Abstract
Free full text

A novel natural variation in the promoter of GmCHX1 regulates conditional gene expression to improve salt tolerance in soybean
Abstract
Identification and characterization of soybean germplasm and gene(s)/allele(s) for salt tolerance is an effective way to develop improved varieties for saline soils. Previous studies identified GmCHX1 (Glyma03g32900) as a major salt tolerance gene in soybean, and two main functional variations were found in the promoter region (148/150 bp insertion) and the third exon with a retrotransposon insertion (3.78 kb). In the current study, we identified four salt-tolerant soybean lines, including PI 483460B (Glycine soja), carrying the previously identified salt-sensitive variations at GmCHX1, suggesting new gene(s) or new functional allele(s) of GmCHX1 in these soybean lines. Subsequently, we conducted quantitative trait locus (QTL) mapping in a recombinant-inbred line population (Williams 82 (salt-sensitive) × PI 483460B) to identify the new salt tolerance loci/alleles. A new locus, qSalt_Gm18, was mapped on chromosome 18 associated with leaf scorch score. Another major QTL, qSalt_Gm03, was identified to be associated with chlorophyll content ratio and leaf scorch score in the same chromosomal region of GmCHX1 on chromosome 3. Novel variations in a STRE (stress response element) cis-element in the promoter region of GmCHX1 were found to regulate the salt-inducible expression of the gene in these four newly identified salt-tolerant lines including PI 483460B. This new allele of GmCHX1 with salt-inducible expression pattern provides an energy cost efficient (conditional gene expression) strategy to protect soybean yield in saline soils without yield penalty under non-stress conditions. Our results suggest that there might be no other major salt tolerance locus similar to GmCHX1 in soybean germplasm, and further improvement of salt tolerance in soybean may rely on gene-editing techniques instead of looking for natural variations.
Introduction
Soil salinization that limits crop production is a global issue in agriculture (Munns and Tester, 2008; Gong et al., 2020; Li et al., 2023), and more than 45 million hectares accounting for at least 20% of total irrigated land has been affected by salt stress (Lauchli et al., 2008; Xiao and Zhou, 2022). This problem is being exacerbated and accelerated by adoption of irrigation (Carillo et al., 2011; Ondrasek et al., 2011; Sahab et al., 2021), and salt-affected farmland is predicted to double by the year 2050. In salinized conditions, plants suffer osmotic stress first, and then ionic stress associated with sodium (Na+) and chloride (Cl−) accumulation (Deinlein et al., 2014; Do et al., 2016, 2018; Wei et al., 2016; Wang et al., 2020; Yin et al., 2023). As a major legume crop, soybean (Glycine max (L.) Merr.) is widely grown and consumed for its high oil and protein contents (Singh, 2010; Duan et al., 2022). Under salt stress, the accumulation of Na+ and Cl− in soybean stems and leaves eventually leads to leaf chlorosis and necrosis, decrease of plant biomass, and yield reduction (Essa, 2002; Lee et al., 2008; Lu et al., 2021). Soybean yield could be reduced by 20–50% due to salt stress (Lee et al., 2008; Patil et al., 2016). To overcome the negative impacts of salt stress in soybean, it is essential to discover genetic resources and to understand the mechanism of salt tolerance (Leung et al., 2023).
A major salt tolerance gene, GmCHX1/GmSALT3/GmNcl, was mapped and cloned from both wild and cultivated soybeans (Guan et al., 2014; Qi et al., 2014; Do et al., 2016). GmCHX1 encodes Cation/Proton Antiporter 2 (CPA2), a transporter with tissue-specific expression in phloem and xylem cells that exports both Na+ and Cl– ions from xylem and reduces the accumulation of both ions in the shoot in saline conditions, but with extra energy cost (Guan et al., 2014; Qi et al., 2014; Liu et al., 2016; Cao et al., 2018). Two major functional variations were identified in GmCHX1: a 3.78-kb retrotransposon insertion in the third exon of GmCHX1 that led to non-functional GmCHX1 (Guan et al., 2014; Qi et al., 2014; Qu et al., 2022) and a 148/150-bp insertion in the GmCHX1 promoter, which may affect the transcriptional regulation of GmCHX1 through disrupting the effect of cis-element located upstream of the insertion site, that led to lower GmCHX1 expression (Guan et al., 2014; Nakano et al., 2020). A haplotype analysis of GmCHX1/GmSALT3 in 172 soybean lines revealed almost all salt-tolerant lines have no retrotransposon insertion in the third exon and no 148/150-bp insertion in the promoter, in contrast to most sensitive lines (Guan et al., 2014). The expression of GmCHX1 in the tolerant lines is consistently higher than that in the sensitive lines under both control and salt stress conditions, which could result in unnecessary energy cost with yield penalty under non-stress conditions (Guan et al., 2014; Qi et al., 2014). Expression and silence of the stress resilience genes under stress and non-stress conditions, respectively, is desirable to avoid unnecessary energy cost (Lin et al., 2003; Zhu et al., 2010). Stress-inducible promoters could be one strategy to precisely control the expression of stress resilience genes in different environments.
Other than the major locus on Chr. 03 harboring GmCHX1 gene (Lee et al., 2004; Hamwieh and Xu, 2008; Hamwieh et al., 2011; Ha et al., 2013; Do et al., 2018), a few loci associated with soybean salt tolerance were identified on Chrs 06, 07, 08, 10, 13, 15, 18, and 19 that explained the smaller phenotypic variation of each locus (Lee et al., 2004; Chen et al., 2008; Do et al., 2018, 2019; Cho et al., 2021; Guo et al., 2021). Therefore, the question of whether there is any other major salt tolerance locus in the soybean germplasm needs to be answered. The objectives of this study were to identify novel genetic resources (loci or alleles) for salt tolerance in soybean and to investigate a more energy cost efficient strategy to manage salt stress in soybean production. A new quantitative trait locus (QTL) for salt tolerance was identified on Chr. 18 in the recombinant inbred line (RIL) population of Williams 82 × PI 483460B (wild soybean). A novel GmCHX1 allele with salt stress-inducible promoter was identified in the same population, which provides a new genetic resource to develop an energy cost efficient (conditional gene expression) strategy to protect soybean yield in saline soils and non-stress conditions.
Materials and methods
Plant materials and growth conditions
Two soybean salt-tolerant lines, Lee and Fiskeby III, and three salt-sensitive lines, Jackson, Hutcheson, and Williams 82 (Luo et al., 2005; Do et al., 2018; Valliyodan et al., 2021), were used as checks in this study. Also, seven additional salt-tolerant lines were selected from the Nguyen Lab’s core soybean germplasm of 305 accessions as previously reported (Do et al., 2019). They included four wild soybean lines and three cultivated soybean lines.
The 182 F7:8 RILs derived from a cross of Williams 82 × PI 483460B as previously described (Patil et al., 2018) were used to analyse the salt tolerance QTLs in this study. The parental lines, Williams 82 and PI 483460B, are classified as maturity group III. The hybrids were made at the Fisher Delta Research Center, University of Missouri, Portageville, MO, USA and the single seed descent method was used to develop the mapping population. Plants were grown under a cycle of 16 h (26 °C) day–8 h (22 °C) night in a greenhouse at the University of Missouri, Columbia, MO, USA.
Evaluations for salt tolerance traits
Soybean plants were tested for salt tolerance using an established greenhouse method described by Lee et al. (2008) with minor modifications. Briefly, seven seedlings of each line at the vegetative growth stage V2, grown in Cone-tainers, were subjected with 100 mM NaCl solution. For the RIL population, the assays were conducted with two biological replicates and each replicate included seven seedlings per RIL. The other tested lines were evaluated with three biological replicates and each replicate had seven seedlings per line. When the two salt-sensitive checks, Jackson and Hutcheson, showed severe leaf scorch (approximately 2 or 3 weeks after the NaCl treatment), individual soybean plants were rated for leaf scorch score (LSS) using a 1–5 scale as previously described (Do et al., 2018), where 1 is no apparent chlorosis, 2 is slight chlorosis (25% of the leaves showed chlorosis), 3 is moderate chlorosis (50% of the leaves showed chlorosis and some necrosis), 4 is severe chlorosis (75% of the leaves showed chlorosis and severe necrosis), and 5 is dead (leaves showed severe necrosis and were withered).
