Abstract
Free full text

Toll/IL-1 domain-containing adaptor inducing IFN-β (TRIF) mediates innate immune responses in murine peritoneal mesothelial cells through TLR3 and TLR4 stimulation
Abstract
Mesothelial cells are composed of monolayer of the entire surface of serosal cavities including pleural, pericardial, and peritoneal cavity. Although mesothelial cells are known to express multiple Toll-like receptors (TLRs) which contribute to trigger innate immune responses against infections, the precise molecular mechanism remains still unclear. In the present study, we investigated the role of Toll/IL-1 domain-containing adaptor inducing IFN-β (TRIF), one of the two major TLRs–adaptor molecules, on innate immune response induced by TLR3 and TLR4 stimulation in murine peritoneal mesothelial cells (PMCs). TRIF was strongly expressed in PMCs and its deficiency led to impaired production of cytokines and chemokines by poly I:C and LPS in the cells. Activation of NF-κB or MAPKs through poly I:C and LPS stimulation was reduced in TRIF-deficient PMCs as compared to the WT cells. TRIF was also necessary for optimal nitric oxide synthesis and gene expression of inducible nitric oxide synthase (iNOS) and IFN-β in PMCs in response to poly I:C and LPS. Furthermore, both Escherichia coli and Pseudomonas aeruginosa induced high level of IL-6, CXCL1, and CCL2 production in PMCs, which was significantly impaired by TRIF deficiency. These results demonstrated that TRIF is required for optimal activation of innate immune responses in mesothelial cells against microbial infections.
1. Introduction
Peritonitis is an inflammation of the peritoneum which lines the inner wall of the abdomen and covers and supports most of abdominal organs. The two major types of life-threatening peritonitis are: (i) primary spontaneous peritonitis, an infection that develops in the peritoneum [1]; and (ii) secondary peritonitis, which usually develops when an injury or infection in the abdominal cavity allows infectious organisms into the peritoneum [2], [3].
Mesothelial cells are monolayer of specialized cells which extends over the entire surface of the three serosal cavity (pleural, pericardial, and peritoneal) and the organs contained within these cavities [4]. The predominant role of the mesothelium is to act as a protective barrier against physical damage and invading pathogens. Recent studies have shown many different roles of mesothelial cells, which include antigen presentation, tumor cell adhesion and growth, initiation and resolution of inflammation, and tissue repair [4]. Under physiologic conditions, the mesothelial cells secrete numerous glycosaminoglycans, proteoglycans, and phospholipids that constitute a glycocalyx surrounding the cells and provide a protective barrier against abrasion and a slippery non-adhesive surface for intracoelomic movement [5]. When faced with an infection, mesothelial cells express specific surface markers that enable them to promote the migration of neutrophils, to interact with extracellular matrix proteins, to present antigens to immune cells, and to produce biologically important molecules such as proinflammatory cytokines, chemokines, and nitric oxide (NO) [6], [7].
Toll-like receptors (TLRs) are type I transmembrane proteins and comprise an ectodomain, which contains leucine-rich repeats that mediate the recognition of pathogen-associated molecular patterns (PAMPs); a transmembrane region; and a cytosolic Toll/IL-1 receptor (TIR) domain that activates downstream signaling pathways [8]. Recognition of microbial components (e.g. LPS, lipoprotein, flagellin, and nucleic acids) by TLRs initiates inflammatory signal cascades via two distinct pathways: (i) myeloid differentiation primary response 88 (MyD88)-dependent; and (ii) Toll/IL-1 receptor domain-containing adaptor inducing IFN-beta (TRIF)-dependent pathways. MyD88 is an adapter molecule that triggers inflammatory signals commonly utilized by various TLRs with the exception of TLR3. Recruitment of MyD88 leads to the activation of nuclear factor-kappa B (NF-κB) and mitogen activated protein kinases (MAPKs) to regulate the pro-inflammatory cytokines genes. On the other hand, TRIF is recruited to TLR3 and TLR4 and activates an alternative pathway that triggers the activation of NF-κB, MAPKs, and IRF3. These signaling cascades lead to the production of proinflammatory cytokines, type I interferons (IFNs), chemokines, and antimicrobial peptides to remove the invading pathogens [9], [10].
