Abstract
Free full text

Isolation and draft genome sequence of Enterobacter asburiae strain i6 amenable to genetic manipulation
Abstract
Enterobacter asburiae is a species of Gram-negative bacteria that is found in soil, water, and sewage. E. asburiae is generally considered to be an opportunistic pathogen, but has also been reported as a plant growth-promoting bacterium (PGPB), which may have beneficial effects on plant growth and development. However, genetic analysis of E. asburiae has been limited, possibly due to its redundant enzymes that digest exogenous DNA in the cell. Here, an E. asburiae strain i6 was isolated from soil in Nara, Japan. This strain was amenable to transformation and the one-step gene inactivation method based on λ Red recombinase. The transformation efficiency of the i6 strain with the 10 kb plasmid DNA pCF430 was at least four orders of magnitude higher than that of the previously sequenced E. asburiae strain ATCC 35953, which could not be transformed with the same plasmid DNA. A draft genome sequence of the i6 strain was determined and deposited into the database, allowing several factors that may determine transformation efficiency to be perturbed and tested. Together with the amenability of the i6 strain to genetic manipulation, the information from the i6 genome will facilitate characterization and fine-tuning of the beneficial and detrimental traits of this species.
Introduction
Enterobacter asburiae is a Gram-negative, facultative anaerobic, oxidase-negative, non-motile, and non-pigmented rod-shaped species belonging to the genus Enterobacter, which includes species that are difficult to identify with biochemical and phylogenetic tests 1-3. The Enterobacter cloacae complex (ECC) is a group of common opportunistic pathogens consisting of Enterobacter asburiae, Enterobacter cloacae, Enterobacter hormaechei, Enterobacter kobei, Enterobacter ludwigii, Enterobacter mori, and Enterobacter nimipressuralis. In addition, recently identified species, including Enterobacter roggenkampii, Enterobacter chengduensis, and Enterobacter bugandensis, are clustered with the ECC species 4. While E. asburiae, as a part of ECC, is considered to be an opportunistic pathogen, not only E. asburiae but also some of Enterobacter genus have been reported as plant growth-promoting bacteria (PGPB). Examples include E. asburiae PDA 134 from date palm 5, E. cloacae from citrus and maize plants 6, 7, and E. asburiae from sweet potato 8. Enterobacter sp. strain P23 promotes rice growth under salt stress. In addition, E. mori, E. asburiae, E. ludwigii, and E. sp. J49 have been shown to promote wheat growth under stress conditions 9. At least some strains of E. asburiae reduce the epiphytic fitness of the human enteric pathogens E. coli O157:H7 and Salmonella on lettuce and Arabidopsis by at least 100-fold 9, 10. While E. asburiae is a preferable candidate species for genome editing to safely further enhance the ability of PGPB and to study cell-cell interactions, genetic analysis of this important species is limited 11, 12, possibly due to its redundant enzymes that digest exogenous DNA within the cell.
The one-step gene inactivation method based on λ Red recombinase is a powerful and efficient technique used to disrupt specific genes 13 and to construct gene fusions 14 in the bacterial genome. Soon after this method was originally invented in Escherichia coli 13, it was widely used to construct deletion mutants and insertion mutants of other Gram-negative species, such as Salmonella enterica 15, Yersinia pestis 16, Klebsiella pneumoniae 17, Pantoea ananatis 18. Basically, the technique uses knockout cassettes with short (40-60 bp) homologous arms that can be produced in a single PCR reaction, but some species or strains only accept knockout cassettes with extended arms (200~1,000 bp), which require additional PCR steps to successfully manipulate the gene with λ Red recombinase 19, 20. To date, the one-step gene inactivation method has been unsuccessful with the E. asburiae ATCC 35953 (NBRC 109912 T) strain. In addition to genetic factors that reduce the stability of linear DNA fragments, genetic factors that reduce transformation efficiency have generally been thought to hinder genetic manipulations, such as the one-step gene inactivation method. Here, I report a strain of E. asburiae i6, isolated from soil in Nara Japan, that is amenable to genetic manipulation.
Materials and Methods
Bacterial strains, plasmids, and growth conditions
Bacterial strains and plasmids used in this study are listed in Table Table1.1. Bacteria were grown at 37ºC in LB Broth (Lennox). Ampicillin and spectinomycin were used at 100 µg/ml, kanamycin at 50 µg/ml, chloramphenicol at 25 µg/ml, and tetracycline at 12.5 µg/ml. Primers used in this study are listed in Table Table22.
Table 1
Bacterial strains and plasmids used in this study.
