Abstract
Free full text

CXCL13 is a plasma biomarker of germinal center activity
Significance
A major challenge for vaccine science is that there is no way to measure germinal center activity in humans. This challenge is particularly acute for human clinical trials of candidate vaccines (and most nonhuman primate studies of candidate vaccines), because germinal centers are the engines of Ab affinity maturation, and generation of highly affinity-matured Ab responses is the goal of all Ab-eliciting vaccines. Here, we report that we have identified the chemokine CXCL13 [chemokine (C-X-C motif) ligand 13] as a biomarker of germinal center activity. We show explicit relationships between plasma CXCL13 concentrations and germinal center frequencies in lymph nodes in a series of different conditions, including licensed and experimental vaccines, and in humans, nonhuman primates, and mice.
Abstract
Significantly higher levels of plasma CXCL13 [chemokine (C-X-C motif) ligand 13] were associated with the generation of broadly neutralizing antibodies (bnAbs) against HIV in a large longitudinal cohort of HIV-infected individuals. Germinal centers (GCs) perform the remarkable task of optimizing B-cell Ab responses. GCs are required for almost all B-cell receptor affinity maturation and will be a critical parameter to monitor if HIV bnAbs are to be induced by vaccination. However, lymphoid tissue is rarely available from immunized humans, making the monitoring of GC activity by direct assessment of GC B cells and germinal center CD4+ T follicular helper (GC Tfh) cells problematic. The CXCL13–CXCR5 [chemokine (C-X-C motif) receptor 5] chemokine axis plays a central role in organizing both B-cell follicles and GCs. Because GC Tfh cells can produce CXCL13, we explored the potential use of CXCL13 as a blood biomarker to indicate GC activity. In a series of studies, we found that plasma CXCL13 levels correlated with GC activity in draining lymph nodes of immunized mice, immunized macaques, and HIV-infected humans. Furthermore, plasma CXCL13 levels in immunized humans correlated with the magnitude of Ab responses and the frequency of ICOS+ (inducible T-cell costimulator) Tfh-like cells in blood. Together, these findings support the potential use of CXCL13 as a plasma biomarker of GC activity in human vaccine trials and other clinical settings.
The germinal center (GC) reaction is a critical immunological process that occurs in draining lymph nodes after immunization (1). The GC response consists of antigen-specific B cells undergoing affinity maturation through a process of somatic hypermutation (SHM) of the B-cell receptor. SHM is necessary for producing high-affinity Ab responses after immunizations and infections. Influenza neutralizing Abs have substantial SHM. Particularly high levels of SHM, 15–30% amino acid mutation (2, 3), are present and necessary for broad Ab neutralization of diverse HIV strains (4, 5). Therefore, as candidate influenza and HIV vaccines are evaluated for the ability to induce broadly neutralizing antibodies (bnAbs), the quantitation and functional characterization of GC responses will be a key parameter for study. Serological analysis of vaccine-specific Ab titers provides important information, but those data are limited. Serological outcomes are measured at time points long after initial immunizations. Neutralizing Ab responses are commonly only measurable after multiple boosts. Those outcomes likely depend on GC activity and affinity maturation at much earlier time points. Several state of the art HIV vaccine strategies rely on long, multistage immunization protocols (6, 7). With bnAb responses as the goal, means of early analysis of the immune response will be essential to understand and improve on vaccination schemes that may end in failure or only partial success. One critical parameter to assess will be the ability of each immunization to generate GC responses.
Central to the GC reaction and SHM is the interaction of GC B cells with germinal center T follicular helper (GC Tfh) cells (8). GC Tfh cells are both required and limiting for the GC reaction (9, 10). GC Tfh cells control the number of GC B-cell divisions and therefore, the amount of SHM by individual GC B-cell clones (11). Currently, the preferred means of quantifying the GC response is the cellular enumeration and analysis of GC Tfh and GC B cells (8). However, in human and nonhuman primate (NHP) vaccination studies, direct analysis of draining lymph node tissues is impractical or undesirable for fear of removing the primary site of the ongoing immune response. Therefore, identification of a plasma biomarker for GC activity would be of great value in immunization studies as well as have utility in a number of other biomedically relevant contexts.
The CXCL13 [chemokine (C-X-C motif) ligand 13]–CXCR5 [chemokine (C-X-C motif) receptor 5] chemokine axis plays a major role in organizing both B-cell follicles and GCs (12, 13). CXCL13 is expressed by both follicular dendritic cells (14) and GC Tfh cells (15, 16) in the B-cell follicles. B- and Tfh-cell expressions of CXCR5, the receptor for CXCL13, are necessary for migration to the B-cell follicle. Although CXCL13 acts locally, it can also be detected in human plasma in the steady state, and perturbations in plasma CXCL13 have been associated with immune activity (17–21). Because GC Tfh cells regulate the size of the GC response and can be major producers of CXCL13, we explored whether plasma CXCL13 changes may reflect lymphoid tissue GC activity.
Results
Plasma CXCL13 Is Elevated in bnAb+ HIV-Infected Individuals.
