Abstract
Free full text

Molecular Diagnosis of Steroid 21-Hydroxylase Deficiency: A Practical Approach
Abstract
Adrenal insufficiency in paediatric patients is mostly due to congenital adrenal hyperplasia (CAH), a severe monogenic disease caused by steroid 21-hydroxylase deficiency (21-OHD, encoded by the CYP21A2 gene) in 95% of cases. CYP21A2 genotyping requires careful analyses that guaranty gene-specific PCR, accurate definition of pseudogene-gene chimeras, gene duplications and allele dropout avoidance. A small panel of well-established disease-causing alterations enables a high diagnostic yield in confirming/discarding the disorder not only in symptomatic patients but also in those asymptomatic with borderline/positive results of 17-hydroxyprogesterone. Unfortunately, the complexity of this locus makes it today reluctant to high throughput techniques of massive sequencing. The strong relationship existing between the molecular alterations and the degree of enzymatic deficiency has allowed genetic studies to demonstrate its usefulness in predicting/classifying the clinical form of the disease. Other aspects of interest regarding molecular studies include its independence of physiological variations and analytical interferences, its usefulness in the diagnosis of simple virilizing forms in males and its inherent contribution to the genetic counseling, an aspect of great importance taking into account the high carrier frequency of CAH in the general population. Genetic testing of CYP21A2 constitutes an irreplaceable tool to detect severe alleles not just in family members of classical forms but also in mild late-onset forms of the disease and couples. It is also helpful in areas such as assisted reproduction and preimplantation diagnosis. Molecular diagnosis of 21-OHD under expert knowledge definitely contributes to a better management of the disease in every step of the clinical course.
1 Introduction
Congenital adrenal hyperplasia (CAH) due to 21-hydroxylase deficiency (21-OHD)(OMIM #201910) is an inherited autosomal recessive disorder responsible of 95% of CAH cases (1, 2). It has its origin in a defect of steroid 21-hydroxylase (21-OH), an enzyme encoded by the CYP21A2 gene. Alterations in CYP21A2 cause an impairment of the enzymatic activity and leads to the accumulation of 17-hydroxyprogesterone (17-OHP), which is diverted towards formation of androgens (1, 3). As an actionable, non-infrequent and life-threatening disease, CAH is included in the neonatal screening of several countries (4).
Although 17-OHP is the metabolic marker of the deficiency, CYP21A2 genotyping contributes as a diagnostic tool due to its independence on physiology and its strong relationship with clinical severity (4). The high carrier frequency (5, 6) and the recurrent impaired fertility in patients (7–9) further evidence the important contribution that genotyping does. Molecular studies provide valuable information in prevention and contribute to a better management of the disease (10, 11).
CAH is related to a wide range of clinical behaviors, with phenotypes varying from severe classical forms (CLF) to moderate late-onset non-classical forms (NCF). As a highly penetrant monogenic disease, 21-OHD shows a strong, although not complete, genotype-phenotype relationship in which the clinical features correspond to the less severely impaired allele (1, 12, 13). Variants causing null or minimal enzymatic activity in both alleles result in salt-wasting forms (SW), whereas their compound heterozygosity with variants causing residual activity result in simply virilizing forms (SV). NCF are due to mild alterations in homozygosity or a compound heterozygosity of either two mild alterations or a severe and a mild one (1, 3, 12, 14–16) ( Supplementary Tables 1 , 2 ). Some lacks of genotype-phenotype relationship may result from extraadrenal 21-hydroxylation mediated by liver P450 cytochromes (17).
2 Gene Locus Structure and Nature of CYP21A2 Alterations
CYP21A2 is arranged in tandem with its inactive pseudogene (CYP21A2P) within a genetic unit designated as RCCX module, where also the genes TNXA/B, C4A/B and RP are harbored (18). Most chromosomes have two RCCX modules, although mono-, tri- or even quadrimodular arrangements have been described (19, 20). The high homology existing between gene and pseudogene (98% in coding and 96% in non-coding regions) together with that existing between RCCX modules favor unequal cross-overs during meiosis making that most pathological alleles in CAH arise from mechanisms of asymmetric recombination (25-30%) and gene conversion events (70%). Consequently, CYP21A2 genotyping requires careful analyses that guaranty gene-specific PCR with allele dropout avoidance, and accurate definition of pseudogene-gene chimeras and gene duplications. Of course, an expert interpretation of the results is needed.
