAS degradation in the vacuole (Figs. 1D and and2B).2B). We next performed pulse and chase experiments to directly compare the degradation kinetics of Fas1 and the nonselective autophagic substrate Pgk1. As shown in Fig. 1E, FAS levels decreased rapidly upon nitrogen starvation, whereas the levels of Pgk1 remained relatively constant. Taken together, our results indicate that the FAS complex is preferentially degraded in the vacuole upon nitrogen starvation.
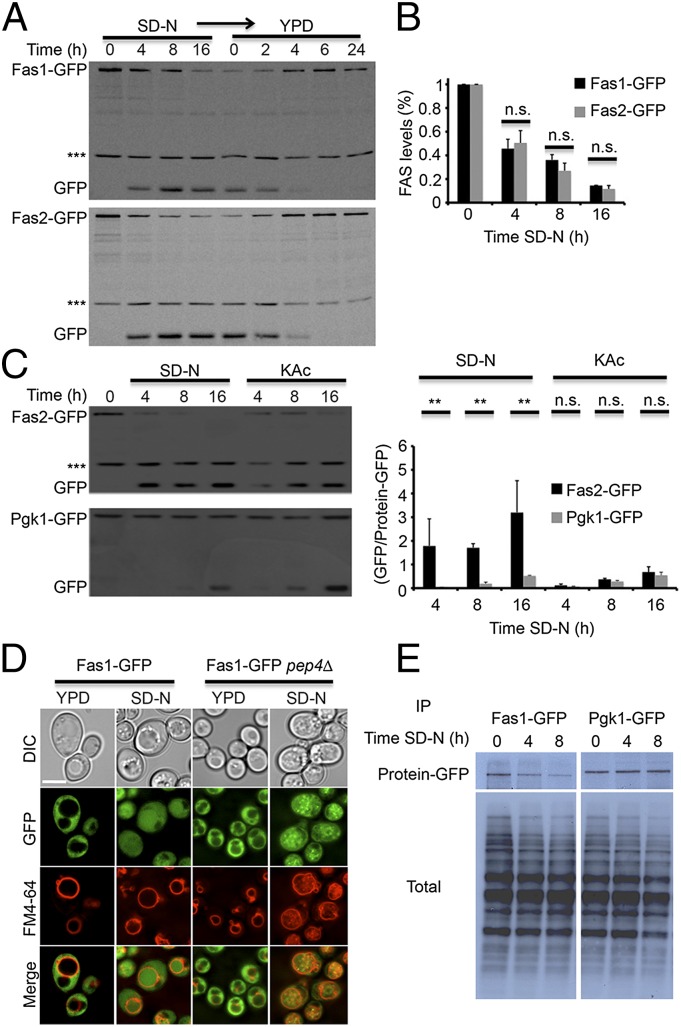
FAS is rapidly degraded under nitrogen starvation. (A) FAS1-GFP (TOS016) and FAS2-GFP (TOS017) cells of S. cerevisiae were grown to midlog phase and shifted to SD-N medium for the indicated time periods. After 16 h in SD-N, the cells were washed and shifted to YPD medium for the indicated time periods. Cell lysates were subjected to SDS/PAGE, followed by Western blot analysis using anti-GFP antibodies. (B) Quantification of Fas1-GFP and Fas2-GFP. Error bars represent the SDs of three independent experiments. n.s., not significant (Student’s t test). (C) FAS2-GFP (TOS017) and PGK1-GFP (TOS018) S. cerevisiae strains were grown to midlog phase and shifted to SD-N or KAc medium for the indicated time periods. Cell lysates were subjected to SDS/PAGE, followed by Western blot analysis using anti-GFP antibodies. Error bars represent the SDs of three independent experiments (Right); n.s., not significant; **P < 0.01 (Student’s t test). (D) FAS1-GFP (TOS016) and FAS1-GFP pep4 (TOS020) S. cerevisiae cells were grown to midlog phase and shifted to SD-N medium for 16 h. Fas1-GFP localization was monitored in YPD medium and after 16 h in SD-N. FM4-64 was used to detect vacuolar membranes. (Scale bar, 5 µm.) (E) FAS1-GFP (TOS016) and PGK1-GFP (TOS018) S. cerevisiae strains were grown to midlog phase, pulse-labeled with [35S] cysteine/methionine for 30 min, and chased in SD-N medium for the indicated time periods. Cell lysates were subjected to immunoprecipitation using anti-GFP antibodies and the proteins were analyzed by SDS/PAGE electrophoresis followed by autoradiography.