The high levels of SHM seen in bnAbs generated against HIV and the association of circulating memory Tfh cells with the generation of bnAbs (22) suggest that individuals able to generate HIV bnAbs may have superior GC responses (6). Because directly measuring these tissue-resident GC responses in humans is not generally feasible, we asked whether CXCL13 was higher in the plasma of individuals able to generate HIV bnAbs than in the plasma of individuals who were not. We tested plasma samples from ART (antiretroviral therapy)-naive HIV+ individuals enrolled in International AIDS Vaccine Initiative (IAVI) Protocol C, a large longitudinal cohort of HIV+ individuals monitored early after infection and followed every 3–6 mo. This cohort has been extensively characterized for the ability of each individual to produce neutralizing Abs against HIV (22, 23). A neutralization score was calculated based on both the breadth and the potency of the neutralizing Ab present in individual samples for each time point after infection; 15% of 228 individuals followed beyond 4 y postinfection were found to have a neutralization score of greater than one and were termed top neutralizers (Fig. 1A). Top neutralizers had Ab responses capable of neutralizing an average of 73% (27 of 37) of HIV pseudoviruses using a principally tier 2 virus panel. Most HIV-infected individuals had Ab responses that scored less than 0.5, neutralizing an average of 27% (10 of 37) of HIV strains; these individuals were termed low neutralizers. Plasma from each individual was tested for neutralizing Abs and CXCL13 concentration at both the earliest time point available after HIV infection (~4 mo postinfection) and the time of bnAb development (~40 mo postinfection). Broad neutralization at the later time point was associated with elevated concentrations of plasma CXCL13 at both the ~4-mo (top neutralizers median: 92.7 pg/mL vs. low neutralizers median: 31.3 pg/mL; P = 0.012) and the ~40-mo (top neutralizers median: 78.9 pg/mL vs. low neutralizers median: 32.2 pg/mL; P = 0.008) time points postinfection (Fig. 1 B and C). A positive correlation between Ab breadth and CXCL13 was also observed in an independent cohort containing a small number (five) of individuals with neutralizing breadth (24). The generation of HIV neutralizing Abs is also positively correlated with HIV viral load (23, 25), and viral load could affect plasma CXCL13 levels (24, 26). We, therefore, asked whether viral load and CXCL13 were independent variables. The difference in plasma CXCL13 between top and low neutralizers remained significant at the ~40-mo time point and continued to show a strong trend at the ~4-mo time point. Multivariate analysis determined that plasma CXCL13 and viral load are largely independent factors [early time point ANCOVA (analysis of covariance), P = 0.021; bnAb development time point ANCOVA, P = 0.066]. Therefore, elevated plasma CXCL13 in top HIV neutralizers suggested that these individuals may have stronger GC responses.
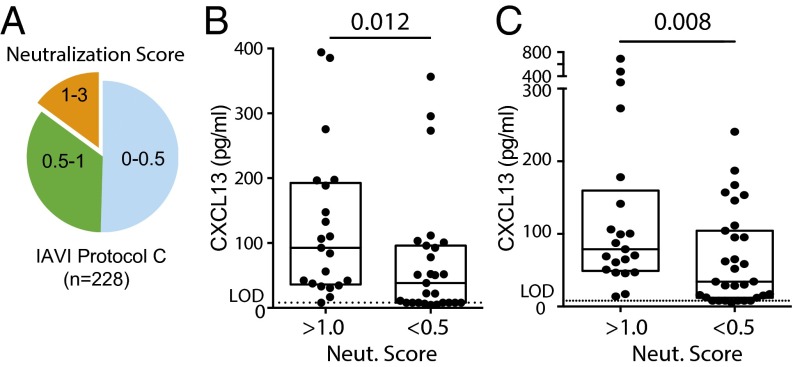
Plasma CXCL13 concentration is associated with HIV bnAb development. (A) HIV neutralizers grouped by neutralization score for IAVI Protocol C. The neutralization score is derived from both breadth and potency data from a 6- or 37-virus cross-clade pseudovirus panel. (B) Plasma CXCL13 from top (neutralization score >1.0) and low (neutralization score <0.5) neutralizing individuals at the earliest time point available after infection (range of 1–9 mo; mean of 4 mo). (C) Plasma CXCL13 from top and low neutralizing individuals at the time of bnAb generation (range of 24–54 mo; mean of 40 mo). CXCL13 ELISA limit of detection (LOD) was 8 pg/mL. Means and interquartile ranges are shown in B and C. Each point represents an individual.
Plasma CXCL13 Is Correlated with Lymphoid Tissue GCs in Humans.
GC Tfh cells are a producer of CXCL13 in secondary lymphoid tissue, such as tonsil (15, 16, 27). We found that GC Tfh cells were uniquely able to produce CXCL13 when analyzed by intracellular FACS analysis (Fig. 2A). Other cell types have been reported to produce CXCL13 under various inflammatory conditions (26, 28). On examination, CXCL13 protein was not produced in the other cell types present in tonsil cell preparations (Fig. 2B) or monocytes in peripheral blood mononuclear cells (PBMCs) (Fig. S1 A and B). Similar results were obtained from human spleen and lymph node tissues, showing CXCL13 expression to be restricted to GC Tfh cells (Fig. 2C).
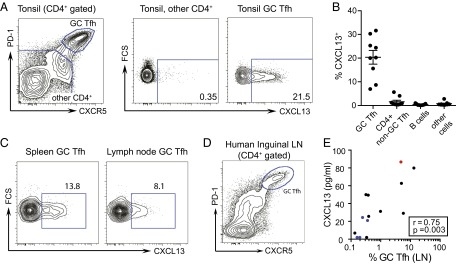
Human GC Tfh cells produce CXCL13 and correlate with plasma CXCL13. (A, Left) Identification of GC Tfh cells as PD-1hi CXCR5hi (gated on CD4+CD3+) T cells in human tonsil. (A, Center and Right) Intracellular cytokine staining for CXCL13 in unstimulated GC Tfh cells and all other non-GC Tfh CD4+ T cells in human tonsil. (B) Intracellular cytokine staining for CXCL13 in unstimulated cell subsets from nine human tonsils. (C) Intracellular cytokine staining for CXCL13 in unstimulated GC Tfh cells in human spleen (representative of two analyzed) and a human lymph node. (D) Identification of GC Tfh cells as PD-1hi CXCR5hi (gated on CD4+CD3+) T cells in human inguinal lymph node. (E) Matched plasma CXCL13 and lymph node GC Tfh cells in 14 human donors. Black indicates HIV+ antiretroviral-treated, blue indicates HIV seronegative, and red indicates HIV+ viremic controller. The Spearman r and P values are shown. The GC Tfh-cell percentage is plotted on log scale for visualization purposes only; the linear correlation results in an r2 value of 0.48. LN, lymph node.

