Abstract
Free full text

Homeostatic T Cell Proliferation
Through positive and negative selection, the thymus allows a small fraction of immature CD4+8+ double positive thymocytes to differentiate into mature CD4+8− or CD4−8+ single positive cells; these cells are released into the periphery to establish the mature T cell pool. Positive selection rescues thymocytes that express TCR with low affinity for peptides bound to MHC molecules expressed on cortical epithelial cells. Conversely, negative selection eliminates thymocytes with high affinity for MHC–peptide complexes, thereby leading to self-tolerance induction 1 2. Via this process of selection, the thymus generates a peripheral repertoire that is largely depleted of overtly autoreactive T cells but retains low but significant reactivity for self-MHC molecules. Retaining weak affinity for self-MHC/peptide ligands has generally been considered a requirement for T cells to optimally recognize foreign antigens in the context of self-MHC molecules. However, recent findings strongly suggest that low-level self-reactivity serves an additional purpose: namely, to maintain survival and homeostasis of naive T cells (for a review, see reference 3).
Mature naive T cells are usually considered to remain in a dormant state unless awakened by foreign antigens expressed on activated APCs. This view has now been modified by the finding that prolonged survival of naive T cells in a resting state requires low-level TCR signaling from contact with self-MHC/peptide ligands (i.e., with MHC class I molecules for CD8+ cells and class II molecules for CD4+ cells 4 5 6 7 8 9 10). In the absence of these self-ligands, naive T cells gradually disappear. Evidence for active signaling through the TCR is also provided by the finding that survival of resting naive T cells requires expression of lung Kruppel-like factor (LKLF), a member of Kruppel-like zinc transcription factor family 11. This molecule is presumably involved in translating covert TCR signaling into cell survival cues.
It has long been known that mature T cells are regulated at a population level by homeostatic mechanisms that maintain the total size of the T cell pool at a near-constant level 12 13 14. Normally, expansion of the T cell pool during an immune response is followed by a deletion phase in which most of the newly generated effector cells are eliminated at the end of the response, thereby restoring total T cells numbers to normal levels 15 16. On the other hand, it is also well established that T cells have the capacity to spontaneously undergo extensive proliferation after transfer into immunodeficient hosts 17. Such “homeostatic” proliferation of T cells occurs when small numbers of T cells are adoptively transferred into T cell–depleted (T-depleted) syngeneic nude, SCID, recombination activating gene (RAG)-deficient, or irradiated hosts 18 19. With more recent use of mice deficient in MHC class I or II molecules, there is now a clear consensus that homeostatic proliferation of CD4+ and CD8+ cells requires contact with self-MHC class II and I molecules, respectively 20 21 22 23 24 25 26 27. Because homeostatic proliferation applies at a polyclonal level and occurs without deliberate antigen injection, the prevailing view has been that such proliferation is driven by foreign antigens (which are common in immunodeficient hosts) and reflects antigen-specific expansion of memory T cells 14 17. Here, the underlying assumption has been that naive T cells are completely unresponsive to self-MHC/peptide ligands because of tolerance induction.
However, recent work from several laboratories strongly suggests that homeostatic proliferation applies to naive T cells and is driven by low-affinity interactions with self-MHC molecules loaded with self-peptides 22 24 25 26 27. Interestingly, there is compelling evidence that homeostatic proliferation of naive T cells is driven by particular MHC–peptide complexes, namely the peptides that initially induced positive selection of the T cells in the thymus. Two lines of investigation support this view. The first involves experiments performed in H2-M− mice, which express MHC class II (Ab) molecules loaded almost exclusively with a single species of self-peptides, class II–associated invariant chain peptides (CLIPs; 28 29 30). The key finding was that wild-type B6 naive CD4+ cells, i.e., cells that were positively selected on Ab molecules loaded with a spectrum of self-peptides, failed to undergo efficient homeostatic proliferation after transfer to T-depleted H2-M− hosts; conversely, naive CD4+ cells from H2-M− hosts, i.e., cells that were positively selected to a single ligand (Ab+CLIP), underwent efficient proliferation when exposed to this ligand in T-depleted H2-M− hosts 22 24. The second approach involved the use of transporter associated with antigen processing (TAP)− mice that were engineered to express MHC class I (Kb) molecules loaded with specific peptides; these mice were used as hosts for OVA-specific, Kb-restricted CD8+ OT-I TCR transgenic T cells. As with CD4+ cells in the above model, homeostatic proliferation of CD8+ OT-I cells was observed only when the T-depleted syngeneic TAP− hosts expressed the particular low-affinity peptide that had been shown previously to induce positive selection of OT-I cells. With host expression of an irrelevant peptide, homeostatic proliferation was minimal 25.