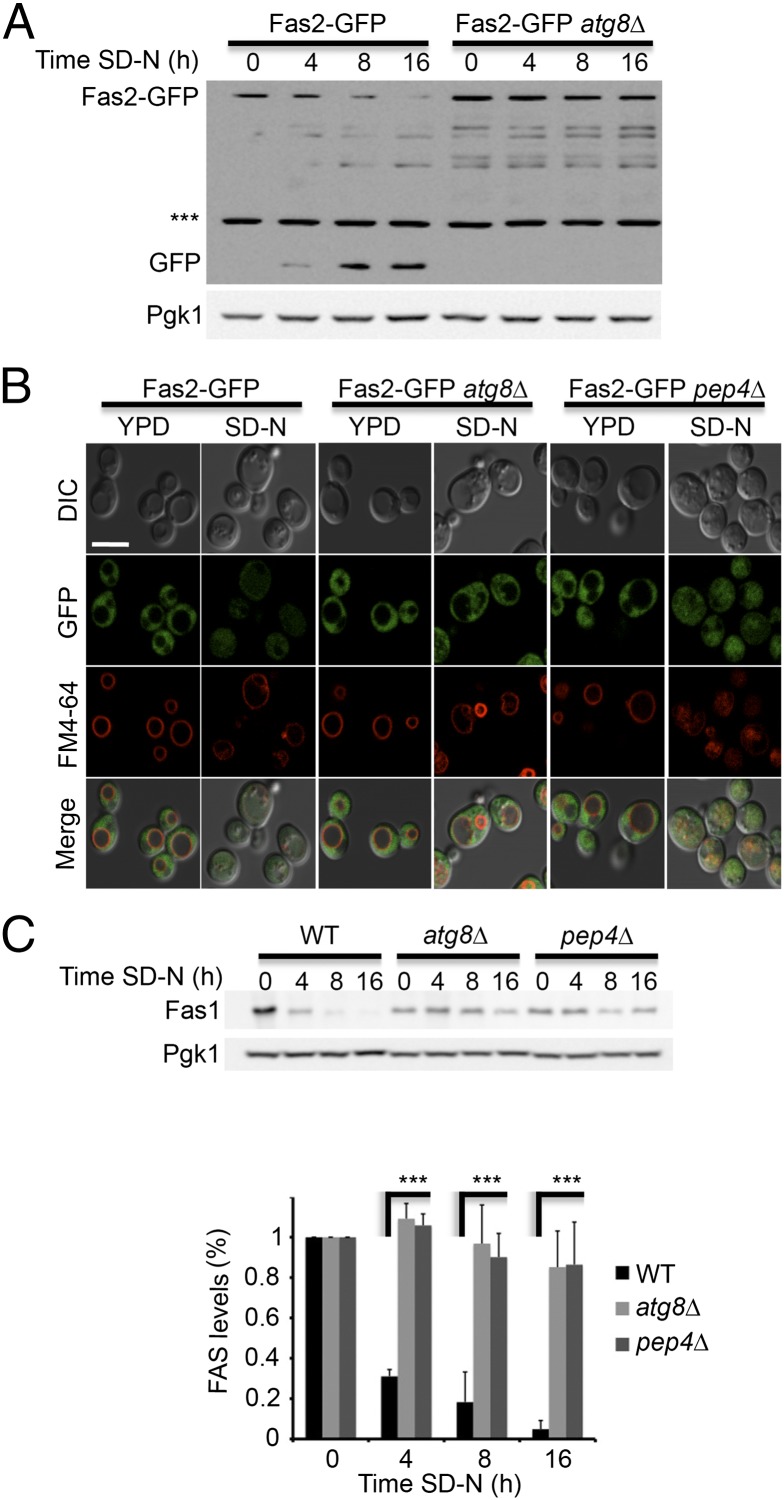
FAS degradation depends on the core autophagy genes. (A) FAS2-GFP (TOS017) and FAS2-GFP atg8 (TOS023) S. cerevisiae cells were grown to midlog phase and shifted to SD-N medium for the indicated time periods. Cell lysates were subjected to SDS/PAGE, followed by Western blot analysis using anti-GFP and anti-Pgk1 antibodies. (B) FAS2-GFP (TOS017), FAS2-GFP atg8
(TOS023), and FAS2-GFP pep4
(TOS022) cells were grown to midlog phase and shifted to SD-N medium. Fas2-GFP localization was monitored in YPD medium and after 16 h in SD-N. FM4-64 was used to detect vacuolar membranes. (Scale bar, 5 µm.) (C) WT (BY4741), atg8
(TOS006), and pep4
(TOS015) cells were grown to midlog phase and shifted to SD-N medium for the indicated time periods. Cell lysates were subjected to SDS/PAGE, followed by Western blot analysis using anti-Fas1 and anti-Pgk1 antibodies. Error bars represent the SDs of three independent experiments. ***P < 0.001 (Student’s t test) (Lower).
Vac8 and Atg24 Are Essential for Efficient Degradation of FAS.
To determine whether the autophagic machinery participates in FAS degradation, we monitored the delivery of FAS to the vacuole in the S. cerevisiae atg8 strain by immunoblotting and fluorescence microscopy. Fas2-GFP in the atg8
strain failed to reach the vacuole and its localization remained cytosolic (Fig. 2 A and B). This result indicated that FAS degradation depends on the core autophagy protein Atg8 and implied that autophagy degrades FAS. To further characterize FAS degradation, we investigated the steady-state levels of endogenous Fas1 at different time points of nitrogen starvation by using Fas1 antibody. After 4 h of nitrogen starvation Fas1 had dropped rapidly in WT cells, and was hardly detectable on Western blot analysis after starvation for 8 h (Fig. 2C). In contrast, Fas1 levels remained relatively stable in the pep4
strain and in the autophagy mutant strain atg8
(Fig. 2C).
The rapid decrease in FAS levels under nitrogen starvation and the need of Atg8 for this process prompted us to examine which autophagy proteins are responsible for its degradation. We did this by monitoring Fas1 levels in rich conditions and under nitrogen starvation in different autophagy-related knockout strains (Fig. S2). Fas1 degradation was inhibited upon deletion of core autophagic genes, but persisted upon deletion of most of the selective autophagy genes, including atg11 (Fig. S2). In contrast, deletion of the selective autophagy-related genes vac8 and atg24 greatly decreased the rate of FAS degradation (Fig. 3).
