Abstract
Scope
α-Cyclodextrin (α-CD), a cyclic polymer of glucose, has been shown to lower plasma cholesterol in animals and humans; however, its effect on atherosclerosis has not been previously described.Methods and results
apoE-knockout mice were fed either low-fat diet (LFD; 5.2% fat, w/w), or Western high fat diet (21.2% fat) containing either no additions (WD), 1.5% α-CD (WDA); 1.5% β-CD (WDB); or 1.5% oligofructose-enriched inulin (WDI). Although plasma lipids were similar after 11 weeks on the WD vs. WDA diets, aortic atherosclerotic lesions were 65% less in mice on WDA compared to WD (P < 0.05), and similar to mice fed the LFD. No effect on atherosclerosis was observed for the other WD supplemented diets. By RNA-seq analysis of 16S rRNA, addition of α-CD to the WD resulted in significantly decreased cecal bacterial counts in genera Clostridium and Turicibacterium, and significantly increased Dehalobacteriaceae. At family level, Comamonadaceae significantly increased and Peptostreptococcaceae showed a negative trend. Several of these bacterial count changes correlated negatively with % atherosclerotic lesion and were associated with increased cecum weight and decreased plasma cholesterol levels.Conclusion
Addition of α-CD to the diet of apoE-knockout mice decreases atherosclerosis and is associated with changes in the gut flora.Free full text

Dietary α-cyclodextrin reduces atherosclerosis and modifies gut flora in apolipoprotein E-deficient mice
Abstract
Scope
α-Cyclodextrin (α-CD), a cyclic polymer of glucose, has been shown to lower plasma cholesterol in animals and humans; however, its effect on atherosclerosis has not been previously described.
Methods and Results
apoE-knockout mice were fed either low-fat diet (LFD; 5.2% fat, w/w), or Western high fat diet (21.2% fat) containing either no additions (WD), 1.5% α-CD (WDA); 1.5% β-CD (WDB); or 1.5% oligofructose-enriched inulin (WDI). Although plasma lipids were similar after 11 weeks on the WD vs. WDA diets, aortic atherosclerotic lesions were 65% less in mice on WDA compared to WD (P < 0.05), and similar to mice fed the LFD. No effect on atherosclerosis was observed for the other WD supplemented diets. By RNA-seq analysis of 16S rRNA, addition of α-CD to the WD resulted in significantly decreased cecal bacterial counts in genera Clostridium and Turicibacterium, and significantly increased Dehalobacteriaceae. At family level, Comamonadaceae significantly increased and Peptostreptococcaceae showed a negative trend. Several of these bacterial count changes correlated negatively with % atherosclerotic lesion and were associated with increased cecum weight and decreased plasma cholesterol levels.
Conclusion
Addition of α-CD to the diet of apoE-knockout mice decreases atherosclerosis and is associated with changes in the gut flora.
1 Introduction
Cyclodextrins (CDs) are cyclic polymers of glucose that are commonly used as food additives and are considered as dietary fibers [1, 2]. α-CD and γ-CD are water-soluble and consist of six or eight α-D-glucopyranose units, respectively, whereas β-CD is a relatively water-insoluble fiber with seven units of α-D-glucopyranose. Other dietary fibers, such as oats, inulin, psyllium, guar gum and pectin, are known to have multiple beneficial effects on healthy subjects [3] and improve several different metabolic disorders related to obesity [4], type-II diabetes [5], and cardiovascular disease [6]. Likewise, several animal studies have demonstrated that CDs can also lower plasma cholesterol and triglycerides [7, 8].
Two possible mechanisms have been proposed for the lipid-lowering effect of CDs. First, CDs can entrap hydrophobic compounds, such as cholesterol and bile acid, into the hydrophobic cavity created by their cyclic glucose structure [1]. β-CD, in particular, has a hydrophobic cavity with an inner diameter of 6.0–6.5 angstroms, which is well-suited for the high affinity binding of cholesterol and bile acids. Thus, oral supplementation of CDs could potentially disturb bile acid and cholesterol absorption by sequestering bile acids and cholesterol [1]. Indeed, methyl β-CD has been shown lower plasma lipids and reduce atherosclerosis in mice [9].
Another potential mechanism for the lipid lowering effect of CDs is based on bacterial fermentation of CDs. Except for γ-CD, most CDs are not readily digested by salivary or pancreatic amylase and intact CDs are also poorly absorbed by the intestine [10]. CDs, however, are known to be hydrolyzed by bacterial enzymes produced by gut flora in the large intestine [10] and can stimulate the production of short chain fatty acids (SCFAs) [11]. Several studies have reported that increases in cecal SCFAs were associated with improved cholesterol and triglyceride levels in plasma, possibly by affecting hepatic AMP kinase [12]. Increased short chain fatty acids (SCFAs) produced from bacterial fermentation of CDs might, therefore, also suppress serum cholesterol levels and have other beneficial effects [11, 13, 14].
Imbalances in healthy gut flora have also been linked to atherosclerosis [15]: hence, it is possible that CDs by altering gut flora could improve atherosclerosis. Interestingly, high fat diets supplemented with 10% oligofructose-enriched inulin, another water-soluble dietary fiber, increased cecal fermentation and diminished the development of atherosclerosis in apoE-KO mice, despite minimal reductions of plasma TC and TG [16].