Leaf chlorophyll content (CC) was quantified on individual plants using a chlorophyll meter (Chlorophyll meter SPAD-502, Konica Minolta). The leaf chlorophyll contents were measured before and after NaCl treatment (CC_CK and CC_Salt). Chlorophyll content ratio (CCR) was calculated as:
DNA, RNA isolation and quantitative real-time PCR
Genomic DNA was isolated from soybean leaves using the CTAB method (Murray and Thompson, 1980). Total RNA was isolated from root samples using the RNeasy Mini Kit (Qiagen, Hilden, Germany). Quantitative real-time PCR (qRT-PCR) was performed in a total volume of 15 μl with 2 μl diluted cDNA, 7.5 μl 2×SYBR, and 0.3 μM of gene-specific primers. The specific primers of GmCHX1 were designed and the housekeeping gene GmUKN1 (Glyma.12G020500) was used as an internal control (Guan et al., 2014). The sequences of primer pairs are listed in Supplementary Table S1. The qRT-PCR reactions were run under the following procedure: initial denaturation at 95 °C for 5 min, 40 cycles of denaturation at 95 °C for 10 s, annealing at 60 °C for 20 s, and extension at 72 °C for 20 s. The Roche 480 Realtime detection system (Roche Diagnostics, Switzerland) was used for the segment amplification and data analysis. The and methods were used to calculate the relative gene expression in transcript levels (Livak and Schmittgen, 2001).
Genotype analysis for GmCHX1 gene
Kompetitive allele specific PCR (KASP) assays were developed for three gene-based markers (GBMs) to genotype the tested soybean lines for GmCHX1. The markers Salt-20, Salt14056, and Salt11655 were designed for single nucleotide polymorphisms (SNPs) in the promotor, the third intron, and the fifth exon of GmCHX1, respectively, as previously described (Patil et al., 2016). The insertion–deletion (InDel) of 148/150 bp in the promoter of GmCHX1 was genotyped by PCR and agar gel electrophoresis using specific primers (Supplementary Table S1). For the 3.78 kb InDel in the third exon of GmCHX1 (Guan et al., 2014), cDNA was used as the template and specific primer pairs were used for PCR (Supplementary Table S1).
Linkage map construction and quantitative trait locus analysis
A bin map of the RIL population with 182 RILs and 4070 bins was available as previously reported by Patil et al. (2018). The program MapQTL 5.0 was initially used to detect the putative QTL by the interval mapping method (Van Ooijen, 2004). Composite interval mapping was then performed using the multi-QTL method (Van Ooijen, 2004). A significant threshold of logarithm of the odds (LOD) score was calculated for each trait by 1000 permutations to determine a QTL at the linkage group and genome-wide significance level of P=0.05 (Doerge and Churchill, 1996). Nomenclature of the identified QTL followed the SoyBase guidelines, where qSalt_Gm stands for qtl_Salt Tolerance_Glycine max_chromosome number. The epistatic interaction between salt tolerance QTL was analysed by the QTLNetwork 2.1 program (Yang et al., 2008).
Dual-luciferase reporter assay for promoter activity
Promoters of GmCHX1 from different soybean lines were cloned and sequenced. The sequence variation was detected by BLAST between different soybean lines, and cis-elements were analysed by PlantCARE (http://bioinformatics.psb.ugent.be/webtools/plantcare/html/). The sequence of the GmCHX1 promoter was cloned into the reporter vector of pGreenII 0800-Luc by One Step Cloning Kit (Vazyme, China) using KpnI and XhoI restriction sites. The recombinant plasmids were transformed into Agrobacterium tumefaciens GV3101. After culture, the GV3101 inoculums containing the reporter gene were injected into tobacco leaves. The infiltrated plants were grown under control or 100 mM NaCl treatment for 48 h. Subsequently, the luciferase activities were measured with a dual-luciferase reporter assay kit (Vazyme). The primer pairs used in this assay are listed in Supplementary Table S1.
Promoter-β-glucuronidase assay in soybean hairy root transformation
The cis-element of STRE (CCCCT) is crucial for the GmCHX1 promoter in responding to salt stress. Therefore, GmCHX1 promoter from PI 483460B (with STRE), Hutcheson (without STRE), and Hutcheson mutant (Hutcheson-mut, with STRE) were constructed into pCAMBIA3301 to drive the β-glucuronidase (GUS) gene in soybean hairy root. Soybean composite transgenic plants were developed according to Kereszt et al. (2007). The composite plants were treated with 1/4 Hoagland solution containing 0 or 100 mM NaCl for 24 h. GUS histochemical staining of hairy roots was conducted as described previously (Jefferson et al., 1987). qRT-PCR of GUS gene was performed in hairy roots under the control and NaCl treatment conditions. The primer pairs used in the assay are listed in Supplementary Table S1.
Statistical analysis
Phenotypic variations of LSS, CC_CK, CC_Salt, and CCR were analysed using the GLM procedure in SAS version 9.2 (SAS Institute, Inc., Cary, NC, USA). Broad-sense heritability was estimated as H2=σ2g/[σ2g+(σ2ge/e)+σ2/re], where σ2g is the genetic variance, σ2ge is the genotype by environment interaction variance, σ2 is the error variance, r is the number of replicates, and e is the number of environments. Duncan’s multiple range test or Student’s t-test was used to estimate the significance of difference and Pearson’s correlation (with SAS software) was used to estimate correlation coefficients.
Results
Identification of new soybean salt tolerance genetic resources
In our previous study, seven soybean accessions were identified as new soybean salt-tolerant sources (Do et al., 2019). Here, we confirmed the tolerant phenotype of the seven soybean lines showing significantly low LSS and high CCR compared with salt-sensitive checks under salt stress (Fig. 1A, ,B).B). We found all seven lines were carrying the salt-sensitive genotype of markers (Salt-20, Salt14056, and Salt11655) genotyped by KASP assays (Table 1) in GmCHX1. On further evaluation of the previously identified functional variations, the 148/150-bp InDel and 3.78-kb retrotransposon insertion (Fig. 2A) (Guan et al., 2014; Qi et al., 2014), four of the seven salt-tolerant lines (PI 424116, PI 483460B, PI 468908, PI 080837) were identified as having the 148/150-bp insertion (salt sensitive variation) in GmCHX1 promoter, the same as the salt-sensitive checks (Hutcheson and Jackson) (Fig. 2B). The 3.78-kb retrotransposon insertion was not detected in the third exon of GmCHX1 of the seven lines, and the insertion was only present in Williams 82 (Supplementary Fig. S1A, B).
Table 1.
Phenotype and genotype of selected soybean salt-tolerant lines
PI number | Species | Phenotype for salt stress | Genotypes of GBMsa in GmCHX1 | ||
---|---|---|---|---|---|
Salt-20 | Salt14056 | Salt11655 | |||
PI 518664 (Hutcheson) | Glycine max | Sensitive check | WT | WT | WT |
PI 548657 (Jackson) | Glycine max | Sensitive check | WT | WT | WT |
PI 424116 | Glycine soja | Tolerant | WT | WT | WT |
PI 483460B | Glycine soja | Tolerant | WT | WT | WT |
PI 468908 | Glycine max | Tolerant | WT | WT | WT |
PI 080837 | Glycine max | Tolerant | WT | WT | WT |
PI 378702 | Glycine soja | Tolerant | WT | WT | WT |
PI 407083 | Glycine soja | Tolerant | WT | WT | WT |
PI 417500 | Glycine max | Tolerant | WT | WT | WT |
PI 548656 (Lee) | Glycine max | Tolerant check | Mut | Mut | Mut |
PI 438471 (Fiskeby III) | Glycine max | Tolerant check | Mut | Mut | Mut |
PI 518671 (Williams 82) | Glycine max | Sensitive | WT | WT | WT |
a GBMs, gene-based markers.