2.1 Alterations Due to Intrinsic Locus-Derived Mechanisms
2.1.1 Point Pathological Variants: Microconversions
Around 70% of the disease causing alterations in CAH are pseudogene-deleterious-variants that have been transferred to the gene by small gene conversion events. As a result, a limited group of pathogenic variants with well-known phenotypic effects is present in all populations (3) ( Figure 1 ).
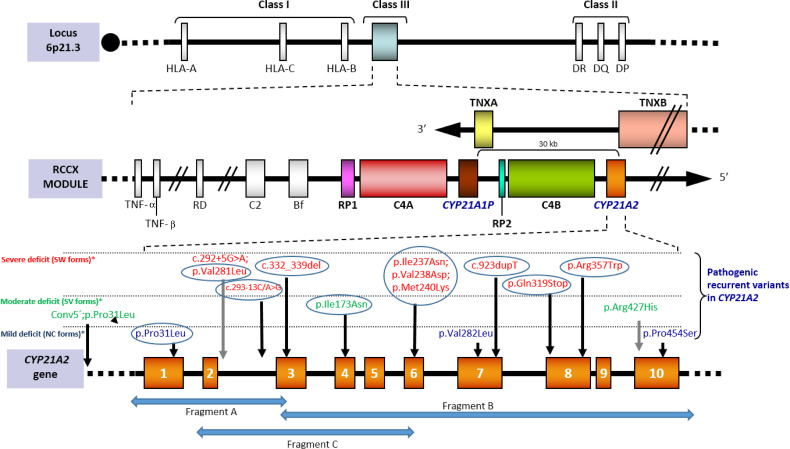
[Adapted from Santomé et al., (21)]. Scheme of the RCCX module located on the short arm of chromosome 6 within the HLA class III region. Tandem duplication affects CYP21 and C4 genes. In humans only CYP21A2 gives rise to the functional protein, whereas CYP21P is a homologous pseudogene that includes several inactivating point variants that can be transferred to the active gene by small gene conversion events. Both C4A and C4B are functional. Tenascin, also duplicated, is encoded in the complementary chain. The bottom of the image shows the recurrent variants grouped according to how they affect the enzimatic functionality: severely (red), moderately severe (green) or mildly (blue). Recurrent variants in all populations are circled. The complete nomenclature of each variant including the cDNA position (NM_000500.9) would be: c.92C>T [p.Pro31Leu], c.292+5C>A, c.293-13C>G, c.332-339del, c.518T>A [p.Ile173Asn], c.(710T>A; 713T>A; 719T>A) p.[Ile237Asn; Val238Glu; Met240Lys], c.844G>T [p.Val282Leu], c.923dupT, c.955C>T [p.Gln319*], c.1069C>T [p.Arg357Trp], c.1280G>A [p.Arg427His] and c.1360C>T [p.Pro454Ser]). The arrows “Fragment A”, “Fragment B” and “Fragment C” represents specific amplicons for CYP21A2 amplification.
2.1.2 Gene Chimeras
Asymmetric recombination between CYP21A2 and CYP21A2P is responsible of about 25-30% of all deficient alleles (3, 22, 23). This mechanism results in the appearance of pseudogene-gene chimeras (traditionally named “gene deletions”) usually extending from somewhere between exons 3 and 8 of CYP21A2P to the corresponding point in CYP21A2, yielding a non-functional gene in which the 5’-end corresponds to CYP21A2P and the 3’-end corresponds to CYP21A2. It is important to mention a subset of patients in which the deletion is extended into the TNXB gene resulting in a contiguous gene syndrome named CAH-X consisting in CAH and Ehlers-Danlos Syndrome (24) that should also be investigated (25).
Chimeras are usually categorized into classic and attenuated depending on the location of the junction site, having been reported nine different types (26). Classic types contain the c.293-13C>G region and produce non-functional alleles whereas attenuated ones have the junction upstream of that region and associate a less severe phenotype (26) (see Avoidable Pitfalls Upon Complementary Characterization of Alleles).