While β-CD and inulin have been extensively studied in various human and animal studies, the effects of α-CD are not as well understood, even though it is more widely used as a food supplement [1, 3]. Several small clinical trials indicate a possible beneficial role of α-CD on plasma lipids and glucose [3, 17], but whether α-CD has a beneficial effect on suppressing the development of atherosclerosis has not yet been investigated. This is particularly important in terms of public health, as α-CD is included in many processed foods mostly to improve texture and shelf life [1].
In the present study, we investigated the effect of α-CD supplementation on atherosclerosis in apoE-knockout (KO) mice. Using both in vitro and in vivo models, we found that α-CD did not bind lipids as well as β-CD, and only had a minimal effect on lipid absorption and plasma lipid levels. Supplementing the WD diet with α-CD caused, however, a significant reduction in atherosclerosis in apoE-KO mice, which was associated with several potential beneficial changes in the gut flora.
3. Results
3.1 α-CD supplementation reduces atherosclerotic aortic lesion area
In an initial pilot study, apoE-KO mice (n = 10 per group) were fed either a Western diet (WD) or a Western diet supplemented with either 1.5 % α-CD (WDA) or 1.5 % β-CD (WDB) for 11 weeks (Supplemental Fig. 1). At the end of the study, aortic lesion area was decreased by over 50% in mice fed WDA compared to WD (4.6 ± 0.4% vs. 11.7 ± 2.1%, P < 0.01), but no reduction in atherosclerosis was observed in mice fed the WDB diet versus the WD diet (10.7 ± 1.5% vs. 11.7 ± 2.1%, P > 0.05). The effect of α-CD on atherosclerosis was then confirmed in a larger more extensive study, which included an additional control fiber (Figure 1). As before, one group of mice was fed a low-fat diet (LFD) and the other 4 groups were fed a Western diet (WD), WDA, WDB, or a Western diet supplemented with 1.5% oligofructose-supplemented inulin (WDI), a linear polysaccharide polymer that does not bind lipids [16]. As seen in the pilot study, mean % atherosclerotic lesion area in aorta of the WDA group was only 5.2 ± 0.5% (mean ± SEM) of total aorta area (Fig. 1A and and1B),1B), which was a 65% reduction compared to the lesion area for the WD group (15.0 ± 1.4%) and similar to the lesion area of the LFD group (4.9 ± 0.5%). In contrast, % lesion area in WDB and WDI were 13.3 ± 1.6%, 12.1 ± 1.4%, respectively, which were slightly less than the WD group but not statistically different. The combined data indicate that 1.5% α-CD supplementation but not 1.5% β-CD or oligofructose-enriched inulin significantly decreased atherosclerotic lesion area in aorta in apoE-KO mice.
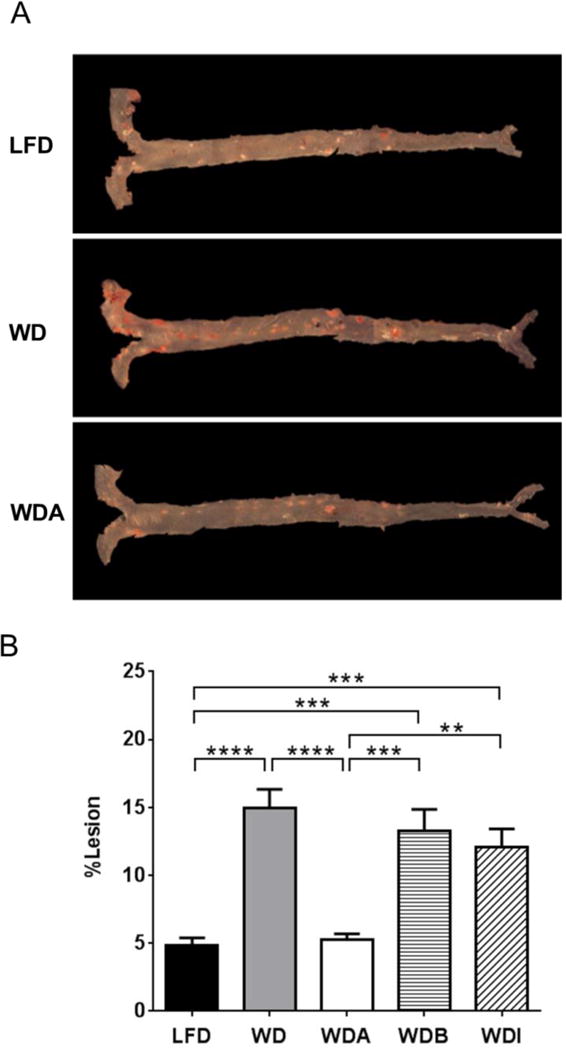
Representative images of aorta stained by Sudan IV (A) and comparisons of % atherosclerotic lesions in aorta among 5 diets (n=10–12 per group) (B). Data shown as mean ± SEM. **, ***, and **** represent p values of P < 0.01, P < 0.005 and P < 0.001, respectively.
3.2 α-CD supplementation does not cause major changes in plasma lipids, SAA, body weight or food consumption
As shown in Figures 2 and and3,3, α-CD supplementation did not significantly alter lipid profiles at the end of the 11-week study compared to other WD-based diets. The WDA group did have a modest and significantly lower levels of plasma FC (6.15 ± 0.30 mmol/L), particularly in VLDL after 2 weeks on the diet compared to mice in the WD group (7.28 ± 0.25 mmol/L)(P < 0.05), but this difference did not persist at 5 weeks and thereafter. No other major differences were observed for total cholesterol, esterified cholesterol or triglycerides for any other time point in the study between the WDA and WD groups (Supplemental Fig. 2). Similarly, supplementing the Western diet with β-CD or oligofructose-inulin also had negligible effects on plasma total lipids (Fig. 2).