The KASP assays of Salt-20, Salt14056, and Salt11655 were designed on SNPs in promotor, the third intron, and the fifth exon of GmCHX1, respectively.

Leaf scorch score (LSS) and chlorophyll content ratio (CCR) of the selected salt-tolerant soybean lines under salt stress conditions. (A) LSS rated from 1 to 5, where 1 is no apparent chlorosis and 5 is dead. (B) CCR calculated as ratio of chlorophyll contents after and before salt treatment. The selected salt-tolerant soybean lines, salt-sensitive checks (Hutcheson, Jackson, and Williams 82), and salt-tolerant checks (Lee and Fiskeby III) were treated with 100 mM NaCl for 3 weeks before LSS and CCR evaluation. Data represent the mean ±SD of three biological replicates. Significant differences (a, b, c, d, or e above the bars) were identified by Duncan’s multiple range test at P=0.05.
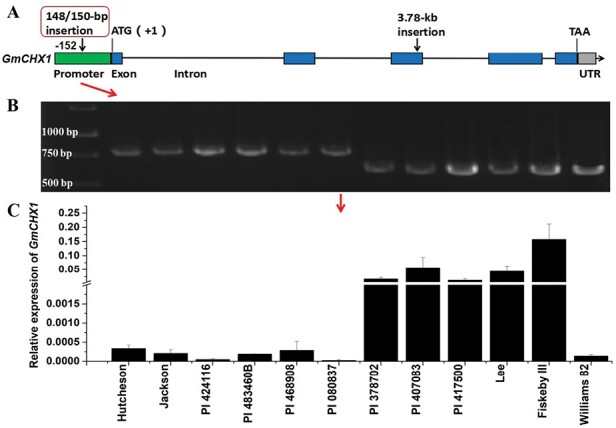
Characterization of the previously identified functional variation in GmCHX1 promoter and the corresponding relative expression of GmCHX1 in the selected salt-tolerant soybean lines and checks. (A) Graphical representation of the gene model and the previously identified functional variations of GmCHX1. (B) The genotype of the functional variation (148/150-bp insertion) in the GmCHX1 promoter of the corresponding soybean lines in (C). (C) The relative expression of GmCHX1 in the selected salt-tolerant soybean lines and checks under non-stress condition. Root samples were collected at vegetative growth stage V2 for qRT-PCR. The expression of GmCHX1 is relative to the house-keeping gene GmUKN1 (Glyma.12G020500). Data represent the mean ±SE of three replicates with three technical replicates for each sample.
The expression level of GmCHX1 under non-stress conditions was examined. As expected, the expression level of GmCHX1 was well-correlated with the presence/absence of the 148/150-bp insertion in the promoter under non-stress condition (Fig. 2B, ,C).C). The presence of the 148/150-bp insertion in the four lines PI 424116, PI 483460B, PI 468908, and PI 080837 led to significantly lower GmCHX1 expression, compared with the lines without the 148/150-bp deletion, under non-stress condition (Fig. 2B, ,C).C). Altogether, these results suggest that these fours lines carry new salt tolerance gene(s) or new alleles of GmCHX1.
Phenotypic variance and inheritance of salt tolerance traits in the recombinant inbred line population from Williams 82 × PI 483460B
To investigate if there was any new salt tolerance locus in these four newly identified lines, PI 483460B was selected for representative study. A RIL population derived from Williams 82 × PI 483460B was used to identify the above suggested new salt tolerance QTL/genes in soybean. The two parents, Williams 82 and PI 483460B, showed significant differences in salt tolerance as expected (Supplementary Fig. S2A). Significant variations in the four salt tolerance representative traits, including LSS, CCR, CC_CK, and CC_Salt, were observed in the RIL population with high heritability (0.80–0.86) (Supplementary Fig. S2B; Supplementary Table S2; Fig. 3A–D). The CC_CK value in Williams 82 was higher than in PI 483460B, and the opposite result for CC_Salt was observed after salt treatment (Fig. 3A, ,B).B). We observed transgressive segregations in these salt tolerance representative traits in this RIL population (Fig. 3C–E). A strong positive correlation between CC_Salt and CCR (r=0.95, P<0.001) and a relatively weak positive correlation between CC_Salt and CC_CK (r=0.39, P<0.001) were detected (Supplementary Table S3). No significant correlation was found between CC_CK and CCR. As expected, LSS had a significantly negative correlation (r≤-0.86, P<0.001) with CCR and CC_Salt (Supplementary Table S3).

Phenotypic evaluation of salt tolerance representative traits in 182 RILs derived from a cross between Williams 82 and PI 483460B. (A) Phenotypic distribution of chlorophyll content before NaCl treatment (CC_CK). (B) Phenotypic distribution of chlorophyll content after 100 mM NaCl treatment (CC_Salt). (C) Phenotypic distribution of chlorophyll content ratio (CCR). (D) Phenotypic distribution of leaf scorch score (LSS) after 100 mM NaCl treatment. (E) Representative RILs with extreme phenotype for salt tolerance in leaf scorch.
Quantitative trait locus mapping for salt tolerance in soybean recombinant inbred line population
QTL mapping was conducted to identify the genetic loci associated with CCR and LSS in the Williams 82 × PI 483460B population. A major locus, named as qSalt_Gm03, was mapped on Chr. 03 as being significantly associated with CCR and LSS, explaining 16.3% and 17.6% of the phenotypic variation (PVE, R2), respectively (Fig. 4A; Table 2). qSalt_Gm03 is located at the same genomic region as the previously identified salt tolerance gene GmCHX1 (Fig. 4A). A new locus on Chr. 18, denoted as qSalt_Gm18, was identified as being significantly associated with LSS, with LOD of 3.11 and R2 of 8.3% (Fig. 4B; Table 2). Both loci associated with salt tolerance had the donor alleles from salt-tolerant parent, PI 483460B (Table 2). No significant epistatic interaction was detected between qSalt_Gm03 and qSalt_Gm18.
Table 2.
QTL associated with salt tolerance-related traits was mapped in the RIL population of Williams 82 × PI 483460B
QTL | Traitsa | Chr | LOD | Flanking markersb | Nearest marker | Position (cM) | Additive effect | R 2 (%)c | Donord |
---|---|---|---|---|---|---|---|---|---|
qSalt_Gm03 | CCR | 3 | 6.97 | bin_3_38712409 bin_3_40006018 | bin_3_39385251 | 59.18 | 0.05 | 17.6 | PI 483460B |
LSS | 3 | 6.42 | bin_3_38712409 bin_3_40006018 | bin_3_39580451 | 59.51 | -0.36 | 16.3 | PI 483460B | |
qSalt_Gm18 | LSS | 18 | 3.11 | bin_18_4215290 bin_18_5198448 | bin_18_4745563 | 22.51 | -0.26 | 8.3 | PI 483460B |
a Traits for QTL mapping: CC_Salt: chlorophyll content after 100 mM NaCl treatment; CCR, chlorophyll content ratio where CCR=CC_Salt/CC_CK; LSS: leaf scorch score after 100 mM NaCl treatment.
b The flanking makers delimiting the QTL regions based on the logarithm of odds (LOD) score distribution in Fig. 4.
c The percentage contribution of the QTL to the total phenotypic variations for the respective traits.
d The parent from which the favorable alleles of the QTL came.