2.2 Alterations Due to Conventional Mechanisms
Alterations other than those derived from recombinant events are less frequent and usually involve functional residues, generate frameshifts or stop codons (16, 21, 30, 31). The number of splicing pathological variants described so far is small (30), with a new candidate recently reported (32). Alterations in regulatory regions are controversial and difficult to demonstrate but tend to be mild changes. To date, more than 200 different pathogenic variants in CYP21A2 have been described (16, 30, 31).
3 Disease Frequency and Origin of CYP21A2 Alterations
CAH constitutes a non-infrequent disease, even in its severe neonatal forms. This seems to be the result of the prolific molecular mechanisms previously mentioned, although a founder effect has also been proposed (33–37). Regarding this latter, some studies have documented a lower mortality in CYP21A2 carriers mainly due to a decreased number of infections in these individuals (38).
Considering that de novo variants in CYP21A2 are infrequent (27, 29, 30) and that alterations are maintained through generations once originated (33, 39), it is not uncommon that the presence of new/rare pathological variants be the result of the dissemination of single original alleles.
4 Genetic Diagnosis of CAH
Since CAH due to 21-OHD accounts for 95% of all CAH cases, CYP21A2 should be the first gene to investigate in males and virilized girls with adrenal insufficiency. The remaining genes causing CAH (1, 2, 12, 40) as well as other involved in adrenal insufficiency (41) should be investigated using high-throughput approaches (massive sequencing gene panels) (40–42). On regard CYP11B1, it is important to highlight its high homology with CYP11B2 and the consequent existence of hybrid genes (43–45).
5 CYP21A2 Genotyping
Traditional approaches for CYP21A2 genotyping usually include methods such as capillary sequencing, allele-specific oligonucleotide hybridization, SNaPshot minisequencing and MLPA, which are labor intensive and have limited multiplexing capability, but which keep being used given their proven clinical usefulness and the difficulty of optimizing the current massive sequencing technologies to this complex locus. Conventional massive platforms are poorly equipped to characterize gene-pseudogene pairs and have the limitation of being based on PCR-amplifications and uniquely aligning short reads (that may not include CYP21A2 gene-specific regions). As a consequence, they are not still the first-choice option for CYP21A2 genotyping although some promising results have been obtained (31, 46–49). Third-generation platforms based on direct sequencing of long DNA strands without previous amplification seem promising tools (50–52).
5.1 Detection of Point Pathological Variants: Gene-Specific PCR
Current strategies for the specific amplification of CYP21A2 rely on regions that are known to be different from those of the pseudogene, either as targets for restriction sites prior to PCR or PCR-specific primers. Since one of these latter regions is located on exon 3 (where the variant c.332-339del is located in CYP21A2P), an extensively used scheme for the specific amplification of CYP21A2 is obtaining two fragments (one from 5’UTR to exon 3 and another from exon 3 to 3'UTR). However, alleles carrying the variant c.332-339del as a single microconversion would not be detected in this way (neither chimeras, conversions or gene duplications including it), so a third fragment in which the 3’-end is located on the specific site on exon 6 (where the cluster of three variants is harbored in CYP21A2P) can be incorporated (34, 39, 53–56) ( Figure 1 ). This last overlapping fragment allows the PCR-detection of pseudogene-gene chimeras with the breaking point before exon 6.
Recurrent variants ( Figure 1 ) may be investigated in a first screening performed by allele-specific oligonucleotide hybridization or SNaPshot minisequencing, although they and other point variants are detected with Sanger sequencing on these gene-specific fragments. Whole gene sequencing must guarantee an accurate interpretation based on well-documented alterations due to the lack of complete knowledge regarding the impact of every variant in this small but polymorphic gene. In vitro analyses (57–59) and/or models investigation (60–62) should support the involvement of new variants, but only clinical validation in different populations and genotypes will confirm their causality.
