Abstract
Free full text

Serum uromodulin associates with kidney function and outcome in a cohort of hospitalised COVID-19 patients
Abstract
This study investigates the prevalence and evaluates the prognostic implications of acute kidney injury (AKI) in COVID-19 patients, with a novel emphasis on the evaluation of serum uromodulin (sUmod) as a potential kidney-specific biomarker. A cohort of hospitalised COVID-19 patients (n=
378) was examined for AKI using standard criteria. In addition to traditional urinary biomarkers, sUmod levels were analysed. Univariable and multivariable regression models were employed to evaluate the association of sUmod and AKI and in-hospital mortality. Levels of sUmod were significantly lower in patients with CKD (91.8
±
60.7 ng/ml) compared to patients with normal kidney function (204.7
±
91.7 ng/ml; p
<
0.001). 151
patients (40.0%) presented with AKI at the time of hospital admission or developed an AKI during hospitalization. 116
patients (76.8%) had an AKI already at the time of hospital admission. COVID-19 patients with AKI had significantly lower levels of sUmod compared to patients without AKI during hospitalisation (124.8
±
79.5 ng/ml) vs 214.6
±
92.3 ng/ml; p
<
0.001). The in-hospital mortality rate in this cohort of COVID-19 patients was 15.3%. Patients with AKI had a higher probability for in-hospital death (OR 5.6, CI
1.76
to
17.881, p
=
0.004). Patients who died during hospital stay, had significantly lower sUmod levels (129.14
±
89.56 ng/ml) compared to patients surviving hospitalisation (187.71
±
96,64 ng/ml; p
<
0.001). AKI is frequently associated with COVID-19 in hospitalized patients. Serum uromodulin may emerge as a promising biomarker for AKI in COVID-19 patients. Further research is warranted to explore its clinical application and refine risk stratification in this patient population.
Supplementary Information
The online version contains supplementary material available at 10.1038/s41598-024-76372-3.
Introduction
Severe acute respiratory syndrome coronavirus 2 (SARS-CoV-2) causing the Coronavirus Disease 2019 (COVID-19)1–3 has infected over 771,191,203 people (as of 12 October 2023) and 6,961,014 of these patients died due to COVID-19. A complex range of pathophysiological mechanisms including pulmonary and endothelial inflammation, thrombotic events and tissue fibrosis lead to lung damage, respiratory failure and hypoxia4. Although SARS-CoV-2 primarily attacks the respiratory system, severe complications of other organ systems including the kidneys can occur and are associated with an increase of adverse outcomes5,6.
Besides the respiratory tract, the kidney is one mayor organ targeted by SARS-CoV-25,7–9. The virus directly colonises, infects and disrupts kidney tissue10,11. It preferentially attacks tubular epithelial cells, but damage of glomerular and vascular structures is also documented8,11–13. In addition, multiple factors that are associated with SARS-CoV-2, such as impaired kidney perfusion and oxygenation, exposure to nephrotoxic agents as well as local cytokine production also harm kidney tissue integrity9,14,15. Consequently, critically ill or hospitalised patients develop acute kidney injury (AKI) in 28–90%5,16,17, which is associated with an increased risk of fatal outcomes18,19. Mortality is increased in patients with preexisting chronic kidney disease (CKD) and those suffering from AKI who require kidney replacement therapy20. This highlights the need for early diagnosis of COVID-19-associated kidney disease and early awareness of kidney complications.
Traditional laboratory markers of kidney function, i.e. serum creatinine and cystatin C, are not linked to defined kidney structures, lack specificity and sensitivity, and are influenced by gender, muscle mass, nutrition and other factors21–23. The widely used estimated glomerular filtration rate (eGFR) depends on the quality of analytical methods applied to assess serum creatinine concentrations and varies due to differing equation methods22,24. Porrini and colleagues even argued that these variations are not based on equation methods but rather on the general use of creatinine and cystatin C as markers of kidney function22. We hypothesize that analysing the kidney tissue marker uromodulin may offer a solution to these limitations.
Uromodulin is a specific defensin produced exclusively by the kidney in order to act as a regulator of ion transport, immunomodulator and protector against oxidative stress, urinary infections and kidney stones25–31. Produced by epithelial cells of the thick ascending limb of Henle’s loop (TAL), the synthesized glycoprotein is mainly excreted into the urine representing the most abundant urinary protein under physiological conditions31. Uromodulin is also transferred into the blood via a second basolateral transport pathway32–34. Cells of the medullary TAL segment are most vulnerable to limited oxygen supply, ischemia–reperfusion injury, as well as various toxins. Damage to these TAL cells mainly leads to apoptosis and necrosis, ultimately resulting in AKI35–39. Uromodulin circulating in human serum (sUmod) was shown to be a novel biomarker closely linked to the structural integrity of the distal nephron as well as to global kidney function34,40. In contrast to the molecule excreted in urine, sUmod is a remarkably stable antigen and exhibits various analytical advantages34,41,42. Receiver operator characteristics revealed that sUmod is a more sensitive marker for kidney function compared to the commonly used creatinine and cystatin C, allowing earlier detection of functional and structural injury43. Thus, sUmod can be defined as a true non-invasive “kidney damage marker”34,40.
To evaluate whether sUmod may present as a biomarker that allows early diagnosis of COVID-19-associated kidney diseases and an associated fatal outcome, we systematically evaluated sUmod in a cohort of 378patients with SARS-CoV-2 infection.