Lack of CXCL13 production by blood monocytes and blood CXCR5+ CD4 T cells. (A) Monocyte gate by size [FSC (forward scatter)] and granularity [SSC (side scatter)] characteristics within human PBMCs. (B) Monocyte gated events from healthy donors or HIV+ individuals were either left unstimulated or stimulated for 4 h with PMA (phorbol 12-myristate 13-acetate) and Ionomycin in the presence of brefeldin A. Blood monocytes from both healthy and HIV+ donors produced TNF but did not produce CXCL13. (C, Upper) CD4+CXCR5+ gated or (C, Lower) CD4+CXCR5+PD1+++ICOS+ gated blood cells were either left unstimulated or stimulated for 4 h with PMA and Ionomycin in the presence of brefeldin A. Both cell populations expressed CD40L after stimulation but did not produce CXCL13 in either condition. (D) Frequency summary of CXCL13 producing cells from blood. Three healthy donor and three HIV+ donor samples were tested. GC Tfh cells from tonsil (from Fig. 2B) are included for comparison.
In an additional cohort of HIV+ and HIV− individuals at Massachusetts General Hospital, we obtained lymph node biopsies, allowing us to directly compare plasma CXCL13 to GC activity in human lymphoid tissue. GC Tfh cells (CXCR5hi PD-1hi) were identified (Fig. 2D) and quantified. In 14 matched plasma and lymph node samples, plasma CXCL13 concentration positively correlated with GC Tfh cells in the lymph node (r = 0.75; P = 0.003) (Fig. 2E). Furthermore, plasma CXCL13 also correlated with GC B cells (r = 0.62; P = 0.02) (Fig. S2A). The strong correlation observed between plasma CXCL13 and lymph node GC Tfh cells within a relatively small human donor set together with the ability of GC Tfh cells to produce CXCL13 suggest that CXCL13 can be a biomarker of GC activity.
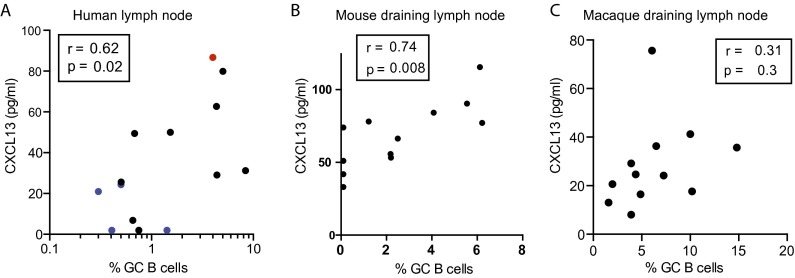
Plasma CXCL13 is correlated with GC B-cell frequency. (A) Human GC B cells as CD38+IgD− (gated on CD19+) B cells in human inguinal lymph node were matched with plasma CXCL13 samples in 14 human donors. Samples are color coded as in Fig. 2E: black, HIV+ antiretroviral-treated; blue, HIV seronegative; red, HIV+ viremic controller. The Spearman r and P values are shown. The GC B-cell percentage is plotted on log scale for visualization purposes only; the linear correlation results in an r2 value of 0.25. This panel corresponds to the GC Tfh-cell–CXCL13 correlation shown in Fig. 2E. (B) Correlation of plasma CXCL13 and GC B cells (Bcl6+B220+) in naïve and alum + OVA immunized mice in Fig. 3C. GC B cells in naïve mice were set at the limit of detection (0.1% of total CD19+ B cells). (C) Nonsignificant positive correlation of plasma CXCL13 and GC B cells (Ki67+Bcl6+CD20+) in the draining popliteal lymph node of macaques 7 d after the second or third protein and adjuvant immunization. Aluminum hydroxide or TLR (Toll-like receptor) ligand-encapsulated PLGA [poly(lactic-co-glycolic acid)] nanoparticles were used as adjuvants for gp140 Env and p55 Gag recombinant SIV (Simian immunodeficiency virus) proteins. This panel corresponds to the GC Tfh-cell–CXCL13 correlation shown in Fig. 4C.
Plasma CXCL13 Is Correlated with Lymph Node GCs in Mice After Immunization.
We next examined the relationship between plasma CXCL13 and GC activity after protein immunization in animal vaccination models (6, 29–31). We first immunized mice with 4-hydroxy-3-nitrophenyl acetyl haptenated ovalbumin in aluminum hydroxide adjuvant (alum + NP-OVA). One-half of the immunized mice received OT-II TCR (T-cell receptor) transgenic CD4 T cells to enhance the antigen-specific and GC Tfh CD4 T-cell response. Seven days after immunization, plasma CXCL13 was increased compared with that in unimmunized mice (Fig. 3A). Plasma CXCL13 was also increased 7 d after acute infection with LCMV (lymphocytic choriomeningitis virus) Armstrong or vaccinia virus (Fig. 3A). In alum + NP-OVA immunized mice, GC Tfh cells were quantified in the draining lymph node (CXCR5hi PD-1hi) (Fig. 3B), and plasma CXCL13 correlated with the frequency of vaccine-induced GC Tfh cells (Fig. 3C) (r = 0.82; P = 0.002) and GC B cells (r = 0.74; P = 0.008) (Fig. S2B).