Although all typical T cells are thought to require positive selection by self-MHC/peptide ligands in the thymus, not all T cells undergo homeostatic proliferation. Thus, studies with polyclonal T cells indicate that only ~30% of the starting population of naive CD4+ and CD8+ cells undergo detectable cell division within 1–2 wk in lymphopenic hosts 24. This heterogeneity also applies to TCR transgenic T; cells. Thus, some transgenic lines, such as CD8+ OT-I, 2C, P14, and CD4+ DO11, 1H3.1 are able to undergo homeostatic proliferation, whereas other lines (e.g., CD8+T3.70+ HY-specific cells and CD4+ OT-II lines) do not undergo homeostatic proliferation 21 22 24 25 26 31. In fact, the past assumption that homeostatic proliferation is directed to foreign antigens was based on the finding that in T-depleted neutral (female) hosts, proliferation of CD8+ cells from the HY line applies only to TCR clonotype-negative (T3.70−) and not to clonotype-positive cells 31. Why only a proportion of T cells can undergo homeostatic proliferation is unclear. One possibility is that some of the peptides inducing positive selection in the thymus are expressed at only a very low level in the periphery. Another possibility, which is not mutually exclusive, is that the strength of affinity for self-ligands required for T cells to undergo homeostatic proliferation is slightly higher than the strength of affinity required for thymic positive selection. According to this latter scenario, homeostatic proliferation is a property restricted to T cells that have been positively selected to self-peptides with “above average” TCR affinity 24. Despite these possibilities, it should be noted that even with TCR transgenic cells, only a proportion of T cells undergo homeostatic proliferation. This stochastic component of homeostatic proliferation has yet to be explained.
In contrast to responses to high-affinity foreign peptides, homeostatic proliferation of naive T cells to self-MHC–peptide complexes is relatively slow and is not associated with upregulation of acute activation markers such as CD25 and CD69 21 24 25 26 27. Nevertheless, the dividing cells acquire cell surface markers typically expressed on memory T cells; e.g., the cells become CD44hi and Ly6Chi (for CD8+ cells [21, 24–27]). This finding raises the question of whether homeostatic proliferation of naive T cells lead to full differentiation into memory T cells. This topic is the focus of three recent papers by Goldrath et al. 32, Cho et al. (33; both of these papers appear in this issue), and Murali-Krishna and Ahmed 34. For these papers, there is a clear consensus that after homeostatic proliferation, naive T cells do acquire characteristics of true memory cells, including the capacity to mount accelerated functional responses to cognate antigens. However, as discussed below, there is some discrepancy as to the ultimate fate of these cells once homeostasis is restored.
To study T cells undergoing homeostatic proliferation, all three groups monitored donor naive CD8+ transgenic cells transferred to T-depleted syngenic mice. Each group used a different CD8+ transgenic line (OT-I, 2C, and P-14). The cellular changes observed during homeostatic proliferation applied equally to all three lines, and also extended to nontransgenic polyclonal wild-type CD8+ cells (studied by Cho et al [33]). Consolidating information from the earlier reports mentioned above, these studies clearly show that homeostasis-driven activation of T cells by low-affinity self-ligands is distinct from the typical overt activation induced by high-affinity foreign antigens. Thus, unlike antigen-activated T cells, T cells undergoing homeostasis proliferation do not enlarge and fail to upregulate CD71 (transferrin receptor [34]). CD44 upregulation is slower, and cells become CD44hi only after multiple rounds of cell division 33 34. In terms of other markers typically used to define memory T cells, T cells undergoing homeostatic proliferation show decreased expression of CD45RB but not CD62L 25 27 34. As with conventional T cell activation, upregulation of CD122 (receptor for IL-2/IL-15) and CD132 (common cytokine receptor, γc) is prominent 32 33 34.