Material and methods
Patient population and data collection
From July 2020 to June 2021, serum specimens were collected from 378patients with SARS-CoV-2 infection treated in the normal ward or intermediate care of the Department of Infectious Diseases and Tropical Medicine at the St. Georg Hospital, Leipzig. All patients gave written informed consent. The research project complies with the Helsinki declaration. The Ethics Committee of the German-Saxonian Board of Physicians, Dresden, Germany (number, EK-BR-88/20–3) provided ethical clearance. Eligible patients were
≥
18 years of age with PCR-confirmed SARS-CoV-2 infection. The patients were staged according to the 8-point WHO Clinical Progression Scale and followed-up until recovery, hospital discharge, or death. At the timepoint of admission and during hospitalisation, follow-up laboratory parameters including kidney function and albuminuria were determined. Clinical information and the course of disease were derived from the medical history of each patient. Comorbidities were extracted from the diagnosis-related group (DRG) coding data of the controlling department. Acute kidney injury (AKI) was graded using the Acute Kidney Injury Network (AKIN) criteria44. Chronic Kidney disease (CKD) was defined by persistent eGFR
<
60 ml/min/1.73
m2 (CKD-EPI).
Processing of serum specimens
Blood was drawn by venipuncture within 48 h of hospital admission. Whole blood samples were immediately sent to Institute of Laboratory Medicine of the hospital for further routine diagnostics (see Supplementary Table S1). Regarding serum uromodulin (sUmod), serum aliquots of the supernatants were stored at -80 °C, until analyses.
Assessment of serum uromodulin concentrations
Serum uromodulin concentrations were measured in serum samples using a sensitive uromodulin ELISA, certified for serum analyses (EUROIMMUN Medizinische Labordiagnostika AG, Luebeck, Germany) and based on monoclonal antibodies as reported earlier34,43.
Statistics
For description of the data, means, standard deviations (SD) median, 1st quartile (Q1) and 3rd quartile (Q3), maximum and minimum as well as sample size (N) were calculated for continuous variables (as e.g., age, sUmod, Albumin) and numbers and percentages were calculated for categorical variables (as e.g., gender, co-morbidities). Descriptive statistics were calculated overall patients as well as separately for the patients with and without AKI and patients surviving or dying during hospital stay. The main goal of the study was to evaluate the association of sUmod (in ng/ml) and the occurrence of AKI. The association of several baseline parameters with the probability of AKI was investigated. First, univariable logistic regression models for each possible influence factor were calculated. Furthermore, a multivariable logistic regression model (with backward selection, excluding variables from the model with a p-value greater than 0.05) was performed accounting for all parameters being significant in the univariable analyses (p<
0.05). Although more parameters were observed, the selection of potential influence factors for the multivariable model was restricted to the above listed 15 to avoid multi-collinearity or sample size reduction due to missing values. Furthermore, only co-morbidities observed more than 20
times within the cohort were considered for statistical testing.
Additionally, for sUmod and serum creatinine, a receiver operating characteristic curve (ROC-curve) as well as the AUC value with 95% confidence intervals were calculated. Similar analyses were performed to assess the influence of several parameters on the probability of in-hospital death. Note that for in-hospital death, the variables malignoma history (yes vs. no), chronic obstructive pulmonary disease (yes vs. no), chronic neurological conditions (yes vs.no), urinary total protein concentration, procalcitonin and lactate dehydrogenase (LDH) were additionally considered for the multivariable model selection. To evaluate the association between sUmod and the other measured parameters, Pearson correlation coefficients were calculated.
All analyses were performed using R, release 4.2.2 or SAS, release 9.4. All p-values smaller than 0.05 were considered as statistically significant. Due to the explorative character of the study, no correction for multiplicity was performed.
Results
Clinical baseline characteristics of COVID-19 patients
Overall, specimens of 378 patients were evaluated at admission to hospital, including those from 167women (44.2%) and 211
men (55.8%). The mean age of the patients was 69.1 (±
16.1) years, ranging from 18 to 97 years. The dataset included 132
patients (34.9%) with diabetes mellitus, 17
patients (4.5%) with arterial hypertension, 87
patients (23.0%) with chronic kidney disease, 34
patients (8.99%) with chronic obstructive pulmonary disease (COPD) and 16
patients (4.23%) with undergoing kidney replacement therapy (KRT). Baseline patient characteristics and comorbidities are listed in Table Table1.1. For a subset of 257
patients, information on current medication was available. 199 of 257
(77.4%) received at least one antihypertensive medication including 169
patients (65.5%) that were either treated with ACE-inhibitors (ACE I) or angiotensin receptor blockers (further pharmaceutical treatments are listed in Supplementary Table S2). Twelve patients (3.2%) received immunosuppressive therapy of any kind.
Table 1
Descriptive statistics are shown as numbers and percent (%) for categorical variables and median (Interquartile-Range) for continuous variables for all patients (overall) as well as separately for patients with and without acute kidney injury (AKI).