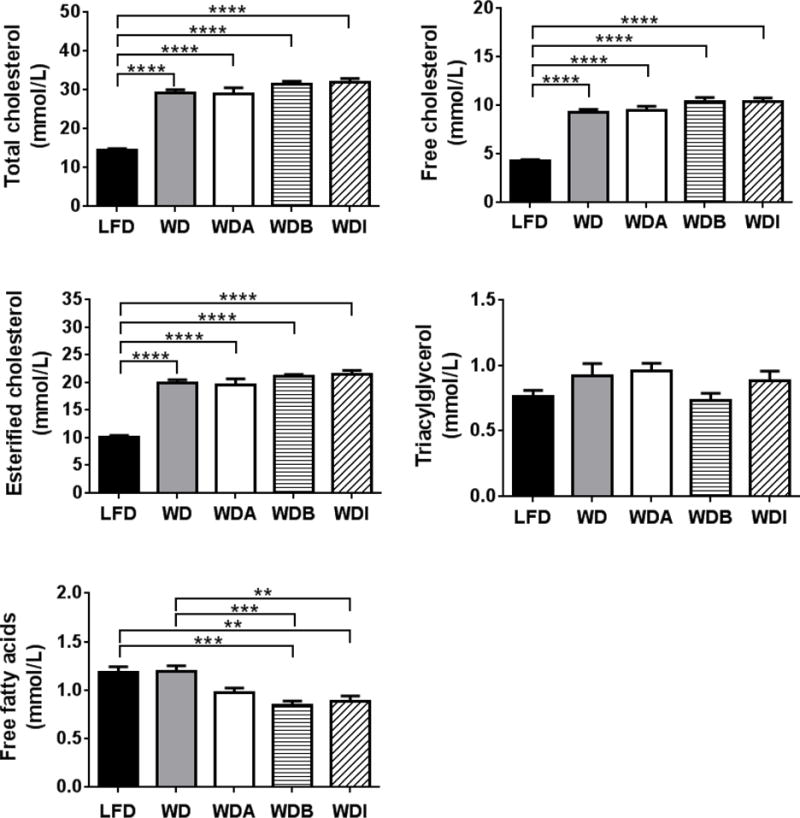
Plasma lipids in apoE-KO mice fed with the indicated diets for 11 weeks (n=10–12 per group). Data shown as mean ± SEM. **, ***, and **** represent p values of P < 0.01, P < 0.005 and P < 0.001, respectively.
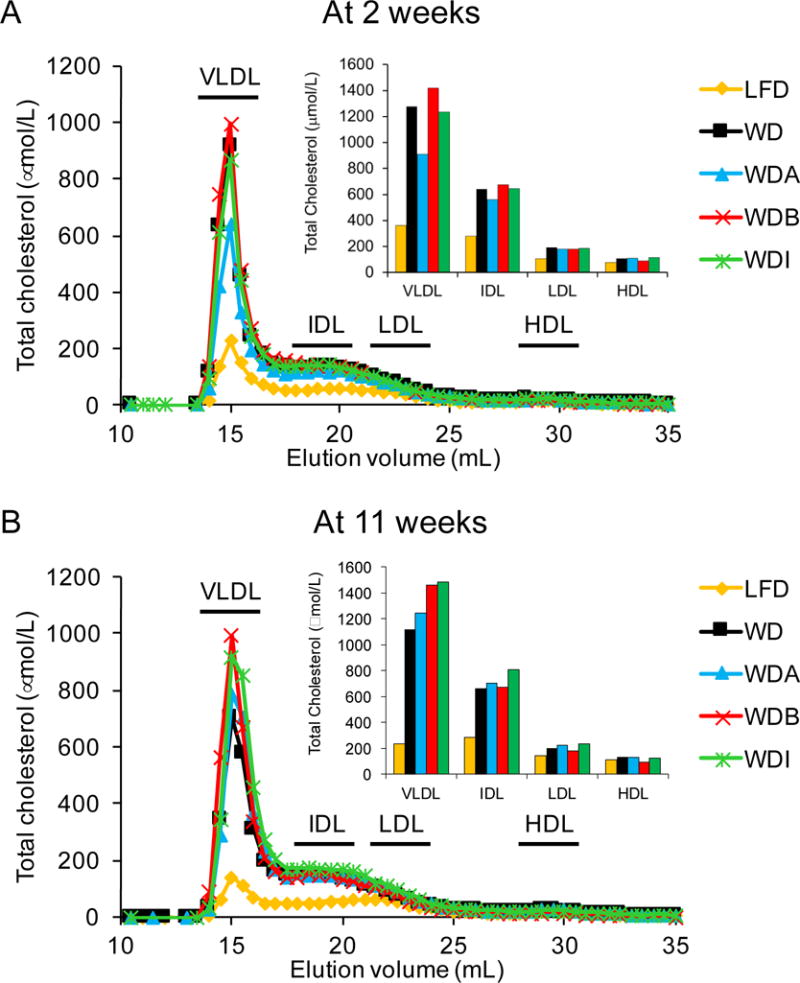
Pooled plasma (n=10–12 per group) from apoE-KO mice fed with the indicated diets for 2 weeks (A) or 11 weeks (B) was separated by FPLC and cholesterol was measured in the fractions. Inset: Sum of area under the curve for each lipoprotein fraction.