It is of particular interest that the progeny of T cells undergoing homeostatic proliferation were found to display significant effector function. Thus, whereas naive CD8+ T cells cannot mediate CTL activity or secrete IFN-γ without prior stimulation, homeostasis-activated T cells displayed CTL activity and produced IFN-γ when stimulated with a foreign antigen directly ex vivo 32 33 34. The intensity of the effector responses displayed by homeostasis-activated T cells resembled that of resting memory T cells and, as expected, were clearly less marked than the responses of overtly activated effector T cells stimulated by foreign antigens. The ability to secrete IFN-γ and display direct CTL activity in vitro applied to all three lines of transgenic T cells (only two were tested for CTL activity). Similar findings applied to nontransgenic T cells. Thus, the polyclonal progeny of normal B6 T cells undergoing homeostatic proliferation rapidly secreted IFN-γ after in vitro stimulation with anti-CD3 mAb and killed Con A–coated target cells directly ex vivo, whereas control naive B6 CD8+ cells had no activity in these assays 33.
As with upregulation of CD44, the ability to secrete IFN-γ after homeostatic proliferation increased progressively with each cell division 33 34. As homeostatic proliferation of T cells eventually ceases when total T cell numbers return to near-normal levels, the question arises whether the progeny of the proliferating cells remain as memory cells or reacquire a naive phenotype. Here, the results were more complex. Cho et al. 33 found that transgenic 2C cells injected into T-depleted (RAG-1−) hosts remained “memory-like” even after 2 mo 33. However, a complication in this study was that the injected 2C cells did not accumulate, and homeostatic proliferation appeared to have continued, presumably indicating that many of the proliferating cells died. Goldrath et al. 32 also observed this phenomenon in RAG-1− hosts injected with OT-I cells. Thus, even after 3 mo, these RAG-1− mice remained partially lymphopenic and contained OT-I cells that continued to undergo homeostatic proliferation (as measured by uptake of the DNA precursor bromodeoxyuridine; reference 32). As with 2C cells, the OT-I cells in RAG-1− hosts remained “memory-like” in terms of phenotype and function.
For obscure reasons, the lack of accumulation of large numbers of proliferating donor T cells applies to RAG-1− hosts but not to normal B6 mice rendered lymphopenic by exposure to a sublethal dose of whole body irradiation, which eliminates >95% of naive T cells. Thus, despite virtually identical rates of homeostatic proliferation, Goldrath et al. 32 found that OT-I cells accumulated in much higher numbers in irradiated B6 hosts than in nonirradiated RAG-1− hosts 32. In irradiated B6 hosts, the injected OT-I cells underwent massive expansion and ceased cycling within 2 mo after injection, when the hosts regained normal T cell numbers. More importantly, even though the OT-I cells closely resembled memory cells during the first few weeks after transfer, the noncycling OT-I cells isolated after 2 mo were naive in terms of both phenotype (CD44lo, Ly6Clo, and CD122lo) and function (no ex vivo CTL activity or IFN-γ secretion). This finding is in agreement with the earlier observation of Bell and Spartshott that, in rats, a proportion of memory phenotype CD4+ cells reverted to a naive phenotype after adoptive transfer into syngeneic athymic nude rats 35.
Despite these findings, reversion of memory phenotype cells to “naive” cells after homeostatic proliferation is apparently not a general phenomenon. Thus, in similar experiments, Murali-Krishna and Ahmed 34 found that P-14 transgenic CD8+ cells remained “memory-like” in phenotype and function (IFN-γ secretion) even 5 mo after the cells were injected into sublethally irradiated B6 hosts 34. It is unlikely that this finding reflects continued proliferation of P-14 cells, as previous studies showed that these cells do not proliferate when the size of the T cell pool is normal, i.e., when P-14 cells are transferred to normal (nonirradiated) B6 mice 21 24. What accounts for the difference in the behavior of OT-I and P-14 cells is unclear. One possibility is that the TCR affinity for the stimulatory self-MHC/peptide ligands is higher for P-14 than OT-I, with the result that differentiation into memory cells becomes “fixed” for P-14 cells but is reversible for OT-I cells. Whatever the explanation, determining the frequency of memory to naive reversion and defining the rules governing this transition will have to await further studies, both with TCR transgenic and polyclonal T cells populations.