Mesothelial cells have been known to express multiple TLRs [11], [12]. TLR1–6 are expressed in murine peritoneal mesothelial cells (PMCs) [12] and each ligand induce the production of chemokines such as CXCL1 and CCL2 [13]. In human PMCs, TLR3 is functionally expressed and its agonist poly I:C leads to the production of IL-6, CCL2, and CCL5 [14], [15]. The role of TLR4 is somewhat controversial. Colmont et al. demonstrated that TLR4 was not expressed in primary cultured human PMCs and LPS also did not induce any inflammatory molecules tested [11], whereas it was strongly expressed in a cell line (HMrSV5 cells) and LPS-induced autophagy was dependent on TLR4 in the cells [16], [17]. Bacterial and viral peritonitis can occur in patients with intestinal perforation or undergoing continuous ambulatory peritoneal dialysis (CAPD) [18], [19]. Corona virus and herpes simplex virus can cause peritonitis in IFN-γ-deficient mice and diabetics patient [20], [21]. In addition to its role in response to viral infection, TLR3 is involved in immune response to bacterial infection-induced tissue damage which is one of the major cause of peritoneal inflammation [22], [23], [24]. Therefore, TLR3 and TLR4-mediated signal pathways are considered to play central roles in activation of host immune system in response to various pathogenic microbes in PMC. Although TRIF is the only adaptor molecule mediating both TLR3 and TLR4 signaling pathways, investigations on the detailed function of TRIF in mesothelial cells have not been available. To address this limitation, in the current study, we characterized molecular mechanisms of TRIF-mediated innate immune response in murine PMCs.
2. Methods
2.1. Reagents and bacterial strains
Polyinosinic–polycytidylic acid (poly(I:C)) and ultrapure LPS were purchased from InvivoGen (San Diego, CA). Escherichia coli O111:B4 and Pseudomonas aeruginosa were grown overnight in Luria Bertoni (LB) broth grown anaerobic conditions at 37 °C. The culture was centrifuged at 3000 rpm for 15 min, and the cell pellet was washed twice with cold PBS. The pellet was suspended in one-tenth the original volume in PBS and the OD600 nm was adjusted to give the approximate desired inocula. The inoculum concentrations were verified by serial 10-fold dilutions of the bacterial suspensions. The bacterial concentrations of the suspensions were adjusted to 1 × 109 CFU/ml. The bacteria were diluted to the desired concentration and used in subsequent experiments.
2.2. Preparation of murine PMCs and BMDMs
TRIF-deficient mice on a C57BL/6 background were kindly provided by Dr. Shizuo Akira (Osaka University, Japan). Wild-type (WT) C57BL/6 mice were purchased from Koatech (Pyeongtaek, Korea). Bone marrow-derived macrophages (BMDMs) were prepared as previously described [25]. Mesothelial cells were prepared from the peritoneum and external surfaces of the liver, spleen, and kidney of adult mice as previously described [13]. Briefly, samples of peritoneum and intact organs were obtained from sacrificed mice and digested with 0.25%-trypsin–EDTA (Invitrogen, Grand Island, NY, USA) solution for 50 min at 37 °C. Intact tissues and tissue debris were discarded and the cell suspension was centrifuged at 90 g for 5 min. The pellet was resuspended in Dulbecco’s modified Eagle’s medium supplemented with 15% heat-inactivated FBS and 1% penicillin/streptomycin and cultured overnight. The next day, floating cells were removed by washing twice with PBS and adherent cells were cultured for five additional days. Mesothelial cells were used between passages 2 and 4.
2.3. RT-PCR
Total RNA was extracted from the cells using easyBLUE (Intron Biotechnology, Daejeon, Korea) according to the manufacturer’s instruction. One microgram of total RNA was reverse transcribed into cDNA and PCR was performed using the Power cDNA Synthesis kit (Intron Biotechnology) and One-step RT-PCR with AccuPower®HotStart PCR PreMix (Bioneer, Daejeon, Korea). The following primer sets were used. Mouse TLR2 forward, 5′-GTGGTACCTGAGAATGATGTGGG-3′; mouse TLR2 reverse, 5′-GTTAAGGAAGTCAGGAACTGGGTG-3′; mouse TLR3 forward, 5′-AGGTACCTGAGTTTGAAGCGAGC-3′; mouse TLR3 reverse, 5′-GAGCATCAGTCTTTGAAGGCTGG-3′; mouse TLR4 forward, 5′-CTGGGTGAGAAATGAGCTGG-3′; mouse TLR4 reverse, 5′-GATACAATTCCACCTGCTGCC-3′; mouse TRIF forward, 5′-ATGGATAACCCAGGGCCTT-3′; mouse TRIF reverse, 5′-TTCTGGTCACTGCAGGGGAT-3′; mouse iNOS forward, 5′-CAGCCCAACAATACAAGATGACCC-3′; mouse iNOS reverse, 5′-CAGTTCCGAGCGTCAAAGACCTGC-3′; mouse IFN-β forward, 5′-ATGAACTCCAGCAGACAG-3′; mouse IFN-β reverse, 5′-ACCACCATCCAGGCGTAGC-3′; mouse GAPDH forward, 5′-GTCGGAGRCAACGGATT-3′; mouse GAPDH reverse, 5′-AAGCTTCCCGTTCTCAG-3′. The PCR reaction condition included pre-denaturing at 94 °C for 30 s, then 35–40 cycle of 56 °C for 30 s, 72 °C for 1 min. PCR products were then electrophoresed on a 1.5% agarose gel and visualized using a gel documentation system.