Variable | Overall | No AKI (n![]() ![]() | AKI (n![]() ![]() | ||||
---|---|---|---|---|---|---|---|
Value | n | Value | n | Value | n | ||
Sex | F | 44.18% | 167 | 65.27% | 109 | 34.73% | 58 |
M | 55.82% | 211 | 55.92% | 118 | 44.08% | 93 | |
Age | 73 (59–82) | 378 | 66 (54–79) | 227 | 79 (69–84) | 151 | |
Invasive ventilation | No | 87.53% | 330 | 65.76% | 217 | 34.24% | 113 |
Yes | 12.47% | 47 | 19.15% | 9 | 80.85% | 38 | |
WHO-score at admission | 1 | 0.26% | 1 | 100.00% | 1 | 0.00% | 0 |
2 | 1.06% | 4 | 100.00% | 4 | 0.00% | 0 | |
3 | 19.58% | 74 | 64.86% | 48 | 35.14% | 26 | |
4 | 47.35% | 179 | 53.63% | 96 | 46.37% | 83 | |
5 | 28.04% | 106 | 61.32% | 65 | 38.68% | 41 | |
6 | 3.70% | 14 | 92.86% | 13 | 7.14% | 1 | |
In-hospital death | No | 84.66% | 320 | 91.15% | 103 | 81.87% | 217 |
Yes | 15.34% | 58 | 8.85% | 10 | 18.11% | 48 | |
Co-morbidities | |||||||
Arterial hypertension | No | 95.49% | 360 | 60.56% | 218 | 39.44% | 142 |
Yes | 4.51% | 17 | 52.94% | 9 | 47.06% | 8 | |
Diabetes mellitus | No | 65.08% | 246 | 69.92% | 172 | 30.08% | 74 |
Yes | 34.92% | 132 | 41.67% | 55 | 58.33% | 77 | |
COPD | No | 91.01% | 344 | 61.92% | 213 | 38.08% | 131 |
Yes | 8.99% | 34 | 41.18% | 14 | 58.82% | 20 | |
History of malignoma | No | 90.74% | 343 | 61.52% | 211 | 38.48% | 132 |
Yes | 9.26% | 35 | 45.71% | 16 | 54.29% | 19 | |
CKD | No | 76.98% | 291 | 69.07% | 201 | 30.93% | 90 |
Yes | 23.02% | 57 | 29.89% | 26 | 70.11% | 61 | |
Renal replacement therapy | No | 95.77% | 362 | 62.15% | 225 | 37.85% | 137 |
Yes | 4.23% | 16 | 12.50% | 2* | 87.50% | 14# | |
Immunocompromised | No | 96.82% | 365 | 60.55% | 221 | 39.45% | 144 |
Yes | 3.18% | 12 | 50.00% | 6 | 50.00% | 6 | |
Asthma | No | 96.03% | 363 | 59.78% | 217 | 40.22% | 146 |
Yes | 3.97% | 15 | 66.67% | 10 | 33.33% | 5 | |
Organ transplantation | No | 99.73% | 376 | 60.37% | 227 | 39.63% | 149 |
Yes | 0.27% | 1 | 0.00% | 0 | 100.00% | 1 | |
Variables at baseline | |||||||
sUmod (ng/ml) | 166.5 (95–251) | 378 | 202 (147.5–288.5) | 127 | 103 (62–168) | 151 | |
eGFR (CKD-EPI; ml/min/1,73m2) | 67 (46–87) | 377 | 82 (66.5–96.5) | 227 | 43 (31–58) | 150 | |
UACR (g/g creatinine) | 0.06 (0.02–0.17) | 291 | 0.04 (0.01–0.1) | 175 | 0.11 (0.03–0.23) | 116 | |
CRP (mg/l) | 64.6 (26.75–104.45) | 375 | 54.25 (20.45–91.82) | 124 | 69.7 (34.15–130.15) | 151 | |
IL-6 (ng/l) | 37.25 (18.52–80.97) | 330 | 31.1 (13.9–55.4) | 189 | 54.1 (26.5–127) | 141 | |
Leukocytes (Gpt/l) | 6.3 (4.5–8.1) | 378 | 6.1 (4.4–7.7) | 227 | 6.6 (4.75–8.9) | 151 | |
Serum urea (mmol/l) | 6.5 (4.6–10.2) | 377 | 5.2 (3.8–6.77) | 226 | 10.5 (7.05–15.55) | 151 | |
Serum albumin (g/l) | 35.7 (31.9–38.9) | 341 | 36.45 (33.98–39.52) | 204 | 34.3 (30.6–37.6) | 137 | |
Urinary albumin (g/l) | 0.05 (0.02–0.15) | 305 | 0.03 (0.01–0.09) | 186 | 0.1 (0.03 -0.27) | 119 | |
Serum creatinine (µmol/l) | 90 (72–113) | 378 | 78 (64.5–90.5) | 227 | 124 (103–160) | 151 | |
PCT (µg/l) | 0.12 (0.07–0.28) | 350 | 0.09 (0.06–0.14) | 207 | 0.22 (0.12–0.53) | 143 | |
LDH (µmol/l*s) | 5.6 (4.3–7.3) | 366 | 5.6 (4.45–7.25) | 219 | 5.8 (4.3–7.3) | 147 | |
Serum protein (g/l) | 63.6 (59.7–68.1) | 349 | 64.5 (60.4–68.2) | 206 | 62.75 (59.08–67.25) | 144 | |
Urinary protein (mg/l) | 239 (130.25–482) | 298 | 212 (116.5–388) | 179 | 299 (159–688.5) | 119 |
CKD: chronic kidney disease, COPD: chronic obstructive pulmonary disease, CRP: C-reactive protein, IL-6: Interleukin-6, LDH: lactate dehydrogenase, PCT: procalcitonin sUmod: serum uromodulin, UACR: urine albumin-creatinine ratio. * chronic dialysis patients. # acute renal replacement therapy in AKI patients (3 of 14 with preexisting CKD, 11/14 had no CKD at baseline).