Despite the convincing evidence that homeostatic proliferation generates memory cells, whether these cells can be equated with “real” memory cells arising after an immune response to foreign antigens is still uncertain (Fig. 1). One point to emphasize is that antigen-specific memory cells are thought to originate from fully activated effector cells, most of which are rapidly eliminated at the end of the primary response. By contrast, the memory cells generated through homeostatic proliferation do not seem to arise from overtly activated effector cells and accumulate progressively, presumably signifying only limited cell death. Likewise, costimulation via CD28 and the availability of IL-2 seems to be much more important for the generation of antigen-specific memory cells than for homeostasis-driven memory cells (reference 33; our unpublished observations). A key question is whether the requirements for maintaining the survival of these two types of memory cells are the same or different. For antigen-specific memory cells, it is now becoming clear that these cells are largely MHC independent, both for survival and intermittent cell division 10 36 37. At least for CD8+ cells, the survival and proliferation of memory cells seem to be driven by cytokines, especially by IL-15 38 39 40 41. Whether cytokines also control the survival and turnover of homeostasis-driven memory cells has yet to be studied, although the high expression of CD122, a receptor for IL-15, on these cells is in favor of this possibility. Further studies may well show that, once formed, many of the memory cells generated by homeostatic proliferation are virtually indistinguishable from true memory cells. However, comprehensive evidence on this question is still lacking.
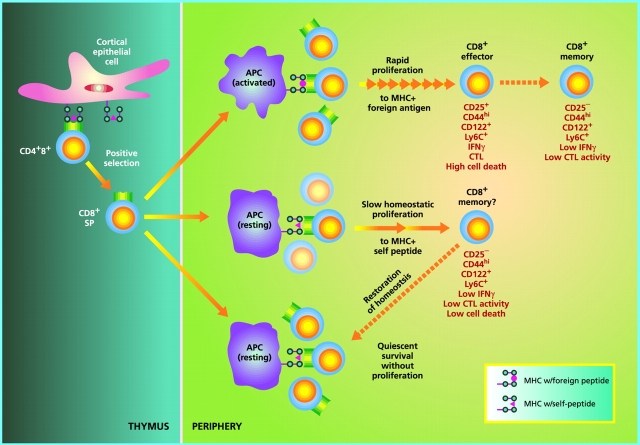
Regulation of naive CD8+ T cell homeostasis by self-MHC/peptide ligands. In the thymus, immature CD4+8+ double positive cells that interact with low but significant affinity for MHC class I molecules loaded with self-peptides become positively selected and differentiate into mature CD8+ single positive cells. Upon exit to the periphery, CD8+ cells continuously interact with the same self-MHC/peptide ligands or possibly with cross-reactive self-ligands on APCs, and receive low-level signals through the TCR. Under physiological conditions with normal numbers of T cells, such TCR signals are covert, i.e., insufficient to induce entry into cell cycle but adequate to keep cells alive (bottom). However, if the number of T cells drops below a certain level, TCR signals become overtly stimulatory and induce T cells to undergo a slow form of “homeostatic” proliferation in an attempt to restore the size of the T cell pool. During homeostatic proliferation, CD8+ cells resemble resting memory cells in terms of phenotype and their ability to mediate low-level effector function in response to foreign antigens (middle). Upon restoration of the naive T cell pool, homeostatic proliferation stops and some CD8+ clones revert to a naive phenotype, whereas others do not; the basis of this difference is unknown. Generation of “conventional” memory cells through T cell interaction with foreign peptide is qualitatively different in several respects (top). Thus, in contrast to memory cell generation via homeostatic proliferation, production of antigen-specific memory cells (a) requires APC activation (via an adjuvant), (b) involves transition through an activated effector cell phase, (c) is associated with prominent cell death (at the end of the primary response), and (d) leads to long-term expression of memory markers, especially CD44, with little or no reversion to naive phenotype cells. These differences presumably also apply to CD4+ cells (not depicted).