2.4. Measurement of the production of cytokines and nitric oxide (NO)
The concentration of IL-6 and CXCL1, CCL2 in the culture supernatants was determined using a commercial ELISA kit (R&D Systems, Minneapolis, MN, USA). NO synthase activity in the supernatant of cultured cells was assayed for nitrite accumulation by the Griess reaction [26].
2.5. Western blot
PMCs (5 × 104/well) and BMDMs (2 × 106/well) were plated in 35 mm culture dishes. The cells were treated with polyI:C (100 μg/ml) or LPS (100 ng/ml) were lysed in buffer containing 1% Nonidet-P40 supplemented with a complete protease inhibitor cocktail (Roche Diagnostics Ltd, Mannheim, Germany), and 2 mM dithiothreitol. Lysates were resolved by 10% SDS–PAGE, transferred onto a polyvinylidene fluoride (PVDF) membrane, and immunoblotted with primary antibodies against regular- and phospho-IκB-α, JNK (Cell Signaling Technology Inc., Beverly, MA, USA), p38, ERK, β-actin, Calretinin (Santa Cruz Biotechnology, Santa Cruz, CA). After immunoblotting with secondary antibodies, proteins were detected with an enhanced chemiluminescence (ECL) reagent (Intron Biotechnology).
2.6. Flow cytometry
PMCs’s phenotypes were characterized by their cell surface markers using fluorescently labeled monoclonal antibodies (mAbs) and analyzed by flow cytometry. The cells were stained with the following mAbs (eBioscience, San Diego, CA, USA): PE-cy7-congugated mAb. Isotype-matched controls were run in parallel. Cell debris was eliminated by forward and side scatter gating. The samples were acquired on a FACS Calibur cell sorter (Becton Dickinson, Mountain view, CA, USA) and the data was analyzed using FlowJo software (Tree Star, Inc., Ashland, OR, USA).
2.7. Statistic analysis
The differences among the mean values of the different groups were tested and the values were expressed as the mean ± SD. All of the statistical calculations were performed by one-way ANOVA followed by the Bonferroni post hoc test for multigroup comparisons using Graph Pad Prism version 5.01 (Graph Pad Software, San Diego, CA). Values of P < 0.05 were considered significant.
3. Results
3.1. TRIF is expressed in murine PMCs
To determine phenotypic characteristics of PMCs, the expression of calretinin that is known as a selective marker for mesothelial cells [27], [28] was evaluated by Western blot. Calretinin was strongly expressed in PMCs, but there was no expression in BMDMs (Fig. 1 a). Flow cytometry analysis also showed that 98.6% cells in BMDMs preparation was positive for F4/80, which is a well-known marker of murine macrophages population [29], whereas only 2.22% was positive in the PMCs preparation (Fig. 1b). The gene of TLR2, TLR3, and TLR4 was strongly expressed in PMCs as well as BMDMs (Fig. 1c), which was consistent with a study Kato et al. showing that TLR1–6 were strongly expressed in PMCs derived from C3H/HeN mice [12]. Moreover, as shown in Fig. 1d, the TRIF gene was highly expressed in the WT PMCs at levels comparable to the WT BMDMs. As expected, the TRIF gene expression was not detected in both TRIF-deficient BMDMs and PMCs (Fig. 1d).
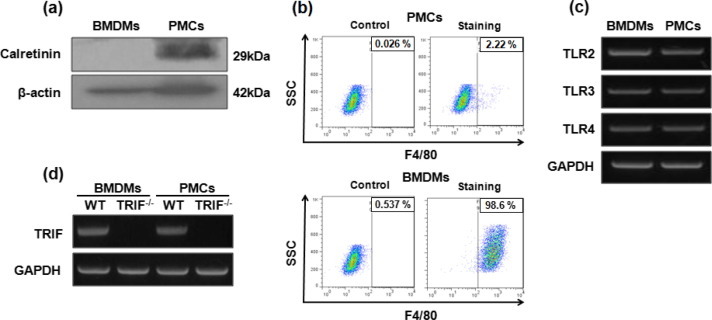
Phenotypic characterization of PMCs and BMDMs and gene expression of TLRs and TRIF. Western blot analysis was performed to determine the expression of calretinin in PMCs and BMDMs (a). The expression of F4/80 surface markers in PMCs and BMDMs was analyzed by flow cytometry (b). The mRNA expression levels of TLR2, TLR3 and TLR4 was determined by RT-PCR in WT BMDMs and PMCs (c). The gene expression of TRIF was also examined in both WT and TRIF-deficient cells (d).
