Abstract
Free full text

Targeting cancer stem cells in drug discovery: Current state and future perspectives
Abstract
In recent decades, cancer stem cells (CSCs) have been increasingly identified in many malignancies. CSC-related signaling pathways and their functions provide new strategies for treating cancer. The aberrant activation of related signaling pathways (e.g., Wnt, Notch, and Hedgehog pathways) has been linked to multiple types of malignant tumors, which makes these pathways attractive targets for cancer therapy. CSCs display many characteristic features, such as self-renewal, differentiation, high tumorigenicity, and drug resistance. Therefore, there is an urgent need to develop new therapeutic strategies to target these pathways to control stem cell replication, survival, and differentiation. Notable crosstalk occurs among different signaling pathways and potentially leads to compensatory escape. Therefore, multitarget inhibitors will be one of the main methods to overcome the drug resistance of CSCs. Many small molecule inhibitors of components of signaling pathways in CSCs have entered clinical trials, and some inhibitors, such as vismodegib, sonidegib, and glasdegib, have been approved. Tumor cells are susceptible to sonidegib and vismodegib resistance due to mutations in the Smo protein. The signal transducers and activators of transcription 3 (STAT3) inhibitor BBI608 is being evaluated in a phase III trial for a variety of cancers. Structural derivatives of BBI608 are the main focus of STAT3 inhibitor development, which is another strategy for CSC therapy. In addition to the potential pharmacological inhibitors targeting CSC-related signaling pathways, other methods of targeting CSCs are available, such as nano-drug delivery systems, mitochondrion targeting, autophagy, hyperthermia, immunotherapy, and CSC microenvironment targeting. In addition, we summarize the latest advances in the clinical development of agents targeting CSC-related signaling pathways and other methods of targeting CSCs.
Core tip: The review aims to introduce the field of cancer stem cells (CSCs) and the important signaling pathways in CSCs and present approved inhibitors as well as candidate drugs. Due to the complexity of the crosstalk among various signaling pathways, current strategies involve the development of multitarget inhibitors, combination therapy, and precision treatments based on the genetic characteristics of patients. Other methods of targeting CSCs are introduced as well, including nano-drug delivery systems, mitochondrion targeting, hyperthermia, immunotherapy, and CSC microenvironment targeting. However, this field remains in its infancy, and considerable research will be required to produce mature products that can contribute to curing cancer.
INTRODUCTION
The concept of cancer stem cells (CSCs) was first proposed in 1983[1], and CSC self-renewal was posited as the core of tumor growth. Therefore, irreversible inactivation of stem cells is the key to killing tumor cells. In 1994, Lapidot et al[2] identified and isolated human acute myeloid leukemia (AML) cells expressing the stem cell surface marker phenotype CD34+/CD38-[3]. Cell transplantation experiments showed that compared with common tumor cells, CD34+/CD38- cells were tumorigenic and the surface antigens of these cells were similar to those of normal hematopoietic stem cells. In 2006, the American Association for Cancer Research clearly defined CSCs as a reservoir of self-sustaining cells with the exclusive ability to self-renew and maintain tumor growth[4].
In recent years, the existence of CSCs in solid tumors, including breast cancer[5,6], central nervous system tumors[7,8], prostate cancer[9,10], pancreatic cancer[11], liver cancer[12], lung cancer[13], colon cancer[14,15], melanoma[16,17], and nasopharyngeal carcinoma[18], has been confirmed, and the CSC model has been successfully established. CSCs generally have the following features (Figure (Figure1):1): (1) Unlimited self-renewal[19]: CSCs are able to produce progeny cells that are identical to the parental cells and maintain continuous tumors through self-renewal; (2) Differentiation potential[20]: CSCs can produce different lineages of differentiated progeny tumor cells; (3) High tumorigenicity[5]: A small number of CSCs cultured in vitro form colonies, and few CSCs are required to form tumors in vivo upon injection into experimental animals; this property is not shared by general tumor cells; and (4) Drug resistance[21]. The main factors for the development of drug resistance are as follows. First, most CSCs are in a resting or dormant state and are not undergoing cell division[22]. Second, CSCs mostly express ATP-binding cassette family membrane transporters[23], which are responsible for the transport and efflux of metabolites, drugs, toxic substances, endogenous lipids, peptides, nucleotides, and sterols and can render these cells resistant to many chemotherapeutic drugs. In addition, CSCs have a powerful DNA repair ability[24]. Finally, the abnormal expression of signaling pathway components and the diversification of the CSC microenvironment are also related to drug resistance. At present, the presence of CSCs is believed to be the main cause of chemotherapy and radiotherapy failure[25].
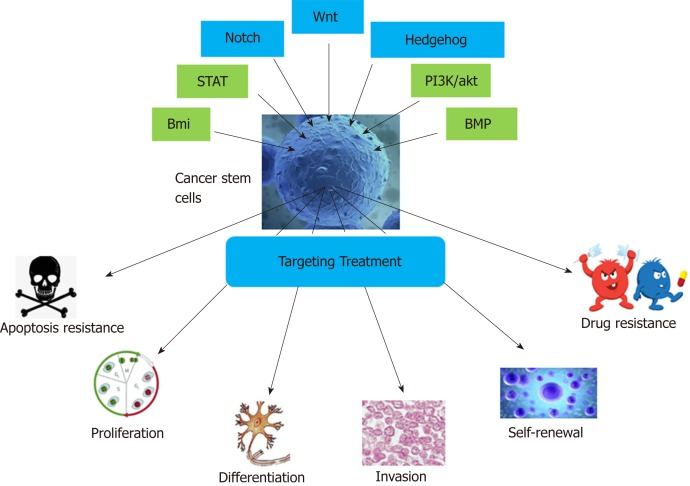
Targeting Wnt, hedgehog, notch, bone morphogenetic protein, B-cell-specific Moloney murine leukemia virus integration site, PI3K/Akt, and signal transducers and activators of transcription signaling pathways and the characteristics of cancer stem cells. Bmi: B-cell-specific Moloney murine leukemia virus integration site; STAT: Signal transducers and activators of transcription; BMP: Bone morphogenetic protein.
Currently, researchers have not only confirmed the existence of CSCs but also identified the specific surface markers of many types of CSCs[26,27] and their signal transduction pathways. Many chemical agents of different classes targeting the Wnt, Notch, Hedgehog, signal transducers and activators of transcription (STAT), bone morphogenetic protein (BMP), Bmi, and PI3K/Akt pathways have entered clinical trials (Figure (Figure1).1). In this review, the approval status and progress of these investigational agents are summarized. In addition to the potential pharmacological inhibitors targeting CSC-related signaling pathways, other methods of targeting CSCs, such as nano-drug delivery systems (NDDSs), mitochondrion targeting, autophagy, hyperthermia, immunotherapy, and CSC microenvironment targeting, are also summarized.
CSC SIGNALING PATHWAYS AND INHIBITORS
There are two main theories about the possible formation of CSCs: From normal stem cells and from non-stem cells. Studies have shown that CSCs are formed by the transformation of adult stem cells caused by genetic mutations. Normal stem cells have activated self-renewal mechanisms, have longer survival time, and can accumulate more mutations; thus, they have more opportunities to mutate into CSCs[28-30]. Therefore, we hypothesize that gene mutations in normal adult stem cells are caused by endogenous or exogenous stimuli, and then they enter the cell cycle, rapidly divide, and transform into CSCs. Moreover, some differentiated cells may also regain self-renewal capacity before canceration and mutate into CSCs[31,32]. Because CSCs are derived from normal cells, their signaling pathways are similar to those of normal cells. The main pathways affecting CSCs include the Wnt, Hedgehog, Notch, BMP, Bmi, PI3K/Akt, and STAT pathways[33], which regulate CSC self-renewal and differentiation. Among them, the Wnt, Hedgehog, and Notch pathways are the most thoroughly studied[34].