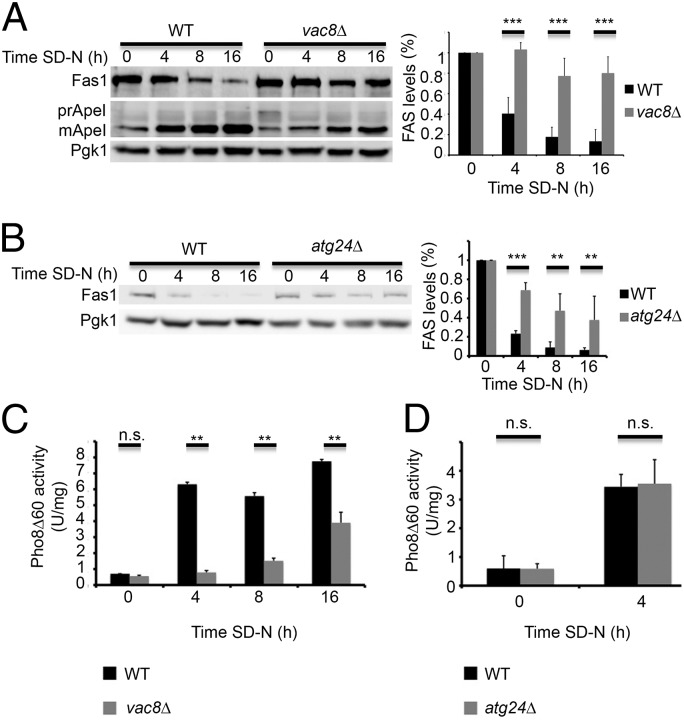
Vac8 and Atg24 are essential for efficient degradation of FAS. (A) WT (BY4741) and vac8 (TOS028) S. cerevisiae cells were grown to midlog phase and shifted to SD-N medium for the indicated time periods. Cell lysates were subjected to SDS/PAGE, followed by Western blot analysis using anti-Fas1, anti-Ape1, and anti-Pgk1 antibodies. Quantifications of three individual experiments are presented on the right. Error bars represent the SDs of three independent experiments. ***P < 0.001 (Student’s t test) (Right). (B) WT (BY4741) and atg24
(TOS012) cells were grown as in A. Cell lysates were subjected to SDS/PAGE, followed by Western blot analysis using anti-Fas1 and anti-Pgk1 antibodies. Quantifications of three individual experiments are presented on the right. Error bars represent the SDs of three independent experiments. **P < 0.01, ***P < 0.001 (Student’s t test) (Right). (C) pho8
::pho8
60 pho13
(TN124) and pho8
::pho8
60 pho13
vac8
(TOS027) cells were grown as in A and shifted to SD-N medium for the indicated time periods. Autophagic activity was measured using the quantitative Pho8
60 assay. Error bars represent the SDs of three independent experiments. n.s., not significant; **P < 0.01, ***P < 0.001 (Student’s t test). (D) pho8
::pho8
60 pho13
(TN124) and pho8
::pho8
60 pho13
atg24
(TOS026) cells were grown as in A and shifted to SD-N medium for the indicated time periods. Autophagic activity was measured using the ALP assay. Error bars represent the SDs of three independent experiments. n.s., not significant (Student’s t test).
Vac8 is a vacuolar membrane protein required for efficient vacuolar fusion and for several selective autophagy processes, including the cytoplasm-to-vacuole targeting (CVT) pathway (11, 12). We found that vac8-knockout strains failed to efficiently degrade FAS upon nitrogen starvation (Fig. 3A and Fig. S3A). Whereas FAS degradation was inhibited, autophagy was active as indicated by delivery of the vacuolar aminopeptidase ApeI to the vacuole (Fig. 3A and Fig. S3 A and B) (12). To determine the effect of vac8 deletion on bulk autophagy, we used the Pho860 assay (14). Pho8
60, a truncated variant of the vacuolar alkaline phosphate Pho8, lacks the N-terminal transmembrane domain. In the absence of this domain the normal translocation of Pho8 into the endoplasmic reticulum is prevented. Pho8
60 accumulates in the cytosol and is delivered to the vacuole for maturation and activation only by nonselective autophagy. Although bulk autophagy was still active in vac8-knockout strains, the activity of Pho8
60 was lower in the vac8
strain than in the WT, as previously reported (Fig. 3C) (12). Thus, we cannot definitively conclude whether the inhibition of FAS degradation in vac8
strains is the outcome of a general decrease in the autophagic flux or a specific effect of Vac8.
The sorting nexin Atg24 reportedly participates in the CVT pathway but not in autophagy (9, 10). We found that the atg24 strain failed to efficiently degrade FAS (Fig. 3B) but maintained its ability to deliver Pho8
60 to the vacuole (Fig. 3D) (9, 10). Moreover, a plasmid expressing Atg24-GFP under the promoter of Atg24 was able to rescue the delivery of FAS to the vacuole in the atg24
knockout strain (Fig. S3C). Of note, the maturation rate of Pho8
60 to the vacuole in WT strains was significantly lower than the degradation rate of FAS (Fig. S3D). Taken together, these results suggest that FAS is selectively delivered to the vacuole upon nitrogen starvation.
FAS Degradation Depends on the Interaction with Atg8.
Atg8 is a key factor in the selective delivery of cargo proteins for degradation. The N-terminal region of Atg8 has been shown to participate in adaptor recruitment and protein recognition (15, 16). In searching for potential cargo or machinery proteins that interact with Atg8, we performed immunoprecipitation experiments on Atg8-null cells transformed with GFP-Atg8 or with GFP-Atg8 lacking the first (GFP-Atg8N8) or both (GFP-Atg8
N24) N-terminal α-helices. As shown in Fig. 4A, a specific high-molecular band that coimmunoprecipitates with the full-length Atg8, but not with its two truncated forms, was identified. Mass spectrometric analysis of the resulting band recognized it exclusively as Fas1 and Fas2 (Fig. S4A). The identity of FAS was confirmed via Western blot analysis with an antibody directed against Fas1 (Fig. 4B) and by reciprocal coimmunoprecipitation using protein A-tagged Fas1 and Fas2 as bait and anti-Atg8 antibody (Fig. 4C). As shown in Fig. 4B and Fig. S4B, FAS was detected only when precipitated with the full-length Atg8, and only the full-length Atg8 precipitated with protein A-tagged FAS (Fig. 4C). It has been previously reported that the unassembled Fas2 subunit is degraded in the proteasome, whereas the unassembled Fas1 subunit is degraded by the vacuole (8). To examine the possibility that Atg8 directly interacts with the Fas1 subunit, recombinant Fas1 fused at its N-terminal to Flag tag was bound to anti-Flag beads and monitored for its ability to pull down recombinant Atg8. As depicted in Fig. 4D, Atg8 from Escherichia coli lysate as well as purified Atg8 interact with recombinant Fas1, suggesting that these proteins interact directly.