74patients (19.6%) were hospitalised without initial need for oxygen supply (WHO score 3), 179
patients (47.4%) needed oxygen supply (WHO score 4), 106
patients (28.0%) were in need of non-invasive ventilation or high-flow oxygen therapy (WHO score 5) and 14
patients (3.7%) were initially admitted to intermediate care but had to be intubated and invasively ventilated shortly after admission (WHO score 6). The average duration of hospitalisation was 13.1 (±
11.3)
days. 5 patients (1,3%) remained as outpatients.
Laboratory parameters at baseline
Laboratory parameters of the COVID-19 cohort in serum and urine are summarised in Table S1. At baseline serum creatinine levels were 107.2±
69.5 µmol/l with mean eGFR (CKD-EPI) of 66.9
±
27.5 ml/min/1.73
m2, and a mean value of serum uromodulin of 178.7 (±
97.8)
ng/ml. Serum uromodulin levels strongly correlated with eGFR (correlation coefficient 0.585, CI 0.514
to
0.648, p
<
0.001). Furthermore, sUmod levels were significantly lower in patients with CKD (91.8
±
60.7 ng/ml) compared to patients with normal kidney function (204.7
±
91.7 ng/ml; p
<
0.001; Fig. 1A).
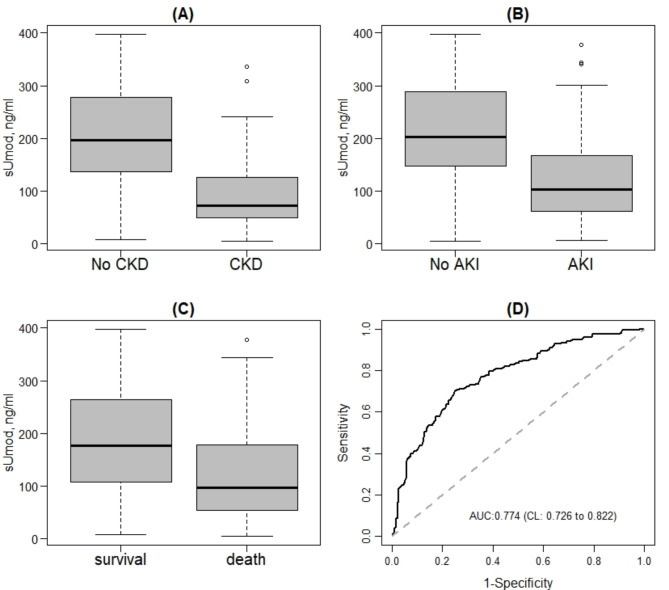
sUmod levels at admission in COVD-19 patients with and without chronic kidney disease (CKD) (A), with and without acute kidney injury (AKI) (B) and survival and death (C). ROC-Curve as well as corresponding AUC and confidence interval for the association between sUmod and AKI (D). sUMOD: serum uromodulin.
Association between uromodulin and acute kidney injury (AKI) in COVID-19 patients
In total, of the 378patients included in this study, 151
patients (40.0%) presented with an AKI at the time of hospital admission or developed an AKI during hospitalisation. Among these patients, 110
patients (72.8%) had AKI AKIN I, 22 (14.6%) had AKI AKIN II and 19
patients (12.6%) patients had AKI AKIN III. 16
patients (10.6%) eventually needed kidney replacement therapy. Of the 151
AKI patients, 116
patients (76.8%) had an AKI already at the time of hospital admission. The remaining 35
patients newly developed an AKI during their hospital stay, and in average 7.3
±
6.7 days after admission.
COVID-19 patients suffering from AKI showed significantly lower levels of sUmod compared to patients without kidney impairment during hospitalisation ((124.8±
79.5 ng/ml) vs 214.6
±
92.3 ng/ml; p
<
0.001; Fig. 1B). Univariable logistic regression models for the association between baseline variables and the occurrence of AKI found that sUmod at hospital admission was significantly associated with the occurrence of AKI (p
<
0.001; Table Table2).2). Patients with higher sUmod concentrations revealed a lower probability for AKI (OR 0.988, CI 0.985 to 0.991; Table Table2).2). These univariable results were confirmed by a multivariable logistic regression model with backward selection, which accounted for further potential confounding factors being significant in the univariable analyses (with p
<
0.05). Importantly, COVID-19 patients with higher sUmod values revealed a lower probability for AKI (OR 0.993, CI 0.993 to 0.989, Table Table2).2). The diagnostic ability of sUmod to predict AKI was evaluated by a ROC curve (Fig. 1D) and the corresponding area under curve (AUC; 0.774, CI 0.726 to 0.822).
Table 2
Odds Ratios (OR) and corresponding 95% Confidence Intervals (95% CI) as well as the p-value for the univariable models and multivariable models (with backward selection) for the probability for AKI.