Wnt signaling pathway and inhibitors
The Wnt signaling pathway regulates cell proliferation, differentiation, and apoptosis and cell-cell interactions and plays an important role in processes involved in embryogenesis and tissue repair[35,36]. Extracellular Wnt protein can trigger different intracellular signal transduction pathways, which are classified as Wnt/β-catenin dependent (canonical pathway) or β-catenin independent (noncanonical pathway). The canonical pathway is activated by the binding of Wnt ligands to the low-density lipoprotein receptor (LRP)-5/6 receptor and the Frizzled (Fzd) receptor. Subsequently, this complex activates the cytoplasmic protein disheveled (Dvl), resulting in the recruitment of protein complexes (axin, GSK-3β, casein kinase 1, and adenomatosis polyposis coli protein) to the receptor[37-39]. The Wnt-Fzd-axin-LRP-5/6 complex sequesters cytosolic GSK-3β, blocking it from phosphorylating β-catenin. As a result, unphosphorylated β-catenin accumulates in the cytoplasm and migrates to the nucleus, thus leading to the transcription of target genes such as c-Myc and cyclin D1[39], which promote the abnormal proliferation of tumor cells.
Examples of the β-catenin-independent pathway include the Wnt/Ca2+ pathway and the planar cell polarity (PCP) pathway[40]. In the Wnt/Ca2+ pathway, Wnt5a and Wnt11 bind to and activate the Fzd receptor to activate Dvl, which in turn inhibits cGMP-dependent protein kinase and activates phospholipase C (PLC), resulting in increased Ca2+ release; moreover, PLC promotes the accumulation of Ca2+ through the generation of inositol 1,4,5-trisphosphate (IP3). Increased Ca2+ levels activate the protein kinases calmodulin-dependent protein kinase II (CaMK-II), protein kinase C, and calcineurin[41]. CaMK-II can phosphorylate T-cell factor (TCF) via transcriptional growth factor β-activated kinase 1 and NEMO kinase, and phosphorylated TCF loses the ability to bind β-catenin. Therefore, this pathway antagonizes the Wnt/β-catenin-dependent pathway[42]. The PCP pathway can be initiated by the Wnt interaction with Fzd receptors or the coreceptors receptor related to tyrosine kinase and retinoic acid-related orphan receptor, which leads to Dvl activation[43]. Myosin and Rho-associated kinase are activated by Rho GTPase and alter actin activity and the cytoskeletal arrangement. Tandem activation of Rac GTPase and Rac stimulates c-Jun N-terminal kinase (JNK) activity[44]. In addition to its regulation of cell proliferation, JNK is involved in a series of physiological processes, such as cell growth, differentiation, migration, and cancer[45].
The canonical Wnt signaling pathway may be involved in the development of malignant breast cancer, which is manifested by elevated β-catenin levels in the nucleus and cytoplasm. In breast cancer patients, elevated β-catenin often indicates a poor prognosis, which may be due to β-catenin mutations[46]. Cyclin Y (CCNY) promotes the proliferation of ovarian cancer cells through the Wnt/β-catenin signaling pathway. Overexpression of CCNY enhances the expression of the Wnt downstream target genes c-Myc, cyclin D1, PFTK1, and N-acetylglucosamine transferase[47]. Downregulation of tumor necrosis factor α-induced protein 2 can inhibit the proliferation of esophageal squamous cell carcinoma by downregulating the downstream target genes of β-catenin (e.g., c-Myc and cyclin D1) and upregulating E-cadherin and p-GSK-3β levels[48]. Silencing of the Golgi phosphoprotein 3 (GOLPH3) gene can inhibit the proliferation of SW620 colon cancer cells, in which β-catenin is downregulated and Wnt pathway activity is decreased. Conversely, overexpression of the GOLPH3 gene promotes colon cancer cell proliferation[49]. Reishi inhibits the proliferation and migration of breast cancer cells by inhibiting the Wnt signaling pathway, and the main mechanism of action is to block the Wnt pathway by inhibiting the phosphorylation of the Wnt coreceptor low-density lipoprotein receptor-related protein 6 (LRP6). Reishi can significantly reduce LRP6 phospho-rylation and inhibit the Wnt3a-mediated expression of the target gene Axin2 in human and mouse breast cancer cell lines[50]. Breast cancer, melanoma, prostate cancer, lung cancer, and other cancers can be generated by altering the expression of the CTNNB1 gene, which encodes β-catenin.
The increased expression of Wnt ligands, such as Wnt1, Wnt2, and Wnt7a, leads to glioblastoma, esophageal cancer, and ovarian cancer, respectively[51]. Deregulation of the Wnt signaling pathway has been found to be associated with many cancers, making it an attractive target for anticancer therapies. The developed Wnt signaling pathway inhibitors are mainly monoclonal antibodies, β-catenin inhibitors, and various small molecule inhibitors. The discovery of small molecule inhibitors has been a research focus, with the main goal of finding small molecules that affect nuclear β-catenin and Wnt signaling pathways through high-throughput screening of small molecule compound libraries. Agents targeting the Wnt signaling pathway that are in clinical trials are summarized below (Table (Table1,1, Figure Figure22).
Table 1
Agents targeting the Wnt signaling pathway in clinical trials
Compound | Target/mechanism | Tumor type | Highest phase | Organization |
LGK974[53] | Porcupine inhibitor | Melanoma; breast cancer; pancreatic cancer | Phase I | Novartis |
ETC-159[54] | Porcupine inhibitor | Solid tumors | Phase I | ETC/Duke-NUS |
PRI-724[55] | β-catenin/CBP | Myeloid leukemia | Phase I/II | Prism/Eisai Pharmaceuticals |
XAV939[59] | Tankyrase 1 and 2 inhibitor | - | Preclinical | Novartis |
IWR1[61] | Tankyrase 1 and 2 inhibitor | - | Preclinical | Tocris Bioscience |
JW74[70] | Tankyrase 1 and 2 inhibitor | - | Preclinical | Tocris Bioscience |
NSC668036[71] | Disheveled | - | Preclinical | Tocris Bioscience |
OMP-18R5[64] (Vantictumab) | Frizzled receptor | Solid tumors; breast cancer; non-small cell lung cancer; pancreatic cancer | Phase I/Ib | OncoMed Pharmaceuticals |
OMP-54F28[65] (Ipafricept) | Fzd8-Fc fusion protein | Solid tumors; hepatocellular carcinoma; ovarian cancer; pancreatic cancer | Phase I/Ib | OncoMed Pharmaceuticals |
Porcupine is an acyltransferase that plays an important role in the formation and secretion of Wnt ligands[52]. Among the currently reported small molecule inhibitors of porcupine, LGK974[53] and ETC-159[54] have successfully entered phase I clinical trials. Studies have shown that LGK974 can inhibit Wnt signaling by reducing LRP6 phosphorylation and Wnt target gene expression both in vivo and in vitro, and LGK974 has significant effects in both tumor models and human cancer cell lines in mice. However, ETC-159, the first small molecule for the treatment of colorectal cancer, was subsequently discovered[54]. PRI-724 is a potent inhibitor of the Wnt signaling pathway that inhibits the binding and recruitment of β-catenin and its coactivator CBP[55]. PRI-724 specifically binds to CBP, disrupting the interaction between CBP and β-catenin. PRI-724 selectively induces apoptosis and inhibits the growth of colon cancer cell lines in vitro but has no effect on normal colonic epithelial cells[56,57].