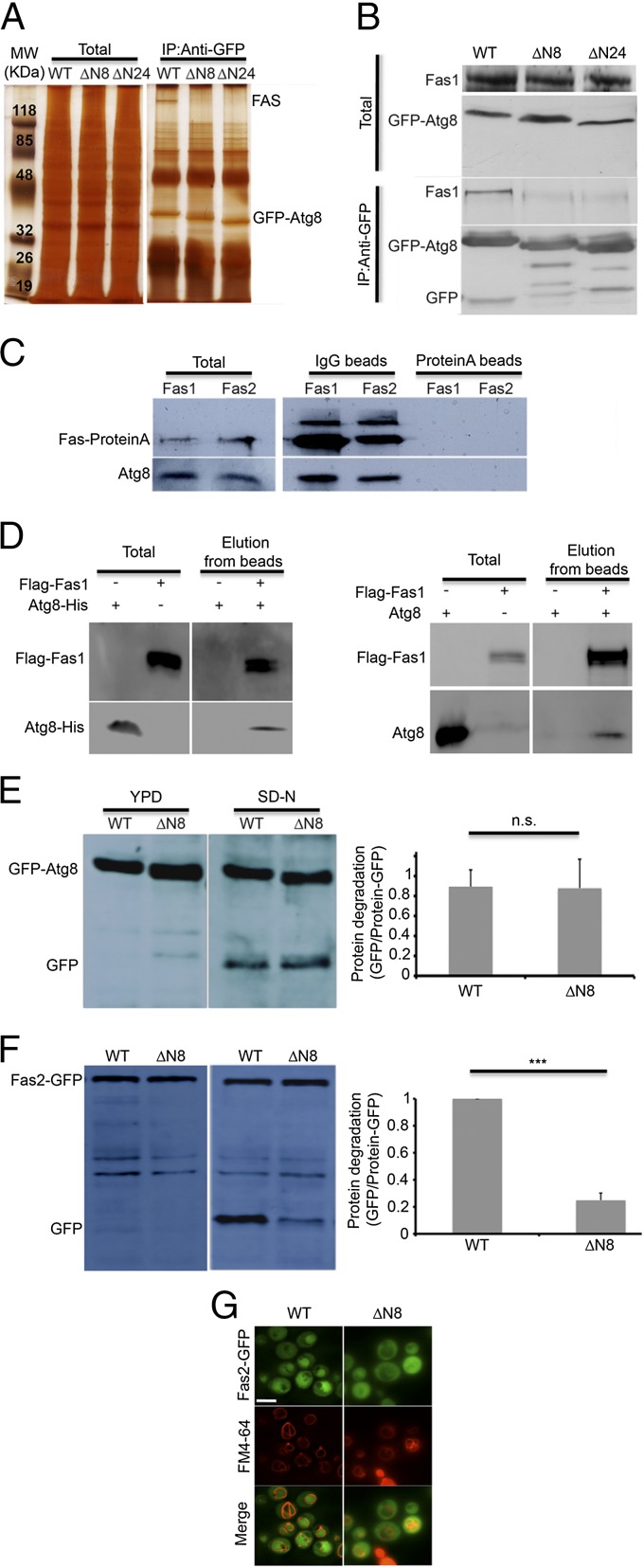
FAS coimmunoprecipitates with Atg8 in an N-terminal-dependent manner. (A) atg8 (TOS006) S. cerevisiae cells expressing pYES-GFP-Atg8, pYES-GFP-Atg8
N8 or pYES-GFP-Atg8
N24 were grown to midlog phase. Cell lysates were subjected to immunoprecipitation using anti-GFP antibodies. The immunoprecipitates were subjected to SDS/PAGE followed by silver staining. Total is 5% of the lysate. (B) atg8
(TOS006) cells expressing pYES-GFP-Atg8, pYES-GFP-Atg8
N8 or pYES-GFP-Atg8
N24 were grown to midlog phase and shifted to SD-N medium for 2 h. Cell lysates were immunoprecipitated using anti-GFP antibodies. Immunoprecipitates were subjected to SDS/PAGE, followed by Western blot analysis using anti-Fas1 and anti-GFP antibodies. Total is 5% of the lysate. (C) Fas1-proteinA (TOS024) and Fas2-proteinA (TOS025) cells were grown to midlog phase. Cell lysates were immunoprecipitated using either IgG or protein A beads. Immunoprecipitates were subjected to SDS/PAGE, followed by Western blot analysis using anti-Fas1 and anti-Atg8 antibodies. Total is 5% of the lysate. (D) Anti-Flag beads were incubated with bacterial lysate (−) or equal amounts of lysate expressing recombinant Flag-Fas1 (+) for 1 h, washed and then incubated with bacterial lysate expressing recombinant Atg8-His for 1 h (Left) or with 4 µg purified Atg8 (Right). Beads were washed and incubated with 75 µg/mL Flag peptide for 2 h. Supernatant was collected (Elution) and subjected to SDS/PAGE, followed by Western blot analysis using anti-Flag, anti-His (Left) and anti-Atg8 (Right) antibodies. Total is 2.5% of the lysate. (E) atg8
(TOS006) cells expressing pRS316-GFP-Atg8 or pRS316-GFP-Atg8
N8 were grown to midlog phase and shifted to SD-N medium for 4 h. Cell lysates were subjected to SDS/PAGE, followed by Western blot analysis using anti-GFP antibodies. Error bars represent the SDs of three independent experiments. n.s., not significant (Student’s t test) (Right). (F) FAS2-GFP atg8
(TOS023) cells expressing pRS316-Atg8 or pRS316-Atg8
N8 were grown to midlog phase and shifted to SD-N medium for 4 h. Cell lysates were subjected to SDS/PAGE, followed by Western blot analysis using anti-GFP antibodies. Error bars represent the SDs of three independent experiments. ***P < 0.001 (Student’s t test) (Right). (G) FAS2-GFP atg8
(TOS023) cells expressing pRS316-Atg8 or pRS316-Atg8
N8 were grown to midlog phase and shifted to SD-N medium. Fas2-GFP localization was monitored after 16 h in SD-N medium. FM4-64 was used to detect vacuolar membranes. (Scale bar, 10 µm.)