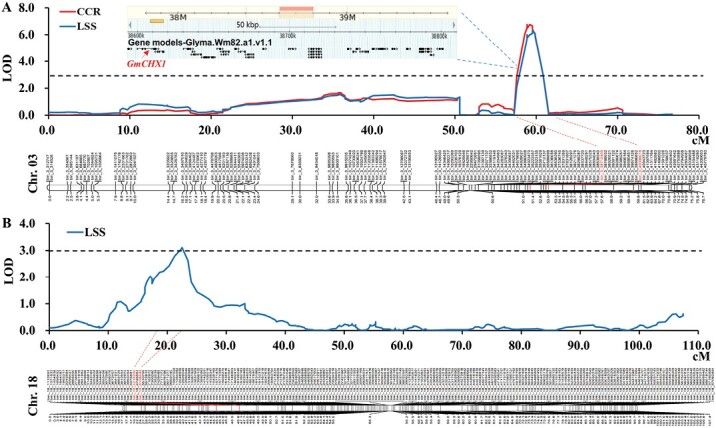
Graphical representation of QTL for salt tolerance mapped in the RIL population. (A) QTL qSalt_Gm03 associated with salt tolerance on chromosome 3. The candidate genes for qSalt_Gm03 are shown in the upper part of the figure and the red arrow indicates GmCHX1. (B) QTL qSalt_Gm18 associated with salt tolerance on chromosome 18. CCR, chlorophyll content ratio; LSS, leaf scorch score. The significance threshold of the logarithm of the odds (LOD) values estimated by 1000 genome-wide permutations tests for CCR and LSS was <3.0.
Identification of the salt-inducible expression of GmCHX1 responsible for qSalt_Gm03 in the four newly identified salt-tolerant lines including PI 483460B
To determine whether GmCHX1 underlies the qSalt_Gm03 locus in PI 483460B and the other three lines, the expression levels of GmCHX1 were examined in these four lines with five checks in response to salt treatment. As expected, under the control condition (0 h), the two tolerant checks (Lee and Fiskeby III) without the 148/150-bp insertion in the GmCHX1 promoter showed significantly higher GmCHX1 expression compared with the other four lines with the insertion (Fig. 5). In response to NaCl treatment from 12 to 48 h, Lee and Fiskeby III showed consistent high expression of GmCHX1, and the sensitive checks (Jackson and Hutcheson) showed consistent low expression of the gene (Fig. 5). Interestingly, the expression of GmCHX1 in the four new salt-tolerant lines was induced after 12 h of NaCl treatment and reached comparable expression levels in Lee and Fiskeby III at 24 h and 48 h, respectively, after the treatment (Fig. 5). These results suggest that a new functional allele of GmCHX1 is responsible for the qSalt_Gm03 locus in these four new salt-tolerant lines.
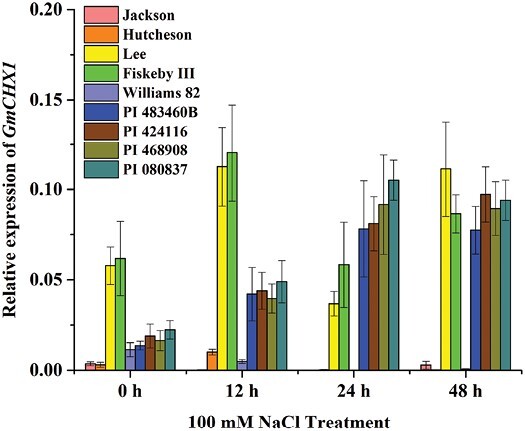
Relative gene expression level of GmCHX1 in response to salt stress in the selected soybean lines. Relative expression of GmCHX1 under 100 mM NaCl treatment for 0, 12, 24, and 48 h in the two parental lines (Williams 82 and PI 483460B), three new salt-tolerant lines (PI 424116, PI 468908, PI 080837), sensitive checks (Hutcheson and Jackson), and tolerant checks (Lee and Fiskeby III). Root samples were collected for qRT-PCR at vegetative growth stage V2 after 100 mM NaCl treatment. Data represent the mean ±SE of three replicates.
To understand the regulation of the salt-inducible expression of GmCHX1 in these four new salt-tolerant lines, we cloned the promoters of GmCHX1 from Hutcheson, PI 483460B (representing the four new salt-tolerant lines), and Fiskeby III into a luciferase reporter construct to evaluate the promoter activity in tobacco leaves using the dual-luciferase transcriptional activity assay. Under control condition, GmCHX1 promoter of Fiskeby III had significant higher transcriptional activity compared with those of Hutcheson and PI 483460B (Fig. 6A, ,B).B). After treating the tobacco leaves transformed with promoter::LUC constructs for 48 h, we observed significant increased transcriptional activity of GmCHX1 promoter in PI 483460B but not in Hutcheson and Fiskeby III (Fig. 6A, ,B).B). These results suggest that the salt-inducible expression pattern of GmCHX1 in these four new salt-tolerant lines is due to new variations in the promoter region of the gene instead of variations in other genes that transcribe GmCHX1.

Different promoter activity and DNA sequence variations in GmCHX1 promoter were detected in the selected soybean lines. (A) Representative images showing the luciferase activities of tobacco leaves infiltrated with an Agrobacteria strain harboring the corresponding reporter constructs with and without salt treatment. The Luciferase gene was driven by GmCHX1 promoter (1.7 kb) cloned from Hutcheson, PI 483460B, and Fiskeby III. Tobacco plants were under control (C) or 100 mM NaCl (Salt) condition for 48 h after leaf infiltration of the transient expression constructs. (B) Quantitative luciferase activities of samples shown in (A). A dual-luciferase assay was used to quantify the luciferase activity, and the relative firefly luciferase (F-Luc) activity was normalized to Renilla luciferase (R-Luc) activity. Data represent the mean ±SD of three replicates. Significant difference was tested by Student’s t-test. (C) cis-element analysis of the GmCHX1 promoters in the selected soybean lines. The physical positions of the nucleotides were numbered according to the reference genome (Williams 82) version Wm82.a2. +/− indicates the presence/absence of the insertion. (D) Two SNPs responsible for the presence/absence of a cis-element of STRE (CCCCT).
Characterization of the DNA variations in the GmCHX1 promoters
We initially sequenced and analysed the promoters of GmCHX1 in six soybean lines, including Jackson, Hutcheson, PI 483460B, Lee, Fiskeby III, and Williams 82 (Supplementary Table S4). Among these six lines, abundant SNPs/InDels were found, in addition to the previously reported 148/150-bp InDel in GmCHX1 promoter region (Supplementary Table S4). Twelve unique SNPs were identified in PI 483460B, which could be responsible to the salt-inducible expression of GmCHX1 (Supplementary Table S4). cis-element analysis was performed to identify all the potential transcription factor/enhancer binding sites in the GmCHX1 promoters of the six soybean lines (Fig. 6C; Supplementary Table S5). The GmCHX1 promoter in PI 483460B was identified as having two unique cis-elements (TC-rich motif and STRE cis-element) due the unique SNPs in PI 483460B at positions of −1500(C/G)/−1498(G/A) and −1530(A/C)/−1527(A/C), respectively (Fig. 6C, ,D;D; Supplementary Table S5). The STRE motif has been previously reported to respond to salt stress and could be responsible for the salt-inducible expression of GmCHX1 in PI 483460B (Schuller et al., 1994; Martinez-Pastor et al., 1996). Furthermore, to investigate if the other three new salt-tolerant lines share the same cis-element features at PI 483460B, we conducted DNA sequencing and sequence analysis. As expected, the STRE motif were also found in GmCHX1 promoter in the other three new salt-tolerant lines due to the same variation as detected in PI 483460B (Supplementary Fig. S3A, B).