Tankyrase belongs to the poly(ADP-ribose) polymerase (PARP) family and has two isoforms, tankyrase 1 (PARP5a) and tankyrase 2 (PARP5b), which are associated with Wnt/β-catenin signaling. Both of these isoforms increase axin degradation through the ubiquitin-proteasome pathways[58]. XAV939 specifically inhibits tankyrase PARP activity[59]. Treatment with 1.0 μM XAV939 for 12 h reduced DNA-PKcs protein levels to a minimum level, and the relative expression was less than 25% of that in the control group[60]. IWR-1 and XAV939 act as reversible inhibitors of the Wnt pathway and have similar pharmacological effects in vivo and in vitro. However, IWR-1 functions through interacting with axin and XAV939 directly binds to TNKS[61].
Monoclonal antibodies against Wnt-1 and Wnt-2 have proven that Wnt inhibition leads to tumor suppression in melanoma, sarcoma, colorectal cancer, non-small cell lung cancer (NSCLC), and mesothelioma[62,63]. OMP-18R5 (vantictumab), developed by OncoMed Pharmaceuticals/Bayer, is a monoclonal antibody that targets five of the ten Fzd receptors. The safety and efficacy of these antibody therapeutics are being assessed separately or in combination with chemotherapy in NSCLC, pancreatic cancer, and breast cancer[64]. The first-in-class recombinant fusion protein ipafricept (OMP-54F28) blocks Wnt signaling by binding to Wnt ligands[65]. Among this class of therapeutics, for example, vantictumab[66], ipafricept[67], and ETC-159[68], which show anti-CSC effects in preclinical model experiments, are in clinical trials to treat cancer patients[69].
Notch signaling pathway and its inhibitors
The Notch signaling pathway is widespread and highly conserved in mammals, and it has various functions and a relatively simple molecular structure[72-75]. The Notch pathway is mainly composed of Notch receptors (Notch 1-4) and Notch ligands (Jagged 1, Jagged 2, Delta-like ligand (DLL)-1, DLL-3, and DLL-4). The DNA binding protein CSL [CBF1/RBP, Su(H)/Lag-1] is the primary effector of the Notch signaling pathway. CSL binds to activated Notch to form the Notch intracellular domain (NICD)-CSL complex.
The Notch signaling pathway is divided into the non-CSL-dependent pathway (noncanonical Notch signaling pathway) and the CSL-dependent pathway (canonical Notch signaling pathway)[76], and these pathways maintain the existence of stem cells and initiate embryonic or fetal cell differentiation[77]. The Notch pathway affects the normal growth and development of cells and tissues by regulating cell differentiation, proliferation, and apoptosis, which play core roles in the development of malignant tumors[78,79].
The canonical Notch signaling pathway involves the binding of receptor and ligand, which releases the NICD via metalloproteinase- and γ-secretase-mediated protease hydrolysis. Then, the NICD enters the nucleus and forms a complex with RBPJ, which mediates the transcription and expression of its downstream target genes Hes and Hey, thus affecting cell differentiation, proliferation, and apoptosis. During the activation and transduction of the Notch signaling pathway, enzymatic cleavage by γ-secretase plays an extremely important role. The inhibition of γ-secretase prevents the release of NICD, thereby blocking the Notch signaling pathway[80]. Treatment strategies directly related to the Notch pathway involve γ-secretase inhibitors (GSIs) and inhibitors of Notch receptors, ligands, or other pathway elements.
Currently, several classes of Notch signaling inhibitors are being clinically researched, and they have significant differences in targets, mechanisms, and tumor types (Table (Table2,2, Figure Figure3).3). A major class of agents targeting the Notch pathway is GSIs, which are the first Notch inhibitors to enter clinical studies[81]. Favorable tissue penetration, low cost, ease of administration, and potential pan-Notch inhibitory activity are the main advantages of GSIs[82,83]. However, the toxicity of these agents, especially the serious toxicity in the gastrointestinal tract, may affect the further development of this class of inhibitors[84]. Moreover, GSIs have a variety of substrates, such as Notch receptors, cadherin, ERBB4, CD44, and amyloid precursor protein, and some scholars believe that GSIs are not druggable[85]. RO4929097 showed insufficient activity as a single agent against metastatic melanoma and platinum-resistant ovarian cancer in clinical trials[86,87], which indicated that it has potential in combination with other drugs to treat related diseases[88,89].
Table 2
Agents targeting the Notch signaling pathway in clinical trials
Compound | Target/mechanism | Tumor type | Highest phase | Organization |
MK-0752 | γ-secretase inhibitor | Breast cancer | Phase I | Merck |
LY-900009 | γ-secretase inhibitor | Metastatic cancer/lymphoma | Phase I | Perrigo and Lilly |
Crenigacestat | Notch signaling inhibitor | T-cell acute lymphoblastic leukemia | Phase I/II | Lilly |
CB-103 | Notch signaling inhibitor | Solid tumors; hematologic cancers | Phase I/II | Cellestia Biotech |
LY-3056480 | Notch signaling inhibitor | Sensorineural hearing loss | Phase I/II | Lilly |
RO4929097 | γ-secretase inhibitor | Kidney cancer; pancreatic cancer; metastatic cancer; prostate cancer; glioblastoma multiforme; metastatic melanoma | Phase II | SpringWorks Therapeutics |
BMS-906024 | Notch signaling pathway | Adenoid cystic carcinoma | Phase II | Bristol-Myers Squibb |
Nirogacestat | γ-secretase inhibitor | Desmoid tumors | Phase II | SpringWorks Therapeutics |
Enoticumab | Anti-DLL4 | Advanced solid malignancies | Phase I | Regeneron and Sanofi |
Nirogacestat (PF-3084014) is another GSI in phase II clinical trials for the treatment of desmoid tumors. Long-term follow-up of patients with desmoid fibromatosis treated with nirogacestat showed that it had promising activity (objective response rate of 71.4%) at relatively low doses and high tolerability, thus leading to prolonged disease control[90,91]. Nirogacestat was also reported to show antitumor and antimetastatic effects in hepatocellular carcinoma[92]. Pretreatment of multiple myeloma (MM) CSCs with the γ-secretase inhibitor RO4929097, a Notch pathway inhibitor, can reverse bruceantin-induced effects on MM-CSC proliferation[93].
BMS-906024, a potent pan-Notch inhibitor, showed robust efficacy in vivo at tolerated doses in Notch-driven leukemia and solid tumors[94]. In one study, BMS-906024 enhanced the efficacy of paclitaxel in lung adenocarcinoma and improved the patient’s response to combination therapy[34]. Notably, whether a specific chemical class of GSIs is preferable with regard to safety and efficacy remains unclear, and the ongoing clinical and preclinical studies will provide valuable data.