To investigate the role of this interaction in FAS degradation, we monitored the GFP processing of FAS in atg8 S. cerevisiae strains expressing either full-length Atg8 or Atg8
N8 under nitrogen starvation. As a control we used atg8
strains expressing GFP-Atg8 or GFP-Atg8
N8. As depicted in Fig. 4E, the truncated form of GFP-Atg8 was readily processed and delivered to the vacuole. In contrast, degradation of FAS-GFP was notably inhibited in strains expressing the truncated form of Atg8, as indicated by GFP cleavage (Fig. 4F) and by fluorescence microscopy (Fig. 4G). Taken together, our results indicate that the interaction between FAS and Atg8 is important for FAS degradation.
FAS Degradation is Essential for Cell Viability Under Nitrogen Starvation.
FAS is an essential enzyme complex whose activity is required for cell survival under growing conditions (17). The rapid degradation of FAS by autophagy prompted us to examine whether this enzymatic complex is essential under nitrogen starvation as well. To this end we generated fas1 and fas2
S. cerevisiae strains, which can grow in rich medium supplemented with saturated fatty acids. Growth of these strains without exogenous fatty acids led to rapid and complete loss of viability (Fig. 5 A and B). In contrast, cells grown overnight under nitrogen starvation conditions in the absence of fatty acids survived (Fig. 5 A and B). These results indicated that although FAS is essential under growing conditions it is not essential for the first 16 h under nitrogen starvation. In addition, treatment of fas
cells with rapamycin partially protected them from loss of viability (Fig. 5 A and B). To validate our results, we used the FAS inhibitor cerulenin (18, 19), which completely blocks FAS activity (Fig. 5E). Under growing conditions treatment with cerulenin led to rapid and complete loss of viability, which was rescued by the addition of fatty acids (Fig. 5C and Fig. S5A). In agreement with the findings in the fas
strain, addition of cerulenin under nitrogen starvation did not lead to loss of viability. In addition, rapamycin was able to rescue cells that were grown under rich conditions in the presence of cerulenin (Fig. 5C and Fig. S5A). These results indicated that nitrogen starvation protects cells from FAS inhibition.
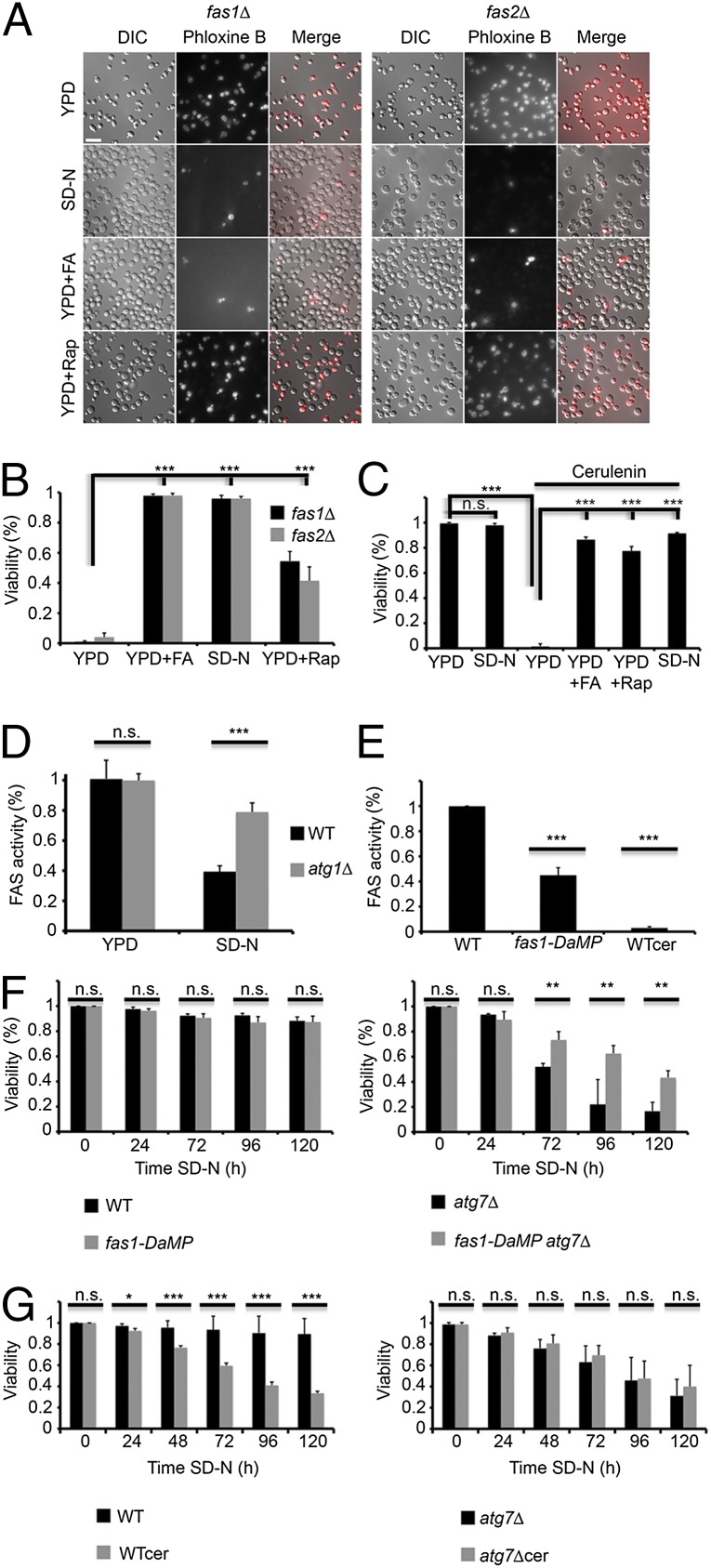
FAS degradation is important for cell survival under nitrogen starvation. (A) fas1 (TOS029) and fas2
(TOS030) S. cerevisiae cells were grown to midlog phase and incubated in YPD, SD-N, YPD + 0.1 mM palmitic/stearic/myristic acids, or YPD + 200 nM rapamycin. Cell viability was determined after 16 h using phloxine B. (Scale bar, 10 µm.) (B) Quantification of A. More than 300 cells were counted for each experiment. Error bars represent the SDs of three independent experiments. ***P < 0.001 (Student’s t test) (Right). (C) WT (BY4741) cells were grown to midlog phase and incubated with DMSO, 50 μM cerulenin (cer) or 50 μM cerulenin + 0.1 mM palmitic/stearic/myristic acids (cer + FA). Cell viability was determined after 16 h using phloxine B. More than 300 cells were counted for each experiment. Error bars represent the SDs of three independent experiments. n.s., not significant; ***P < 0.001 (Student’s t test). (D) WT (BY4741) and atg1