3.2. TRIF is differently involved in the production of IL-6, CXCL1, and CCL2 in murine PMCs in response to poly I:C and LPS
To assess levels of IL-6, CXCL1, and CCL2 secretion in response to poly I:C or LPS, ELISA assays were performed on supernatants derived from the WT and TRIF-deficient mice. Both poly I:C and LPS increased the IL-6, CXCL1, and CCL2 secretion in the WT PMCs in a dose-dependent manner (Fig. 2 a–f). In contrast to the WT PMCs, poly I:C-induced production of IL-6 and CXCL1 was completely abolished in TRIF-deficient PMCs (Fig. 2a and b). Furthermore, CCL2 production by poly I:C was also partially impaired in TRIF-deficient PMCs (Fig. 2c). Although TRIF deficiency did not affect LPS-induced production of CXCL1 and CCL2 in PMCs (Fig. 2e and f), level of IL-6 was decreased significantly with LPS stimulation as compared to the WT PMCs. These findings suggest that TRIF may distinctly regulate cytokines and chemokines production in PMCs in response to TLR3 and TLR4 stimulators.

TRIF differently regulates IL-6, CXCL1, and CCL2 production induced by poly I:C and LPS in PMCs. WT and TRIF-deficient PMCs were incubated with indicated doses of poly I:C or LPS for 24 h. IL-6 (a, d), CXCL1 (b, e) and CCL2 (c, f) in supernatant were measured by ELISA. The data were from one representative result of three independent experiments and are presented as the means ± SD. P < 0.05 and
P < 0.001.
3.3. TRIF differently mediates NF-κB and MAPKs activation in PMCs in response to poly I:C and LPS
NF-κB and MAPKs are essential for chemokines production in PMCs in response to bacterial infection [30]. Accordingly, we investigated whether TRIF is involved in the activation of NF-κB and MAPKs in PMCs in response to poly I:C or LPS. Poly I:C stimulation led to degradation of IκB-α and its phosphorylation in WT PMCs, but not in TRIF-deficient cells (Fig. 3 a). Phosphorylation of p38, JNK, and ERK MAPKs was also detected in both WT and TRIF-deficient PMCs in response to poly I:C at 15 min after treatment (Fig. 3a). However, at 60 min after treatment, such phosphorylation was much strongly detected in WT PMCs, whereas it was gradually diminished in TRIF-deficient cells (Fig. 3a). LPS-induced phosphorylation of IκB-α was found in WT PMCs since 15 min after treatment, whereas it was detected only at 60 min after treatment in TRIF-deficient cells (Fig. 3b). IκB-α degradation was also found only in WT PMCs at 60 min after treatment (Fig. 3b). In contrast, kinetics of phosphorylation of p38, JNK, and ERK MAPKs were similar in both WT and TRIF-deficient PMCs in response to LPS, although densities of phosphorylated JNK and ERK were slightly stronger in TRIF-deficient cells at 15 min after stimulation (Fig. 3b). These findings suggest that TRIF may differently mediate the activation of NF-κB and MAPKs in PMCs depending on upstream TLRs.
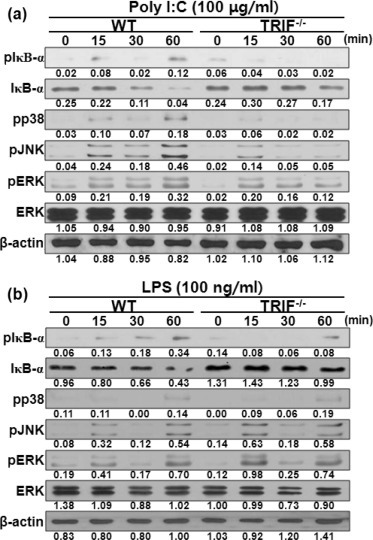
TRIF is critical for activation of NF-κB and MAPKs in PMCs in response to poly I:C or LPS. WT and TRIF-deficient PMCs were stimulated with 100 μg/ml of poly I:C (a) or 100 ng/ml LPS (b) and cellular proteins from stimulated PMCs were extracted at the indicated time points. IκB-α degradation and the phosphorylation of IκB-α, p38, JNK, and ERK were examined by Western blotting. Primary antibodies against total ERK and β-actin were used to verify equal loading. The results are from one representative experiment of two independent experiments.
3.4. TRIF is involved in iNOS expression and NO production by poly I:C or LPS in murine PMCs
The inducible form of nitric oxide synthase (iNOS) is generally associated with NO production related to immune responses [31]. NO synthesis is enhanced by certain types of cytokines and bacterial product in mesothelial cells [13], [32]. We thus assessed whether stimulation of TLR3 and TLR4 promotes iNOS expression and NO synthesis in a TRIF dependent manner in PMCs. As shown in Fig. 4 a, poly I:C-induced expression of iNOS was detected at 4 h after stimulation and continuously increased by 24 h in the WT PMCs. In contrast, the iNOS gene expression was not detected until 24 h post-stimulation in TRIF-deficient cells (Fig. 4a). LPS could induce strong iNOS expression in both WT and TRIF-deficient PMCs at 4 h after stimulation (Fig. 4b). However, the high level of gene expression persisted only in the WT PMCs through 24 h post-stimulation, whereas it was gradually diminished in TRIF-deficient cells after 4 h time point (Fig. 4b).