Importance of STRE cis-element in the GmCHX1 promoter in response to salt stress
To test whether the STRE cis-element is responsible to the salt-inducible expression of GmCHX1 in the four new salt-tolerant lines, a promoter::GUS assay was performed in soybean transgenic hairy roots with the GUS gene driven by the GmCHX1 promoter of Hutcheson type (ACCAT), Hutcheson-mut (CCCCT, STRE, Hutcheson promoter backbone), and PI 483460B type (CCCCT, STRE) (Fig. 7A). As expected, no expression induction of GUS treated by NaCl was observed in the hairy roots transformed with the Hutcheson promoter::GUS construct, while significant induction of GUS expression was identified in the hairy roots transformed with the Hutcheson-mut promoter::GUS or PI 483460B promoter::GUS construct (Fig. 7B, ,C).C). This result confirmed the hypothesis that variation in the STRE element (ACCAT to CCCCT) is responsible for the salt-inducible expression of GmCHX1 under salt stress in the four new salt-tolerant lines.

Effects of the two SNPs in the STRE cis-element in regulation of the salt-inducible GmCHX1 expression. (A) Transgenic constructs to test the function of DNA variations in the STRE cis-element of the GmCHX1 promoters. The Hutcheson type GmCHX1 promoter was cloned directly from Hutcheson genomic DNA, and the Hutcheson-mut type GmCHX1 promoter was generated by mutating the original Hutcheson promoter from ACCAT into CCCCT at the STRE site. The PI 483460B type GmCHX1 promoter was cloned directly from PI 483460B genomic DNA. Promoters were cloned from a 1.7-kb region upstream the GmCHX1 gene start codon (ATG). (B) GUS staining in soybean hairy roots transformed with the transgenic construct from (A) under control and salt stress conditions. Scale bar: 1 mm. (C) GUS gene expression in soybean hairy roots from (B). Data represent the mean ±SD of three replicates. Significance of difference was tested by Student’s t-test. (D–F) GUS expression and staining in specific tissue driven by Hutcheson type (D), Hutcheson-mut type (E), and PI 483460B type (F) GmCHX1 promoter. Scale bar: 100 µm. Soybean composite plants with transgenic hairy roots were under control (C) or 100 mM NaCl (Salt) for 24 h.
Additionally, we observed GUS stain in the phloem and xylem cells of both control and salt-treated transgenic hairy roots transformed with all three promoter::GUS plasmids (Fig. 7D–F). This result suggests that the STRE motif in GmCHX1 promoter does not affect the previously reported phloem and xylem tissue-specific expression of GmCHX1 under salt stress (Guan et al., 2014), and the tissue-specific expression of GmCHX1 could be regulated by other common cis-elements shared with all alleles.
Discussion
New genetic resource for salt tolerance in soybean
Continuing efforts towards the identification of salt-tolerant germplasm and the cloning of salt-tolerant gene(s) is providing new genetic resources for salt tolerance improvement in crops, including soybean (Hamwieh et al., 2011; Guan et al., 2014; Qi et al., 2014; Do et al., 2016, 2018; Li et al., 2017). In soybean, QTL/gene mapping work for salt tolerance was initiated using 106 RILs derived from ‘S-100’ (salt tolerant) × ‘Tokyo’ (salt sensitive) (Lee et al., 2004). Several new soybean salt-tolerant germplasm lines were identified after large-scale salt tolerance evaluation, such as JWS156-1, W05, Tiefeng 8, Fiskeby III, IT162669, and NY36-87 (Luo et al., 2005; Hamwieh and Xu, 2008; Chen et al., 2013; Ha et al., 2013; Qi et al., 2014; Do et al., 2018; Cho et al., 2021; Guo et al., 2021). In this study, seven soybean lines were confirmed as salt-tolerant germplasm (Fig. 1), and four of them (PI 424116, PI 483460B, PI 468908, and PI 080837) were identified as untapped resources for potential novel determinants of salt tolerance other than GmCHX1 (Fig. 2; Table 1).
A major salt tolerance gene, GmCHX1, was previously mapped and cloned from soybean salt-tolerant germplasm (Guan et al., 2014; Qi et al., 2014; Do et al., 2016). Additionally, some putative QTLs for salt tolerance with relatively minor effects were reported on Chrs 07, 08, 13, 15, 18, and 19 in soybean (Lee et al., 2004; Chen et al., 2008; Do et al., 2018). In this study, a mapping population of 182 RILs derived from a cross of Williams 82 × PI 483460B was used to detect QTLs for salt tolerance (Fig. 3). A new salt tolerance locus, qSalt_Gm18, was mapped on Chr. 18 (Fig. 4B; Table 2), which was at least 7.5 Mb away from the salt tolerance-related QTL on Chr. 18 reported by Chen et al. (2008) and Do et al. (2019). The major salt tolerance locus in the newly identified four soybean lines is the same as GmCHX1 with a novel salt-inducible promoter (Fig. 4A; Table 2) (Lee et al., 2004; Guan et al., 2014; Qi et al., 2014; Do et al., 2018). After screening more than 300 soybean diverse lines (representing the USDA soybean germplasm collection), no new major salt tolerance locus was identified (Do et al., 2019). These results suggest that there might be a few other major salt tolerance loci like GmCHX1 in soybean germplasm and further improvement of salt tolerance in soybean may rely on gene pyramiding and gene-editing techniques.
New allele of GmCHX1 with a potential efficient energy cost for salt tolerance
Soybean germplasm is distributed widely in the world and exhibits genetic diversity for salt tolerance (Phang et al., 2008; Wu et al., 2014; Valliyodan et al., 2016; Chen et al., 2018). Molecular markers were developed to clone the major salt tolerance gene, GmCHX1, for marker-assisted selection in a soybean breeding program (Patil et al., 2016). The current study showed the expression of GmCHX1 was successively induced in PI 483460B and three other new salt-tolerant lines after 12 h of NaCl treatment, reaching a similar level to the salt-tolerant checks, Lee and Fiskeby III (Fig. 5). The dual-luciferase reporter assay confirmed the function of GmCHX1 promoter in salt-inducible expression of the gene in PI 483460B (Fig. 6A, ,B).B). DNA sequence alignment and cis-element analysis discovered a unique STRE cis-element in the GmCHX1 promoter of PI 483460B, which was confirmed to be responsible for the salt-inducible expression of GmCHX1 in a subsequent promoter::GUS assay (Fig. 7A, ,B,B, ,C).C). This discovery is consistent with the STRE motif responding to salt stress as previously reported (Schuller et al., 1994; Martinez-Pastor et al., 1996). Natural variations in the promoter affecting its expression pattern were also detected in GsERD15B (early responsive to dehydration 15B) and SlSOS1 (Salt overly sensitive 1) for salt tolerance (Jin et al., 2021; Wang et al., 2021). The STRE motif does not change the tissue expression profile of GmCHX1 promoter in phloem and xylem cells and the tissue-specific expression of GmCHX1 could be regulated by other common cis-elements shared with all alleles (Fig. 7D–F).
Qi et al. (2014) revealed that elimination of the salt tolerance gene in salt-sensitive germplasm could be due to negative selection when its function was not required under an unstressed environment. The consistent high expression of the resistance/tolerance gene allele could have extra energy cost and metabolic burden and even toxic effects to the host plants. A trade-off can often occur between the benefits of using the consistent high-expression resistance/tolerance genes and a yield penalty (Gururani et al., 2012; Vyska et al., 2016). The GmCHX1 promoter from PI 483460B with unique STRE motif identified in the current study can accurately control GmCHX1 expression to reduce adverse effects of salt stress on soybean plants when needed. Thus, the novel GmCHX1 allele with an accurate gene expression switch in the present study could provide an optimal strategy to improve salt tolerance and avoid a yield penalty under non-stress conditions.
Supplementary data
The following supplementary data are available at JXB online.
Fig. S1. The genotype of 3.78-kb insertion in the third exon of GmCHX1.
Fig. S2. Phenotype of leaf scorch and leaf scorch ranking in soybean lines under salt stress.
Fig. S3. The sequence alignment of GmCXH1 promoter for the other three new salt-tolerant soybean lines.