DLL4 is the only Notch ligand specifically expressed in endothelial cells. The DLL4-mediated Notch pathway plays a vital role in regulating angiogenesis, especially in regulating embryonic vascular development and tumor angiogenesis[95,96]. The DLL4/Notch pathway in adult vasculature mainly inhibits the activity, migration, and differentiation of endothelial cells, thereby impeding angiogenesis and maintaining vascular stability[97,98]. Generally, vascular endothelial growth factor (VEGF) induces DLL4 production in endothelial cells, which promotes the activation of the Notch signaling pathway, downregulates VEGF3, and promotes endothelial cell sprouting[99]. Therefore, targeting DLL4 with monoclonal antibodies is another strategy to block Notch signaling, and this concept is being tested in the clinic. Enoticumab is a fully human IgG1 antibody to DLL4 in phase I clinical development for the treatment of advanced solid malignancies. Intravenous enoticumab was found to have promising clinical activity in phase I safety, dose-limiting toxicity, and pharmacokinetics studies in patients with solid tumors[100]. However, it should be noted that chronic treatment with anti-DLL4 monoclonal antibodies causes hemangiomas in animal models[101].
Hedgehog signaling pathway and its inhibitors
The Hedgehog signaling pathway plays a significant role in mammalian development, is involved in the regulation of cell proliferation, survival, and differentiation[102,103], and is responsible for the formation of the neural tube, axial bone, hair, and teeth[104-106]. The Hedgehog gene is inhibited in most adult tissues and involved in the maintenance and repair of only certain tissues. Abnormally activated Hedgehog signaling directly regulates various growth factors and promotes the proliferation and survival of tumor cells.
When the Hedgehog ligand (Hh) is absent, Patched (Ptch) inhibits the activation of Smoothened (Smo), glioma-associated oncogene homolog (Gli) is suppressed by a protein complex mainly composed of suppressor of fused (Sufu), and Gli is phosphorylated and unable to enter the nucleus. In the presence of Hh, Ptch is bound by Hh, which relieves Ptch-mediated Smo inhibition. Activated Smo promotes the dissociation of the Sufu and Gli complex, allowing Gli to enter the nucleus and trigger downstream target gene expression, which results in the activation of the canonical Hedgehog signaling pathway[107]. To date, the most successful drugs targeting the Hedgehog signaling pathway are the three Smo inhibitors vismodegib, sonidegib, and glasdegib, which have been approved by the Food and Drug Administration (FDA) for the treatment of basal cell carcinoma (BCC). Meanwhile, many other Smo inhibitors are being clinically researched and have therapeutic effects on a variety of tumors. In addition, Gli inhibitors and Shh ligand inhibitors are in the preliminary stage of research.
Smo inhibitors: Smo is a major target for Hedgehog signaling pathway inhibitors. Inhibiting Smo activity can directly block the activation of the downstream transcription factor Gli and thus inhibit the expression of genes downstream of the Hedgehog signaling pathway. However, Smo-specific inhibitors are prone to drug resistance. Currently, resistance to two launched Smo inhibitors has been observed, and ubiquitous toxic side effects limit their application. The following agents targeting the Smo protein are under development in clinical trials (Table (Table3,3, Figure Figure44).
Table 3
Agents targeting the Smoothened protein in clinical development
Compound | Target/ mechanism | Cancer type | Highest phase | Organization |
Vismodegib (GDC-0449) | Smo | Basal cell carcinoma | Launched | Roche |
Sonidegib (NVP-LDE225) | Smo | Basal cell carcinoma | Launched | Novartis |
Glasdegib | Smo | AML | Launched | Pfizer |
Patidegib | Smo | Basal cell nevus syndrome; skin cancer | Phase II | PellePharm |
BMS-833923 | Smo | Leukemia | Phase II | Bristol-Myers Squibb |
Taladegib | Smo | Esophageal cancer; gastroesophageal junction cancer; solid tumors; small cell lung carcinoma | Phase I/II | Ignyta |
G-024856 | Smo | Basal cell carcinoma | Phase I | Roche |
LEQ-506 | Smo | Advanced solid tumors | Phase I | Novartis |
Smo: Smoothened.
Applications for the approval of vismodegib, a first-in-class small molecule inhibitor of Hedgehog signaling, were filed by Genentech in the United States and the E.U. in 2011 for the treatment of advanced BCC. In 2012, vismodegib was approved by the FDA and launched. Phase II clinical development is ongoing for the treatment of breast cancer, gastrointestinal cancer, pancreatic cancer, chondrosarcoma, keratocystic odontogenic tumor, medulloblastoma, and meningioma. This drug is also in phase I/II clinical trials for the treatment of prostate cancer and sarcoma. Treatment of HCC38 cells, a triple-negative breast cancer (TNBC) stem cell line, with vismodegib significantly decreased TNBC cell proliferation, cell invasion, and mammosphere formation while inducing cell apoptosis by inhibiting the protein expression or phosphorylation of downstream signaling molecules. Tumor formation and growth of HCC1806 cells (another TNBC stem cell line) pretreated with vismodegib are effectively suppressed in xenograft mouse models. Treatment with vismodegib may provide a novel alternative therapeutic strategy against TNBC that targets breast CSCs (BCSCs), and it could provide promising insights for clinical applications in patients with TNBC[108].
A Smo mutation (D473H) was identified in tumor samples from a medulloblastoma patient who relapsed after an initial response to vismodegib. Acquired mutations in a serpentine receptor with features of a G protein–coupled receptor can lead to vismodegib resistance in human cancer[109]. Overall, vismodegib could represent an important treatment option for patients with advanced BCC.
Sonidegib, the second Smo antagonist developed by Novartis, was launched in the U.S. in 2015 for the treatment of advanced BCC. Phase II clinical trials are ongoing for the treatment of MM, and phase I/II clinical trials are underway for the treatment of pancreatic adenocarcinoma, myelofibrosis, and essential thrombocythemia. A variety of murine Smo mutations that lead to sonidegib resistance have been found in mouse models of medulloblastoma[110].
Glasdegib, developed by Pfizer, is a Hedgehog pathway inhibitor that was approved and launched in the U.S. in 2018 for the oral treatment of newly diagnosed AML. Pfizer is also conducting phase II clinical trials on the treatment of myelo-dysplastic syndrome and chronic myelomonocytic leukemia.
As single-target agents, the approved Smo inhibitors have common drug resistance problems. For example, Smo mutations in the tumor tissue of patients with medulloblastoma cause vismodegib to lose efficacy. A variety of drug-resistant Smo mutants were found in a mouse tumor model treated with sonidegib, which further indicates the limitations of sonidegib for cancer treatment. To overcome drug resistance and the side effects of traditional Smo inhibitors, novel Hedgehog signaling pathway inhibitors must be developed.
Gli inhibitors: The Gli transcription factor is the terminal component of the Hedgehog signaling pathway, and it is also regulated by other important signaling pathways. Activation of Gli1 and Gli2 promotes tumor growth, survival, angio-genesis, and drug resistance[111].
Arsenic trioxide (ATO) is an FDA-approved drug for the treatment of acute promyelocytic leukemia, and ATO was later discovered to be a Gli transcription factor inhibitor and entered as such into phase II clinical trials[112]. Studies have shown that ATO inhibits the Hedgehog signaling pathway by directly binding to Gli1 and Gli2[113]. GANT-61 is another Gli inhibitor developed by the U.S. National Cancer Institute that significantly inhibits DNA binding to Gli1 and Gli2[114]. GANT-61 can induce the apoptosis of AML cells, inhibit cell proliferation, and enhance the cytotoxic effect of cytarabine on cancer cells[115]. This drug is currently under preclinical study.