Univariable models | Multivariable models | ||||
---|---|---|---|---|---|
Variable | Comparison | OR (95% CI) | p-value | OR (95% CI) | p-value |
Age | 1.055 (1.037–1.072) | ![]() ![]() | n.s | ||
Sex | M vs F | 1.481 (0.974–2.251) | 0.066 | n.i | |
Invasive ventilation | Yes vs. No | 8.108 (3.787–17.36) | ![]() ![]() | 5.784 (1.961–17.06) | 0.002 |
CKD | Yes vs. No | 5.240 (3.109–8.831) | ![]() ![]() | n.s | |
Diabetes | Yes vs. No | 3.254 (2.095–5.055) | ![]() ![]() | n.s | |
WHO-score at admission | 0.959 (0.746–1.232) | 0.742 | n.i | ||
sUmod (ng/ml) | 0.988 (0.985–0.991) | ![]() ![]() | 0.993 (0.989–0.997) | ![]() ![]() | |
Serum creatinine (µmol/l) | 1.045 (1.034–1.057) | ![]() ![]() | n.s | ||
Urinary albumin (g/l) | 1.854 (0.920–3.738) | 0.084 | n.i | ||
Serum albumin (g/l) | 0.914 (0.874–0.955) | ![]() ![]() | n.s | ||
Serum urea (mmol/l) | 1.467 (1.345–1.601) | ![]() ![]() | 1.323 (1.195–1.466) | ![]() ![]() | |
UACR (g/g creatinine) | 2.021 (0.961–4.249) | 0.064 | n.i | ||
CRP (mg/l) | 1.006 (1.003–1.009) | ![]() ![]() | n.s | ||
IL-6 (ng/l) | 1.012 (1.007–1.016) | ![]() ![]() | 1.007 (1.001–1.013) | 0.027 | |
Leukocytes (Gpt/l) | 1.100 (1.032–1.172) | 0.003 | n.s |
Note that n.s. indicates that the variable was not significant in the multivariable model with backward selection and was therefore excluded from the models. Variables with n.i. were not included in the multivariable model because they were not significant already in the univariable models. CKD: chronic kidney disease, CRP: C-reactive protein, IL-6: Interleukin-6, sUmod: serum uromodulin, UACR: urine albumin-creatinine ratio.
Besides sUmod, invasive ventilation, serum concentrations of IL-6 and serum urea showed significant associations with AKI (Table (Table2).2). Patients receiving invasive ventilation showed a much higher risk for AKI (OR 5.784, CI 1.961 to 17.06, p=
0.002). In more detail, 80.9% of patients receiving invasive ventilation had AKI. In contrast, AKI occurred in 34.2% of patients, who did not receive invasive ventilation. Patients with higher IL-6 and serum urea concentrations showed a higher risk to develop an AKI (OR 1.007, CI 1.001 to 1.013, p
=
0.027 and OR 1.323, CI 1.195 to 1.466, p
<
0.001, respectively. All other parameters did not remain significant after model selection.
Association between AKI and fatal outcome in COVID-19 patients
The in-hospital mortality rate in this cohort of COVID-19 patients was 15.3%. Among patients being dismissed alive, 6.9% died within 6 months. Out of the 47patients receiving invasive ventilation, 91.5% died during hospital stay, while out of the 330
patients who did not receive invasive ventilation, 4.6% died. Univariable logistic regression models for the association between baseline variables and the occurrence of in-hospital death revealed that AKI in COVID-19 patients was significantly associated with in-hospital death (p
<
0.001; Table Table3).3). Patients with AKI showed a higher probability for in-hospital death (OR 10.113, CI 4.92 to 20.785; Table Table3).3). These results from univariable regression models were confirmed by a multivariable logistic regression model with backward selection, which was performed for all parameters being significant in the univariable analyses (with a p
<
0.05). Patients with AKI had a higher probability for in-hospital death (OR 5.6, CI
1.76
to
17.881, p
=
0.004). In more detail, out of 151
patients presenting with AKI, 31.8% died during hospital stay. In contrast, out of the 227
patients not presenting with AKI merely 4.4% died.
Table 3
Odds Ratios (OR) and corresponding 95% Confidence Intervals (95% CI) as well as the p-value for the univariable models and multivariable models (with backward selection) for the probability for in-hospital death.