Table S1. The primer pairs were used in this study.
Table S2. ANOVA was performed for chlorophyll content and leaf scorch traits in the RIL population.
Table S3. Pearson correlation coefficients among chlorophyll content traits and leaf scorch score.
Table S4. The sequence polymorphism in GmCHX1 promoter among the parental lines and checks.
Table S5. The information for stress-related cis-elements in GmCXH1 promoter predicted by PlantCARE database.
erad404_suppl_Supplementary_Figure_S1-S3_Tables_S1-S3
erad404_suppl_Supplementary_Tables_S4-S5
Acknowledgements
The author YL gratefully acknowledge the financial support from China Scholarship Council (CSC) for visiting University of Missouri and carrying out this project. The authors acknowledge Dr Cheng Liu for advice on paper writing and editing.
Contributor Information
Yang Li, Institute of Nanfan & Seed Industry, Guangdong Academy of Science, Guangzhou, 510316, China. Division of Plant Science and Technology, University of Missouri, Columbia, MO 65211, USA. National Key Laboratory of Crop Genetics and Germplasm Enhancement, National Center for Soybean Improvement, Key Laboratory for Biology and Genetic Improvement of Soybean (General, Ministry of Agriculture), Jiangsu Collaborative Innovation Center for Modern Crop Production, Nanjing Agricultural University, Nanjing, 210095, China.
Heng Ye, Division of Plant Science and Technology, University of Missouri, Columbia, MO 65211, USA.
Tri D Vuong, Division of Plant Science and Technology, University of Missouri, Columbia, MO 65211, USA.
Lijuan Zhou, Division of Plant Science and Technology, University of Missouri, Columbia, MO 65211, USA.
Tuyen D Do, Division of Plant Science and Technology, University of Missouri, Columbia, MO 65211, USA.
Sushil Satish Chhapekar, Division of Plant Science and Technology, University of Missouri, Columbia, MO 65211, USA.
Wenqian Zhao, National Key Laboratory of Crop Genetics and Germplasm Enhancement, National Center for Soybean Improvement, Key Laboratory for Biology and Genetic Improvement of Soybean (General, Ministry of Agriculture), Jiangsu Collaborative Innovation Center for Modern Crop Production, Nanjing Agricultural University, Nanjing, 210095, China.
Bin Li, National Key Laboratory of Crop Genetics and Germplasm Enhancement, National Center for Soybean Improvement, Key Laboratory for Biology and Genetic Improvement of Soybean (General, Ministry of Agriculture), Jiangsu Collaborative Innovation Center for Modern Crop Production, Nanjing Agricultural University, Nanjing, 210095, China.
Ting Jin, National Key Laboratory of Crop Genetics and Germplasm Enhancement, National Center for Soybean Improvement, Key Laboratory for Biology and Genetic Improvement of Soybean (General, Ministry of Agriculture), Jiangsu Collaborative Innovation Center for Modern Crop Production, Nanjing Agricultural University, Nanjing, 210095, China.
Jinbao Gu, Institute of Nanfan & Seed Industry, Guangdong Academy of Science, Guangzhou, 510316, China.
Cong Li, Institute of Nanfan & Seed Industry, Guangdong Academy of Science, Guangzhou, 510316, China.
Yanhang Chen, Institute of Nanfan & Seed Industry, Guangdong Academy of Science, Guangzhou, 510316, China.
Yan Li, National Key Laboratory of Crop Genetics and Germplasm Enhancement, National Center for Soybean Improvement, Key Laboratory for Biology and Genetic Improvement of Soybean (General, Ministry of Agriculture), Jiangsu Collaborative Innovation Center for Modern Crop Production, Nanjing Agricultural University, Nanjing, 210095, China.
Zhen-Yu Wang, Institute of Nanfan & Seed Industry, Guangdong Academy of Science, Guangzhou, 510316, China.
Henry T Nguyen, Division of Plant Science and Technology, University of Missouri, Columbia, MO 65211, USA.
Author contributions
HY, HTN, and ZW oversaw the project and conceived research ideas; HY and YL designed the experiments and conducted phenotyping experiments for salt tolerance in RIL population; TDV, LZ, and TDD extracted DNA for the plant materials and performed the KASP assay; TDV, YL, and HY developed the mapping population; YL, WZ, JG, CL, and YC performed promoter sequence analysis and promoter activity assays; YL, HY, SSC, and LZ analysed the data and wrote the manuscript. All authors reviewed the manuscript.
Conflict of interest
The authors declare that they do not have any commercial or associative interest that represents a conflict of interest in connection with this work.
Funding
This work was supported by GDAS Project of Science and Technology Development (2022GDASZH-2022010102, 2020GDASYL-2020102011), Missouri Agricultural Experiment Station USDA Hatch project (MO-HAPS0002), Guangdong Basic and Applied Basic Research Foundation (2022A1515111146), Zhanjiang Science and Technology Plan Project (2022A01009), and Zhanjiang innovation and entrepreneurship team ‘pilot plan’ (211207157080997).
Data availability
All data supporting the findings of this study are available within the paper and its supplementary data published online.
References
- Cao D, Li Y, Liu B, Kong F, Tran L-SP. 2018. Adaptive mechanisms of soybean grown on salt-affected soils. Land Degradation & Development 29, 1054–1064. [Google Scholar]
- Carillo P, Annunziata MG, Pontecorvo G, Fuggi A, Woodrow P. 2011. Salinity stress and salt tolerance. In: Shanker A, Venkateswarlu B., eds. Abiotic stress in plants – mechanisms and adaptations, London: IntechOpen, 21–38. [Google Scholar]
- Chen HT, Cui SY, Fu SX, Gai JY, Yu DY. 2008. Identification of quantitative trait loci associated with salt tolerance during seedling growth in soybean (Glycine max L). Australian Journal of Agricultural Research 59, 1086–1091. [Google Scholar]
- Chen HT, Liu XQ, Zhang HM, Yuan XX, Gu HP, Cui XY, Chen X. 2018. Advances in salinity tolerance of soybean: Genetic diversity, heredity, and gene identification contribute to improving salinity tolerance. Journal of Integrative Agriculture 17, 2215–2221. [Google Scholar]
- Chen P, Yan K, Shao HB, Zhao SJ. 2013. Physiological mechanisms for high salt tolerance in wild soybean (Glycine soja) from Yellow River Delta, China: photosynthesis, osmotic regulation, ion flux and antioxidant capacity. PLoS One 8, e83227. [Europe PMC free article] [Abstract] [Google Scholar]
- Cho KH, Kim MY, Kwon H, Yang X, Lee SH. 2021. Novel QTL identification and candidate gene analysis for enhancing salt tolerance in soybean (Glycine max (L.) Merr). Plant Science 313, 111085. [Abstract] [Google Scholar]
- Deinlein U, Stephan AB, Horie T, Luo W, Xu GH, Schroeder JI. 2014. Plant salt-tolerance mechanisms. Trends in Plant Science 19, 371–379. [Europe PMC free article] [Abstract] [Google Scholar]
- Do TD, Chen HT, Vu HTT, et al. . 2016. Ncl synchronously regulates Na+, K+, and Cl −in soybean and greatly increases the grain yield in saline field conditions. Scientific Reports 6, 19147. [Europe PMC free article] [Abstract] [Google Scholar]