(TOS001) cells were grown to midlog phase and shifted to SD-N medium for 16 h. FAS activity was determined at the indicated time points as described in Materials and Methods. Error bars represent the SDs of three independent experiments. n.s., not significant; ***P < 0.001 (Student’s t test). (E) WT (BY4741) and DaMP-fas1 (TOS031) cells were grown to midlog phase. Cells were treated with either DMSO or 50 μM cerulenin for 30 min. FAS activity was determined as described in Materials and Methods. Error bars represent the SDs of three independent experiments. ***P < 0.001 (Student’s t test). (F) WT (BY4741), atg7
(TOS005), DaMP-fas1 (TOS031) and DaMP-fas1 atg7
(TOS032) cells were grown to midlog phase and shifted to SD-N medium. Cell viability was determined at the indicated time points using phloxine B. More than 300 cells were counted for each experiment. Error bars represent the SDs of three independent experiments. n.s., not significant; **P < 0.01 (Student’s t test). (G) WT (BY4741) and atg7
(TOS005) strains were grown to midlog phase and shifted to SD-N medium in the presence of DMSO or 50 μM cerulenin. Cell viability was determined using phloxine B at the indicated time periods. Error bars represent the SDs of three independent experiments. n.s., not significant; *P < 0.05, **P < 0.01, ***P < 0.001 (Student’s t test).
Next, we used biochemical assays to test the activity of FAS under nitrogen starvation. FAS activity was markedly reduced, but not completely abolished, under nitrogen starvation conditions in WT cells, reaching ~40% after 16 h, whereas it remained relatively constant in the autophagy-deficient strain atg1 (Fig. 5D). These results suggest that under nitrogen starvation autophagy is a major regulator of FAS activity.
To mimic the reduction in FAS activity during nitrogen starvation in autophagy-deficient cells, we took advantage of a strain with decreased abundance by mRNA perturbation (DAmP) of the fas1 gene (20). Examination of the activity of FAS in this strain confirmed a reduction in its activity in comparison with that of WT strains (Fig. 5E). We examined the effect of reduced FAS activity in this strain on the viability of the autophagy-deficient strain atg7. As depicted in Fig. 5F, the DAmP fas1 atg7
survived better under nitrogen starvation than the atg7
strain. Consistent with these results, under nitrogen starvation the vac8
strain (which fails to degrade FAS) lost its viability, whereas the atg24
strain (which degrades FAS at a lower rate) survived (Fig. S5B). Taken together, our results suggest that FAS degradation under nitrogen starvation reduces its activity and is beneficial for cell survival.
FAS activity is reduced under nitrogen starvation; however, it is not completely abolished. The remaining activity of FAS may be important for cell survival. To investigate this possibility, cells were treated with cerulenin and examined for their viability under nitrogen starvation. As depicted in Fig. 5G, cerulenin treatment led to similar losses of viability in the WT and the autophagy-deficient strain atg7, indicating that a delicate balance of FAS is essential for maintaining cell viability.
Discussion
We report here that FAS is preferentially degraded by autophagy under nitrogen starvation. The selective degradation of FAS was dependent on Vac8 and Atg24 and on its interaction with Atg8. Notably, degradation of FAS resulted in a decrease in its activity. Although FAS is an essential protein, we demonstrate that nitrogen starvation protects cells from FAS inactivation. Finally, we provide evidence that FAS degradation is important for cell survival.