Other signaling pathways and inhibitors
In addition to the well-studied Wnt, Hedgehog, and Notch signaling pathways, the BMP, Bmi, PI3K/Akt, and STAT signaling pathways also harbor abnormalities that can lead to tumor development. The STAT family is a group of cytoplasmic proteins that are activated by cytokine receptors, and they act as a transducer in the process of receptor-mediated cytokine signaling, and subsequently, extracellular signals are transmitted to the nucleus to ultimately regulate downstream gene transcription[116]. There are multiple subfamilies of the STAT family; for example, STAT3 signaling regulates the self-renewal, differentiation, and apoptosis of CSCs[117]. Napabucasin (BBI608) is a first-in-class STAT3 inhibitor in phase III clinical trials sponsored by Sumitomo Dainippon Pharma for the treatment of metastatic colorectal carcinoma and pancreatic cancer (Figure (Figure5).5). In one study, BBI608 inhibited stemness gene expression, depleted CSCs, and overcame cisplatin resistance in NSCLC[118]. Napabucasin is effective in mice as a monotherapy or in combination with other agents.
In 2015, Li et al[119] demonstrated that BBI608 has strong anti-CSC effects in vitro and in vivo in a broad range of cancer types. Zhang et al[120] confirmed that BBI608 is able to kill prostate CSCs, inhibit CSC properties, and inhibit the expression of stemness-related genes. Currently, BBI608 is being assessed in clinical trials, and the results suggest that BBI608 is a potent anti-tumorigenic and anti-CSC drug in different tumor types[118,121-123]. To further improve the pharmacokinetic properties of BBI608 and its antitumor activity, our research team designed and synthesized a series of BBI608 derivatives that display stronger inhibitory activity than BBI608 in HepG2 cells[124].
B-cell-specific Moloney murine leukemia virus integration site 1 (Bmi-1) is a member of the PcG family and a nuclear chromatin regulatory factor. The encoded protein plays an important role in tumorigenesis[125]. The Bmi-1 gene is highly expressed in many malignant tumors and closely related to tumor invasion, metastasis, and recurrence. Decreased Bmi-1 gene expression in embryos and adults leads to defects in stem cell proliferation and self-renewal[126]. Bmi-1 is required for the self-renewal and maintenance of acute myelocytic leukemia stem cells[127]. PTC-596 is an oral Bmi-1 inhibitor in phase I clinical development for the treatment of female reproductive system cancer. Studies using mouse xenograft models demonstrated that PTC-596 inhibited leukemia cell growth in vivo while sparing normal hematopoietic cells[128].
BMP, an important member of the transforming growth factor-β (TGF-β) family, represents a class of secreted glycoproteins that induce ectopic bone and cartilage formation, and it is involved in many important biological processes, such as embryonic development, cell differentiation, and organ formation[129]. BMP-7 has been shown to reduce macrophage infiltration and tissue damage in animal models of acute and chronic renal failure[130]. BMP-7 was originally approved in 2001 for the treatment of the nonunion of long bone fractures secondary to trauma, and it has also been studied in phase II/III trials for the treatment of periodontal disease.
The PI3K/Akt signaling pathway is important in various types of cells, in which it regulates cell growth, proliferation, differentiation, apoptosis, and cytoskeletal rearrangement. Akt, a downstream molecule of PI3K, is a serine/threonine protein kinase. The PI3K/Akt signaling pathway is both a convergence and divergence point for many cell signals that regulate the phosphorylation of signaling molecules, and this pathway engages in crosstalk with the BMP/Smad and Wnt/β-catenin pathways, among others. Then, downstream signaling affects the expression of nuclear transcription factors, ultimately regulating cell proliferation, self-renewal, and multidirectional differentiation potential[130,131]. GDC-0084 (Figure (Figure5),5), a small molecule inhibitor of the PI3K/AKT/mTOR pathway, is being studied in a phase II clinical trial for the treatment of glioblastoma multiforme (GBM). GDC-0084, which is distributed throughout the brain and intracranial tumors, potently inhibits the PI3K pathway[132]. In vitro and in vivo experiments showed that GDC-0084 can inhibit human cutaneous squamous cell carcinoma cell growth by blocking PI3K-Akt-mTOR and DNA-PKcs signaling[133].
Adverse effects of inhibitors
ETC-159 has shown dose-limiting toxicities, including hyperbilirubinemia and skeletal fragility fractures[134]. The preliminary results of the clinical phase I trial are available, and they showed that PRI-724 results in tertiary reversible hyperbil-irubinemia with dose-limiting toxicity. An open phase I/II clinical study involving PRI-724 dose escalation in patients with advanced malignant myeloid blood diseases is still ongoing[51].
For enoticumab, the common severe adverse effects (AEs) are fatigue, headache, hypertension, and nausea[135]. However, six treatment-related serious adverse events were reported in four patients: Brain natriuretic peptide increase, troponin I increase, right ventricular dysfunction and pulmonary hypertension, and left ventricular dysfunction and pulmonary hypertension[135]. One patient had a dose-limiting toxicity of grade 3 vomiting and diarrhea[136]. Fragility fractures were reported at an ipafricept dose of 20 mg/kg. The hypophosphatemia and decrease in weight are grade III AEs associated with ipafricept treatment[137]. A dose-limiting toxicity of grade III mucosal inflammation was observed at the 30 mg dose level of LY-900009[138]. When patients were treated with crenigacestat, the most frequent related AEs included diarrhea, vomiting, nausea, decreased appetite, fatigue, asthenia, and hypophosphataemia[139]. In the phase I clinical trial, dose escalation of LY3056480 confirmed that trans-tympanic injection at the highest dose of 250 μg is safe and well tolerated and safety issues were not observed[140]. Patients with glioma were treated with RO-4929097 in combination with radiotherapy and temozolomide, and the results were positive, safe, and effective[141]. Treatment with BMS-906024 was found to be relatively well tolerated, with minimal diarrhea observed in the subjects[142].
Moreover, the AEs commonly observed in vismodegib-treated patients, including muscle spasms, ageusia/dysgeusia, alopecia, weight loss, and fatigue, lead to poor clinical outcomes because of the decreased quality of life and treatment discontinuation[143], and the more severe AEs can lead to death[144]. In some cases, treatment with vismodegib resulted in the development of squamous cell carcinoma[145], amenorrhea[146], and persistent alopecia[147]. Patient treated with vismodegib for one month can develop severe nausea, jaundice, and cholestasis with significantly elevated BUN, creatinine, and liver enzymes[148].
Sonidegib shows the same issues of drug resistance and AEs as vismodegib. However, a phase I trial demonstrated that sonidegib treatment is well tolerated and effective. The maximum tolerated dose is 800 mg administered once daily[149]. Common grade 3/4 hematological AEs are thrombocytopenia (91%), followed by neutropenia (84%) and anemia (77%). The ClinicalTrials.gov Identifier is NCT02129101, and the treatment is under examination. Patients treated with glasdegib develop some adverse events, such as dysgeusia, muscle spasms, alopecia, decreased appetite, increased blood creatinine phosphokinase, constipation, and diarrhea[147].