- Do TD, Vuong TD, Dunn D, et al. . 2018. Mapping and confirmation of loci for salt tolerance in a novel soybean germplasm, Fiskeby III. Theoretical and Applied Genetics 131, 513–524. [Abstract] [Google Scholar]
- Do TD, Vuong TD, Dunn D, Clubb M, Valliyodan B, Patil G, Chen PY, Xu D, Nguyen HT, Shannon JG. 2019. Identification of new loci for salt tolerance in soybean by high-resolution genome-wide association mapping. BMC Genomics 20, 318. [Europe PMC free article] [Abstract] [Google Scholar]
- Doerge RW, Churchill GA. 1996. Permutation tests for multiple loci affecting a quantitative character. Genetics 142, 285–294. [Europe PMC free article] [Abstract] [Google Scholar]
- Duan Z, Zhang M, Zhang Z, Liang S, Fan L, Yang X, Yuan Y, Pan Y, Zhou G, Liu S, Tian Z. 2022. Natural allelic variation of GmST05 controlling seed size and quality in soybean. Plant Biotechnology Journal 20, 1807–1818. [Europe PMC free article] [Abstract] [Google Scholar]
- Essa TA. 2002. Effect of salinity stress on growth and nutrient composition of three soybean (Glycine max L. Merrill) cultivars. Journal of Agronomy and Crop Science 188, 86–93. [Google Scholar]
- Gong ZZ, Xiong LM, Shi HZ, et al. . 2020. Plant abiotic stress response and nutrient use efficiency. Science China Life Sciences 63, 635–674. [Abstract] [Google Scholar]
- Guan RX, Qu Y, Guo Y, et al. . 2014. Salinity tolerance in soybean is modulated by natural variation in GmSALT3. Plant Journal 80, 937–950. [Abstract] [Google Scholar]
- Guo X, Jiang J, Liu Y, Yu L, Chang R, Guan R, Qiu L. 2021. Identification of a novel salt tolerance-related locus in wild soybean (Glycine soja Sieb. & Zucc.). Frontiers in Plant Science 12, 791175. [Europe PMC free article] [Abstract] [Google Scholar]
- Gururani MA, Jelli V, Chandrama PU, Akula N, Shashank KP, Se WP. 2012. Plant disease resistance genes: current status and future directions. Physiological and Molecular Plant Pathology 78, 51–65. [Google Scholar]
- Ha BK, Vuong TD, Velusamy V, Nguyen HT, Shannon JG, Lee JD. 2013. Genetic mapping of quantitative trait loci conditioning salt tolerance in wild soybean (Glycine soja) PI 483463. Euphytica 193, 79–88. [Google Scholar]
- Hamwieh A, Tuyen DD, Cong H, Benitez ER, Takahashi R, Xu DH. 2011. Identification and validation of a major QTL for salt tolerance in soybean. Euphytica 179, 451–459. [Google Scholar]
- Hamwieh A, Xu DH. 2008. Conserved salt tolerance quantitative trait locus (QTL) in wild and cultivated soybeans. Breeding Science 58, 355–359. [Google Scholar]
- Jefferson RA, Kavanagh TA, Bevan MW. 1987. GUS fusions: beta-glucuronidase as a sensitive and versatile gene fusion marker in higher plants. The EMBO Journal 6, 3901–3907. [Europe PMC free article] [Abstract] [Google Scholar]
- Jin T, Sun Y, Shan Z, He J, Wang N, Gai J, Li Y. 2021. Natural variation in the promoter of GsERD15B affects salt tolerance in soybean. Plant Biotechnology Journal 19, 1155–1169. [Europe PMC free article] [Abstract] [Google Scholar]
- Kereszt A, Li DX, Indrasumunar A, Nguyen CDT, Nontachaiyapoom S, Kinkema M, Gresshoff PM. 2007. Agrobacterium rhizogenes-mediated transformation of soybean to study root biology. Nature Protocols 2, 948–952. [Abstract] [Google Scholar]
- Lauchli A, James RA, Huang CX, McCully M, Munns R. 2008. Cell-specific localization of Na+ in roots of durum wheat and possible control points for salt exclusion. Plant, Cell and Environment 31, 1565–1574. [Abstract] [Google Scholar]
- Lee GJ, Boerma HR, Villagarcia MR, Zhou X, Carter TE, Li Z, Gibbs MO. 2004. A major QTL conditioning salt tolerance in S-100 soybean and descendent cultivars. Theoretical and Applied Genetics 109, 1610–1619. [Abstract] [Google Scholar]
- Lee JD, Smothers SL, Dunn D, Villagarcia M, Shumway CR, Carter TE, Shannon JG. 2008. Evaluation of a simple method to screen soybean genotypes for salt tolerance. Crop Science 48, 2194–2200. [Google Scholar]
- Leung HS, Chan LY, Law CH, Li MW, Lam HM. 2023. Twenty years of mining salt tolerance genes in soybean. Molecular Breeding 43, 45. [Europe PMC free article] [Abstract] [Google Scholar]
- Li MW, Xin D, Gao Y, Li KP, Fan K, Muñoz NB, Yung WS, Lam HM. 2017. Using genomic information to improve soybean adaptability to climate change. Journal of Experimental Botany 68, 1823–1834. [Abstract] [Google Scholar]
- Li J, Zhu Q, Jiao F, et al. . 2023. Research progress on the mechanism of salt tolerance in maize: a classic field that needs new efforts. Plants 12, 2356. [Europe PMC free article] [Abstract] [Google Scholar]
- Lin Y-H, Ludlow E, Kalla R, Pallaghy C, Emmerling M, Spangenberg G. 2003. Organ-specific, developmentally-regulated and abiotic stress-induced activities of four Arabidopsis thaliana promoters in transgenic white clover (Trifolium repens L). Plant Science 165, 1437–1444. [Google Scholar]
- Liu Y, Yu L, Qu Y, et al. . 2016. GmSALT3, which confers improved soybean salt tolerance in the field, increases leaf Cl −exclusion prior to Na+ exclusion but does not improve early vigor under salinity. Frontiers in Plant Science 7, 1485. [Europe PMC free article] [Abstract] [Google Scholar]
- Livak KJ, Schmittgen TD. 2001. Analysis of relative gene expression data using real-time quantitative PCR and the method. Methods 25, 402–408. [Abstract] [Google Scholar]
- Lu L, Wei W, Tao JJ, et al. . 2021. Nuclear factor Y subunit GmNFYA competes with GmHDA13 for interaction with GmFVE to positively regulate salt tolerance in soybean. Plant Biotechnology Journal 19, 2362–2379. [Europe PMC free article] [Abstract] [Google Scholar]
- Luo QY, Yu BJ, Liu YL. 2005. Differential sensitivity to chloride and sodium ions in seedlings of Glycine max and G. soja under NaCl stress. Journal of Plant Physiology 162, 1003–1012. [Abstract] [Google Scholar]
- Martinez-Pastor MT, Marchler G, Schuller C, Marchler-Bauer A, Ruis H, Estruch F. 1996. The Saccharomyces cerevisiae zinc finger proteins Msn2p and Msn4p are required for transcriptional induction through the stress-response element (STRE). The EMBO Journal 15, 2227–2235. [Europe PMC free article] [Abstract] [Google Scholar]
- Munns R, Tester M. 2008. Mechanisms of salinity tolerance. Annual Review of Plant Biology 59, 651–681. [Abstract] [Google Scholar]
- Murray MG, Thompson WF. 1980. Rapid isolation of high molecular weight plant DNA. Nucleic Acids Research 8, 4321–4325. [Europe PMC free article] [Abstract] [Google Scholar]
- Nakano Y, Kusunoki K, Maruyama H, Enomoto T, Tokizawa M, Iuchi S, Kobayashi M, Kochian LV, Koyama H, Kobayashi Y. 2020. A single-population GWAS identified AtMATE expression level polymorphism caused by promoter variants is associated with variation in aluminum tolerance in a local Arabidopsis population. Plant Direct 4, e00250. [Europe PMC free article] [Abstract] [Google Scholar]
- Ondrasek G, Rengel Z, Veres S. 2011. Soil salinisation and salt stress in crop production. In: Shanker A, Venkateswarlu B., eds. Abiotic stress in plants: mechanisms and adaptations. Rijeka: InTech, 171–190. [Google Scholar]