Interestingly, plasma levels of FFA in WDB (0.85 ± 0.05 mmol/L) and WDI (0.88 ± 0.06 mmol/L) were significantly lower than those in the LFD (1.20 ± 0.07 mmol/L) and WD (1.16 ± 0.06 mmol/L) groups (Fig. 2), but α-CD addition to the WD diet did not alter plasma FFA levels. As expected, mice in each WD-based diet group showed significant increases in TC, as well as FC and EC, compared to the LFD group at 11 weeks (Fig. 2), but there were no significant differences in plasma TG between the WD groups and the control LFD group.
FPLC analysis demonstrated increased cholesterol levels in the VLDL, IDL and LDL fractions in mice fed WD-based diets compared to the LFD at all time periods tested (Fig. 3). Consistent with total plasma lipid values, cholesterol levels in VLDL and IDL fractions in WDA group were lower than those in the other diet groups but only 2 weeks after starting the diet (Fig. 3A) and then reached similar levels as the other WD-based diet groups thereafter (Fig. 3B). α-CD consumption also did not alter plasma serum amyloid levels (SAA), a mouse acute phase protein [18], and did not affect body weight or food consumption (Supplemental Fig. 3).
3.3 β-CD but not α-CD supplementation decreases intestinal lipid absorption
Cholesterol absorption in C57BL/6 mice was determined using a radiotracer gavage study (Supplemental Fig. 4A). Lower cholesterol absorption compared to control was noted in all mice treated with either α– or β-CD, but only for the high dose β-CD (45 mg, comparable to levels of CD supplementation in WDA and WDB diets) was there a statistically significant difference. The mean cholesterol absorption for the high-dose β-CD group was approximately half of the control group cholesterol absorption (29.1 ± 11.0% vs. 54.7 ± 1.6%, P < 0.05). These findings are consistent with greater lipid binding we observed for β-CD with an in vitro lipid binding/lipolysis assay, using a pH-stat titration system (Supplemental Fig. 5), as previously described [19].
We also analyzed the distribution of cholesterol and TG in feces in mice fed a LFD or WD with and without added CDs (Supplemental Fig. 4B and 4C). Excretion of both fecal TC and TG were higher in the animals fed WD versus LFD but only mice fed the WDB diet, containing β-CD, had significantly increased TG in stool compared to the WD group. Overall, these results are consistent with prior studies showing an effect of β-CD on decreasing intestinal lipid absorption [7], whereas α-CD at the doses tested had minimal effect.
3.4 CD supplementation alters cecum content
Although α-CD supplementation of the Western diet did not greatly alter plasma lipids or fecal excretion, it has been reported that it can enhance fermentation by bacteria in the cecum [16]. As a measure of bacterial load and fermentation, we weighed the full cecum (cecum wall + cecum contents), cecum contents and emptied cecum (Fig. 4, Supplemental Fig. 4D and 4E). Mice fed with either WDA or WDI had significantly increased full cecum weights compared to WD consistent with increased fermentation. The weights of the cecum contents and emptied cecum also tended to increase for the various fiber supplemented diets compared to WD. Consistent with this observation, cecal pH also tended to decrease in WDA (8.33 ± 0.11, mean ± SEM), WDB (8.28 ± 0.07) and WDI (8.43 ± 0.06) compared to WD (8.38 ± 0.08) (LFD, 8.58 ± 0.11; ANOVA, P = 0.184; Supplemental Fig. 4F). A decrease in cecal pH is can occur by the fermentation of supplemented fibers by fecal bacteria, which results in the production of acidic SCFAs [13, 14, 20].
3.5 CD supplementation alters cecal microbiome
We next examined the abundance and distribution of gut microbes in the cecum of the mice on the various diets by next generation sequencing, using the MiSeq sequencer. Rarefaction analysis was performed, which allows the calculation of species richness for a given number of individual samples [21]. It is based on the construction of rarefaction curves, which plots the number of species as a function of the number of samples. The rarefaction analysis revealed the total number of microbial species detected by 16S rRNA gene sequencing and showed that the WD diet remarkably diminished the diversity of gut microbiota compared to LFD but also that adding the various CD’s to WD further decreased diversity, in the order WDA > WDI > WDB (Fig. 5A).