Strain or plasmid | Description | Reference or source |
---|---|---|
E. asburiae | ||
109912 T(ATCC 35953) | Wild type | NBRC |
i6 | Wild type | This work |
AK1599 | i6 wzc-FRT-KmR-FRT | This work |
AK1602 | i6 ![]() | This work |
AK1603 | i6 ![]() | This work |
AK1604 | i6 ![]() | This work |
AK1605 | i6 ![]() | This work |
E. coli | ||
DH5α | F- Φ80dlacZΔM15 Δ(lacZYA-argF)U169 deoR recA1 endA1 hsdR17(rK- mK+) phoA supE44 thi-1 gyrA96 relA1 | Laboratory stock |
JM109λpir | recA1 endA1 gyrA96 thi1 hsdR17(rK- mK+) e14- (mcrA-) supE44 relA1 Δ(lac-proAB)/F' [traD36, proAB+, lacIq, lacZΔM15] λpir | Laboratory stock |
Salmonella enterica | ||
MS5996 | phoQ::Tn10 | 40 |
EG16468 | ![]() | 23 |
Plasmids | ||
pKD3 | repR6Kγ ApR FRT CmR FRT | 36 |
pKD4 | repR6Kγ ApR FRT KmR FRT | 36 |
pCP20 | reppSC101ts ApR CmR cl857 λPR flp | 41 |
pCF430 | repRK2 oriTRK2 pBAD araC TcR | 33 |
pACYC177 | repp15A ApR KmR | 35 |
RSFRedTER | repRSF1010 oriVRSF1010 lacI sacB CmR γ β exo | 18 |
RSFRedTER-Sp | repRSF1010 oriVRSF1010 lacI sacB SpR γ β exo | This work |
pAK1001 | reporiS, CmR, FRT-KmR-FRT | 34 |
Table 2
Primers used in this study.
Primer name | Sequence |
---|---|
63f | CAGGCCTAACACATGCAAGTC |
1387r | GGGCGGWGTGTACAAGGC |
Hsp60-F | GGTAGAAGAAGGCGTGGTTGC |
Hsp60-R | ATGCATTCGGTGGTGATCATCAG |
A1190 | CAGCATCCTTGAACAAGGACAATTAACAGTTAACAAATAAGCTGTAATGCAAGTAGCG |
A1191 | AGGTGGGACCACCCGCGCTACTGCCGCCAGGCAAAGAATCTTTATTTGCCGACTACCTTGA |
A2414 | CTCCCCTCTGGGGAGAGGGTTAGGGTGAGGGGGATTTTTAGTGTAGGCTGGAGCTGCTTC |
A2415 | CGGCTATTACGAGTACGAATATAAATCTGACAGCAAATAACATATGAATATCCTCCTTAG |
A2416 | AAAAGCTTGCTGTAGCAAGGTAGCCTTATACATGAACAATGTGTAGGCTGGAGCTGCTTC |
A2417 | TCCCCGCGGGAGAGGGACGGGGTGAGACACCCGTCCGGGACATATGAATATCCTCCTTAG |
A2418 | GCGATGCCGGAACCGAGAATATGTTAACCGTGGAGGATCTGTGTAGGCTGGAGCTGCTTC |
A2419 | TCTCCTTTAGTCGGGCGGCATCGCCGCCCGTGGTCAGTCACATATGAATATCCTCCTTAG |
A2420 | CGGGCATTAAACAACAGCACCAGCATAAGGAAATAGTTTACTCTAATGCGCTGTTAATCACT |
A2421 | GCACCGGGGATAATGCAGCATTCATGTTCGGCATTCCTCACTAAGCACTTGTCTCCTGTT |
A2424 | AATTATCAAATTCACGCGTTCGGACATCTTCCCTGACGGCGGAATAGGAACTTCAAGATC |
A2425 | GCTGCTGCATATCTGGTATACCGACGGCTGGACGCCGTCACATATGAATATCCTCCTTAG |
PCR. PCRs for 16S and groEL were performed using primer sets 63f/1387r 21 and Hsp60-F/Hsp60-R 22 according to their protocols 21, 22. Purified PCR products were analyzed by DNA sequencing.
Construction of chromosomal gene deletion mutants
Strain AK1602, which has a deletion of the rcsB gene and a CmR cassette, was constructed by the one-step inactivation method 13 using primers A2414 and A2415 with pKD3 as the template.
Strain AK1603, which has a deletion of the klcA1 gene and a tetA, was constructed by the one-step inactivation method 13 using primers A2418 and A2419 with the phoQ::Tn10 chromosomal DNA as the template.
Strain AK1599, which has an insertion of the KmR cassette behind the wzc gene, was constructed by the one-step inactivation method 13 using primers A2416 and A2417 with pKD4 as the template.
Strain AK1604, which has a deletion of the klcA2 gene and a KmR cassette, was constructed by the one-step inactivation method 13 using primers A2420 and A2421 with pKD4 as the template.
Strain AK1605, which has a deletion of the dgeC gene and a KmR cassette, was constructed by the one-step inactivation method 13 using primers A2424 and A2425 with pKD4 as the template.
Plasmid construction
Plasmid RSFRedTER-Sp for λ Bet Exo Gam expression was constructed as a spectinomycin-resistant version of RSFRedTER 18 by the one-step inactivation method 13 using primers A1190 and A1191 with EG16468 chromosomal DNA 23 as the SpR marker template.