Treatment with patidegib results in fatigue, muscle cramps, and rash[150]. A phase I study demonstrated that BMS-833923 administered to patients with cancer was safe and well tolerated at all doses[151]. Adverse events related to napabucasin administration have been mostly mild, although some patients have experienced grade 3 gastrointestinal adverse events. More severe adverse events can be alleviated by dose reduction, discontinuation of napabucasin, or medication to reverse or manage symptoms[122]. Patients developed fatigue, hyperglycemia, nausea, rash hypertriglyceridemia, mucositis, hypophosphatemia, decreased appetite, and diarrhea after treatment with GDC-0084[152].
OTHER METHODS FOR THE TREATMENT OF CSCS
In addition to the potential pharmacological inhibitors targeting CSC-related signaling pathways, other methods of targeting CSCs are available, such as nanoparticles, mitochondria targeting, autophagy, hyperthermia, and antibodies.
NDDS targeting CSCs
A NDDS with a variety of properties is often used for targeted delivery and sustained release drugs[153]. Due to the strong phagocytic ability of tumor cells, abundant blood vessels in diseased tissues, and large gaps in vascular endothelial cells, NDDSs are more likely to enter the capillary wall of a tumor and become enriched in tumor tissues, which provides a new method for eradicating CSCs. The nanocarriers are small sized, have a large specific surface area, and present high surface activity, degradation in vivo, good biocompatibility, and no immunogenicity; moreover, the drug loading and pharmacokinetic properties can be improved by surface modifications to achieve targeted delivery and sustained release of drugs[154,155].
Liu et al[156] prepared the paclitaxel-loaded nanoparticles modified by cell-penetrating peptide R8, which specifically target CSCs, block angiogenesis, and reduce nutrient and blood supplement, thus leading to the suppressed proliferation of glioma CSCs. Han et al[157] delivered gemcitabine to BCSCs using hyaluronic acid (HA)-modified liposomes, and this modification improved the drug stability, prolonged the half-life, enhanced cell toxicity and anti-tumor metastasis, and inhibited cell clone formation.
Cui et al[158] synthesized a gelatinase-sensitive polymer nanocarrier that was loaded with miR-200c and acted on gastric cancer cells, and their results indicated that gastric cancer cells were significantly sensitized to radiotherapy. In addition, the expression of CD44 was down-regulated, the number of BGC823 cells (CD44+) was decreased, the cell invasion, metastasis, and anti-apoptosis ability were weakened, and the resistance characteristics of CSCs were decreased[158]. Moreover, other NDDSs have been used for targeted therapy of CSCs, such as nano-loaded genes, nano-loaded siRNA[159], and nano-compound both drugs[160].
Targeting mitochondrion to inhibit CSCs
Changes in the mitochondria in CSCs, including morphological changes, abnormal activation of signaling pathways, dysfunction, reactive oxygen species (ROS) generation, and mitochondrial autophagy, are key to regulating the proliferation and apoptosis of CSCs and represent one of the causes of anticancer therapy failure. CSCs exhibited significant anaerobic glycolysis characteristics, such as increased expression of glycolytic enzymes, increased production of lactic acid, and decreased or rested mitochondrial function[161]. These characteristics are similar to those of stem cells, suggesting that the mitochondrial glycolysis pathway plays a key role in regulating the proliferation and apoptosis of CSCs.
Liu et al[162] reported that CSCs could utilize the glycolytic pathway to supply energy induced by glucose and increase the expression of hexokinase 1 (HK-1), HK-2 and pyruvate dehydrogenase kinase 1 (PDK-1), which can prolong the life of CSCs. Dichloroethyl ester can inhibit PDK phosphorylation of pyruvate dehydrogenase and promote the conversion of pyruvate to Acyl-CoA, which converts mitochondrial metabolism from glycolytic to oxidative phosphorylation, thereby reducing cell proliferation, promoting apoptosis, and inhibiting tumor growth[163]. In addition to producing ATP, mitochondria are the main site for ROS production. BCSCs have been reported to have a higher ability to scavenge ROS than the corresponding tumor cells, thereby maintaining ROS at a relatively low level, although BCSCs die when the ROS levels are too high[164]. Kawano et al[165] found that knocking out the CD44 variant gene could reduce the defense ability of CSCs against ROS and enhance the killing ability of CD44 variant strongly positive cells treated with cisplatin but not CD44 variant weakly positive cells. Increasing the ROS level and destroying the protective effect of ROS on CSCs may also offer an alternative method for cancer treatment[166].
Autophagy and CSCs
Over the past several years, autophagy has emerged as a requirement for the maintenance of stemness in both normal tissue stem cells[167] and CSCs[168,169]. Autophagy plays a vital role in promoting and inhibiting two different effects in different stages of tumor and tumor development; therefore, activation and inhibition of autophagy could improve the therapeutic effects for tumors[170]. Autophagy maintains the function of different types of stem cells, such as microenvironmental homeostasis and stem cell characteristics[171].
Some autophagy-related genes promote the survival of CSCs in the autophagy process. Gong et al[172] discovered that Beclin1 and autophagy are also essential for CSC maintenance and tumorigenesis in vivo. A key role for autophagy in maintaining BCSCs has been identified according to two different shRNA screens, with Beclin-1/ATG6 identified from an shRNA screen for genes that modulate the plasticity of BCSCs[173] and ATG4A identified from a screen for genes required for mammosphere formation[174]. Damage-regulated autophagy modulator 1 (DRAM1) and P62 are abundantly expressed in adult glioblastoma, and glioma stem cells are regulated in biological metabolism, tumor migration and invasion by specific siRNA acting on DRAM1 and P62 proteins[175]. When knocking out LC3 and ATG12 genes, the CD44+/CD24-/low BCSCs are inhibited. The autophagy flux of non-adherent cells (CD44+/CD24-/low) in TNBC is higher than that of adherent cells, and the expression of CD44+/CD24-/low in TNBC is decreased after treatment with the autophagy inhibitor chloroquine[176]. Jiang et al[177] found that CSCs from malignant gliomas cause cell death due to the accumulation of autophagy-related proteins and autophagosomes. Similarly, the activation of autophagy under hypoxic conditions promotes the dedifferentiation of non-pancreatic CSCs into stem-like cells, suggesting that autophagy can affect the source transformation process of CSC[178].
Because autophagy changes the sensitivity of CSCs to conventional treatment or directly destroys cells using the toxicity produced by CSC autophagy, autophagy could be used as a treatment for CSCs. Conventional therapy presents difficulty in the specific killing of tumor cells and shows many negative effects in patients. Although autophagy therapy has milder effects on normal cells than conventional therapy, it may also have unknown effects that remain to be explored.
Hyperthermia for CSCs
As a curative treatment for cancer, hyperthermia is attracting increasing attention and recognition from doctors and patients. In hyperthermia treatment, tumor tissue is directly heated through ultrasound, microwave, radiofrequency, infrared, visible light, alternating magnetic fields, or heat-generating substances. This therapy does not include ionizing radiation and thus avoids the damage from radiation to patients and operators and pollution to the environment.