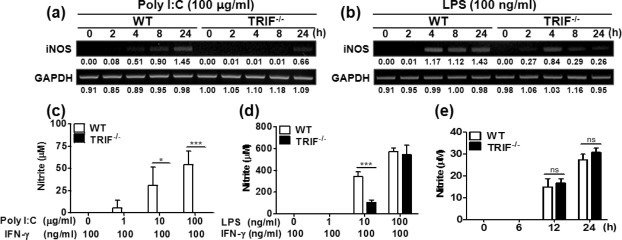
TRIF is required for iNOS expression and NO production by poly I:C or LPS stimulation in PMCs. PMCs isolated from WT and TRIF-deficient mice were incubated with poly I:C and LPS. mRNA was extracted from the cells at indicated time points and iNOS expression was examined by RT-PCR (a, b). Indicated levels were obtained by dividing densities of IFN-β by those of GAPDH (a, b). At the present of IFN-γ (100 ng/ml), the cells were incubated with various doses of poly I:C (c) and LPS (d) for 48 h and nitrite concentration in culture supernatants was measured. An additional experiment with stimulation of LPS (100 ng/ml) and IFN-γ (100 ng/ml) was performed at earlier time points (e). The data (c–e) were from one representative result of three independent experiments and are presented as the means ± SD. P < 0.05 and
P < 0.001.
We next examined NO synthesis by measuring nitrite concentration. Although poly I:C and LPS induced iNOS gene expression (Fig. 4a and b), either poly I:C or LPS alone failed to induce NO synthesis (data not shown). Previously, it has been shown that IFN-γ modulates NO synthesis by mesothelial cells in response to bacterial products such as LPS and KF1B (a Nod1 ligand) [13], [32]. We then measured nitrite concentration in culture supernatant of PMCs stimulated by poly I:C or LPS in the presence of IFN-γ (100 ng/ml). In our pilot experiments, nitrite was not detectable in PMCs in response to any dose of poly I:C (1–100 μg/ml) by 24 h post-stimulation (data not shown). Thus, culture supernatant was collected at 48 h post-stimulation and nitrite was measured. As shown in Fig. 4c–e, IFN-γ alone could not induce NO synthesis in PMCs at any time points, which is consistent with a study by Park et al. [13]. Poly I:C induced NO synthesis in the WT PMCs in a dose-dependent manner at 48 h time point, while the TRIF-deficient cells exhibited no detectable level of NO (Fig. 4c). Similarly, LPS also induced NO synthesis in PMCs in a dose dependent manner. Interestingly, NO synthesis was significantly reduced in the TRIF-deficient PMCs in response to 10 ng/ml of LPS as compared to the WT cells (Fig. 4d). However, the impaired production of NO at 10 ng/ml of LPS was restored at high concentration of LPS (100 ng/ml) in the TRIF-deficient PMCs (Fig. 4d). Since iNOS expression was impaired in TRIF-deficient PMCs when treated with same concentration of LPS (100 ng/ml), we measured nitrite at earlier time points (6–24 h). Similarly, there was no significant difference in nitrite production between WT and TRIF-deficient PMCs in response to 100 ng/ml LPS (Fig. 4e). These finding suggest that TRIF may be critical for TLR3-mediated NO production in PMCs and its influence on NO production induced by TLR4 activation may depend on concentration of stimuli.
3.5. TRIF is required for IFN-β gene expression in PMCs stimulated by poly I:C or LPS
TRIF is a well-known MyD88-independent pathway responsible for expression of IFN-β in immune cells stimulated by poly I:C or LPS [33]. In addition, poly I:C can induce gene expression of type I IFNs in human mesothelial cells [14]. Therefore, we investigated whether TRIF is required for IFN-β gene expression in PMCs in response to poly I:C and LPS. RT-PCR analyses revealed that poly I:C strongly induced mRNA expression of IFN-β in WT PMCs from 2 h post-stimulation, which lasted until 24 h time point (Fig. 5 a). In contrast, the gene expression by poly I:C was significantly reduced in TRIF-deficient cells (Fig. 5a). LPS-induced expression of IFN-β peaked at 2 h post-stimulation in WT PMCs, but was not induced in TRIF-deficient cells (Fig. 5b).