Univariable models | Multivariable models | ||||
---|---|---|---|---|---|
Variable | Comparison | OR (95% CI) | p-value | OR (95% CI) | p-value |
Age | 1.068 (1.039–1.097) | ![]() ![]() | 1.08 (1.025–1.139) | 0.004 | |
Sex | M vs F | 1.766 (0.978–3.189) | 0.059 | n.i | |
AKI | Yes vs. No | 10.113 (4.920–20.785) | ![]() ![]() | 5.6 (1.76–17.811) | 0.004 |
Invasive ventilation | Yes vs. No | 225.750 (71.623–711.547) | ![]() ![]() | 165.146 (45.142–604.165) | ![]() ![]() |
CKD | Yes vs. No | 4.129 (2.295–7.429) | ![]() ![]() | n.s | |
Diabetes | Yes vs. No | 1.938 (1.101–3.413) | 0.022 | n.s | |
Malignom History | Yes vs. No | 2.458 (1.111–5.440) | 0.026 | n.s | |
COPD | Yes vs. No | 1.203 (0.475–3.049) | 0.696 | n.i | |
WHO-score at admission | 1.337 (0.946–1.890) | 0.1 | n.i | ||
sUmod (ng/ml) | 0.993 (0.989–0.996) | ![]() ![]() | n.s | ||
Serum creatinine (µmol/l) | 1.010 (1.006–1.014) | ![]() ![]() | n.s | ||
Urinary albumin (g/l) | 2.312 (1.151–4.641) | 0.018 | n.s | ||
Serum albumin (g/l) | 0.884 (0.833–0.938) | ![]() ![]() | n.s | ||
Serum urea (mmol/l) | 1.134 (1.086–1.184) | ![]() ![]() | n.s | ||
Serum sodium (mmol/l) | 1.082 (1.017–1.150) | 0.013 | n.s | ||
UACR (g/g creatinine) | 1.850 (0.977–3.504) | 0.059 | n.i | ||
CRP (mg/l) | 1.009 (1.006–1.013) | ![]() ![]() | n.s | ||
IL-6 (ng/l) | 1.009 (1.005–1.013) | ![]() ![]() | n.s | ||
Leukocytes (Gpt/l) | 1.097 (1.022–1.176) | 0.01 | n.s | ||
Urinary protein (mg/l) | 1.001 (1.000–1.001) | 0.013 | n.s | ||
PCT (µg/l) | 1.101 (0.999–1.213) | 0.051 | n.i | ||
LDH (µmol/l*s) | 1.232 (1.109–1.368) | ![]() ![]() | 1.356 (1.107–1.661) | 0.003 |
Note that n.s. indicates that the variable was not significant in the multivariable model with backward selection and was therefore excluded from the models. Variables with n.i. were not included in the multivariable model because they were not significant already in the univariable models. AKI: acute kidney injury, CKD: chronic kidney disease, COPD: chronic obstructive pulmonary disease, CRP: C-reactive protein, IL-6: Interleukin-6, LDH: lactate dehydrogenase, PCT: procalcitonin, sUmod: serum uromodulin, UACR: urine albumin-creatinine ratio.
Association between serum uromodulin and fatal outcome in COVID-19 patients
Since sUmod concentrations at hospital admission of COVID-19 patients were significantly associated with the occurrence of AKI, the association between sUmod and in-hospital death was evaluated. Notably, COVID-19 patients who died during hospital stay, had significantly lower sUmod levels (129.14±
89.56 ng/ml) compared to patients surviving hospitalisation (187.71
±
96,64 ng/ml; p
<
0.001; Fig. 1C). Univariable logistic regression models for the association between baseline parameters and in-hospital death showed a significant association of sUmod and the probability of in-hospital death (p
<
0.001; Table Table3).3). Patients presenting with higher sUmod levels had a lower probability for death (OR 0.993, CI 0.989 to 0.996) compared to patients with lower sUmod concentrations. However, sUmod did not remain significant in multivariable logistic regression model with backward selection, which was performed for all parameters being significant in the univariable analyses (with a p
<
0.05).
Besides health-related sUmod levels, patients receiving invasive ventilation showed the largest risk of death (OR165.146, CI: 45.14 to 604.17). In more detail, out of 47
patients receiving invasive ventilation, 91.5% died during hospital stay, while out of 330
patients who did not receive invasive ventilation, merely 4.6% died. Increasing age as well as patients having higher LDH values also showed a significantly larger probability of death (OR age: 1.08, OR LDH: 1.356). All other parameters including serum creatinine did not remain significant after model selection (see Table Table33).
Correlation between serum uromodulin with other kidney markers
To evaluate how serum uromodulin correlates with laboratory routine parameters including other kidney markers such as serum creatinine and eGFR, Pearson correlation coefficients (CC) were determined (Supplementary Table S3). sUmod was negatively correlated with serum creatinine. Patients with higher sUmod values presented with lower serum creatinine concentrations (CC -0.344, CI -0.430 to -0.252, p<
0.001). In contrast, eGFR values positively correlated with sUmod (CC 0.585, 0.514 to 0.648, p
<
0.001). In addition, sUmod had a significant negative association with serum uric acid levels (CC -0.478, CI -0.585 to -0.354, p
<
0.001) and serum urea concentrations (CC -0.51, CI -0.581 to -0.431, p
<
0.001).
Discussion
While the most severe phases of the COVID-19 pandemic have subsided, there remains a necessity for early detection of complications related to COVID-19 and subsequent fatal outcomes45. Acute kidney injury is a common complication in COVID-19 patients. Here, we analysed a cohort of 378patients hospitalised with SARS-CoV-2 infection between August 2020 and May 2021 focusing on the circulating kidney-specific glycoprotein sUmod.
Prevalence of AKI in COVID-19 patients
AKI commonly occurs in individuals hospitalised with COVID-1916,46. Our findings align with and contribute to the evolving body of literature on kidney complication associated with SARS-CoV-2 infection. In the present study, 40% of the entire cohort of COVID-19 patients experienced AKI. Notably, recent meta-analyses reported lower AKI rates ranging from 8 to 28%17,47,48. Varying rates of AKI can be explained by the heterogeneity of COVID-19 cases. Variations in viral loads, host-related nephrotoxic susceptibility, undetected preexisting risk factors, a diverse “disease spectrum”, and the potential influence of autoimmunity and autoinflammation can collectively contribute to the observed variety in AKI rates among individuals with COVID-1949. Our study enrolled hospitalised patients, inherently encompassing a higher proportion of individuals with severe COVID-19 cases. This distinction in patient profiles, with a focus on hospitalised cases compared to outpatient clinic and intensive care unit (ICU) cohorts, could contribute to the observed differences in AKI prevalence rates. Another reason for the observed lower prevalence of AKI in other studies could be a potential underreporting of AKI. In accordance with our findings, an investigation in 3993 hospitalised COVID-19 patients revealed a prevalence of 46% for AKI46. Notably, the majority of AKI cases in our study were categorised as AKIN stage I (72.8%), deviating from prevalences of 42% and 44% documented in other reports17,46. Here, a potential source of error may also lie in diagnostic discrepancies between physicians and nephrological experts, as the missing cases likely correspond to AKIN stage I instances. Our study expands on existing knowledge by exploring the timing of AKI onset. We observed that almost three quarters of AKI cases were already present at the time of hospital admission. Nevertheless, a considerable portion of AKI cases (23.2%) developed during the hospital stay, with an average of 7.36 days post-admission. This temporal analysis adds granularity to the understanding of AKI progression in COVID-19 patients, offering insights into potential windows for preventive measures.