With regard to physiological significance, it should be emphasized that homeostatic proliferation of T cells to self-ligands is probably quite limited under normal conditions, i.e., where the lymphoid tissues contain large numbers of naive T cells. However, under conditions of T lymphocytopenia, homeostatic proliferation to self-ligands may make a substantial contribution to replenishment of the T cell pool. This situation may arise in patients treated with cytotoxic drugs and/or irradiation, and perhaps also during severe viral infections, which may induce a marked lymphocytopenia. Homeostatic proliferation may also operate in old age. Thus with the decline in thymus function in old age, the gradual decrease in numbers of naive T cells may cause homeostatic proliferation of residual naive cells; differentiation of these cells into memory cells could then account for the marked overrepresentation of memory-phenotype cells in old age 42 43.
It is important to point out that homeostatic proliferation of T cells is not limited to naive T cells and can also apply to memory cells 10. Indeed, homeostatic proliferation tends to be more marked for memory cells than for naive cells. Interestingly, in marked contrast to naive cells, homoestatic proliferation of memory cells in T-depleted hosts is MHC independent (10; our unpublished observations). In addition, whereas homeostatic proliferation by naive T cells is inhibited by bystander naive cells, homeostatic proliferation of memory cells is not suppressed by naive cells (our unpublished observations). Hence, the mechanisms controlling homeostatic proliferation of naive and memory cells seem to be fundamentally different.
On this point, it is striking that despite the decline in the thymic function in old age and diminished production of naive T cells, the total size of the T cell pool remains relatively constant throughout life 44 45. How homeostasis is controlled at the levels of total T cell numbers remains a mystery. Similar to the factors controlling cell growth in other tissues such as the skin and liver 46 47, T cells somehow seem to be able to sense the density of neighboring T cells and discriminate between naive and memory cells. Whether this awareness of total T cell density reflects direct T–T interaction, competition for “space” at the APC level, restrictions in the availability of growth factors or soluble mediators, or all of these factors combined is unknown. Clearly a great deal remains to be known about T cell homeostasis and about homeostasis in general.
Acknowledgments
This work was supported by grants AG-01473, AI-21487, AI-35385, AI-41079, AI-45809, AI-46710, CA-25803, and CA-38355 from the National Institutes of Health. C.D. Surh is a Scholar of the Leukemia & Lymphoma Society.
References
- Jameson S.C., Hogquist K.A., Bevan M.J. Positive selection of thymocytes. Annu. Rev. Immunol. 1995;13:93–126. [Abstract] [Google Scholar]
- Sprent J., Webb S. R. Intrathymic and extrathymic clonal deletion of T cells. Curr. Opin. Immunol. 1995;7:196–205. [Abstract] [Google Scholar]
- Goldrath A.W., Bevan M.J. Selecting and maintaining a diverse T-cell repertoire. Nature. 1999;402:255–262. [Abstract] [Google Scholar]
- Takeda S., Rodewald H.-R., Arakawa H., Bluethmann H., Shimizu T. MHC class II molecules are not required for survival of newly generated CD4+ T cells but affect their long-term life span. Immunity. 1996;5:217–228. [Abstract] [Google Scholar]
- Brocker T. Survival of mature CD4 T lymphocytes is dependent on major histocompatibility complex class II–expressing dendritic cells. J. Exp. Med. 1997;186:1223–1232. [Europe PMC free article] [Abstract] [Google Scholar]