Segregation of alterations in parental samples is an important issue since gene chimeras and large or double micro-conversions include several alterations within the same allele (carrier status), a very different situation from that in which alterations are located in different alleles (affected patient). Approximately 5-7% of affected alleles carrying two or more point alterations (63). Patients carrying gene chimeras/conversions that include the specific regions used in PCR protocols result in hemizygosity and directly stablish the segregation, although not the carrier status of progenitors (see Family Studies).
5.2 Analysis of Gene Chimeras: MLPA
MLPA allows to identify gene deletions/conversions avoiding the inconvenients linked to Southern blotting. Nevertheless, since it also has unavoidable limitations [reduction of signal when alterations/polymorphisms exist in a probe-binding region (64), inability to detect the cis/trans disposition of the alterations, or lack of probes addressed to some frequent variants], must be always complemented with other analyses. Unfortunately, a comprehensive revision defining every MLPA pattern and its deduced genotype is still lacking, although some studies are contributing to a better definition of this issue (65). MLPA should also be applied in the complementary characterization of some complex alleles (see Avoidable Pitfalls Upon Complementary Characterization of Alleles).
5.3 Avoidable Pitfalls Upon Complementary Characterization of Alleles
Some of the seemingly lacks of genotype-phenotype relationship in several frequent point variants are not further sustained when alleles are better characterized. An efficient multistep approach (64) allows a comprehensive mutation analysis. Apparently mild alleles which are not really such are those carrying the variant c.92C>T [p.Pro31Leu] with a cis pseudogene-conversion in 5’ (26, 56, 66, 67), and those carrying the variant c.844G>T [p.Val282Leu] in cis with the intronic change c.292+5G>A, an alteration observed in SW from Mediterranean populations (15, 68) ( Figure 1 and Supplementary Table 1 ).
Examples of “severe” alleles that are not really such are those with the variant c.955C>T [p.Gln319*] and two copies of the gene, present in several populations (6, 30, 69) ( Supplementary Table 1 ). Fortunately, since the whole gene is involved in these alleles, MLPA allows its detection in spite of the salsa MLPA Probemix P050-C1 CAH (MRC Holland) no longer includes exon 8 probes. It is important to mention that some of these alleles carry additional alterations [e.g. c.518T>A (p.Ile173Asn) or the combination of c.293-13 C>G and c.332_339del (6, 70)] and are severe. Some gene conversions involving exons 4 to 8 are not such deficient-alleles. Pseudogenes including the gene-specific region in exon 3, although infrequent (71), result in an identical pattern upon PCR amplification, so these conversions should be investigated with a complementary MLPA analysis.
Discrimination of homo/hemizygosity of mild variants is crucial in NCF (72) as hemizygosity requires genetic counseling. Also essential is to guarantee the efficient amplification of both alleles in order to avoid incorrect interpretations such as false homozygotes due to allele dropout of the normal allele (73). A complementary indirect analysis also provides useful information preventing serious mistakes in prenatal samples (see Usefulness of an Indirect Analysis).
6 Usefulness of an Indirect Analysis
Indirect analyses performed by either microsatellite typing or SNPs (6, 74–76) are a useful tool (see Contribution of CYP21A2 Genotyping) since informative polymorphic markers on both sides of the gene in each family configure distinct haplotypes in normal and affected chromosomes. They are helpful with prenatal samples, in preimplantation studies and in allele segregation, being able to reveal/discard consanguinity in patients carrying rare variants in homozygosity [useful as a complement of a basic/first study in patients with borderline/false positive results in the neonatal screening (see Neonatal Screening)]. Also in epidemiology, since the same haplotype for a new variant in unrelated patients suggests the variant dissemination and the potential interest of its inclusion in the basic screening of that population. Some informative microsatellite loci flanking the CYP21A2 gene are D6S2792-D6S273 and D6S1014- D6S439 together with two intronic ones in genes TNF and TAP1 (34, 63, 74, 77, 78).