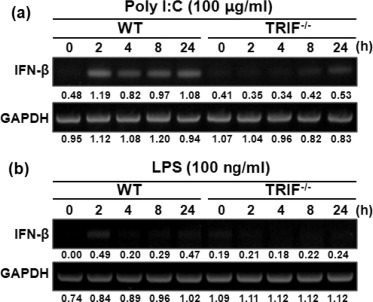
TRIF deficiency leads to impaired gene expression of IFN-β in PMCs in response to poly I:C or LPS. WT and TRIF-deficient PMCs were stimulated with 100 μg/ml of poly I:C (a) or 100 ng/ml of LPS and mRNA was extracted from the cells at indicated time points. Gene expression of IFN-β was assessed by RT-PCR. Indicated levels were obtained by dividing densities of IFN-β by those of GAPDH (a, b).
3.6. TRIF is involved in optimal production of IL-6, CXCL1, and CCL2 by PMCs in response to live P. aeruginosa and E. coli
Live bacteria possess multiple PAMPs including lipoprotein, dsRNA, and LPS. We wondered the role of TRIF on innate immune response against live bacteria. P. aeruginosa and E. coli are the most frequent bacteria causing Gram-negative peritonitis [34]. Accordingly, we finally sought to determine the effect of TRIF on production of IL-6, CXCL1, and CCL2 by live P. aeruginosa and E. coli in PMCs. As shown in Fig. 6, unlike the WT PMCs, IL-6 production was completely impaired in TRIF-deficient PMCs infected with low number of P. aeruginosa (MOI 0.1) or E. coli (MOI 1). Although TRIF-deficient cells could produce IL-6 under higher MOIs of the bacterial pathogens, the level was significantly lower than that of the WT cells (Fig. 6a and b). These data were well correlated with the result of LPS response shown in Fig. 2d. Interestingly, the production of CXCL1 and CCL2 by P. aeruginosa and E. coli was also impaired in TRIF-deficient PMCs, as compared to the WT PMC level (Fig. 6 c–f). These findings indicate the existence of more complex mechanisms in TRIF-mediated signaling pathways in response to bacterial infections.
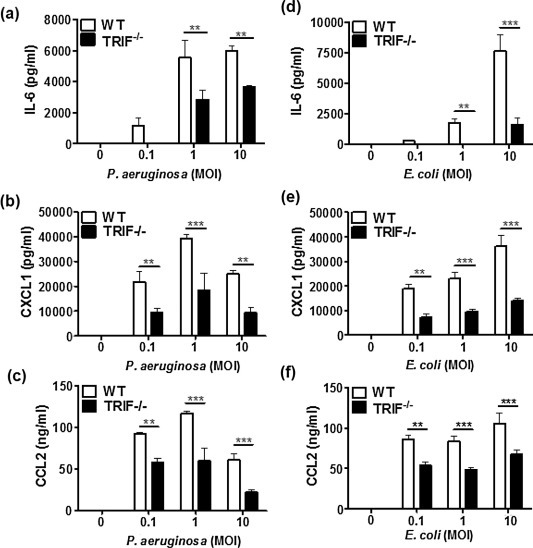
TRIF is essential for optimal production of IL-6, CXCL1, and CCL2 induced by live P. aeruginosa and E. coli in PMCs. WT and TRIF-deficient PMCs were stimulated with different MOIs of P. aeruginosa (a–c) and E. coli (d–f) for 6 h. The level of IL-6, CXCL1, and CCL2 in culture supernatants was measured by ELISA. The data were from one representative result of three independent experiments and are presented as the means ± SD. P < 0.01 and
P < 0.001.
4. Discussion
Peritonitis is a common cause of pathology and mortality in humans. Mesothelial cells are a sentinel for inflammatory response of the mesothelium caused by bacterial products or infection, however, the precise mechanism by which mesothelial cells trigger innate immune responses against microbial infection still remains to be elucidated. In the present study, we provided evidences that TRIF is essential for optimal induction of innate immune responses in response to TLR3 and TLR4 stimulation in PMCs. Production of IL-6 and CXCL1 by PMCs in response to poly I:C was entirely TRIF-dependent. However, poly I:C could induce CCL2 in TRIF-deficient PMCs, although the amount was still lower than that of WT cells, indicating the existence of a TRIF-independent pathway responsible for poly I:C-induced CCL2 production in mesothelial cells. A previous study revealed expression of cytosolic receptors such as RIG-I and MDA5 in human mesothelial cells [14], which are responsible for cytosolic recognition of nucleic acids. Inhibition of RIG-I using siRNA reduced gene expression and protein production of CCL2 induced by poly I:C in mesothelial cells [14]. In addition, although its expression and functionality have not been studied in mesothelial cells, CD11b/CD18 (Mac-1) should be considered as a receptor responsible for poly I:C-induced immune responses in PMCs, because extracellular dsRNA activates Mac-1 to enhance TLR3-dependent signaling and to trigger inflammatory oxidative signaling in macrophages via Mac-1-dependent pathway [35].