Plasma CXCL13 correlates with GC Tfh cells in mice after immunization. (A) Plasma CXCL13 in naïve mice or mice 7 d after alum + NP-OVA footpad immunization, LCMV infection, or vaccinia virus infection. Black circles indicate the presence of transferred CD4+ TCR transgenic T cells: OT-II cells into the alum + NP-OVA group and NIP cells into the LCMV group. White circles indicate untransferred mice. (B) Identification of GC Tfh cells as PD-1hi CXCR5hi cells in the draining popliteal lymph node of an NP-OVA immunized mouse. (C) Correlation of plasma CXCL13 and GC Tfh cells in naïve and alum + NP-OVA immunized mice from A. GC Tfh cells in naïve mice were set at the limit of detection (0.1% of the total CD4+ T cells). (D and E) Plasma CXCL13 in B6 mice immunized in the footpad with KLH + alum. Data representative of two experiments of 10 mice each. (D) Plasma CXCL13 pre- and postprimary immunization. (E) Plasma CXCL13 in the same mice as those in D after a boost with KLH + alum at 50 d postprimary immunization. (F) Correlation of plasma CXCL13 and GC Tfh cells in the draining popliteal lymph node at 10 and 18 d postboost from animals shown in E. CXCL13 is plotted as fold change of day 10 or 18 postbooster immunization over preboost (d50) CXCL13 concentration.
In a larger study of immunized mice that did not receive transgenic CD4 T cells, keyhole limpet hemocyanin plus aluminum hydroxide (KLH + alum) immunized mice had higher plasma concentrations of CXCL13 after immunization compared with those at the preimmunization time point in the same mice, peaking at 2 wk postimmunization (Fig. 3D). The mice were given a booster immunization with KLH + alum 50 d after the primary immunization. Plasma CXCL13 concentrations were increased at both 10 and 18 d postboost (Fig. 3E) and again, correlated with the frequency of GC Tfh cells in the draining lymph node (r = 0.69; P = 0.023) (Fig. 3F). Thus, in small animal immunization models, plasma CXCL13 concentrations were a positive biomarker of GC activity and GC Tfh cells.
Plasma CXCL13 Is Correlated with GC Activity in Macaques After Immunization.
NHPs are considered the best animal model for many preclinical vaccine studies. We considered that CXCL13 expression by GC Tfh cells in NHPs might be more similar to that of humans. We, therefore, examined the relationship between plasma CXCL13 and GC activity in rhesus macaques after protein immunization. Our previous identification of an anti-human CXCR5 Ab (clone MU5UBEE) reactive to rhesus macaque CXCR5 (used in the works of refs. 32–35) allowed detection of CXCR5hi PD1hi (programmed cell death 1) GC Tfh cells in macaque lymphoid tissue (Fig. 4A). In pilot experiments, we determined that GC Tfh cells in macaques highly express Bcl6, ICOS (inducible T-cell costimulator), and CD200 (Fig. 4A), directly analogous to human GC Tfh cells (8). Seven days after a protein and adjuvant immunization, GC Tfh- and GC B-cell responses were found in the draining lymph node but not in a nondraining lymph node (Fig. 4B). We examined plasma CXCL13 in these animals at the same day 7 postimmunization time point. We observed a strong positive correlation between plasma CXCL13 concentration and GC Tfh-cell abundance in the draining lymph node (r = 0.71; P = 0.013) (Fig. 4C). A nonsignificant positive trend was observed with GC B-cell frequency (Fig. S2C). The correlation between plasma CXCL13 and GC Tfh cells in immunized rhesus macaques was confirmed in an additional study of 12 animals. In summary, plasma CXCL13 responses in both mice and rhesus macaques strongly correlated with GC Tfh-cell frequencies in draining lymph node after immunization.

Plasma CXCL13 correlates with GC Tfh cells in rhesus macaques after immunization. (A) Identification and characterization of GC Tfh cells (PD1hi CXCR5hi CD4+ T cells) in lymph node from rhesus macaques. Expression of Bcl-6, ICOS, and CD200 is shown for GC Tfh cells (red), mantle CXCR5+ Tfh cells (blue), and non-Tfh cells (gray). (B) Identification of (Left) GC Tfh cells and (Right) GC B cells (identified as Ki67+Bcl6+ cells; gated on CD20+ cells) in nondraining (axillary) and draining lymph nodes (popliteal) of macaques 7 d after protein and adjuvant immunization in the leg. (C) Correlation of plasma CXCL13 and GC Tfh cells in the draining popliteal lymph node of macaques 7 d after the second or third protein and adjuvant immunization. The correlation holds even with removal of the potential outlier point (highest GC Tfh cell and highest CXCL13), with values of r = 0.62 and P = 0.048. Aluminum hydroxide or TLR (Toll-like receptor) ligand-encapsulated PLGA [poly(lactic-co-glycolic acid)] nanoparticles were used as adjuvants for gp140 Env and p55 Gag recombinant SIV (Simian immunodeficiency virus) proteins. All data are representative of two similar immunization experiments in rhesus macaques totaling 22 animals. LN, lymph node.
Plasma CXCL13 Is Increased in Humans After Immunization.
Because plasma CXCL13 was elevated after immunization in animal models, correlated with GC activity, and correlated with bnAb development in HIV+ individuals, we investigated plasma CXCL13 responses after vaccination in humans. We initially examined plasma CXCL13 in a small cohort of influenza vaccine recipients. We found mixed plasma CXCL13 responses after influenza immunization that did not exhibit a statistically significance change (Fig. S3). The lack of a clear increase in plasma CXCL13 could be because of the fact that there was low overall antiinfluenza Ab response generated to the immunization caused by preexisting Ab titers (36). We, therefore, moved to study immunizations that generated more robust immune responses. Two separate cohorts were studied. The first cohort was a vaccine cohort immunized with the Food and Drug Administration-approved yellow fever virus vaccine (37). The second group comprised study participants in an HIV Vaccine Trials Network (HVTN) protocol testing a candidate HIV vaccine regimen (HVTN068) (38).