- Kirberg J., Berns A., von Boehmer H. Peripheral T cell survival requires continual ligation of the T cell receptor to major histocompatibility complex–encoded molecules. J. Exp. Med. 1997;186:1269–1275. [Europe PMC free article] [Abstract] [Google Scholar]
- Rooke R., Waltzinger C., Benoist C., Mathis D. Targeted complementation of MHC class II deficiency by intrathymic delivery of recombinant adenoviruses. Immunity. 1997;7:123–134. [Abstract] [Google Scholar]
- Tanchot C., Lemonnier F.A., Pérarnau B., Freitas A.A., Rocha B. Differential requirements for survival and proliferation of CD8 naive or memory T cells. Science. 1997;276:2057–2062. [Abstract] [Google Scholar]
- Nesic D., Vukmanovic S. MHC class I is required for peripheral accumulation of CD8+ thymic emigrants. J. Immunol. 1998;160:3705–3712. [Abstract] [Google Scholar]
- Murali-Krishna K., Lau L.L., Sambhara S., Lemonnier F., Altman J., Ahmed R. Persistence of memory CD8 T cells in MHC class I-deficient mice. Science. 1999;286:1377–1381. [Abstract] [Google Scholar]
- Kuo C.T., Veselits M.L., Leiden J.M. LKLFa transcriptional regulator of single-positive T cell quiescence and survival. Science. 1997;277:1986–1990. [Abstract] [Google Scholar]
- Freitas A.A., Rocha B.B. Lymphocyte lifespanhomeostasis, selection and competition. Immunol. Today. 1993;14:25–29. [Abstract] [Google Scholar]
- Bell E.B., Sparshott S.M. The peripheral T-cell poolregulation by non-antigen induced proliferation? Semin. Immunol. 1997;9:347–353. [Abstract] [Google Scholar]
- Mackall C.L., Hakim F.T., Gress R.E. Restoration of T-cell homeostasis after T-cell depletion. Semin. Immunol. 1997;9:339–346. [Abstract] [Google Scholar]
- Webb S., Morris C., Sprent J. Extrathymic tolerance of mature T cellsclonal elimination as a consequence of immunity. Cell. 1990;63:1249–1256. [Abstract] [Google Scholar]
- Van Parijs L., Abbas A.K. Homeostasis and self-tolerance in the immune systemturning lymphocytes off. Science. 1998;280:243–248. [Abstract] [Google Scholar]
- Tanchot C., Rosado M.M., Agenes F., Freitas A.A., Rocha B. Lymphocyte homeostasis. Semin. Immunol. 1997;9:331–337. [Abstract] [Google Scholar]
- Bell E.B., Sparshott S.M., Drayson M.T., Ford W.L. The stable and permanent expansion of functional T lymphocytes in athymic nude rats after a single injection of mature T cells. J. Immunol. 1987;139:1379–1384. [Abstract] [Google Scholar]
- Rocha B., Dautigny N., Pereira P. Peripheral T lymphocytesexpansion potential and homeostatic regulation of pool sizes and CD4/8 ratios in vivo . Eur. J. Immunol. 1989;19:905–911. [Abstract] [Google Scholar]
- Beutner U., MacDonald H.R. TCR-MHC class II interaction is required for peripheral expansion of CD4 cells in a T cell-deficient host. Int. Immunol. 1998;10:305–310. [Abstract] [Google Scholar]
- Oehen S., Brduscha-Riem K. Naive cytotoxic T lymphocytes spontaneously acquire effector function in lymphocytopenic recipientsa pitfall for T cell memory studies? Eur. J. Immunol. 1999;29:608–614. [Abstract] [Google Scholar]
- Viret C., Wong F.S., Janeway C.A., Jr. Designing and maintaining the mature TCR repertoirethe continuum of self-peptide:self-MHC complex recognition. Immunity. 1999;10:559–568. [Abstract] [Google Scholar]
- Bender J., Mitchell T., Kappler J., Marrack P. CD4+ T cell division in irradiated mice requires peptides distinct from those responsible for thymic selection. J. Exp. Med. 1999;190:367–374. [Europe PMC free article] [Abstract] [Google Scholar]