All strains constructed using PCR reactions were analyzed by DNA sequencing to confirm that the PCR-generated DNA regions had the predicted sequences.
Transformation efficiency analysis
Bacterial cells cultured overnight in LB (Lennox) were added to 10 ml of fresh medium diluted 1:50 in test tubes and shaken at 37°C until the OD595 value reached 0.3~0.8. Cells were collected by centrifugation and the supernatant was removed. The cell pellet was washed twice with 1 ml of 10% glycerol, and then the cells were suspended in 400 µl of 10% glycerol. 1-3 µl of plasmid DNA was mixed with 100 µl of competent cells (the cell suspension) and electroporated using a 2 mm cuvette and a Gene Pulser II (Bio-Rad) at 2.5 kV, 200 Ω, and 25 µF. Immediately after the pulse, SOB medium was added to the cuvette and collected in 1.5 ml tubes. Cells were spread on LB (Lennox) plates containing kanamycin (pAK1001 and pACYC177) or tetracycline (pCF430) and incubated at 37°C overnight. Colonies were then counted.
Genome sequencing, assembly and annotation
Whole genome sequencing was performed using DNBSEQ-G400 (MGI Tech Co., Ltd.). Libraries were prepared using IMGIEasy FS DNA Library Prep Set (MGI Tech Co., Ltd.) and fragments were validated using Fragment Analyzer and dsDNA 915 Reagent Kit (Agilent Technologies). Sequences were de novo assembled using SPAdes assembler version 3.15.5 42. Genome annotation was performed using DFAST.
Average nucleotide identity analysis
Average nucleotide identity (ANI) analysis 24 was performed using the complete genome sequences of all Enterobacter species available in the NCBI database.
Results and Discussion
Isolation and genome sequencing of the Enterobacter asburiae i6
On July 6, 2016, an E. asburiae strain was isolated from the soil near the lawn of the campus of Kindai University Faculty of Agriculture in Nara, Japan, as a strain that somewhat affects the luminescence of a Salmonella enterica strain carrying plasmid luciferase reporter under the control of the RcsB-regulated wza gene promoter, when cross-streaked. In addition, the colony morphology of this strain is slightly mucoid (or encapsulated) on LB agar plate. 16s rRNA and groEL sequencing determined that the strain was approximately E. asburiae, and this strain was designated i6. Because the i6 strain may produce a signal molecule that is recognized by S. enterica by an unknown mechanism, it would be useful to sequence the genome of strain i6 for future analyses. Therefore, chromosomal DNA was extracted from strain i6 and the genome was sequenced on a next-generation sequencer (DNBSEQ-G400) with X290 coverage. Automated assembly resulted in the formation of 28 contigs, and the longer contigs were dissected by redundant copies of 5s rRNA, 16S rRNA, 23 rRNA, and tRNA. The assembled genome sequences and their annotations were deposited in a database (accession numbers: BTPF01000001~BTPF01000028, DRR495940, PRJDB16388, and SAMD00634955). The sequences of the ATCC 35953, FDAARGOS_892, L1, and RHBSTW-01009 strains, all of which belong to E. asburiae (sensu stricto) and not to the four provisional classes (B-E) of E. asburiae according to GTDB 25, were the closest to i6 among the complete E. asburiae genomes (Table (Table3).3). The FDAARGOS_892 strain had the highest ANI value of 98.75%, whereas the L1 strain had the longest average alignment length of 70.08%. Thus, the i6 strain is most likely to be E. asburiae (sensu stricto). Alignment of the ends of each contig sequence of i6 to these sequences suggested that the large contigs 1-7, 9, and 10 form an almost complete chromosome of i6 (Table (Table4),4), and that contig 8 is a single plasmid DNA itself. Furthermore, the gaps between these contigs were shown to correspond to repetitive 16S rRNA and 23 rRNA, tRNA-Ala, tRNA-Ile, and tRNA-Glu (Table (Table44).
Table 3
Average nucleotide identity analysis (ANI) data calculated from the nearly complete genome of Enterobacter asburiae i6 strain and the whole genome sequence of each Enterobacter strains.