AKI as prognostic indicator for in-hospital mortality in COVID-19 patients
The association between AKI and outcomes in COVID-19 patients, including in-hospital mortality, has been a subject of significant interest16,18,19. In agreement with these studies, our investigation revealed a substantial impact of AKI on in-hospital mortality among COVID-19 patients. The in-hospital mortality rate in our AKI cohort was 15.3%, aligning with the reported mortality rates across various studies19. The dynamic interaction between the kidneys and the lungs during SARS-CoV-2 infections could potentially intensify during the occurrence of AKI50,51. The temporal aspect of mortality is noteworthy, as indicated by the 6.9% mortality rate within 6 months among patients dismissed alive. This mirrors concerns raised in recent reports about long-term complications and mortality in COVID-19 survivors52,53.
The uni- and multivariable regression models conducted in our study further affirm the association between AKI and in-hospital death. These results are in concordance with former studies that highlighted the prognostic significance of AKI in predicting adverse outcomes in COVID-19 patients7,16,18,45. The identified odd ratios in our study indicate a substantially higher risk of in-hospital death among COVID-19 patients with AKI compared to those without AKI. These findings reinforce the importance of recognising and managing AKI promptly in the clinical care of COVID-19 patients to potentially improve survival outcomes.
Uromodulin as prognostic indicator for AKI in COVID-19 patients
Previous studies have used mainly serum creatinine or cystatin C for recognising AKI in COVID-19 patients. These biomarkers are not directly related to kidney structure and reveal diagnostic uncertainties23,54. The specific examination of sUmod in the context of COVID-19 is less explored. It has been demonstrated that higher sUmod levels are protective against various diseases, while low concentration is associated with increased risks for chronic kidney failure, arterial hypertension, diabetes mellitus, and all-cause mortality43,55–61. Importantly, sUmod decreases early in chronic kidney failure before serum creatinine and cystatin C rise34,43. Additionally, sUmod has been considered a diagnostic tool for assessing ‘kidney mass’, a unique feature among other markers40. Our results underscore the potential of sUmod as a biomarker, supporting the need for further investigation into its early AKI detection and risk stratification. We present new data on kidney-specific uromodulin circulating in serum in hospitalized COVID-19 patients and the data confirm that injury of the medullary nephron is closely involved in COVID-19 kidney damage. A distinct benefit of sUmod is its association with very early stages of kidney injury, where sUmod levels already decline while serum creatinine and eGFR still remain within normal range34,43. It is crucial to acknowledge that a substantial portion of the study cohort already presented with AKI at hospital admission. This factor introduces a challenge in precisely evaluating sUmod’s advantage in detecting very early stages of kidney injury, as a considerable proportion of COVID-19 patients had pre-existing kidney abnormalities at the timepoint of hospital admission. Merely 23.2% of all AKI cases developed during hospital stay, limiting the evaluation of sUmod as predictive marker for AKI development of COVID-19 patients.
Uromodulin as indicator of fatal outcome
Building on the association with AKI, our study evaluated a potential link between sUmod levels and in-hospital mortality in COVID-19 patients. The significant difference in sUmod values between patients who survived hospitalisation and those who succumbed to the disease suggests that sUmod may not only be a marker for kidney impairment but also a potential prognostic indicator for fatal outcomes. Despite the strong univariable association, sUmod did not retain significance in the multivariable logistic regression model with backward selection. This highlights the intricate interplay of various factors influencing mortality in COVID-19 patients and emphasises the need for a comprehensive understanding of the underlying mechanisms. Our study identified patients receiving invasive ventilation as having the highest risk of mortality, with an odds ratio of 165.1 (CI: 45.1–604.2). The in-hospital mortality rate among these patients was 91.5%, highlighting the critical role of invasive ventilation as a significant predictor of fatal outcomes in severe cases of COVID-19. Increasing age and higher LDH values were also significantly associated with mortality, underscoring the impact of age-related and inflammatory factors in the risk of death in COVID-19 patients.