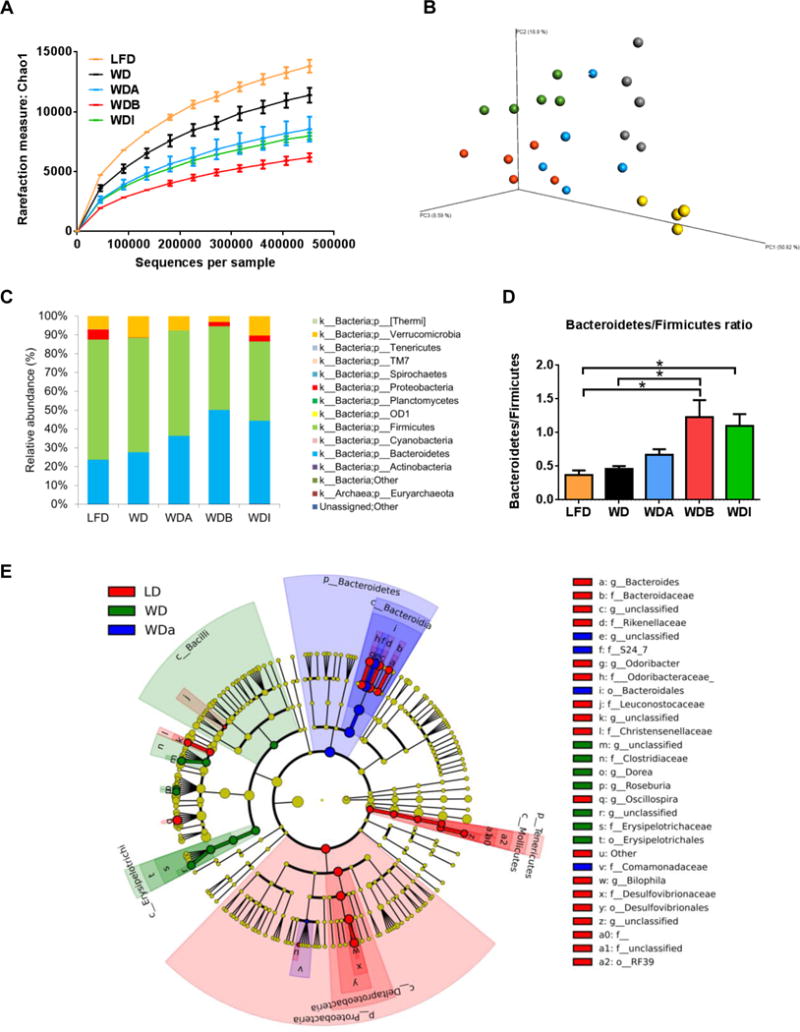
(A) α-diversity: Rarefaction analysis by counting total number of detected species in 16S rRNA gene sequencing. Data were indicated as mean ± SEM. (B) β-diversity: principal coordinates analysis (PCoA) of unweighted UniFrac distances of 16S rRNA genes. The plots (n = 4–5 in each diet group) were indicated as 3 dimensions by using three axis of Principal Coordinates (PC) 1, 2 and 3. Clusters of mice in each diet group are observed. (C) Proportions of gut flora in phyla level. (D) Ratio of Bacteroidetes/Firmicutes. Data were represented as mean ± SEM. (E) Taxonomy cladogram. Enriched microbes in LFD (red), WD (green) and WDA (blue) were represented by different colors; specific microbes enriched in each diet are listed alongside of the color for each diet at the right of the cladogram. * P < 0.05.
Pyrosequencing yielded a total of 41,283,706 quality sequences, with an average of 1,720,154 sequences per sample and identified a total of 14 phyla and 173 genera of bacteria. Principle Coordinates Analysis (PCoA) showed that each diet (n=4–5 per group) was well separated from others, indicating that the gut microbial communities were distinct among different diets (Fig. 5B).
Taxonomic composition of the gut microbiome at the phyla level was then analyzed as percentage of relative abundance for total microbes in each diet group (Fig. 5C). This analysis demonstrated that Firmicutes was the most predominant species in all groups (42.0%-63.8%), consistent with previous reports [22]. Bacteroidetes was the second most predominant species in LFD, WD and WDA, whereas it was almost the same percentage as Firmicutes in WDB and WDI. Accordingly, the ratio of Bacteroidetes/Firmicutes, which is considered to be more beneficial when the ratio is higher for obese mice fed with high fat diet [23], was increased in the order of WDA, WDI, WDB compared to WD (Fig. 5D). Two other notable changes were observed in the gut microbiome from the diets. Verrucomicrobia was over 10% in WD and WDI, whereas a lower percentage of this species were found in LFD (7.0%), WDA (7.6%) and WDB (3.1%) (Fig. 5C). We also observed that Proteobacteria was abundantly present in LFD, WDB and WDI (2.2–5.4%) but not in WD or WDA groups (0.02–0.04%) (Fig. 5C).
A cladogram of microbes significantly altered on each diet is shown in Fig. 5E. To identify statistical differential representation of the various taxa, linear discriminant analysis effect size (LEfSe) method was applied on the relative abundances [24]. For each significant taxon detected, the corresponding node and branch area in the taxonomic cladogram is colored, according to the highest-ranked diet group for that taxon. The LefSe result depicts that the phylum Proteobacteria was differentially represented in LFD, whereas Bacteroidetes was differentially represented in WDA, and Firmicutes was differentially represented in WD.
In accordance with the LefSe result, several bacteria species were found with statistically significant differences between the WD and WDA groups and also showed similar increases or decreases between WDA and LFD consistent with their possible role in modulating atherosclerosis (Fig. 6). For example, at the family level, the relative abundance of Comamonadaceae was decreased on WD but restored with WDA, and thus it shows an inverse relationship with atherosclerosis observed in mice and could be possible beneficial in reducing atherosclerosis. In contrast, the relative abundance of several bacteria, such as Erysipelotrichi at the class level, increased with WD and then decreased on WDA, suggesting that these bacterial groups could be contributing to the development of atherosclerosis. To further identify what changes in the species of gut flora best correlated with changes in atherosclerosis in WDA, the data was also presented as a heat map (Supplemental Fig. 6). Most species that showed a positive correlation with % atherosclerotic lesions in mice were also associated with decreased cecal fermentation (weights of cecum wall, cecum contents and full cecum). In contrast, most species that were negatively correlated with % atherosclerotic lesion were associated with increased cecal fermentation, as well as slightly decreased plasma cholesterol levels. Interestingly, Family Comamonadaceae also showed a strong negative correlation with % lesions in aorta, as well as negative correlations with total, free and esterified cholesterol.