Bacterial strains | Total length (bp) | Average aligned length (bp) | Average aligned length (%) respect to Enterobacter asburiae i6 | ANI value (%) respect to Enterobacter asburiae i6 |
---|---|---|---|---|
Enterobacter asburiae i6 | 4,623,660 | 4,623,660 | 100.00 | 100.00 |
Enterobacter asburiae (sensu stricto) FDAARGOS_892 | 4,784,820 | 3,045,120 | 65.86 | 98.75 |
Enterobacter asburiae (sensu stricto) ATCC 35953 | 4,804,200 | 3,122,446 | 67.53 | 98.71 |
Enterobacter asburiae (sensu stricto) RHBSTW-01009 | 4,652,220 | 2,968,019 | 64.19 | 98.67 |
Enterobacter asburiae (sensu stricto) L1 | 4,626,720 | 3,240,164 | 70.08 | 98.63 |
Enterobacter asburiae (B) MNCRE14 | 4,491,060 | 3,000,470 | 64.89 | 97.01 |
Enterobacter asburiae (B) UBA11899 | 4,111,620 | 2,892,021 | 62.55 | 96.99 |
Enterobacter asburiae (B) 17Nkhm-UP2 | 4,709,340 | 3,041,453 | 65.78 | 96.95 |
Enterobacter asburiae (B) UBA8264 | 4,433,940 | 3,091,305 | 66.86 | 96.93 |
Enterobacter asburiae (B) 1808-013 | 4,769,520 | 3,236,906 | 70.01 | 96.91 |
Enterobacter asburiae (D) R_A5.MM | 4,837,860 | 3,144,464 | 68.01 | 95.90 |
Enterobacter asburiae (C) TN152 | 5,042,880 | 2,899,550 | 62.71 | 95.22 |
Enterobacter roggenkampii DSM 16690 | 4,899,060 | 3,026,517 | 65.46 | 93.03 |
Enterobacter chengduensis WCHECl-C4 | 5,183,640 | 2,917,804 | 63.11 | 93.21 |
Lelliottia nimipressuralis 51 (Enterobacter nimipressuralis) | 4,862,340 | 2,888,082 | 62.46 | 92.70 |
Enterobacter bugandensis EB-247 | 4,717,500 | 2,825,931 | 59.90 | 91.52 |
Enterobacter mori ACYC.E9L | 4,807,260 | 2,814,942 | 60.88 | 90.17 |
Enterobacter cloacae subsp. cloacae ATCC 13047 (GCF_000025565.1) | 5,596,740 | 2,783,201 | 60.19 | 88.73 |
Enterobacter ludwigii EN-119 | 4,952,100 | 2,777,488 | 56.09 | 88.54 |
Enterobacter hormaechei ATCC 49162 | 4,884,780 | 2,696,351 | 55.20 | 87.69 |
Enterobacter asburiae (E) INSAq146 | 4,456,380 | 2,514,955 | 54.39 | 86.68 |
GTDB 25 categories for E. asburiae, sensu stricto or provisional classes (B-E), are indicated in bold.
Table 4
Contig sequences and gaps in E. asburiae i6 assigned to the complete genome of the closest E. asburiae strains.
Bacterial strain | Enterobacter asburiae (sensu stricto) ATCC 35953 | |||||||||
i6 contig# | 1 | 2 | 4 | 7 | 5 comp | 9 comp | 10 | 6 | 3 comp | 1 |
Aligned 5'-end position on ATCC 35953 (nt) | 0 | 1691756 | 2687714 | 3150068 | 3315195 | 3588808 | 3689839 | 3776714 | 3973404 | 4652519 |
Aligned 3'-end position on ATCC 35953 (nt) | 1691839 | 2683077 | 3144943 | 3310295 | 3583909 | 3684694 | 3771799 | 3968594 | 4647866 | 4713742 |
Gap to right next contig (nt) | -83 | 4637 | 5125 | 4900 | 4899 | 5145 | 4915 | 4810 | 4653 | |
Gene assigned at gap right next contig | 23S rRNA | 23S rRNA | 23S rRNA | 16S rRNA | 16S rRNA | 16S rRNA | 16S rRNA | 16S rRNA | ||
tRNA-Ala | tRNA-Glu | 16S rRNA | tRNA-Glu | tRNA-Ile | tRNA-Ile | tRNA-Glu | tRNA-Glu | |||
tRNA-Ile | 16S rRNA | 23S rRNA | tRNA-Ala | tRNA-Ala | 23S rRNA | 23S rRNA | ||||
16S rRNA | 5S rRNA | 23S rRNA | 23S rRNA | |||||||
5S rRNA | ||||||||||
Bacterial strain | Enterobacter asburiae (sensu stricto) FDAARGOS_892 | |||||||||
i6 contig# | 1 comp | 4 | 7 | 5 comp | 9 comp | 10 | 6 | 3 comp | 2 comp | 1 comp |
Aligned 5'-end position on FDAARGOS_892 (nt) | 0 | 17644 | 479998 | 645123 | 918757 | 1019791 | 1106666 | 1303359 | 1981865 | 2972903 |
Aligned 3'-end position on FDAARGOS_892 (nt) | 12628 | 474876 | 640226 | 913839 | 1014646 | 1101753 | 1298549 | 1977602 | 2972986 | 4717539 |
Gap to right next contig (nt) | 5016 | 5122 | 4897 | 4918 | 5145 | 4913 | 4810 | 4263 | -83 | |
Gene assigned at gap right next contig | 23S rRNA | 23S rRNA | 23S rRNA | 16S rRNA | 16S rRNA | 16S rRNA | 16S rRNA | 16S rRNA | ||
tRNA-Ala | tRNA-Glu | 16S rRNA | tRNA-Glu | tRNA-Ile | tRNA-Ile | tRNA-Glu | tRNA-Glu | |||
tRNA-Ile | 16S rRNA | 23S rRNA | tRNA-Ala | tRNA-Ala | 23S rRNA | 23S rRNA | ||||