The proteasome is responsible for the degradation of short-lived proteins (21). Oligomers are unable to penetrate its limited barrels and may block it (22–24). FAS, being an oligomeric, long-lived enzymatic complex, is therefore targeted to the vacuole for degradation. FAS degradation under potassium acetate starvation was reported to be nonselective (8). Consistently, we found that under potassium acetate starvation FAS degradation was indeed much lower than under nitrogen starvation. Upon nitrogen starvation however, the FAS complex was rapidly degraded and its levels decreased in comparison with that of the nonselective autophagic cargo Pgk1 and to the maturation of Pho860. It therefore seems that different carbon sources regulate selective autophagy processes by a yet uncharacterized mechanism.
Despite considerable advancement in the recognition of selective cargo that undergoes degradation by autophagy, the selective degradation of functional metabolic enzymes is poorly characterized. In yeasts, Atg11 plays a key role in numerous selective autophagy pathways, including the CVT, mitophagy, pexophagy, and piecemeal microautophagy of the nucleus (25). FAS degradation was Atg11-independent but required interaction with Atg8. In addition, we found that Vac8 and Atg24 are essential for efficient degradation of FAS. Vac8 plays a role in several selective autophagy pathways. This protein is not essential for bulk autophagy, although it hampers the autophagic flux, making it difficult to determine whether it participates directly in the degradation of cytosolic proteins including FAS. Nevertheless, Vac8 was recently implicated in the degradation of lipid droplets (26) (organelles that store neutral lipids). Thus, Vac8 may play an important role in the regulation of lipids and fatty acids. Atg24, a sorting nexin that participates in retrograde transport from early endosomes to late Golgi, has also been implicated in several selective autophagy pathways (9, 10). In contrast to Vac8, Atg24 does not hamper bulk autophagy, but is essential for efficient degradation of FAS. The fact that FAS is preferentially degraded and the unique requirement for Atg8, Atg24, and Vac8 in the selective degradation of FAS provides evidence for a new degradation pathway that is induced under starvation conditions to promote cell homeostasis.
Atg8 is part of the machinery responsible for the recruitment of proteins that are delivered for degradation and of proteins that play a role in autophagosome biogenesis (6). Our findings indicated that FAS interacts with Atg8 in an N-terminal–dependent manner. The N terminal of Atg8 reportedly participates in adaptor recruitment and protein recognition (15, 16). FAS degradation was indeed found to be dependent on its interaction with Atg8, further supporting selective degradation.
FAS is an essential enzymatic complex whose inhibition leads to a rapid loss of viability under growing conditions. We showed here that under nitrogen starvation, autophagy is the main process responsible for the reduction in FAS activity. In agreement with a previous report (17), we found that under nitrogen starvation cells cope with loss of FAS activity. These results fit well with the fate of FAS under these conditions. Moreover, we provide evidence that failure to degrade FAS under nitrogen starvation may be toxic to yeasts on one hand, whereas on the other hand low activity of FAS is essential to maintain cell viability. Our results suggest that a delicate balance of FAS levels is required under nitrogen starvation, and we propose that starvation-induced selective autophagy is essential for maintenance of cell homeostasis in yeasts.
Materials and Methods
Reagents, microscopy, coimmunoprecipitation, and mass spectrometry techniques are detailed in SI Material and Methods.
Yeast Strains and Media.
The yeast (S. cerevisiae) strains used in this study are listed in Table S1. Yeast transformation of S. cerevisiae was performed by the lithium acetate method (27). Standard techniques for sporulation, tetrad analysis, and gene disruption in yeast were used as described previously (28). Yeast strains were grown in complete medium [YPD: 1% yeast extract, 2% peptone (wt/vol), 2% dextrose (wt/vol)], or in synthetic defined (SD) minimal medium (0.67% yeast nitrogen base lacking amino acids and nutrients, 2% dextrose (wt/vol) supplemented with amino acids depending on the strain selection), or in nitrogen starvation medium [SD-N: 2% glucose (wt/vol), 0.67% yeast nitrogen base without amino acids or ammonium sulfate].
Assays for Autophagy.
The quantitative Pho860 assay for the measurement of nonselective autophagy was performed as previously described (14). To determine FAS levels and for the GFP-processing assay, cells were grown overnight, diluted to OD 0.2−0.4, and grown to OD 0.8−1. Cells were then washed twice and shifted to nitrogen starvation medium. Cell lysis was performed as described previously (29).
FAS Activity.
Fas activity was assayed as previously described (30). Yeasts were grown to midlog phase, washed twice, and shifted to SD-N medium. At the indicated time points, 10 OD units were harvested (1,500 × g, 5 min) and washed with double distilled water. The pellet was resuspended in ice-cold 0.1 M potassium phosphate buffer pH 7.4 containing protease inhibitors and PMSF. Lysis was performed with glass beads (seven cycles of 1-min vortexing and 1 min cooling on ice). The lysate was centrifuged at 8,000 × g for 20 min at 4 °C. The assay was carried out at room temperature in a mixture containing 0.1 M potassium phosphate pH 6.5, 2 mM EDTA, 10 mM cysteine, 0.2 mM NADPH, 0.05 mM acetyl CoA, 0.3 mg BSA, 20‒40 µg total protein extract, and 0.08 mM malonyl-CoA in a total volume of 1 mL. The decrease in amount of NADPH was measured spectrophotometrically in a quartz cuvette at 340 nm. The blank rate for NADPH oxidation was measured before the addition of malonyl-CoA and was subtracted from the total rate of NADPH oxidation in the presence of malonyl-CoA. Next, 1 mU of FAS is defined as the consumption of 1 µmole of malonyl-CoA/min. FAS activity was measured as mU/mg protein and is presented as a percentage.
Cell Viability.