- Ernst B., Lee D.-S., Chang J.M., Sprent J., Surh C.D. The peptide ligands mediating positive selection in the thymus control T cell survival and homeostatic proliferation in the periphery. Immunity. 1999;11:173–181. [Abstract] [Google Scholar]
- Goldrath A.W., Bevan M.J. Antagonist ligands for the TCR drive proliferation of mature CD8+ T cells in lymphopenic hosts. Immunity. 1999;11:183–190. [Europe PMC free article] [Abstract] [Google Scholar]
- Kieper W.C., Jameson S.C. Homeostatic expansion and phenotypic conversion of naive T cells in response to self peptide/MHC ligands. Proc. Natl. Acad. Sci. USA. 1999;96:13306–13311. [Europe PMC free article] [Abstract] [Google Scholar]
- Muranski P., Chmielowski B., Ignatowicz L. Mature CD4+ T cells perceive a positively selecting class II MHC/peptide complex in the periphery. J. Immunol. 2000;164:3087–3094. [Abstract] [Google Scholar]
- Fung-Leung W.-P., Surh C.D., Liljedahl M., Pang J., Leturcq D., Peterson P.A., Webb S.R., Karlsson L. Antigen presentation and T cell development in H2-M-deficient mice. Science. 1996;271:1278–1281. [Abstract] [Google Scholar]
- Miyazaki T., Wolf P., Tourne S., Waltzinger C., Dierich A., Barois N., Ploegh H., Benoist C., Mathis D. Mice lacking H2-M complexes, enigmatic elements of the MHC class II peptide-loading pathway. Cell. 1996;84:531–541. [Abstract] [Google Scholar]
- Martin W.D., Hicks G.G., Mendiratta S.K., Leva H.I., Ruley H.E., Van Kaer L. H2-M mutant mice are defective in the peptide loading of class II molecules, antigen presentation, and T cell repertoire selection. Cell. 1996;84:543–550. [Abstract] [Google Scholar]
- Rocha B., von Boehmer H. Peripheral selection of the T cell repertoire. Science. 1991;251:1225–1228. [Abstract] [Google Scholar]
- Goldrath A.W., Bogatzki L.Y., Bevan M.J. Naive T cells transiently acquire a memory-like phenotype during homeostasis-driven proliferation. J. Exp. Med. 2000;192:557–564. [Europe PMC free article] [Abstract] [Google Scholar]
- Cho B.K., Rao V.P., Ge Q., Eisen H.N., Chen J. Homeostasis-stimulated proliferation drives naive T cells to differentiate directly into memory T cells. J. Exp. Med. 2000;192:549–556. [Europe PMC free article] [Abstract] [Google Scholar]
- Murali-Krishna K., Ahmed R. Naive T cells masquerading as memory cells. J. Immunol. 2000;In press [Abstract] [Google Scholar]
- Bell E.B., Sparshott S.M. Interconversion of CD45R subsets of CD4 T cells in vivo. Nature. 1990;348:163–166. [Abstract] [Google Scholar]
- Garcia S., DiSanto J., Stockinger B. Following the development of a CD4 T cell response in vivofrom activation to memory formation. Immunity. 1999;11:163–171. [Abstract] [Google Scholar]
- Swain S.L., Hu H., Huston G. Class II-independent generation of CD4 memory T cells from effectors. Science. 1999;286:1381–1383. [Abstract] [Google Scholar]
- Zhang X., Sun S., Hwang I., Tough D.F., Sprent J. Potent and selective stimulation of memory-phenotype CD8+ T cells in vivo by IL-15. Immunity. 1998;8:591–599. [Abstract] [Google Scholar]
- Lodolce J.P., Boone D.L., Chai S., Swain R.E., Dassopoulos T., Trettin S., Ma A. IL-15 receptor maintains lymphoid homeostasis by supporting lymphocyte homing and proliferation. Immunity. 1998;9:669–676. [Abstract] [Google Scholar]
- Kennedy M.K., Glaccum M., Brown S.N., Butz E.A., Viney J.L., Embers M., Matsuki N., Charrier K., Sedger L., Willis C.R. Reversible defects in natural killer and memory CD8 T cell lineages in interleukin 15–deficient mice. J. Exp. Med. 2000;191:771–780. [Europe PMC free article] [Abstract] [Google Scholar]
- Ku C.C., Murakami M., Sakamoto A., Kappler J., Marrack P. Control of homeostasis of CD8+ memory T cells by opposing cytokines. Science. 2000;288:675–678. [Abstract] [Google Scholar]