16S rRNA | 5S rRNA | 23S rRNA | 23S rRNA | |||||||
5S rRNA | ||||||||||
Bacterial strain | Enterobacter asburiae (sensu stricto) L1 | |||||||||
i6 contig# | 3 comp | 2 comp | 1 comp | 4 | 7 | 5 comp | 9 comp | 10 | 6 | 3 comp |
Aligned 5'-end position on L1 (nt) | 0 | 482989 | 1332744 | 3039704 | 3527433 | 3673798 | 3956299 | 4060595 | 4147496 | 4348070 |
Aligned 3'-end position on L1 (nt) | 478726 | 1332827 | 3034691 | 3522308 | 3668651 | 3951379 | 4055451 | 4142585 | 4343261 | 4561905 |
Gap to right next contig (nt) | 4263 | -83 | 5013 | 5125 | 5147 | 4920 | 5144 | 4911 | 4809 | |
Gene assigned at gap right next contig | 16S rRNA | 23S rRNA | 23S rRNA | 5S rRNA | 16S rRNA | 16S rRNA | 16S rRNA | 16S rRNA | ||
tRNA-Glu | tRNA-Ala | tRNA-Glu | 23S rRNA | tRNA-Glu | tRNA-Ile | tRNA-Ile | tRNA-Glu | |||
23S rRNA | tRNA-Ile | 16S rRNA | 16S rRNA | 23S rRNA | tRNA-Ala | tRNA-Ala | 23S rRNA | |||
16S rRNA | 5S rRNA | 23S rRNA | 23S rRNA | |||||||
5S rRNA | ||||||||||
Bacterial strain | Enterobacter asburiae (sensu stricto) RHBSTW-01009 | |||||||||
i6 contig# | 5 | 7 comp | 4 comp | 1 | 2 | 3 | 6 comp | 10 comp | 9 | 5 |
Aligned 5'-end position on RHBSTW-01009 (nt) | 0 | 252913 | 396771 | 889563 | 2497134 | 3403492 | 4148822 | 4353708 | 4440814 | 4541619 |
Aligned 3'-end position on RHBSTW-01009 (nt) | 248012 | 391646 | 884543 | 2495763 | 3399229 | 4144010 | 4348790 | 4435670 | 4536700 | 4586750 |
Gap to right next contig (nt) | 4901 | 5125 | 5020 | 1371 | 4263 | 4812 | 4918 | 5144 | 4919 | |
Gene assigned at gap right next contig | 16S rRNA | 16S rRNA | 16S rRNA | Lrp/AsnC family transcriptional regulator | 23S rRNA | 23S rRNA | 23S rRNA | 5S rRNA | 5S rRNA | |
23S rRNA | tRNA-Glu | tRNA-Ile | DMT family transporter | tRNA-Glu | tRNA-Glu | tRNA-Ala | 23S rRNA | 23S rRNA | ||
23S rRNA | tRNA-Ala | 16S rRNA | 16S rRNA | tRNA-Ile | tRNA-Ala | tRNA-Glu | ||||
23S rRNA | 16S rRNA | tRNA-Ile | 16S rRNA | |||||||
16S rRNA |
comp: complementary.
Possible genes involved in plant growth promoting traits in the E. asburiae i6 genome
Although strain i6 was not originally isolated as PGPB, a list of candidate genes that may contribute to plant growth was compiled from the genome sequence of strain i6 (Table (Table5).5). Soil beneficial bacteria can promote plant growth by synthesizing molecules similar to plant hormones 26, 27. Auxin like indole acetic acid (IAA) is quantitatively the most plant hormones secreted by Phytophthora rhizobacteria 28, 29, suggesting that auxin is a signaling molecule in microorganisms 30. Similar to the genome features previously reported for Enterobacter sp. J49 30, genes responsible for the synthesis of indole-pyruvate decarboxylase and indole-3-acetaldehyde dehydrogenase were detected in the i6 genome, and no other IAA pathway-related genes were detected. The bacterial volatiles 2,3-butanediol and acetoin are also known to induce plant growth promotion 31. Acetolactate synthase BudB converts pyruvate to acetolactate, which is subsequently converted to acetoin by acetoin decarboxylase BudA. The (S)-acetoin-forming diacetyl reductase BudC catalyzes the conversion of acetoin to 2,3-butanediol, which is reversible. All genes for butA, butB and butC were found, as well as other genes encoding acetolactate synthases (ilvB, ilvN_2, ilvG etc.). Similar to the J49 genome 30, the gcd gene encoding GDH synthesis, responsible for the production of gluconic acid, the major organic acid in the phosphate solubilization mechanism most widely used by soil bacteria, was detected in the i6 genome, but the pqq gene cluster, required for the biosynthesis of the PQQ cofactor, was not detected. Redundant genes for siderophore-production, iron ABC transporters, and type VI secretion systems which may compete with (plant) pathogens for low iron levels and cell growth, were also found in the i6 genome (data not shown).