3.6 CD supplementation alters the plasma metabolome
To gain further insight into the possible mechanism for the decreased atherosclerosis in the WDA group, the plasma metabolome of the mice on the various diets was examined by quantitative CE-TOF-MS analysis. A total of 201 plasma metabolites (102 metabolites in Cation mode and 99 metabolites in Anion mode) were detected, using a HMT’s standard library. Because previous studies have indicated a potential role for trimethylamine N-oxide (TMAO) made by gut microbes in atherosclerosis [15, 25], we analyzed this metabolite but did not find a significant difference in plasma TMAO levels (Supplemental Fig. 7) between WD and WDA. In addition, mRNA levels of flavin-containing monooxygenase 3 (Fmo3), which is known to produce TMAO in liver, determined by real-time PCR, did not differ significantly between WD and WDA (Fig. 7A). We did observe a reduction of trimethylamine (TMA), a precursor converted by the liver to TMAO, in the WDA group versus the WD group, but the significance of this observation is unclear given that the plasma TMAO levels were the same in each group. We also compared the level of plasma fatty acids (Fig. 7B), because of the prior association of SCFAs with the gut microbiome and atherosclerosis [11–14]. No differences were observed in SCFAs in the plasma of mice on the different diets, but decanoic acid, a medium chain fatty acid, was 81.3% higher on the WD diet compared to LFD and did not show as much as an increase when on the WDA diet. No other major differences in the other 201 detected plasma metabolites was observed between the different diet groups.
4 Discussion
Because α-CD is granted GRAS status and thus is generally recognized as safe, it is a widely used food additive. There has been a limited number of animal and human studies [3, 5, 17] indicating that α-CD may even have beneficial effects on plasma lipids, but there has not been a previous study on its effect on atherosclerosis. We show here in apoE-knockout mice that supplementation of a Western diet with α-CD markedly reduces atherosclerosis (Fig. 1), with only minimal changes in plasma lipids (Fig. 2).
Although plasma lipids are a major determinant of atherosclerosis both in mice and humans, many other factors can impact on the development of atherosclerosis. In humans, it is well known that serum lipids only account for about half of the variation in the propensity of atherosclerosis [17]. In mouse models of atherosclerosis, other types of treatment, such as inulin [16], blueberries [26], long-chain monounsaturated fatty acid-rich fish oil [27], and the angiotensin II type 1 receptor blocker telmisartan [28] all demonstrated decreased atherosclerotic lesions despite the absence of major effect on plasma lipids. Most relevant for this study, high fat diets supplemented with 10% oligofructose-enriched inulin diminished the development of atherosclerosis in apoE-KO mice, with an increase in cecal fermentation, despite minimal reductions of plasma TC and TG [16]. Another study demonstrated that inulin accelerated cecal fermentation in rats [14] and induced changes in gut flora, by especially increasing Bifidobacterium [29], which is known to be one of the gut microbes that helps maintain a healthy gut environment and, in fact, is used as a probiotic. In the present study, however, the difference in % atherosclerotic lesions in WDI vs. WD was not significantly lower, which may be due to the considerably lower amounts of inulin (1.5%, w/w) used in this study compared to previous studies (10%, w/w) [14,16]. Previous studies showing a benefit from β-CD supplementation on serum lipids and atherosclerosis in animal models [11,14] were also used at much higher levels (5–10%, w/w) than in our study.
No major hepatic gene expression changes in individual genes, such as Hmgcr and Cyp7a1, related to hepatic cholesterol/bile acid metabolism were observed in the mice on the different diets. In addition, Srebp2, a transcription factor that regulates numerous other genes, such as the LDL receptor and PCSK9, in the cholesterol/bile acid metabolism pathways [30], were unchanged (Supplemental Fig. 8). There was also not a significant difference in hepatic cholesterol and triglycerides levels between WD and WDA (Supplemental figure 3). Thus, it is not likely that changes in lipoprotein synthesis and catabolism accounts for the atheroprotection observed in the mice on the α-CD diet, but this should be tested in the future.
To furher explore the potential paradox between decreased atherosclerotic lesions without changes in plasma lipids by α-CD, we investigated whether the water-soluble fermentable fiber α-CD, like other prebiotics, may suppress the development of atherosclerosis by inducing changes in gut flora. The gut microbiota is now a well-recognized factor that may affect host metabolism and contribute to pathological conditions, such as obesity, diabetes and CVD [31]. Dietary fibers have been shown to prevent obesity by producing SCFA through bacterial fermentation of the dietary fiber in cecum [32]. Several animal studies also showed that oral administration of α-CD, β-CD or inulin produced SCFA and reduced the pH in the cecum [12, 14, 33]. Butyrate, a SCFA produced by bacteria, is the preferred energy source in enterocytes and high production of butyric acid may result in a beneficial trophic effect within the gastrointestinal tract [34]. In addition, SCFAs exhibited beneficial metabolic effects for obesity and insulin resistance in mice possibly through regulation of AMP kinase [35]. Interestingly, a recent report showed that a chow diet supplemented with 1% butyrate slowed the progression of atherosclerosis in aorta of apoE-KO mice by 50% compared to a normal chow diet [36].