- Cossarizza A., Ortolani C., Paganelli R., Barbieri D., Monti D., Sansoni P., Fagiolo U., Castellani G., Bersani F., Londei M., Franceschi C. CD45 isoforms expression on CD4+ and CD8+ T cells throughout life, from newborns to centenariansimplications for T cell memory. Mech. Ageing Dev. 1996;86:173–195. [Abstract] [Google Scholar]
- Miller R.A. The aging immune systemprimer and prospectus. Science. 1996;273:70–74. [Abstract] [Google Scholar]
- Sprent J., Tough D.F. Lymphocyte life-span and memory. Science. 1994;265:1395–1400. [Abstract] [Google Scholar]
- Sprent J., Webb S.R. Function and specificity of T-cell subsets in the mouse. Adv. Immunol. 1987;41:39–133. [Abstract] [Google Scholar]
- Michalopoulos G.K., DeFrances M.C. Liver regeneration. Science. 1997;276:60–66. [Abstract] [Google Scholar]
- Raff M.C. Size controlthe regulation of cell numbers in animal development. Cell. 1996;86:173–175. [Abstract] [Google Scholar]
Articles from The Journal of Experimental Medicine are provided here courtesy of The Rockefeller University Press
Full text links
Read article at publisher's site: https://doi.org/10.1084/jem.192.4.f9
Read article for free, from open access legal sources, via Unpaywall:
https://rupress.org/jem/article-pdf/192/4/F9/1129678/001016.pdf
Citations & impact
Impact metrics
Citations of article over time
Alternative metrics
Smart citations by scite.ai
Explore citation contexts and check if this article has been
supported or disputed.
https://scite.ai/reports/10.1084/jem.192.4.f9
Article citations
Case report: Artificial thymic organoids facilitate clinical decisions for a patient with a <i>TP63</i> variant and severe persistent T cell lymphopenia.
Front Immunol, 15:1438383, 18 Sep 2024
Cited by: 0 articles | PMID: 39364398 | PMCID: PMC11448704
Reappraising the Role of T Cell-Derived IFN-γ in Restriction of Mycobacterium tuberculosis in the Murine Lung.
J Immunol, 213(3):339-346, 01 Aug 2024
Cited by: 0 articles | PMID: 38912839 | PMCID: PMC11249196
Microbiota metabolites in bone: Shaping health and Confronting disease.
Heliyon, 10(7):e28435, 20 Mar 2024
Cited by: 3 articles | PMID: 38560225 | PMCID: PMC10979239
Review Free full text in Europe PMC
Downregulation of MALAT1 is a hallmark of tissue and peripheral proliferative T cells in COVID-19.
Clin Exp Immunol, 212(3):262-275, 01 Jun 2023
Cited by: 2 articles | PMID: 36869729 | PMCID: PMC10243916
Robust immunity to influenza vaccination in haematopoietic stem cell transplant recipients following reconstitution of humoral and adaptive immunity.
Clin Transl Immunology, 12(6):e1456, 27 Jun 2023
Cited by: 0 articles | PMID: 37383182 | PMCID: PMC10294294
Go to all (191) article citations
Similar Articles
To arrive at the top five similar articles we use a word-weighted algorithm to compare words from the Title and Abstract of each citation.
Homeostatic expansion versus antigen-driven proliferation: common ends by different means?
Microbes Infect, 4(5):531-537, 01 Apr 2002
Cited by: 27 articles | PMID: 11959508
Review
The immunological synapse: a molecular machine controlling T cell activation.
Science, 285(5425):221-227, 01 Jul 1999
Cited by: 1828 articles | PMID: 10398592
Dancing the immunological two-step.
Science, 285(5425):207-208, 01 Jul 1999
Cited by: 13 articles | PMID: 10428718
T cell memory.
Annu Rev Immunol, 20:551-579, 04 Oct 2001
Cited by: 357 articles | PMID: 11861612
Review
Funding
Funders who supported this work.
NCI NIH HHS (1)
Grant ID: R37 CA038355
NIA NIH HHS (1)
Grant ID: AG-01473
NIAID NIH HHS (4)
Grant ID: AI-21487
Grant ID: R01 AI045809
Grant ID: R01 AI046710
Grant ID: AI-35385