Table 5
Genes in the genome of E. asburiae i6 strain that may contribute to plant growth promotion.
gene | locus_tag | gene product | function |
---|---|---|---|
ipdC | EAI6_22530 | indolepyruvate decarboxylase | phytohormone synthesis |
iaaH | EAI6_10770 | Indole-3-acetyl-aspartic acid hydrolase | indole acetic acid (IAA) synthesis |
budA | EAI6_03130 | acetolactate decarboxylase | acetoin synthesis |
budB | EAI6_03140 | acetolactate synthase AlsS | acetoin synthesis |
budC | EAI6_03150 | (S)-acetoin forming diacetyl reductase | 2,3-butanediol synthesis |
- | EAI6_31530 | acetolactate synthase small subunit | acetoin synthesis |
- | EAI6_31540 | acetolactate synthase 3 large subunit | acetoin synthesis |
ilvB | EAI6_35410 | acetolactate synthase large subunit | acetoin synthesis |
ilvN_2 | EAI6_35420 | acetolactate synthase small subunit | acetoin synthesis |
ilvG | EAI6_42550 | acetolactate synthase 2 catalytic subunit | acetoin synthesis |
ilvM | EAI6_42560 | acetolactate synthase 2 small subunit | acetoin synthesis |
gcd | EAI6_31050 | quinoprotein glucose dehydrogenase | gluconic acid synthesis |
Genes involved in restriction and anti-restriction in the E. asburiae i6 genome
Interestingly, KlcA1 and KlcA2, two homologues of KlcA, which have been reported to function as an anti-type I restriction modification (RM) system in other species 32, were predicted on this genome and plasmid respectively, but only one type IV R enzyme was predicted to be encoded and no type I RM system (Table (Table6).6). This is in contrast to other E. asburiae strains ATCC 35953, FDAARGOS_892, and RHBSTW-01009, which encode two type I RM systems, one type IV restriction enzyme, and KlcA1 (Table (Table6).6). L1 also encodes a type I RM system, a type IV R enzyme, and other restriction systems, but no KlcA homologs. Because it is important for successful genetic manipulation to suppress restriction enzymes and allow bacteria to introduce foreign plasmids, the transformation efficiency of several plasmid DNAs was tested by electroporation using the i6 and ATCC 35953 strains (Fig. (Fig.11).
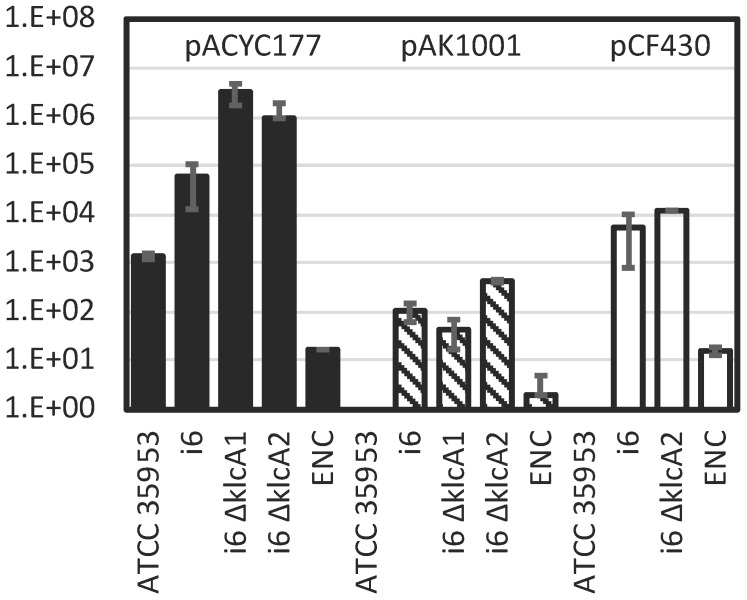
Transformation efficiency of E. asburiae strains i6, i6 klcA1 (AK1603), i6
klcA2 (AK1604), and ATCC 35953 (NBRC 109912 T) and E. cloacae ATCC 1304 (ENC) strain with pACYC177 (dark bar), pAK1001 (gray bar), and pCF430 (white bar). Transformation efficiency (colony count/µg plasmid DNA) was calculated by counting colonies after electroporation, recovery in SOB for 1 h, and overnight growth at 37°C on LB (Lennox) plates containing kanamycin (pACYC177 and pAK1001) or tetracycline (pCF430).
Table 6
Highly identical (>90%) ortholog list of restriction enzymes and antirestriction proteins among E. asburiae strains.