- Patil G, Do T, Vuong TD, Valliyodan B, Lee JD, Chaudhary J, Shannon JG, Nguyen HT. 2016. Genomic-assisted haplotype analysis and the development of high-throughput SNP markers for salinity tolerance in soybean. Scientific Reports 6, 19199. [Europe PMC free article] [Abstract] [Google Scholar]
- Patil G, Vuong TD, Kale S, et al. . 2018. Dissecting genomic hotspots underlying seed protein, oil, and sucrose content in an interspecific mapping population of soybean using high-density linkage mapping. Plant Biotechnology Journal 16, 1939–1953. [Europe PMC free article] [Abstract] [Google Scholar]
- Phang TH, Shao GH, Lam HM. 2008. Salt tolerance in soybean. Journal of Integrative Plant Biology 50, 1196–1212. [Abstract] [Google Scholar]
- Qi XP, Li MW, Xie M, et al. . 2014. Identification of a novel salt tolerance gene in wild soybean by whole-genome sequencing. Nature Communications 5, 4340. [Europe PMC free article] [Abstract] [Google Scholar]
- Qu Y, Guan R, Yu L, Berkowitz O, David R, Whelan J, Ford M, Wege S, Qiu L, Gilliham M. 2022. Enhanced reactive oxygen detoxification occurs in salt-stressed soybean roots expressing GmSALT3. Physiologia Plantarum 174, e13709. [Europe PMC free article] [Abstract] [Google Scholar]
- Sahab S, Suhani I, Srivastava V, Chauhan PS, Singh RP, Prasad V. 2021. Potential risk assessment of soil salinity to agroecosystem sustainability: Current status and management strategies. The Science of the Total Environment 764, 144164. [Abstract] [Google Scholar]
- Schuller C, Brewster JL, Alexander MR, Gustin MC, Ruis H. 1994. The HOG pathway controls osmotic regulation of transcription via the stress-response element (STRE) of the Saccharomyces cerevisiae CTT1 gene. The EMBO Journal 13, 4382–4389. [Europe PMC free article] [Abstract] [Google Scholar]
- Singh G. 2010. The soybean: botany, production and uses. Wallingford, UK: CABI. [Google Scholar]
- Valliyodan B, Brown AV, Wang J, et al. . 2021. Genetic variation among 481 diverse soybean accessions, inferred from genomic re-sequencing. Scientific Data 8, 50. [Europe PMC free article] [Abstract] [Google Scholar]
- Valliyodan B, Qiu D, Patil G, et al. . 2016. Landscape of genomic diversity and trait discovery in soybean. Scientific Reports 6, 23598. [Europe PMC free article] [Abstract] [Google Scholar]
- Van Ooijen J. 2004. MapQTL® 5, Software for the mapping of quantitative trait loci in experimental populations. Wageningen, Netherlands: Kyazma BV. [Google Scholar]
- Vyska M, Cunniffe N, Gilligan C. 2016. Trade-off between disease resistance and crop yield: a landscape-scale mathematical modelling perspective. Journal of the Royal Society, Interface 13, 20160451. [Europe PMC free article] [Abstract] [Google Scholar]
- Wang Z, Hong Y, Li Y, Shi H, Yao J, Liu X, Wang F, Huang S, Zhu G, Zhu JK. 2021. Natural variations in SlSOS1 contribute to the loss of salt tolerance during tomato domestication. Plant Biotechnology Journal 19, 20–22. [Europe PMC free article] [Abstract] [Google Scholar]
- Wang Z, Hong Y, Zhu G, et al. . 2020. Loss of salt tolerance during tomato domestication conferred by variation in a Na+/K+ transporter. The EMBO Journal 39, e103256. [Europe PMC free article] [Abstract] [Google Scholar]
- Wei PP, Wang LC, Liu AL, Yu BJ, Lam HM. 2016. GmCLC1 confers enhanced salt tolerance through regulating chloride accumulation in soybean. Frontiers in Plant Science 7, 1082. [Europe PMC free article] [Abstract] [Google Scholar]
- Wu G, Zhou ZD, Chen P, Tang XL, Shao HB, Wang HY. 2014. Comparative ecophysiological study of salt stress for wild and cultivated soybean species from the Yellow River Delta, China. The Scientific World Journal 2014, 651745. [Europe PMC free article] [Abstract] [Google Scholar]
- Xiao F, Zhou H. 2022. Plant salt response: perception, signaling, and tolerance. Frontiers in Plant Science 13, 1053699. [Europe PMC free article] [Abstract] [Google Scholar]
- Yang J, Hu CC, Hu H, Yu RD, Xia Z, Ye XZ, Zhu J. 2008. QTLNetwork: mapping and visualizing genetic architecture of complex traits in experimental populations. Bioinformatics 24, 721–723. [Abstract] [Google Scholar]
- Yin P, Liang X, Zhao H, Xu Z, Chen L, Yang X, Qin F, Zhang J, Jiang C. 2023. Cytokinin signaling promotes salt tolerance by modulating shoot chloride exclusion in maize. Molecular Plant 16, 1031–1047. [Abstract] [Google Scholar]
- Zhu LP, Yu Z, Zou CX, Li QL. 2010. Plant stress-inducible promoters and their function. Yi Chuan 32, 229–234. [Abstract] [Google Scholar]
Articles from Journal of Experimental Botany are provided here courtesy of Oxford University Press
Full text links
Read article at publisher's site: https://doi.org/10.1093/jxb/erad404
Read article for free, from open access legal sources, via Unpaywall:
https://academic.oup.com/jxb/advance-article-pdf/doi/10.1093/jxb/erad404/52346069/erad404.pdf
Citations & impact
Impact metrics
Alternative metrics

Discover the attention surrounding your research
https://www.altmetric.com/details/155942630
Article citations
Natural Variation in the Promoter of GmSPL9d Affects Branch Number in Soybean.
Int J Mol Sci, 25(11):5991, 30 May 2024
Cited by: 0 articles | PMID: 38892178 | PMCID: PMC11172651
Salt Tolerance in Soybeans: Focus on Screening Methods and Genetics.
Plants (Basel), 13(1):97, 28 Dec 2023
Cited by: 0 articles | PMID: 38202405 | PMCID: PMC10780708
Review Free full text in Europe PMC
Similar Articles
To arrive at the top five similar articles we use a word-weighted algorithm to compare words from the Title and Abstract of each citation.
Genomic-assisted haplotype analysis and the development of high-throughput SNP markers for salinity tolerance in soybean.
Sci Rep, 6:19199, 19 Jan 2016
Cited by: 82 articles | PMID: 26781337 | PMCID: PMC4726057
Identification of a major QTL allele from wild soybean (Glycine soja Sieb. & Zucc.) for increasing alkaline salt tolerance in soybean.
Theor Appl Genet, 121(2):229-236, 05 Mar 2010
Cited by: 49 articles | PMID: 20204319
Identification of new loci for salt tolerance in soybean by high-resolution genome-wide association mapping.
BMC Genomics, 20(1):318, 25 Apr 2019
Cited by: 26 articles | PMID: 31023240 | PMCID: PMC6485111
Overexpression of an aquaporin protein from Aspergillus glaucus confers salt tolerance in transgenic soybean.
Transgenic Res, 30(6):727-737, 30 Aug 2021
Cited by: 4 articles | PMID: 34460070
Review
Funding
Funders who supported this work.
GDAS Project of Science and Technology Development (2)
Grant ID: 2022GDASZH-2022010102
Grant ID: 2020GDASYL-2020102011
Guangdong Basic and Applied Basic Research Foundation (1)
Grant ID: 2022A1515111146
United States Department of Agriculture (1)
Grant ID: MO-HAPS0002
Zhanjiang Science and Technology Plan Project (1)
Grant ID: 2022A01009
Zhanjiang innovation and entrepreneurship team 'pilot plan' (1)
Grant ID: 211207157080997
Zhanjiang innovation and entrepreneurship team ‘pilot plan’ (1)
Grant ID: 211207157080997