7 Contribution of CYP21A2 Genotyping
7.1 Neonatal Clinical Suspicion
Although clinical manifestations such as adrenal insufficiency or virilization in girls perform the suspicion in the neonatal period, there are unspecific signs (e.g. hypoglycemia, clitoromegaly or genital hyperpigmentation) frequent in combination with 17-OHP elevations (4, 79). Genotyping of CYP21A2 allows to confirm/discard the disease in both scenarios (80) especially when analytical interferences in the direct immunoassay exist (81, 82). Comprehensive CYP21A2 genotyping should be guaranteed paying special attention to variants with a significance still poorly established. Failure to detect well-stablished pathogenic variants in CYP21A2 must prompt further studies.
7.2 Neonatal Screening
Clinical guidelines recommend a second-tier analysis by liquid chromatography–tandem mass spectrometry to improve the positive predictive value of CAH screening (4). Neonates with borderline/high levels of 17-OHP in these programs can take benefit from CYP21A2 analyses (80, 83–86). Not only CLF, but also neonatal cryptic forms (NCF and SV in males) are detected at this stage, being molecular studies able to correctly classify them (11, 80, 86, 87) by a firstly analysis focused on the identification of recurrent variants (in order to eliminate uncertainty) followed by Sanger sequencing when just one deficient-allele or microsatellite-homozygosity is detected (80).
7.3 Non-Classical Forms
The high recurrence of c.844G>T [p.Val282Leu] in some populations (88, 89) helps to “unmask” severe alleles through the clinical expressiveness of NCF [70% carrying severe alleles (4); 41% in paediatric patients, Supplementary Table 1 ]. CYP21A2 should be always considered in NCF to allow a proper genetic counseling.
Levels of 17-OHP, either basal or post-ACTH, constitute the most sensitive parameter to define a NCF since CYP21A2 mild alterations are not fully characterized. A proper threshold for 17-OHP values is difficult to define since some carriers are prone to present a hyperandrogenism similar to that shown in NCF (56, 85, 90–92). Genotyped carriers inside fully characterized segregated families are useful to achieve this goal (56, 91). Compound heterozygosity with severe alleles in NCF may be suspected based on 17-OHP levels ( Figure 2A and Supplementary Table 3 ) (56, 63) conversely to what happen with carriers of severe vs. mild variants.
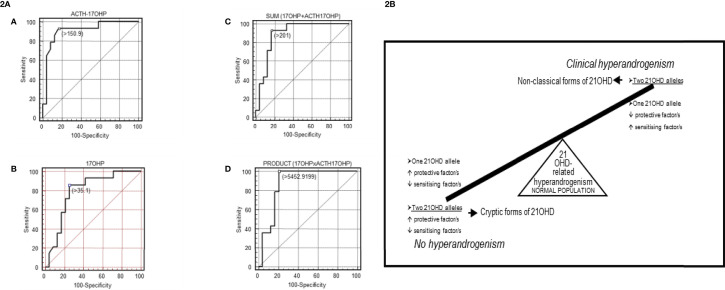
(2A) [Taken from Ezquieta et al., (56)]: Receiver operating characteristic (ROC) curves analyses in fully genotyped children affected with NCF of 21-OHD (mild/mild vs severe/mild genotype) for: (A) adrenocorticotropic hormone (ACTH)-stimulated 17-OHP, (B) basal 17-OHP, or (C, D) the combination of both parameters [(C): sum; (D): product]. Areas under the curves (SE): ACTH-stimulated 17-OHP, 0.908 (0.057); basal 17-OHP, 0.790 (0.081); sum 0.866 (0.068); product 0.884 (0.064). Cut-off values, nmol/L (A–C) and nmol2/L2 (D). The cut-offs for maximum predictive values are represented by small, empty squares in the Figures. (2B) [From Ezquieta et al., (91)] Diagram of a hypothetical interaction between protective and sensitizing factors modulating the clinical expressivity of 21-OHD-related hyperandrogenism. CAPN10-UCSNP44C and TNFR2-R196 are proposed in this study to be sensitizing and protective factors, respectively.
Monogenic and polygenic models in paediatric hyperandrogenism due to 21-OHD have been detected (91)( Figure 2B ), being carriers (monoallelic) with hyperandrogenism the counterpart of cryptic forms (biallelic alterations) without clinical expression. Considering the important contribution of the “back door” pathway to circulating levels of the potent androgen 11-ketotestosterone in CAH (93, 94), investigation of gene variants coding for the enzymes involved seems interesting.