Enterobacter asburiae (sensu stricto) strain | |||||
---|---|---|---|---|---|
i6 | L1 | ATCC 35953 | FDAARGOS_892 | RHBSTW-01009 | |
gene product | locus_tag | ||||
antirestriction protein (KlcA1) | EAI6_00720 | ND | ACJ69_22495 | I6G49_09840 | HV349_19355 |
antirestriction protein (KlcA2) | EAI6_40610 | ND | ND | ND | ND |
type IV restriction enzyme | EAI6_32920 | ND | ND | ND | ND |
type IV restriction enzyme | ND | ND | ACJ69_09575 | I6G49_13575 | ND |
PD-(D/E)XK nuclease superfamily protein | ND | ND | ACJ69_09580 | I6G49_13570 | ND |
type I restriction enzyme, R subunit | ND | ND | ACJ69_09595 | I6G49_13555 | HV349_13365 |
type I restriction enzyme, S subunit | ND | ND | ACJ69_09610 | I6G49_13540 | HV349_13375* |
type I restriction enzyme M protein | ND | ND | ACJ69_09615 | I6G49_13535 | HV349_13390 |
type II restriction enzyme M protein | ND | ND | ACJ69_21260 | I6G49_08600 | HV349_10105/HV349_12830 |
type I restriction enzyme M protein | ND | ND | ACJ69_22055 | I6G49_09395 | ND |
type I restriction enzyme, S subunit | ND | ND | ACJ69_22060 | I6G49_09400 | ND |
type I restriction enzyme, R subunit | ND | ND | ACJ69_22070 | I6G49_09410 | ND |
type I restriction enzyme, R subunit | ND | ND | ND | ND | HV349_19450 |
type I restriction enzyme, S subunit | ND | ND | ND | ND | HV349_13375 |
type I restriction enzyme M protein | ND | ND | ND | ND | HV349_13380 |
5-methylcytosine-specific restriction enzyme B | ND | DI57_15815 | ND | ND | ND |
5-methylcytosine-specific restriction enzyme subunit McrC | ND | DI57_15820 | ND | ND | ND |
putative restriction endonuclease, HNH endonuclease | ND | DI57_15875 | ND | ND | ND |
restriction methylase | ND | DI57_15880 | ND | ND | ND |
Type IV restriction system protein | ND | DI57_15975 | ND | ND | ND |
type I restriction enzyme, R subunit | ND | DI57_15980 | ND | ND | ND |
type I restriction enzyme, S subunit | ND | DI57_15985 | ND | ND | ND |
type I restriction enzyme M protein | ND | DI57_15990 | ND | ND | ND |
ND: not detected. *Identity was less than 90%. Type I restriction enzyme, R subunit was shown in bold.
The results showed that the ATCC 35953 strain could not be transformed by the relatively large plasmid DNAs pCF430 (10 kb) 33 and pAK1001 (8.4 kb) 34, whereas i6 formed colonies, showing transformation efficiency at least four orders of magnitude higher than that of ATCC 35953 especially with pCF430 (Fig. (Fig.1).1). Using a relatively small plasmid DNA, pACYC177 (3.9 kb) 35, the ATCC 35953 strain also formed colonies on LB plates containing kanamycin, and the transformation efficiency of i6 was two orders of magnitude higher than that of ATCC 35953 (Fig. (Fig.11).
Application of the one-step gene inactivation method to the i6 strain
Unlike the ATCC 35953 strain, the i6 strain was able to accept foreign DNA with high efficiency (Fig. (Fig.1),1), so I attempted to apply the one-step gene inactivation method 36. Prior to this, RSFRedTER-Sp was constructed by replacing the CmR marker of the RSFRedTER plasmid 18, which can express λ Red recombinase, with the SpR marker, so that the CmR marker could be used in addition to the KmR and TcR markers in the i6 strain. (Ap-resistant pKD46 was not used because two β-lactamase genes were predicted in the i6 genome.) Gene deletions in klcA1, klcA2, and dgeC were constructed in the i6 strain expressing λ Red recombinase from RSFRedTER-Sp using TcR, CmR, and KmR markers, respectively. Similarly, a deletion in rcsB and a KmR insertion behind wzc were made in the i6 strain using CmR and KmR markers, respectively. The recombinants of interest grew with high colony formation rates (Table (Table7).7). Colony PCR in the junction region of the recombination site confirmed that the desired recombinants were constructed with high accuracy (Table (Table7).7). The klcA1 and
klcA2 strains were included in the transformation efficiency analysis because KlcA was originally reported as a restriction enzyme inhibitor in other bacteria, such as E. coli and Klebsiella pneumoniae
32, and may have contributed to the high transformation efficiency of the plasmid DNA of strain i6. However, contrary to expectations, these deletions increased rather than decreased the transformation efficiency of some plasmid DNA (Fig. (Fig.1).1). Yet, this result may have been confounded by the fact that the type I RM system, a potential target of KlcAs, is not encoded in the i6 genome. In conclusion, this report successfully applied the one-step gene inactivation method to the newly identified E. asburiae strain i6, whose transformation efficiency was much higher than that of ATCC 35953.