Advantages of sUmod as a diagnostic marker for AKI in the context of COVID-19
Our study presents insights into the pathological involvement of specific and vulnerable segments of the human nephron in COVID-19 related kidney injury. Epithelia of the TAL segment were shown to be highly susceptible towards hypoxia, various toxins, oxygen radicals, disturbance in haemodynamics etc., all common factors characteristic in a clinical setting of intensive care increasing the risk of an impaired synthesis of uromodulin (Heyman et al. 1991, 2010; Steubl et al., 2017, Thielemans et al. 2023). A distinctive aspect of our investigation is its pioneering role as the first study to assess sUmod in COVID-19 patients, shedding light on the substantial engagement of the medullary epithelia in virus-mediated kidney injury. Compared to conventional urinary biomarkers, sUmod emerges as a remarkably robust biomarker. This robustness is particularly noteworthy considering the challenges associated with urine processing, as highlighted by previous studies on the timing and stability of proteins/peptides42,62. Importantly, sUmod remains stable even under conditions such as water loading, distinguishing it from certain limitations associated with urine-based markers63. In contrast to other ‘kidney biomarkers’, sUmod stands out for its specificity to kidney tissue components. Our study contributes to the understanding that a broad ‘biomarker panel’, encompassing urinary proteins and proinflammatory interleukins such as NGAL, KIM-1, EGF, urinary uromodulin, and albumin, showed relatively low diagnostic relevance for assessing kidney damage in COVID-19 patients62. Furthermore, our investigation revealed a compelling correlation between sUmod and other markers, such as serum creatinine and cystatin C. Despite the lack of significance in the multivariable model to predict fatal outcome, the initial univariable association suggests sUmod may have potential implications for risk stratification and early intervention in COVID-19 patients. Clinicians should be attentive to the dynamic nature of biomarkers and their utility in predicting patient outcomes. However, its initial association warrants further exploration in larger cohorts or specific patient subgroups.
Limitations
While recognising the intricacies of our study, it is important to acknowledge certain limitations.
A significant portion of the study cohort presented with AKI at the timepoint of hospitalisation. This introduces challenges and bias in evaluating the advantages of sUmod in detecting very early stages of kidney injury and in assessing the potential of sUmod as predictive biomarker for AKI in COVID-19 patients.
The reported data need to be interpreted within the framework of an infectious disease department encompassing both a general and an intermediate care ward. Individuals in critical condition at the timepoint of hospital admission requiring interventions such as invasive ventilation or pressure support, were excluded from this study. This impacts the representation of severe cases in the study.
The overall sample size (n=
378) may influence the statistical power and generalisability of findings, particularly in subgroup analyses.
Conclusion
The prevalent occurrence of AKI in hospitalised COVID-19 patients underscores its clinical significance. sUmod, a kidney-specific protein and defensin, appears as a robust diagnostic tool for early AKI detection and risk assessment. Lower sUmod levels are significantly associated with the occurrence of AKI. Beyond its diagnostic benefits to mirror kidney health, sUmod may emerge as a general indicator for fatal outcomes in COVID-19 patients. While acknowledging the nuanced nature of AKI in COVID-19 patients, our study evaluates sUmod as a potential player in advancing diagnostic and prognostic precision. Integrating sUmod into clinical practice holds promise for more early therapeutic interventions and refined risk management in the dynamic landscape of COVID-19-related complications.
Electronic supplementary material
Below is the link to the electronic supplementary material.
Acknowledgements
The authors thank technical personnel of EUROIMMUN Medizinische Labordiagnostika AG, Department of Experimental Immunology, for supporting uromodulin analyses, which was funded by EUROIMMUN Medizinische Labordiagnostika AG, Luebeck.
Author contributions
RW, MM, AG and JS conceptualised the study. VH, TH, and MK did the uromoduline analyses. HJA and AG did the statistical analysis. RW, MM, AG, MK and JS wrote the manuscript, MM, SK, JB and CL were study investigators and participated in the conduct of the study, including recruitment, clinical care and follow-up of patients. NH was responsible for data retrieval from the clinical information system. All authors interpreted the results, commented on successive drafts of the manuscript and approved the final version.
Funding
EUROIMMUN bore the expenses for logistic costs and provided reagents and laboratory capacity for uromodulin analysis.
Data availability
The data that support the findings of this study are available on request from the corresponding author.
Declarations
VH, MK and TH are employees of EUROIMMUN Medizinische Labordiagnostika AG, a company that commercialises serological assays and co-owns a patent application related to immunoassays for the diagnosis of a SARS-CoV-2 infection. All other authors declare no conflict of interest related to the topic of this study.
Footnotes
Publisher’s note
Springer Nature remains neutral with regard to jurisdictional claims in published maps and institutional affiliations.
Contributed equally.
References
Articles from Scientific Reports are provided here courtesy of Nature Publishing Group
Similar Articles
To arrive at the top five similar articles we use a word-weighted algorithm to compare words from the Title and Abstract of each citation.
Serum Uromodulin Levels in Prediction of Acute Kidney Injury in the Early Phase of Acute Pancreatitis.
Molecules, 22(6):E988, 14 Jun 2017
Cited by: 11 articles | PMID: 28613246 | PMCID: PMC6152627
Lower uromodulin serum levels are associated with poorer prognosis in patients with cirrhosis and hepatorenal syndrome type 2.
Am J Physiol Gastrointest Liver Physiol, 326(5):G583-G590, 19 Mar 2024
Cited by: 0 articles | PMID: 38502914
Serum uromodulin-a marker of kidney function and renal parenchymal integrity.
Nephrol Dial Transplant, 33(2):284-295, 01 Feb 2018
Cited by: 62 articles | PMID: 28206617 | PMCID: PMC5837243
Association of Serum Uromodulin With ESKD and Kidney Function Decline in the Elderly: The Cardiovascular Health Study.
Am J Kidney Dis, 74(4):501-509, 22 May 2019
Cited by: 19 articles | PMID: 31128770 | PMCID: PMC7188359