7.4 Carrier Detection
The biochemical marker 21-deoxicortisol detects carriers (10, 40, 95), although only molecular analyses are able to discriminate carriers of severe alleles. Individuals with hyperandrogenism and moderately elevated post-ACTH 17-OHP levels (not reaching the NCF threshold) may take benefit from CYP21A2 analyses since alterations are more frequent in these patients (91) ( Supplementary Table 2 ).
7.5 Genetic Counseling
The high carrier frequency of severe variants in general population (about 1:60) (15, 96, 97) ( Supplementary Table 1 , false severe alleles) makes reasonable to refine the risk of having an affected child by genotyping CYP21A2 in couples where one member is affected/carrier. Individuals with CLF present a risk of 1:120 of having a newborn affected with a CLF. The theoretical risk is lower in NCF [1:250 (4)] although some studies have documented to be higher (1.5-2.5%) (98).
7.6 Family Studies
Family studies are necessary to ascertain parental genotype and segregation of the pathological alleles among the offspring. They are initially addressed to detect/discard alterations documented in the index case, but the high carrier frequency justifies the subsequent screening of frequent pathological variants to discard its coexistence in the family. Progenitors must not be considered obligate carriers since de novo variants are detected in 1-2% of deficient alleles (27, 29, 30).
7.7 Assisted Reproductive Techniques and Genetic Counseling
CAH due to 21-OHD should be considered in reproductive assistance and genetic counseling (7, 99, 100) due to the associated infertility (7–9, 40) and the high frequency of carriers in general population. The strong genotype-phenotype relationship (13, 101) facilitates counseling in couples even in absence of an index case, but it should not be forgotten that expressivity vary particularly in moderately-severe forms (13).
7.8 Prenatal Diagnosis
Prenatal studies are normally performed inside CLF-families. It is still accomplished on samples from corionic villus through direct analysis addressed to investigate those alterations detected in the index case. An additional indirect analysis (6, 15, 74) provides the possibility of detecting maternal contamination and avoids eventual allele dropout artefacts.
Prenatal treatment prevents virilization in girls affected with CAH but is still considered experimental (4). Prenatal diagnosis establishes treatment withdraw in non-affected foetus (carriers and non-carriers). Protocols must include screening for Y-chromosomal DNA in maternal blood (4) to minimize (40) treatment in males. Since prenatal treatment is only effective if established at 6th-7th weeks (4, 102), it is unfeasible totally avoid treatment in males since cfDNA analyses must be performed in samples with a foetal fraction about 3.5-4% (9th-10th week).
CYP21A2 genotyping from cfDNA in maternal blood is a promising approach not suitable in clinical settings yet (4, 102). For its application, massive sequencing based on an indirect analyses conducted by SNP-haplotypes previously defined in parents and index case is necessary due to the coexistence of foetal and maternal DNA in the same sample (76).
7.9 Preimplantation Genetic Diagnosis
This particular approach enables to study the embryo before the transference to the uterus. These tests are mentioned in the last Clinical Practice Guidelines from the Endocrine Society although subjected to their own risk and ethical controversies (4).
Microsatellite typing is the most appropriate approach, since the paucity of sample hampers a direct gene analysis and haplotypes detected in the directly genotyped index case provide the information.
7.10 Other Prenatal Scenarios
Suspicion of CAH due to anomalies detected by fetal ultrasound or genetic counseling for a couple at risk (not previously genotyped) with an ongoing pregnancy are prenatal situations in which CYP21A2 genotyping are requested. When the index case is unknown, the only suitable approach is the direct analysis. Only well-documented pathogenic alterations should be considered.
8 Conclusions
CYP21A2 genotyping favorably contributes to confirm/discard CLF after neonatal or prenatal suspicion. The high frequency of carriers in general population and the infertility associated to the disease turn molecular diagnosis into an irreplaceable tool to detect/discriminate severe alleles in family members and in genetic counseling, as well as in specific areas as assisted reproduction and preimplantational diagnosis. The high complexity of the locus makes essential the performance of CYP21A2 genotyping under supervision of expert personnel in the field. There is no doubt that molecular diagnosis of 21-OHD definitely contributes to a better management of the disease in every step of the clinical course.