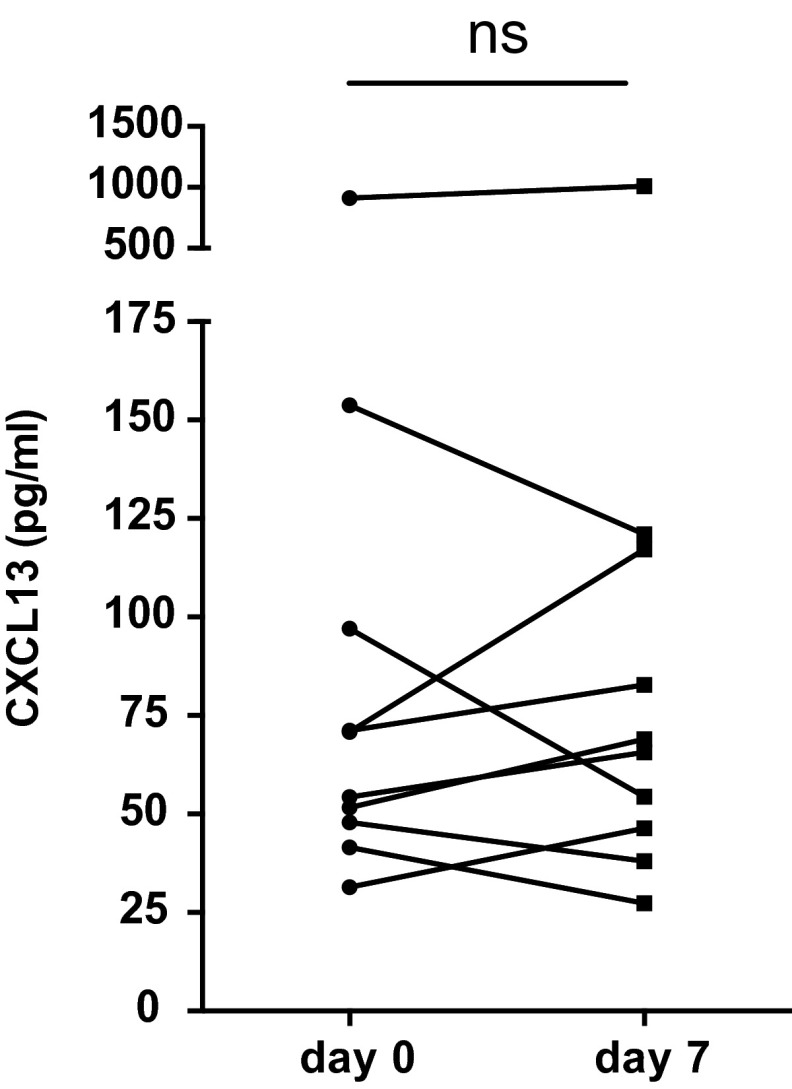
Plasma CXCL13 is not significantly increased after influenza immunization in humans. Plasma CXCL13 measured before immunization (day 0) and 7 d postinfluenza vaccination in 10 individuals. Healthy donors were enrolled in an influenza vaccine study at the Stanford–Lucile Packard Children’s Hospital Vaccine Program during the 2010–2011 influenza season and received a single dose of TIV Fluzone (Sanofi Pasteur). ns, not significant.
We tested pre- and postvaccination plasma samples obtained from 17 yellow fever vaccine recipients. Seven days after immunization, statistically significant increases in plasma CXCL13 were observed (P = 0.04) (Fig. 5A). Plasma CXCL13 was 30% higher than prevaccinations levels in 9 of 17 individuals. We next assessed the kinetics of plasma CXCL13 in 11 vaccinated individuals in the HVTN068. After adenovirus-5 vector with HIV-1 gene inserts (Ad5/HIV) immunization, plasma CXCL13 concentrations were significantly increased over preboost levels at both days 7 (P = 0.001) and 14 (P = 0.014) (Fig. 5B). For each individual donor, peak plasma CXCL13 was detected at either the 7- or 14-d time point. Seven days after immunization, plasma CXCL13 was 50% higher than at the prevaccination time point in 9 of 11 individuals. In a larger set of samples available at only the 7-d time point, plasma CXCL13 positively correlated with the vaccine-specific gp41 Env IgG Ab response (r = 0.41; P = 0.037) (Fig. 5C) and showed a strong positive trend with the gp140 Env IgG Ab response (Fig. S4) measured 28 d after immunization. In a small number of individuals for whom cryopreserved PBMCs were available, we evaluated the frequency of activated ICOS+PD1+++CXCR5+ CD4 T cells after vaccination. ICOS+PD1+++CXCR5+ CD4 T cells are found in the blood of individuals with ongoing immune responses and may be a subpopulation of Tfh cells trafficking through the blood before becoming GC Tfh cells (8, 22, 39, 40). Even with only six individuals available for analysis, plasma CXCL13 concentration responses correlated with the increase in activated ICOS+PD1+++CXCR5+ CD4 T cells found in the blood 7 d after immunization (r = 0.85; P = 0.03) (Fig. 5D). Thus, plasma CXCL13 correlates with GC-associated Ab and Tfh-cell responses in immunized humans.