Tumor cells are more sensitive to heat, and the tumor area radiates more slowly; therefore, the temperature in the tumor area can be changed by hyperthermia, which leads to the death of tumor cells. At present, the temperature range of the hyperthermia method is 42-45 °C and the temperature of the thermal ablation is above 65 °C[179,180]. Hyperthermia can enhance the expression of apoptotic genes, such as P53 and FAS, thereby blocking the cell cycle, inhibiting tumor cell proliferation, and leading to tumor cell apoptosis[181]. The expression of heat shock protein is increased during hyperthermia, which stimulates the body's anti-tumor immune response[182]. The endothelial cells of the tumor microvessels proliferate and are more sensitive under heat; thus, the microvessels in the tumor tissue are more susceptible to heat damage than those of normal tissues.
Hyperthermia enhances the responses of the immune system to cancer, such as by up-regulating the homing of immune cells and the function of adhesion molecules on both immune cells and endothelial cells, activating cytotoxic T cells, dendritic cells, and natural killer cell, and inhibiting immune suppression[183]. As an adjunct to cancer therapy, hyperthermia plays an increasingly important role in the treatment of tumors[184]. By using hyperthermia in combination with chemotherapy[185], radiotherapy[186], immunotherapy[187], or surgery[188], the dose of these therapies may be reduced to ease their side effects without reducing their therapeutic effects.
The AEs of acute or chronic periods of regional hyperthermia do not develop often and are usually minor, and they include skin burns and pain; however, these events usually heal spontaneously[189]. Overall, hyperthermia is considered an alternative therapeutic method when it is used appropriately.
Immunotherapy targeting CSCs
The CD44 receptor is a specific tumor antigen located on the surface of CSCs, and hyaluronan (HA) is the ligand for all CD44 receptors or subtypes. CD44 receptor-specific antigens play an important role in CSCs. Utilizing the monoclonal antibody MEN-85 that binds to the C-terminus of the hyaluronate-binding domain of CD44 causes a conformational rearrangement that results in the CD44 receptor detaching from the surface of CSCs, thereby blocking the signaling pathway of HA-CD44[190]. CD44 is a known marker of CSCs, and the CD44 gene splice isoforms are CD44s and CD44v. Li et al[191] reported that the CSC marker CD44s is up-regulated in human pancreatic tumors and associated with patient survival time. CD44s is necessary for the initiation, growth, metastasis, and postradiation recurrence of xenograft tumors in mice. Antibodies targeting the CD44 receptor can eliminate bulk tumor cells and CSCs from the tumors. CD44s is the predominant isoform expressed in BCSCs. Elimination of the CD44s isoform impairs CSC traits. However, manipulating the splicing regulator epithelial splicing regulatory protein 1 to shift alternative splicing from CD44v to CD44s leads to the induction of CSC properties[192]. These results suggest that alternative splicing provides functional gene versatility that is essential for different cancer cell states and thus cancer phenotypes. CSCs can also express a variety of specific antigens; for example, the CD44 receptor can also be expressed in lung cancer cells as well as BCSCs[193]. BCSCs not only express CD44 receptor but also express aldehyde dehydrogenase (ALDH)[194].
The Notch signaling pathway is abnormally active in the tumor microenvironment (TME). Inhibitor targeting that blocks signal transmission can reduce the number of CSCs and inhibit the development of tumors[195]. Blocking Wnt signaling can reduce the expression of CD44 and ALDH on the CSC surface and inhibit tumor self-renewal and metastasis[196]. ALDH1 is widely distributed in humans and highly expressed in stem cells of normal tissues as a marker of normal stem cells. Notably, the activity of ALDH1 is increased in MM and myeloid leukemia[197]. Moreover, ALDH1 is also expressed in most cancer tissues at different levels, such as breast cancer, lung cancer, and colon cancer[198].
In 2019, Chen et al[199] demonstrated that the depletion of ubiquitin-specific protease 9X (USP9X) markedly downregulated ALDH1A3, thereby resulting in the loss of the self-renewal and tumorigenic capacity of mesenchymal (MES) glioblastoma stem cells (GSCs). Furthermore, the USP9X inhibitor WP1130 induced ALDH1A3 degradation and showed marked therapeutic efficacy in MES GSC-derived orthotopic xenograft models.
Humanized anti-CD47 antibody Hu5F9-G4 can safely and effectively treat five invasive childhood brain tumors in mice[200]. Hu5F9-G4 demonstrated therapeutic efficacy in vitro and in vivo in patient-derived orthotopic xenograft models; notably, Hu5F9-G4 showed minimal activity against normal human neural cells. Advani et al[201] confirmed that the macrophage checkpoint inhibitor Hu5F9-G4 synergized with rituximab can safely and effectively eliminate aggressive and indolent lymphoma, and no clinically significant safety events were observed in this study. Liu and coworkers indicated that CpG oligodeoxynucleotide, a toll-like receptor 9 agonist, combined with CD47 inhibitors can rapidly induce tumor shrinkage and prolong survival in mice[202]. It is worth noting that a number of new drugs targeting CD47 are undergoing clinical trials, such as AO-176, CC-90002, NI-1701, IBI118, and TI-063. Therefore, macrophage immunological checkpoint blocking therapy (e.g., CD47 antibody) is expected to provide a new cancer immunotherapy strategy.
Targeting the CSC microenvironment
Although tumors initiate from oncogenic changes in a cancer cell, subsequent tumor progression and therapeutic responses depend on interactions between the cancer cells and the TME[203,204]. The cells and molecules in the TME are in a dynamic process that leads to a large number of immunosuppressive cells (e.g., myeloid-derived suppressor cells, regulatory cells, and tumor-associated macrophage), and a large number of inflammatory related factors (e.g., chemokines, TGF-β, and interleukins) assemble together in the TME. Then, they jointly promote tumor immune escape, tumor growth, and metastasis[205].
Among the identified CXC chemokine receptors (CXCRs), CXCR4 is most closely related to tumor cells, and it was first discovered as a co-receptor of HIV. Until now, CXCR4 has been found in a variety of cancers, including breast cancer, prostate cancer, lung cancer, colon cancer, and MM[206]. The overexpression of CXCR4 is associated with a poor prognosis in GBM. Wu et al[207] indicated that anti-CXCR4 and anti-programmed cell death protein 1 (PD-1) combination immunotherapy can modulate tumor-infiltrating populations of the glioma microenvironment. Some CXCR4 antagonists (e.g., BL-8040 and X4P-001-IO) are used in combination with PD-(L)1 drugs, such as Keytruda, Tecentri, and Opdivo. CXCL12/CXCR4-mediated desmoplasia in metastatic breast cancers (mBCs) promotes immunosuppression; therefore, it is a potential target for overcoming therapeutic resistance to immune checkpoint blockade in mBC patients[208,209].
Neutrophils are first-responders to sites of infection and tissue damage[210]. Powell et al[211] demonstrated that the chemokine receptor CXCR1 promotes neutrophil recruitment, CSC proliferation, and neoplastic mass formation. Therefore, neutrophil recruitment signaling pathways, such as CXCL8-CXCR1, have the potential for use as targets for anti-cancer therapies. Danhier et al[212] utilized the acidic TME as a target of nano-theranostics to enable cancer-specific imaging and therapy, and this approach showed advantages over conventional tumor targeting strategies. However, the acidic TME not only plays an essential role in the initiation, progression, and metastasis of tumors but also participates in the induction of treatment resistance. The TME is related with oncogenesis, host genetics, the microbiome, and immune cell activity[213]. More novel therapies targeting CSCs and the TME require further exploration, comprehensive analysis, and consolidation of both clinical and experimental data.