We observed both indirect and direct evidence for changes in the gut microbiome by the addition of α-CD to a Western diet at relatively low doses (1.5%, w/w). First, similar to what has been previously reported [11, 13], the addition of α-CD to WD increased total cecal weight and content (Fig. 4), suggesting that it promoted increased bacterial fermentation by acting as an energy source. Similar changes, however, were observed with the other fibers that did not affect atherosclerosis. By 16S rRNA sequencing, we directly observed major changes in the abundance of numerous types of bacteria with α-CD supplementation, as well with the other types of fibers tested (Fig. 5, ,6).6). Although changes in the abundance of several groups of bacteria appeared to correspond to changes in mouse atherosclerosis, such as Comamonadaceae (Fig. 6, Supplemental. Fig. 6), it is difficult without more studies to attribute any of these bacterial species changes as the cause for the reduction in atherosclerosis. In case of fish oil supplementation, it has recently been shown in mice that it promotes the cecal growth of Akkermansia muciniphila, which correlates with decreased weight gain and improvements in glucose metabolism [37]. Some of these same benefits could also be observed in mice on lard diet after fecal transplants from mice fed fish oil diet [37] and a similar strategy could be used, in the future, to better understand the relationship between changes in the gut microbiome and atherosclerosis after α-CD supplementation.
Another possible way to better understand the relationship between the gut microbiome changes and atherosclerosis is to examine plasma metabolites, because both pro-atherogenic and anti-atherogenic metabolites altered by gut bacteria have been described. The best understood proatherogenic metabolite produced by bacteria is trimethylamine oxide TMAO, which is produced by the bacterial conversion of carnitine and phosphatidycholine to TMA and then to TMAO by Fmo3 in liver [15, 25]. We did not observe, however, a difference in TMAO levels on the different diets (Supplemental Fig. 7). Butyrate, as already discussed, is a short chain fatty acid produced by bacteria with anti-atherogenic properties, but we also did not observe any difference in this metabolite on the different diets, although it usually only accumulates in portal blood and not systemic blood [38], which we tested. We did detect a relatively large increase in systemic plasma levels of the medium chain fatty acid decanoic (C10:0) on WD (Fig. 7B), and this increase was blunted when α-CD was added to the diet. Decanoic acid is a potent PPAR-γ agonist and increases both mitochondrial oxidative phosphorylation and mitochondrial content of tissues [39]. In addition, it reverses some of the metabolic abnormalities in polycystic ovary syndrome [40]. Given its reported beneficial metabolic effects and the fact that it increased not decreased on the WD diet, the relevance of the observed changes in decanoic acid on atherosclerosis, in this study, is uncertain.
Given the important role of inflammation in the pathogenesis of atherosclerosis, differences in cytokine levels in the 4 diets could also possibly contribute to the lower atherosclerosis observed in mice on the WDA diet. However, no obvious differences were found between WD and WDA diets for plasma levels of SAA, MCP-1 and IL-6 or in hepatic gene expression levels of IL-6 and IL-10 (Supplemental Fig. 3C; Supplemental Fig. 8). Aortic tissue levels of cytokines were not measured in this study and should be investigated in the future.
In summary, dietary supplementation of α-CD to a high fat Western diet in apoE-knockout mice was found to markedly reduce atherosclerosis without significantly changing plasma lipids. Future studies will be necessary to determine if changes observed in the gut microbiome and plasma metabolites contribute to the anti-atherogenic properties of α-CD, but the results suggest that the current widespread supplementation of food with α-CD could have beneficial effects on cardiovascular health.
Table 1
Diet composition
(g/kg) | |||||
---|---|---|---|---|---|
LFD | WD | WDA | WDB | WDI | |
Casein | 195 | 195 | 195 | 195 | 195 |
DL-Methionine | 3 | 3 | 3 | 3 | 3 |
Sucrose | 120 | 341.46 | 341.46 | 341.46 | 341.46 |
Corn Starch | 432.99 | 150 | 150 | 150 | 150 |
Anhydrous Milkfat | 37.2 | 210 | 210 | 210 | 210 |
Cholesterol | 0 | 1.5 | 1.5 | 1.5 | 1.5 |
Cellulose | 50 | 50 | 35 | 35 | 35 |
Mineral Mix, AIN-76 (170915) | 35 | 35 | 35 | 35 | 35 |
Calcium Carbonate | 4 | 4 | 4 | 4 | 4 |
Vitamin Mix, Teklad (40060) | 10 | 10 | 10 | 10 | 10 |
Ethoxyquin, antioxidant | 0.01 | 0.04 | 0.04 | 0.04 | 0.04 |
alpha-Cyclodextrin | – | – | 15 | – | – |
beta-Cyclodextrin | – | – | – | 15 | – |
Oligofructose-enriched inulin | – | – | – | – | 15 |
Maltodextrin | 100 | – | – | – | – |
Soybean oil | 12.8 | – | – | – | – |
| |||||
Total | 1000 | 1000 | 1000 | 1000 | 1000 |
% by weight | |||||
LFD | WD | WDA | WDB | WDI | |
| |||||
Protein | 17.3% | 17.3% | 17.3% | 17.3% | 17.3% |
Carbohydrate | 61.3% | 48.5% | 48.5% | 48.5% | 48.5% |
Fat | 5.2% | 21.2% | 21.2% | 21.2% | 21.2% |
| |||||
% kcal | |||||
LFD | WD | WDA | WDB | WDI | |
| |||||
Protein | 19.2% | 15.2% | 15.2% | 15.2% | 15.2% |
Carbohydrate | 67.9% | 42.7% | 42.7% | 42.7% | 42.7% |
Fat | 13.0% | 42.0% | 42.0% | 42.0% | 42.0% |
Supplementary Material
Supplementary Figures
Supporting Information
Acknowledgments
α-CD and β-CD were a kind gift from Wacker Chemie AG. Oligofructose-enriched inulin was also kindly gifted from BENEO. We thank Dr. Megumi Nishimukai, Iwate University, Iwate, Japan, for helpful advice on procedures for lipid extractions of liver and feces, and cecal analysis. We thank the DNA Sequencing and Genomics Core, National Heart, Lung, and Blood Institute (NHLBI), National Institutes of Health (NIH), for sequencing of 16S rRNA using MiSeq. This research was supported by intramural National Institutes of Health funds from the National Heart, Lung, and Blood Institute.