Bacterial LPS can also lead to the production of various chemokines such as CXCL1, CXCL2, and CCL2 in mesothelial cells [12], [13]. In the current study, we demonstrated that TRIF is not required for LPS-induced production of CXCL1 and CCL2 in murine PMCs, whereas IL-6 production by LPS was partially impaired in TRIF-deficient PMCs. Pro-inflammatory genes expression induced by LPS seems to be differently regulated via MyD88- or TRIF-dependent signaling. In macrophages, expression of Ccl5 and Cxcl10 by LPS stimulation was entirely TRIF-dependent [36]. In contrast, genes regulated by MyD88 alone included Tnf, Il1b, Cxcl1, Cxcl2, and Ccl [36]. LPS-induced Il6 expression required both MyD88 and TRIF [36]. Ccl2 mRNA expression was also enhanced by LPS in WT macrophages, which was absolutely abolished in MyD88-deficient cells [37]. These published data are in line with our results in PMCs. Taken together, our poly I:C induction data suggest that the production of specific cytokines and chemokines seem to be regulated in differentially based on types of stimulators or associated receptor signaling pathways.
TRIF is essential for poly-induced NF-κB activation in lung fibroblasts [38], which is consistent with our results showing that the activation of NF-κB and MAPKs induced by poly I:C was impaired in TRIF-deficient PMCs. LPS-induced activation of macrophages was temporally regulated by MyD88 and TRIF [33]. MyD88 was involved in early activation of NF-κB and MAPKs, whereas late phase activation of such molecules was controlled by TRIF-dependent pathway [38], [39], [40]. LPS stimulation also resulted in almost normal activation of NF-κB and JNK in TRIF-deficient lung fibroblasts [38]. Moreover, NF-κB and MAPKs activation in response to LPS was delayed in MyD88-deficient cells [39], [40] and diminished in TRIF-deficient macrophages at late time of stimulation [41]. Accordingly, we predicted that LPS-induced activation of NF-κB and MAPKs may not be different between the WT and TRIF-deficient PMCs at early time. However, in this study, NF-κB activation by LPS was delayed in TRIF-deficient PMCs, although impact of TRIF on LPS-induced MAPKs activation was very limited, suggesting that TRIF may be involved in rapid activation of NF-κB in PMCs. Future studies will need to determine whether this inconsistency is due to difference in cell types.
NO production in macrophages is important for the control of bacterial clearance [42]. It also has antiviral property by inhibiting a viral protease [43]. Moreover, NO participates in intraperitoneal inflammatory reaction and is involved in the modulation of peritoneal permeability during peritonitis [44]. Endothelial cells of peritoneal vasculature or peritoneal macrophages are considered as major source of local NO production during peritonitis [45], [46]. However, there are evidences showing that mesothelial cells can produce NO after stimulation by combination of cytokines and bacterial products [13], [32], [47]. The current study for the first time demonstrates that dsRNA can induce iNOS gene expression and NO production in PMCs via a TRIF-dependent manner, which is consistent with a previous study showing that poly I:C induces intracellular reactive oxygen species (ROS) in macrophages via TLR3- and TRIF-dependent manner [48]. In addition, contribution of TRIF to LPS-induced NO synthesis in PMCs was specific dose-dependent response. NO synthesis was impaired in TRIF-deficient PMCs in response to 10 ng/ml LPS, whereas high dose LPS (100 ng/ml) led to synthesis of comparable level of nitrite between the WT and TRIF-deficient PMCs. Unreasonably, at this concentration, LPS-induced expression of iNOS was diminished in TRIF-deficient PMCs at late time points (8 and 24 h), as compared to the WT cells. It is likely that early induction of iNOS is sufficient for optimal NO synthesis by LPS in PMCs. Moreover, although MyD88 is dispensable for endotoxin-induced nitrite synthesis in a human macrophage cell line [49], its role on PMCs should not be disregarded. The precise mechanism of LPS-induced NO synthesis in PMCs remains to be elucidated. Future studies will be designed to adjudicate these limitations.
Type I IFNs (IFN-α/β) play important roles in both innate and adaptive antiviral immune responses [50]. Gene expression of type I IFNs is enhanced by poly I:C in mesothelial cells [14]. In current study, we provided evidence that TRIF is critical for poly I:C-induced gene expression of IFN-β, which is in agreement with previous studies in other cell types such as epithelial cells and dendritic cells [51], [52]. Remarkably, IFN-β expression by poly I:C was detected in TRIF-deficient PMCs at 24 h time point after infection, indicating that TRIF is required for earlier expression of type I IFNs by poly I:C and TRIF-independent pathway may contribute to their expression in late response in PMCs. Involvement of RIG-I and MDA5 in such a late response should be clarified, as both cytosolic receptors are functionally expressed in mesothelial cells [14], [53], [54]. In addition, as expected, LPS induced IFN-β expression in WT PMCs in a TRIF-dependent manner.