CONCLUSION
In recent decades, numerous new agents and methods for treating patients with cancer, such as CSC-related singling pathway inhibitors, monoclonal antibodies, hyperthermia, or NDDSs, have become available or are under clinical trial. The CSC hypothesis has provided an explanation for the failure of traditional cancer treatments. Targeting CSCs via the Wnt, Hedgehog, and Notch signaling pathways has promise in preventing disease recurrence. However, the development of such agents is hindered by many challenges. It is now clear that all signaling pathways do not function in isolation but rather as a coordinated network. The output from the entire signaling network regulates the phenotype of CSCs. Therefore, the development of CSC inhibitors will require an understanding of the stem cell signaling network. For example, combination therapy could pave the way for new and innovative strategies for the pharmacological treatment of CSCs to increase the efficacy and decrease the toxicity of individual agents.
However, NDDSs can effectively utilize the microenvironmental properties of CSCs and efficiently deliver anti-tumor drugs to tumors, which can improve the chemotherapy effect, reduce the toxicity and AEs, and improve patient compliance. Therefore, NDDSs may provide a novel alternative therapeutic strategy targeting CSCs. In addition, immunotherapy that targets the surface markers expressed by CSCs (e.g., CD47, ALDH1, and CD44) demonstrates a remarkably inhibiting effect on CSCs. Notably, a number of new drugs targeting CD47 are undergoing clinical trials, such as AO-176, CC-90002, NI-1701, IBI118, and TI-063. Hence, macrophage immunological checkpoint blocking therapy is expected to provide a new method for cancer immunotherapy.
As for the optimal time for the use of a CSC-specific therapy, there is no related reference to clarify this key issue. However, once the related cancer is identified, the therapy targeting CSCs can be concurrently proceeded with neoadjuvant chemo/radiotherapy. Generally, conventional chemotherapy can only inhibit tumor growth and lead to drug resistance, but cannot kill CSCs. However, the key to cancer treatment is how to deracinate CSCs. Therapy targeting CSCs can effectively remove CSCs and avoid drug resistance. Moreover, synergy therapy with chemo/ radiotherapy may reduce the dosage and AEs while not changing the physiological effect. However, when and how to use targeting CSCs to treat related cancers requires clinical trials to determine.
Research on CSC-related signaling pathways provides new avenues for the development of related drugs. With further developments in science and technology, CSCs will eventually be fully understood and cancer development and progression and the underlying signal regulatory mechanisms will be thoroughly clarified, thereby laying the foundation for the treatment of malignant tumors. In addition, clarifying the considerable crosstalk between cell signaling pathways is critical for designing effective therapeutic approaches. The focus of future trials should be on the use of combination therapies that affect multiple pathways in the tumor. However, this field remains in its infancy, and considerable research will be required to produce mature products. However, for the first time in medical history, we have the tools to understand the fate of tumor cells and target them therapeutically.
Footnotes
Conflict-of-interest statement: The authors declare no potential conflicts of interest.
Peer-review started: February 22, 2019
First decision: June 4, 2019
Article in press: June 27, 2019
Specialty type: Cell and tissue engineering
Country of origin: China
Peer-review report classification
Grade A (Excellent): A
Grade B (Very good): B
Grade C (Good): C, C
Grade D (Fair): 0
Grade E (Poor): 0
P-Reviewer: Andrisani OM, Labusca L, Luo WR, Valenti MT S-Editor: Yan JP L-Editor: Wang TQ E-Editor: Ma YJ
Contributor Information
Fang-Yu Du, Key Laboratory of Structure-Based Drug Design and Discovery of Ministry of Education, Shenyang Pharmaceutical University, Shenyang 110016, Liaoning Province, China.
Qi-Fan Zhou, Key Laboratory of Structure-Based Drug Design and Discovery of Ministry of Education, Shenyang Pharmaceutical University, Shenyang 110016, Liaoning Province, China.
Wen-Jiao Sun, Key Laboratory of Structure-Based Drug Design and Discovery of Ministry of Education, Shenyang Pharmaceutical University, Shenyang 110016, Liaoning Province, China.
Guo-Liang Chen, Key Laboratory of Structure-Based Drug Design and Discovery of Ministry of Education, Shenyang Pharmaceutical University, Shenyang 110016, Liaoning Province, China. nc.ude.uhpys@gnailougnehc.
References
Articles from World Journal of Stem Cells are provided here courtesy of Baishideng Publishing Group Inc
Citations & impact
Impact metrics
Article citations
Cancer Stem Cells in Oral Squamous Cell Carcinoma: A Narrative Review on Experimental Characteristics and Methodological Challenges.
Biomedicines, 12(9):2111, 16 Sep 2024
Cited by: 0 articles | PMID: 39335624 | PMCID: PMC11429394
Review Free full text in Europe PMC
Unveiling the Dynamic Interplay between Cancer Stem Cells and the Tumor Microenvironment in Melanoma: Implications for Novel Therapeutic Strategies.
Cancers (Basel), 16(16):2861, 16 Aug 2024
Cited by: 0 articles | PMID: 39199632 | PMCID: PMC11352669
Review Free full text in Europe PMC
FXN targeting induces cell death in ovarian cancer stem-like cells through PRDX3-Mediated oxidative stress.
iScience, 27(8):110506, 14 Jul 2024
Cited by: 0 articles | PMID: 39184439 | PMCID: PMC11342215
Cobalt(III)-Macrocyclic Scaffolds with Anti-Cancer Stem Cell Activity.
Molecules, 29(12):2743, 08 Jun 2024
Cited by: 0 articles | PMID: 38930809
Historical Perspective and Current Trends in Anticancer Drug Development.
Cancers (Basel), 16(10):1878, 15 May 2024
Cited by: 1 article | PMID: 38791957 | PMCID: PMC11120596
Review Free full text in Europe PMC
Go to all (43) article citations
Data
Data behind the article
This data has been text mined from the article, or deposited into data resources.
BioStudies: supplemental material and supporting data
Clinical Trials
- (1 citation) ClinicalTrials.gov - NCT02129101
Similar Articles
To arrive at the top five similar articles we use a word-weighted algorithm to compare words from the Title and Abstract of each citation.
Cancer Stem Cells in Oral Squamous Cell Carcinoma: A Narrative Review on Experimental Characteristics and Methodological Challenges.
Biomedicines, 12(9):2111, 16 Sep 2024
Cited by: 0 articles | PMID: 39335624 | PMCID: PMC11429394
Review Free full text in Europe PMC
Targeting Notch, Hedgehog, and Wnt pathways in cancer stem cells: clinical update.
Nat Rev Clin Oncol, 12(8):445-464, 07 Apr 2015
Cited by: 700 articles | PMID: 25850553 | PMCID: PMC4520755
Review Free full text in Europe PMC
Self-Renewal Signalling Pathway Inhibitors: Perspectives on Therapeutic Approaches for Cancer Stem Cells.
Onco Targets Ther, 13:525-540, 16 Jan 2020
Cited by: 11 articles | PMID: 32021295 | PMCID: PMC6970631
Review Free full text in Europe PMC
Recent advances in drug delivery systems for targeting cancer stem cells.
Acta Pharm Sin B, 11(1):55-70, 02 Oct 2020
Cited by: 93 articles | PMID: 33532180 | PMCID: PMC7838023
Review Free full text in Europe PMC