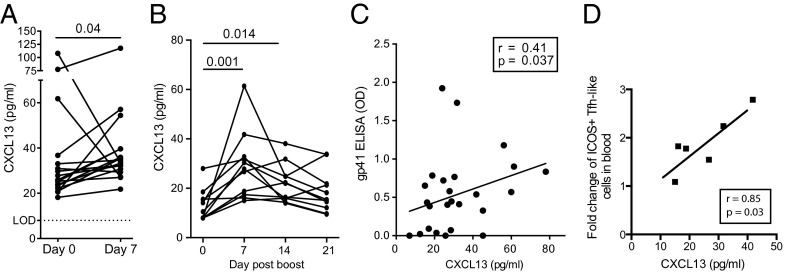
Plasma CXCL13 is increased after immunization in humans. (A) Plasma CXCL13 measured before immunization (day 0) and 7 d postyellow fever vaccination in 17 individuals. (B–D) HVTN068 participants who received an Ad5/vector encoding HIV gag and envelope immunization 6 mo postprime. (B) Kinetic analysis of plasma CXCL13 post-Ad5/HIV boost. Plasma CXCL13 was measured in 11 vaccinated individuals. (C) Correlation of plasma CXCL13 7 d postimmunization and anti-gp41 Env Ab responses (ELISA OD) 4 wk postimmunization in 26 vaccinated individuals. Anti-gp41 Ab ELISA OD is background-subtracted. (D) Correlation of plasma CXCL13 7 d postimmunization and the fold change of ICOS+ blood Tfh-like cells (percentage at day 7 postboost over percentage at preboost time point) in six vaccinated donors. LOD, limit of detection; OD, optical density.
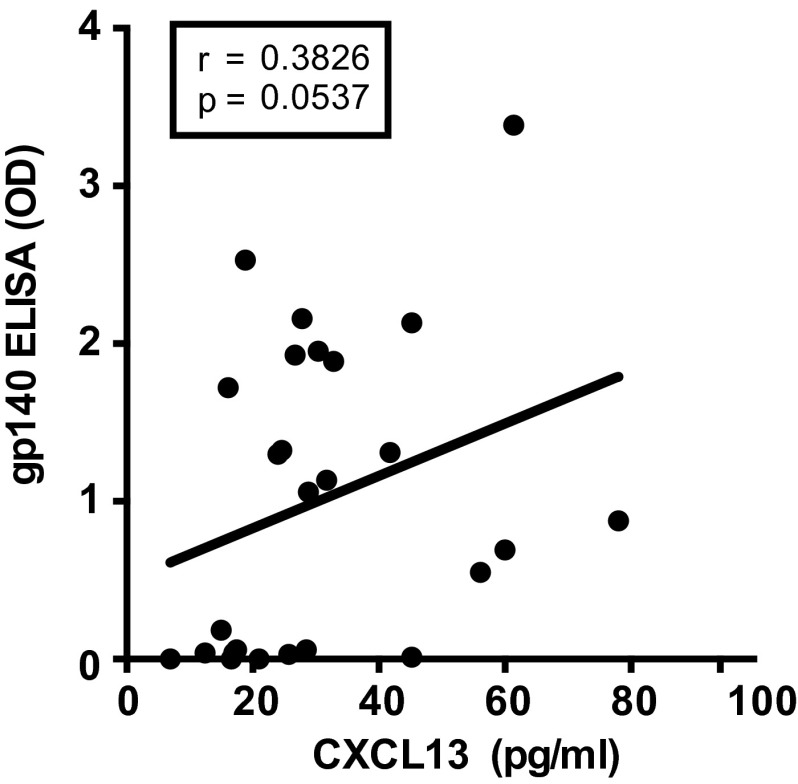
Correlation of plasma CXCL13 concentration and Ab response after immunization. As in Fig. 5C except plasma CXCL13 concentration 7 d postimmunization correlated with anti-gp140 (ConS; consensus group M) Env Ab responses (ELISA OD) 4 wk postimmunization in 26 vaccinated individuals (HVTN068). Anti-gp140 Ab ELISA OD is background-subtracted.
Discussion
The GC response is a critical immune mechanism by which Ab affinity occurs, memory B cells develop, and long-lived plasma cells are produced. Here, we show a means to monitor GC activity in lymphoid tissues using a plasma biomarker. Plasma CXCL13 positively correlates with the lymph node GC response in mice, macaques, and humans. Increases in plasma CXCL13 were found in a number of different immune-activating conditions: aluminum hydroxide or TLR (Toll-like receptor) ligand adjuvants plus recombinant protein immunizations, acute viral infections, an adenovirus vector candidate HIV vaccine, the licensed yellow fever vaccine, and HIV infection. Based on the strong correlation of GC Tfh cells and plasma CXCL13 and the significant measurable change in plasma CXCL13 in two human vaccine cohorts, monitoring plasma CXCL13 could be useful in human and NHP vaccine trials, where direct analysis of lymphoid tissue is either not possible or undesirable for fear of disturbing the ongoing immune response.
If bnAbs against HIV are to be generated by vaccination, the GC response will play a central role. Measuring CXCL13 in vaccine studies can provide data on postvaccination GC activity, a major driver of Ab quality by SHM. Furthermore, in some cases, antigen-specific Ab results are not measured until after a final boost 6 mo after the primary immunization. CXCL13 can be measured after each immunization, providing much earlier data on the progress of the immune response to the immunization scheme, which could be important for in-trial decision-making. Our studies detecting increases in plasma CXCL13 in the majority but not all of the immunized individuals suggest that GCs were not generated in certain individuals, a potentially critical observation. We do not suggest that CXCL13 analysis should replace antigen-specific Ab titer data, but rather that CXCL13 monitoring be added as a valuable parameter to gain an understanding of the magnitude of the GC activity that is necessary for the development of improved Ab quality. Given that GC B cells do not exist in peripheral blood, CXCL13 may be the best available proxy for those inaccessible cells.