Abbreviations
ANOVA | One-way analysis of variance |
CD | Cyclodextrin |
CE-TOF-MS | Capillary electrophoresis time-of-flight mass spectrometry |
EC | Esterified cholesterol |
FC | Free cholesterol |
FFA | Free fatty acid |
Fmo3 | Flavin-containing monooxygenase 3 |
FPLC | fast protein liquid chromatography |
KO | Knockout |
LFD | Low fat diet |
PCoA | Principle Coordinates Analysis |
PL | Phospholipids |
SAA | Serum amyloid A |
SCFA | Short chain fatty acid |
TC | Total cholesterol |
TG | Triglyceride |
LEfSe | linear discriminant analysis effect size |
TMA | Trimethylamine |
TMAO | Trimethylamine N-oxide |
V4 | fourth hypervariable |
WD | Western diet |
Footnotes
Author contributions: T.S. and A.T.R designed the study. A.S., B.L.V., M.J.A., M.J., S.G.T., Z.H.Y. and L.A.F. have carried out animal studies and the data analysis. Y.C., X.Z., X.W., Y.Z. and J.Z. performed the measurement of 16S rRNA of gut flora and analyzed the metagenomics data. T.S., Z.H.Y., L.A.F. and A.T.R wrote this manuscript. All authors read and approved the final version of this manuscript.
The authors have declared no conflicts of interest.
References
Full text links
Read article at publisher's site: https://doi.org/10.1002/mnfr.201600804
Read article for free, from open access legal sources, via Unpaywall:
https://europepmc.org/articles/pmc5548635?pdf=render
Citations & impact
Impact metrics
Citations of article over time
Alternative metrics
Smart citations by scite.ai
Explore citation contexts and check if this article has been
supported or disputed.
https://scite.ai/reports/10.1002/mnfr.201600804
Article citations
Impact of the gut microbiome on atherosclerosis.
mLife, 3(2):167-175, 01 Apr 2024
Cited by: 2 articles | PMID: 38948150
Review
Emodin Inhibited Pathological Cardiac Hypertrophy in Response to Angiotensin-Induced Hypertension and Altered the Gut Microbiome.
Biomolecules, 13(9):1274, 22 Aug 2023
Cited by: 0 articles | PMID: 37759673 | PMCID: PMC10526847
Oligosaccharides as Potential Therapeutics against Atherosclerosis.
Molecules, 28(14):5452, 17 Jul 2023
Cited by: 0 articles | PMID: 37513323 | PMCID: PMC10386248
Review Free full text in Europe PMC
Reduction of thyroid radioactive iodine exposure by oral administration of cyclic oligosaccharides.
Sci Rep, 13(1):6979, 28 Apr 2023
Cited by: 1 article | PMID: 37117274 | PMCID: PMC10147713
Tackling Atherosclerosis via Selected Nutrition.
Int J Mol Sci, 23(15):8233, 26 Jul 2022
Cited by: 12 articles | PMID: 35897799 | PMCID: PMC9368664
Review Free full text in Europe PMC
Go to all (12) article citations
Data
Data behind the article
This data has been text mined from the article, or deposited into data resources.
BioStudies: supplemental material and supporting data
Similar Articles
To arrive at the top five similar articles we use a word-weighted algorithm to compare words from the Title and Abstract of each citation.
The effect and mechanism of inulin on atherosclerosis is mediated by the characteristic intestinal flora and metabolites.
Coron Artery Dis, 35(6):498-508, 17 May 2024
Cited by: 0 articles | PMID: 38767579
Lingonberries reduce atherosclerosis in Apoe(-/-) mice in association with altered gut microbiota composition and improved lipid profile.
Mol Nutr Food Res, 60(5):1150-1160, 22 Mar 2016
Cited by: 38 articles | PMID: 26890232
Long-term Western diet fed apolipoprotein E-deficient rats exhibit only modest early atherosclerotic characteristics.
Sci Rep, 8(1):5416, 03 Apr 2018
Cited by: 12 articles | PMID: 29615808 | PMCID: PMC5882891
Berberine treatment increases Akkermansia in the gut and improves high-fat diet-induced atherosclerosis in Apoe-/- mice.
Atherosclerosis, 268:117-126, 24 Nov 2017
Cited by: 93 articles | PMID: 29202334
Funding
Funders who supported this work.
Intramural NIH HHS (1)
Grant ID: Z99 HL999999