Author Contributions
Conception and design: MA and BE. Manuscript writing: MA and BE. Manuscript revision: MA and BE. All authors contributed to the article and approved the submitted version.
Conflict of Interest
The authors declare that the research was conducted in the absence of any commercial or financial relationships that could be construed as a potential conflict of interest.
Publisher’s Note
All claims expressed in this article are solely those of the authors and do not necessarily represent those of their affiliated organizations, or those of the publisher, the editors and the reviewers. Any product that may be evaluated in this article, or claim that may be made by its manufacturer, is not guaranteed or endorsed by the publisher.
Supplementary Material
The Supplementary Material for this article can be found online at: https://www.frontiersin.org/articles/10.3389/fendo.2022.834549/full#supplementary-material
References
Articles from Frontiers in Endocrinology are provided here courtesy of Frontiers Media SA
Full text links
Read article at publisher's site: https://doi.org/10.3389/fendo.2022.834549
Read article for free, from open access legal sources, via Unpaywall:
https://www.frontiersin.org/articles/10.3389/fendo.2022.834549/pdf
Citations & impact
Impact metrics
Citations of article over time
Alternative metrics

Discover the attention surrounding your research
https://www.altmetric.com/details/125536549
Article citations
Evaluation of 3β-hydroxysteroid dehydrogenase activity using progesterone and androgen receptors-mediated transactivation.
Front Endocrinol (Lausanne), 15:1480722, 02 Oct 2024
Cited by: 0 articles | PMID: 39415787 | PMCID: PMC11479897
CYP21A2 Intron 2 Genetic Variants Might Be Associated with the Clinical Characteristics of Women with PCOS.
Biomedicines, 12(7):1528, 09 Jul 2024
Cited by: 0 articles | PMID: 39062101 | PMCID: PMC11274513
Challenging Molecular Diagnosis of Congenital Adrenal Hyperplasia (CAH) Due to 21-Hydroxylase Deficiency: Case Series and Novel Variants of CYP21A2 Gene.
Curr Issues Mol Biol, 46(5):4832-4844, 16 May 2024
Cited by: 1 article | PMID: 38785559 | PMCID: PMC11119849
Case Report: A combination of chimeric CYP11B2/CYP11B1 and a novel p.Val68Gly CYP11B1 variant causing 11β-Hydroxylase deficiency in a Chinese patient.
Front Endocrinol (Lausanne), 14:1216767, 13 Nov 2023
Cited by: 0 articles | PMID: 38027139 | PMCID: PMC10679387
Landscape of Adrenal Tumours in Patients with Congenital Adrenal Hyperplasia.
Biomedicines, 11(11):3081, 16 Nov 2023
Cited by: 1 article | PMID: 38002081
Review
Go to all (8) article citations
Data
Data behind the article
This data has been text mined from the article, or deposited into data resources.
BioStudies: supplemental material and supporting data
Diseases
- (1 citation) OMIM - 201910
Similar Articles
To arrive at the top five similar articles we use a word-weighted algorithm to compare words from the Title and Abstract of each citation.
A rational, non-radioactive strategy for the molecular diagnosis of congenital adrenal hyperplasia due to 21-hydroxylase deficiency.
Gene, 526(2):239-245, 06 Apr 2013
Cited by: 8 articles | PMID: 23570880
Molecular genetic analysis in 93 patients and 193 family members with classical congenital adrenal hyperplasia due to 21-hydroxylase deficiency in Croatia.
J Steroid Biochem Mol Biol, 165(pt a):51-56, 31 Mar 2016
Cited by: 15 articles | PMID: 27041116
Allele-specific PCR and Next-generation sequencing based genetic screening for Congenital Adrenal Hyperplasia in India.
Eur J Med Genet, 64(12):104369, 27 Oct 2021
Cited by: 5 articles | PMID: 34718183
Congenital adrenal hyperplasia: update on prenatal diagnosis and treatment.
J Steroid Biochem Mol Biol, 69(1-6):19-29, 01 Apr 1999
Cited by: 40 articles | PMID: 10418977
Review