In macrophages, TRIF is required for cytokines and chemokines production in response to P. aeruginosa and E. coli infections [41], [55]. In the present study, IL-6, CXCL1, and CCL2 production was impaired in TRIF-deficient PMCs, which was inconsistent with the LPS-induced responses, as TRIF deficiency did not affect CXCL1 and CCL2 production in PMCs under LPS stimulation. One possibility is that live bacteria may possess other molecules to stimulate TLR4-independent and TRIF-mediated signaling. More recent studies also revealed that TRIF is involved in TLR2-mediated immune responses [56], [57]. In addition, TRIF mediates TLR5-induced signalings such as NF-κB and MAPKs activation in intestinal epithelial cells [58]. As TLR2 and TLR5 ligands are known to induce chemokines production in mesothelial cells [11], [13], it is likely that TRIF mediates multiple TLRs-mediated immune responses in mesothelial cells. Extracellular RNA released from necrotic cells can also induce various cellular signalings in host cells via TLR3-dependent manner. Necrotic macrophages and cardiomyocytes induced robust production of cytokines and chemokines in rat cardiomyocytes, which was impaired TRIF-deficient cells [59]. However, Eigenbrod et al. demonstrated that TRIF is not required for CXCL1 production in mesothelial cells in response to necrotic cell extracts [60].
In conclusion, our results showed that TRIF is essential for TLR3- and TLR4-mediated innate immune responses in PMCs. In addition to the roles in inflammatory response, mesothelial cells are involved in the process of tissue repair, antigen presentation, and tumor growth and dissemination [4], [7]. At present, we are attempting to define the role of pattern recognition receptors in the context of diverse physiological role of mesothelial cells.
Conflicts of interest
The authors declare no financial or commercial conflict of interest.
Acknowledgement
This study was financially supported by Chonnam National University, South Korea (Grant No. 2014-2026).
References
Citations & impact
Impact metrics
Citations of article over time
Article citations
Mechanisms of mesothelial cell response to viral infections: HDAC1-3 inhibition blocks poly(I:C)-induced type I interferon response and modulates the mesenchymal/inflammatory phenotype.
Front Cell Infect Microbiol, 14:1308362, 27 Feb 2024
Cited by: 0 articles | PMID: 38476167 | PMCID: PMC10927979
Inhibition of LPS-mediated TLR4 activation abrogates gastric adenocarcinoma-associated peritoneal metastasis.
Clin Exp Metastasis, 39(2):323-333, 12 Nov 2021
Cited by: 4 articles | PMID: 34767138
Time-serial expression of toll-like receptor 4 signaling during polymicrobial sepsis in rats.
Int J Immunopathol Pharmacol, 36:3946320221090021, 01 Jan 2022
Cited by: 5 articles | PMID: 35603454 | PMCID: PMC9127845
Characterization of Early-Onset Finger Osteoarthritis-Like Condition Using Patient-Derived Induced Pluripotent Stem Cells.
Cells, 10(2):317, 04 Feb 2021
Cited by: 5 articles | PMID: 33557199 | PMCID: PMC7913990
Host fibrinogen drives antimicrobial function in Staphylococcus aureus peritonitis through bacterial-mediated prothrombin activation.
Proc Natl Acad Sci U S A, 118(1):e2009837118, 21 Dec 2020
Cited by: 9 articles | PMID: 33443167 | PMCID: PMC7817220
Go to all (12) article citations
Data
Similar Articles
To arrive at the top five similar articles we use a word-weighted algorithm to compare words from the Title and Abstract of each citation.
Cellular stress amplifies TLR3/4-induced CXCL1/2 gene transcription in mononuclear phagocytes via RIPK1.
J Immunol, 193(2):879-888, 11 Jun 2014
Cited by: 18 articles | PMID: 24920846 | PMCID: PMC4091718
Synergistic Proinflammatory Responses by IL-17A and Toll-Like Receptor 3 in Human Airway Epithelial Cells.
PLoS One, 10(9):e0139491, 29 Sep 2015
Cited by: 9 articles | PMID: 26418032 | PMCID: PMC4587973
Role of adaptor TRIF in the MyD88-independent toll-like receptor signaling pathway.
Science, 301(5633):640-643, 10 Jul 2003
Cited by: 2059 articles | PMID: 12855817
Toll-like receptor 3 (TLR3) regulation mechanisms and roles in antiviral innate immune responses.
J Zhejiang Univ Sci B, 22(8):609-632, 01 Aug 2021
Cited by: 56 articles | PMID: 34414698 | PMCID: PMC8377577
Review Free full text in Europe PMC
Funding
Funders who supported this work.
Chonnam National University (1)
Grant ID: 2014-2026