Cell viability was assayed by phloxine B staining as previously described (31). More than 300 cells were counted for each experiment. The average of three independent experiments is presented.
Plasmid Construction.
Yeast Atg8 was cloned into the pET28-TevH expression vector (32) fused to the C-terminal 6×His-tag or to pGEX-5X-1. Yeast Fas1 was cloned into a derivative of the expression vector pACYCDUet-1 (Novagen), harboring an N-terminal Flag-tag. Cloning of both plasmids was performed using the Restriction Free (RF) cloning strategy (33, 34).
Protein Expression and Partial Purification.
Expression of Atg8 and Fas1 in E. coli BL21(DE3) cells was induced by 200 mM IPTG (isopropyl β-d-1-thiogalactopyranoside) at 15 °C for about 18 h (32). Fas1 clone was purified using anti-DYKDDDDK G1 affinity resin (GenScript, Catalog no. L00432), according to the manufacturer’s instructions. Following the binding of Fas1 to anti-DYKDDDDK G1 affinity resin, the enriched resin was used for detection of Fas1 and Atg8 interaction. GST-Atg8 was purified with glutathione agarose and eluted with Xa factor, as previously described (35).
Atg8−Fas1 Interaction.
Interaction between Atg8 and Fas1 was examined by incubation of a total-cell extract harboring Atg8 or with purified Atg8 with enriched resin of Fas1. Incubation was performed in 1.5-mL tubes at 4 °C for 1 h with gentle rotation. The resins were then extensively washed with buffer containing 50 mM TrisHCl pH 7.5 and 150 mM NaCl for anti-DYKDDDDK G1 affinity resin (Flag-tag resin). Proteins were eluted from the resins with the above buffer containing 75 µg/mL Flag peptide. Patterns of the proteins eluted from the resins were detected by Western blot analysis.
Acknowledgments
Z.E. is the incumbent of the Harold Korda Chair of Biology. This study was supported in part by the Israeli Science Foundation (Grant 535/11), the German-Israeli Foundation (Grant 1129/157), the Legacy Heritage Fund (Grant 1309/13), and the Conseil Pasteur-Weizmann.
Footnotes
The authors declare no conflict of interest.
This article is a PNAS Direct Submission. H.S. is a guest editor invited by the Editorial Board.
This article contains supporting information online at www.pnas.org/lookup/suppl/10.1073/pnas.1409476112/-/DCSupplemental.
References
Articles from Proceedings of the National Academy of Sciences of the United States of America are provided here courtesy of National Academy of Sciences
Full text links
Read article at publisher's site: https://doi.org/10.1073/pnas.1409476112
Read article for free, from open access legal sources, via Unpaywall:
https://www.pnas.org/content/pnas/112/5/1434.full.pdf
Citations & impact
Impact metrics
Citations of article over time
Alternative metrics
Smart citations by scite.ai
Explore citation contexts and check if this article has been
supported or disputed.
https://scite.ai/reports/10.1073/pnas.1409476112
Article citations
Nitrogen starvation leads to TOR kinase-mediated downregulation of fatty acid synthesis in the algae Chlorella sorokiniana and Chlamydomonas reinhardtii.
BMC Plant Biol, 24(1):753, 06 Aug 2024
Cited by: 0 articles | PMID: 39107711 | PMCID: PMC11302099
An APEX2-based proximity-dependent biotinylation assay with temporal specificity to study protein interactions during autophagy in the yeast <i>Saccharomyces cerevisiae</i>.
Autophagy, 20(10):2323-2337, 03 Jul 2024
Cited by: 0 articles | PMID: 38958087 | PMCID: PMC11423678
Receptor-mediated cargo hitchhiking on bulk autophagy.
EMBO J, 43(15):3116-3140, 16 May 2024
Cited by: 2 articles | PMID: 38755257 | PMCID: PMC11294605
MFN2 suppresses the accumulation of lipid droplets and the progression of clear cell renal cell carcinoma.
Cancer Sci, 115(6):1791-1807, 13 Mar 2024
Cited by: 1 article | PMID: 38480904 | PMCID: PMC11145141
Ksp1 is an autophagic receptor protein for the Snx4-assisted autophagy of Ssn2/Med13.
Autophagy, 20(2):397-415, 25 Jan 2024
Cited by: 0 articles | PMID: 37733395 | PMCID: PMC10813586
Go to all (40) article citations
Data
Data behind the article
This data has been text mined from the article, or deposited into data resources.
BioStudies: supplemental material and supporting data
Similar Articles
To arrive at the top five similar articles we use a word-weighted algorithm to compare words from the Title and Abstract of each citation.
The ubiquitin-proteasome system degrades fatty acid synthase under nitrogen starvation when autophagy is dysfunctional in Saccharomyces cerevisiae.
Biochem Biophys Res Commun, 733:150423, 18 Jul 2024
Cited by: 0 articles | PMID: 39053108
Bulk RNA degradation by nitrogen starvation-induced autophagy in yeast.
EMBO J, 34(2):154-168, 02 Dec 2014
Cited by: 77 articles | PMID: 25468960 | PMCID: PMC4337068
Lap3 is a selective target of autophagy in yeast, Saccharomyces cerevisiae.
Biochem Biophys Res Commun, 378(3):551-557, 04 Dec 2008
Cited by: 29 articles | PMID: 19061865
Regulation of autophagy in yeast Saccharomyces cerevisiae.
Biochim Biophys Acta, 1793(9):1413-1421, 22 Jan 2009
Cited by: 78 articles | PMID: 19344676
Review
Funding
Funders who supported this work.
Israeli Science Foundation (1)
Grant ID: 535/11
The German Israeli Foundation (1)
Grant ID: 1129/157
The Legacy Heritage Fund (1)
Grant ID: 1309/13