Plasma CXCL13 has been proposed to serve as a biomarker of autoimmune diseases, such as rheumatoid arthritis, systemic lupus erythematosus, Sjogren’s syndrome, and Myasthenia Gravis (41). Elevated plasma CXCL13 was detected in patients with systemic lupus erythematosus and further increased in individuals with severe disease presenting with nephritis or anti-DNA Ab responses (19). In rheumatoid arthritis, CXCL13 was not only followed as a plasma biomarker of disease, but also, CXCL13 blockade has been proposed as a treatment (42). It is important to note that analysis of plasma CXCL13 is not an antigen- or disease-specific readout. Plasma CXCL13 reports total GC activity, and the basal levels detected in unimmunized humans, macaques, and mice likely reflect ongoing GC activity in tonsillar- and gut-associated lymphoid tissue. For these reasons, we consider a multiparameter approach to be the best approach. Analysis of plasma CXCL13 together with analysis of other potential biomarkers specific to the immunological and pathological setting under study are advisable.
We have shown a strong correlation between CXCL13 and lymphoid tissue resident GC Tfh cells. With the additional observation that GC Tfh cells are robust producers of CXCL13, it is suggestive of a direct relationship between GC Tfh cells and plasma CXCL13. We did not identify other cell types in lymphoid tissue, monocytes in PBMCs, or CXCR5+ Tfh cells in PBMCs as producers of CXCL13 by intracellular staining. Although follicular dendritic cells (FDC) and some dendritic cell subsets are likely lost during tonsil tissue processing, a histological study suggests that much of the CXCL13 observed in the tonsil GC costains with PD-1, and PD-1 is an excellent marker of GC Tfh cells (27). In the same study, CXCL13 was only weakly associated with CD21+ FDC. Monocytes (26) and dendritic cells (28) have been reported to express CXCL13 and could contribute to plasma CXCL13 concentrations in different inflammatory settings. The observation that monocytes can produce CXCL13 in response to IFN-I may be relevant in HIV infection and immunizations with viral vectors or adjuvants containing TLR ligands. However, we did not identify CXCL13-producing monocytes in PBMCs from HIV+ individuals ex vivo or after a short in vitro stimulation, and IFN-I responses are normally short in duration, peaking at 24–48 h after immunization (43, 44). Therefore, monocyte-generated CXCL13 may not significantly impact plasma CXCL13 measured in our immunization studies. These later time points examined are contemporaneous with GC activity.
Here, we show that CXCL13 acts as a plasma biomarker for GC activity in generally inaccessible lymphoid tissue. Plasma CXCL13 correlates with GC activity after immunization in animal models and in HIV+ humans. Furthermore, plasma CXCL13 is associated with generation of HIV bnAbs, and CXCL13 was elevated in humans after immunization. Together, these findings support the use of CXCL13 as a plasma biomarker of GC activity useful for studying differences in humoral immunity among patients and vaccinees.
Materials and Methods
IAVI Protocol C and Human Vaccine Cohorts.
The IAVI Protocol C cohort has been described elsewhere (22, 23). An HIV neutralization score for each plasma sample was determined as in the work by Simek et al. (45) to account for both breadth and potency. All donors were ART-free and had CD4 counts >200 cells per 1 mL at each time point tested.
CXCL13 was analyzed in the serum before and 1 wk after a single immunization of TIV Fluzone (Sanofi Pasteur) in vaccine recipients from the Stanford–Lucile Packard Children’s Hospital Vaccine Program (36). Yellow fever vaccine recipients received the Food and Drug Administration-approved 17D yellow fever vaccine (37). Plasma samples from HIV-uninfected candidate vaccine recipients from the HVTN068 trial (38) were analyzed for CXCL13 in plasma after a booster immunization (Ad5/HIV) given at the 6-mo time point. Primary immunizations were either Ad5/HIV at month 0 or DNA/HIV at months 0 and 1. Some participants received placebo.
Human Lymph Node, Spleen, and Tonsil.
Inguinal lymph nodes from HIV-infected persons and HIV− healthy volunteers were obtained by excisional surgical biopsy under local anesthesia at Massachusetts General Hospital and processed as in the work by Lindqvist et al. (46) Additional nonidentifiable, discarded, excess spleen, lymph node, and tonsil tissues were obtained at Massachusetts General Hospital or Rady Children’s Hospital–San Diego. Spleen and lymph node tissues (46) and tonsils (15) were processed as previously described.
Macaque and Mouse Immunization.
Macaques were immunized s.c. with gp140 (SIVmac239) Env and p55 Gag protein mixed with either aluminum hydroxide (Alhydrogel 2% adjuvant; Invivogen) or MPL (monophosphoryl lipid A) + R848-encapsulated PLGA [poly(lactic-co-glycolic acid)] nanoparticles (47). A full description of the vaccine trial methods and results will be published elsewhere.
C57BL/6 (B6) mice (Jackson Laboratory) were immunized with alum + NP-OVA (Sigma-Aldrich); 10 μg NP-OVA was mixed 1:1 with 10% (wt/vol) aluminum hydroxide (aluminum potassium sulfate dodecahydrate; Sigma-Aldrich) in PBS. In some mice, 2 × 105 OVA-specific OT-II TCR transgenic CD4 T cells were transferred 3.5 d before immunization. For KLH + alum immunized mice, 10 μg KLH was mixed 1:1 with 10% aluminum hydroxide in PBS. For LCMV immunization experiments, 2 × 105 NIP CD4+ TCR transgenic cells (48) were transferred before immunization with 2 × 106 pfu LCMV Armstrong by i.p. injection. For vaccinia virus immunization experiments, B6 mice were i.p. injected with 6 × 105 pfu.
CXCL13 ELISA and Flow Cytometry.