Table 7
Recombination efficiency and accuracy for deletion and insertion of targeted genes in the i6 strain
Target gene | Chromosome or plasmid | Deletion or insertion | Marker | Number of recombinants | Targeting success rate (%) |
---|---|---|---|---|---|
klcA1 | chromosome | deletion | TcR | 289 | 100 (8/8) |
klcA2 | plasmid | deletion | CmR | 143 | 100 (8/8) |
dgeC | chromosome | deletion | KmR | 48 | 100 (8/8) |
wzc | chromosome | insertion | KmR | 6 | 100 (6/6) |
Among the ECC, E. cloacae and E. hormaechei are the most frequently isolated species in clinical infections, especially in immunocompromised patients and those admitted to intensive care units 37, whereas E. asburiae had lower survival rates against serum than E. cloacae, E. hormaechei, and E. ludwigii isolates 4. In this report, the E. asburiae i6 genome unexpectedly exhibited most, if not all, of the genetic features of PGPB previously reported in Enterobater sp. J49. This was also true for the genome closest to i6, ATCC 35953, FDAARGOS_892, L1, RHBSTW-01009 (data not shown). Thus, E. asburiae is a preferred PGPB candidate in the ECC. Even if the current form of the i6 strain is not optimal in promoting plant growth, it would be advantageous and useful as a starting material for testing and fine-tuning any beneficial and detrimental traits. This is because the i6 strain, with all its potential as a PGPB, can be successfully genetically engineered. First of all, potential risk factors such as genes responsible for antibiotic resistance, biofilm formation, and some of the type VI secretion apparatus/effectors, etc. should be deleted by the one-step gene disruption method or its derivative, scarless genome editing 38. Such a risk negative i6 derivative strain could then be chemically mutagenized to express PGPB factors at optimal levels. Alternatively, or in addition, the ability of strain i6 to be a PGPB can be further enhanced by incorporating plasmid clones harboring other PGPB factors, even from different species, but only as genetically modified organisms. On the other hand, the amenability of this strain must also be useful to identify the relevant genes by deleting candidate genes from this genome that could produce a signal molecule that activates RcsB in Salmonella or even within the i6 strains. The recently developed conjugation-mediated versatile site-specific single-copy luciferase fusion system 39, which is broadly applicable to Gram-negative bacteria, should also be helpful in detecting intercellular interactions between the i6 strain and Salmonella, etc., and even within the i6 strains.
Nucleotide Sequence Accession Number
The draft genome sequence of E. asburiae i6 has been deposited in the DDBJ/EMBL/GenBank databases under accession numbers BTPF01000001 to BTPF01000028. The raw sequence reads were deposited in DDBJ under BioProject number PRJDB16388 and BioSample number SAMD00634955.
Acknowledgments
I thank Dr. Yoshihiko Hara for providing the plasmid RSFRedTER.
References
Articles from Journal of Genomics are provided here courtesy of Ivyspring International Publisher
Full text links
Read article at publisher's site: https://doi.org/10.7150/jgen.91337
Read article for free, from open access legal sources, via Unpaywall:
https://www.jgenomics.com/v12p0026.pdf
Data
Data behind the article
This data has been text mined from the article, or deposited into data resources.
BioStudies: supplemental material and supporting data
BioProject
- (2 citations) BioProject - PRJDB16388
Nucleotide Sequences (2)
- (1 citation) ENA - UBA11899
- (1 citation) ENA - DRR495940
Similar Articles
To arrive at the top five similar articles we use a word-weighted algorithm to compare words from the Title and Abstract of each citation.
High-quality genome sequence of human pathogen Enterobacter asburiae type strain 1497-78T.
J Glob Antimicrob Resist, 8:104-105, 07 Jan 2017
Cited by: 10 articles | PMID: 28082144
Enterobacter asburiae strain L1: complete genome and whole genome optical mapping analysis of a quorum sensing bacterium.
Sensors (Basel), 14(8):13913-13924, 30 Jul 2014
Cited by: 15 articles | PMID: 25196111 | PMCID: PMC4178997
Draft Genome Sequence of Enterobacter asburiae NCR1, a Plant Growth-Promoting Rhizobacterium Isolated from a Cadmium-Contaminated Environment.
Microbiol Resour Announc, 10(35):e0047821, 02 Sep 2021
Cited by: 2 articles | PMID: 34472973 | PMCID: PMC8411915
Nematicidal and Plant Growth-Promoting Activity of Enterobacter asburiae HK169: Genome Analysis Provides Insight into Its Biological Activities.
J Microbiol Biotechnol, 28(6):968-975, 01 Jun 2018
Cited by: 9 articles | PMID: 29642290