The Human CXCL13 Quantikine ELISA Kit (R&D Systems) was used for both human and macaque (49) plasma samples as described in the instructions. The mouse CXCL13 DuoSet (R&D Systems) was used for quantification of CXCL13 in mouse serum. All samples were tested in duplicate.
GC Tfh cells were characterized by flow cytometry as previously described in mouse lymph node (50), human tonsil (15, 22), or human lymph node (46). Previously cryopreserved human PBMCs from HVTN068 subjects were stained. Intracellular CXCL13 was detected by intracellular cytokine staining as described (15). Cells were acquired on a BD Fortessa Analyzer.
Statistics.
The Mann–Whitney test was used for evaluating differences among groups. The Wilcoxon test was used to evaluate differences between time points for the same individuals. The Spearman correlation test was used for all correlative analysis. Covariance of plasma CXCL13 and viral load was evaluated with the ANCOVA multivariate statistical test (VassarStats). Prism 5.0 (GraphPad) was used for all other data statistical analyses.
Study Approvals.
Informed, written consent was obtained from all human study participants before enrollment in the human studies listed above and approved by the local institutional review boards (La Jolla Institute Internal Review Board, The Scripps Research Institute Internal Review Board, Massachusetts General Hospital Partners Human Research Committee, the Institutional Review Board of the Research Compliance Office at Stanford University, Institutional Review Boards at Emory University, Centers for Disease Control, and the Institutional Review Board at the HVTN). Rhesus macaque study procedures were performed in accordance with Emory School of Medicine Institutional Animal Care and Use Committee-approved protocols. Mouse study procedures were performed in accordance with approved animal protocols at La Jolla Institute for Allergy and Immunology.
Acknowledgments
We thank all project study volunteers and clinical and study team members. This work was supported by grants from the National Institute of Allergy and Infectious Diseases, International AIDS Vaccine Initiative (IAVI), and the Scripps Center for HIV/AIDS Vaccine Immunology and Immunogen Discovery (Scripps CHAVI-ID).
Footnotes
The authors declare no conflict of interest.
This article is a PNAS Direct Submission.
This article contains supporting information online at www.pnas.org/lookup/suppl/10.1073/pnas.1520112113/-/DCSupplemental.
References
Articles from Proceedings of the National Academy of Sciences of the United States of America are provided here courtesy of National Academy of Sciences
Full text links
Read article at publisher's site: https://doi.org/10.1073/pnas.1520112113
Read article for free, from open access legal sources, via Unpaywall:
https://www.pnas.org/content/pnas/113/10/2702.full.pdf
Citations & impact
Impact metrics
Citations of article over time
Alternative metrics
Smart citations by scite.ai
Explore citation contexts and check if this article has been
supported or disputed.
https://scite.ai/reports/10.1073/pnas.1520112113
Article citations
Single-cell sequencing reveals immune features of treatment response to neoadjuvant immunochemotherapy in esophageal squamous cell carcinoma.
Nat Commun, 15(1):9097, 22 Oct 2024
Cited by: 0 articles | PMID: 39438438 | PMCID: PMC11496748
Modeling memory B cell responses in a lymphoid organ-chip to evaluate mRNA vaccine boosting.
J Exp Med, 221(10):e20240289, 06 Sep 2024
Cited by: 0 articles | PMID: 39240335
Immunogenicity and safety of an Entamoeba histolytica adjuvanted protein vaccine candidate (LecA+GLA-3M-052 liposomes) in rhesus macaques.
Hum Vaccin Immunother, 20(1):2374147, 01 Aug 2024
Cited by: 0 articles | PMID: 39090779 | PMCID: PMC11296537
Interferon subverts an AHR-JUN axis to promote CXCL13<sup>+</sup> T cells in lupus.
Nature, 631(8022):857-866, 10 Jul 2024
Cited by: 3 articles | PMID: 38987586
Lymph Node-on-Chip Technology: Cutting-Edge Advances in Immune Microenvironment Simulation.
Pharmaceutics, 16(5):666, 16 May 2024
Cited by: 1 article | PMID: 38794327 | PMCID: PMC11124897
Review Free full text in Europe PMC
Go to all (209) article citations
Data
Similar Articles
To arrive at the top five similar articles we use a word-weighted algorithm to compare words from the Title and Abstract of each citation.
Plasma IL-5 but Not CXCL13 Correlates With Neutralization Breadth in HIV-Infected Children.
Front Immunol, 10:1497, 02 Jul 2019
Cited by: 3 articles | PMID: 31333650 | PMCID: PMC6615198
A Novel Rabies Vaccine Expressing CXCL13 Enhances Humoral Immunity by Recruiting both T Follicular Helper and Germinal Center B Cells.
J Virol, 91(3):e01956-16, 18 Jan 2017
Cited by: 25 articles | PMID: 27852854 | PMCID: PMC5244327
The Role of CXCL13 in Antibody Responses to HIV-1 Infection and Vaccination.
Front Immunol, 12:638872, 25 Feb 2021
Cited by: 11 articles | PMID: 33732259 | PMCID: PMC7959754
Review Free full text in Europe PMC
Cytokine-Independent Detection of Antigen-Specific Germinal Center T Follicular Helper Cells in Immunized Nonhuman Primates Using a Live Cell Activation-Induced Marker Technique.
J Immunol, 197(3):994-1002, 22 Jun 2016
Cited by: 104 articles | PMID: 27335502 | PMCID: PMC4955744
Funding
Funders who supported this work.
NIAID NIH HHS (4)
Grant ID: R37 AI048638
Grant ID: UM1 AI068614
Grant ID: UM1 AI100663
Grant ID: UM1 AI068618
NIDDK NIH HHS (1)
Grant ID: R37 DK057665