Abstract
Free full text

Apical Surface Expression of Aspartic Protease Plasmepsin 4, a Potential Transmission-blocking Target of the Plasmodium Ookinete*
Associated Data
Abstract
To invade its definitive host, the mosquito, the malaria parasite must cross the midgut peritrophic matrix that is composed of chitin cross-linked by chitin-binding proteins and then develop into an oocyst on the midgut basal lamina. Previous evidence indicates that Plasmodium ookinete-secreted chitinase is important in midgut invasion. The mechanistic role of other ookinete-secreted enzymes in midgut invasion has not been previously examined. De novo mass spectrometry sequencing of a protein obtained by benzamidine affinity column of Plasmodium gallinaceum ookinete axenic culture supernatant demonstrated the presence of an ookinete-secreted plasmepsin, an aspartic protease previously only known to be present in the digestive vacuole of asexual stage malaria parasites. This plasmepsin, the ortholog of Plasmodium falciparum plasmepsin 4, was designated PgPM4. PgPM4 and PgCHT2 (the P. gallinaceum ortholog of P. falciparum chitinase PfCHT1) are both localized on the ookinete apical surface, and both are present in micronemes. Aspartic protease inhibitors (peptidomimetic and natural product), calpain inhibitors, and anti-PgPM4 monoclonal antibodies significantly reduced parasite infectivity for mosquitoes. These results suggest that plasmepsin 4, previously known only to function in the digestive vacuole of asexual blood stage Plasmodium, plays a role in how the ookinete interacts with the mosquito midgut interactions as it becomes an oocyst. These data are the first to delineate a role for an aspartic protease in mediating Plasmodium invasion of the mosquito and demonstrate the potential for plasmepsin 4 as a malaria transmission-blocking vaccine target.
Introduction
Malaria is the most important parasitic disease of man and a globally dominant cause of morbidity and mortality in humans (1). The lack of an effective vaccine, the emergence of drug-resistant Plasmodium falciparum and Plasmodium vivax, and insecticide-resistant vector mosquitoes all contribute to the increasing human toll of malaria. Malaria is transmitted through the bite of infected Anopheles mosquitoes. Preventing transmission from the human reservoir to the definitive host, the mosquito, is one approach to malaria control (2). Delineating the mechanisms by which the malaria parasite invades and infects mosquitoes may lead to new strategies to block malaria transmission (3, 4).
After a mosquito ingests infectious Plasmodium gametocytes, male and female gametes emerge in the midgut and rapidly fuse to form diploid zygotes. Parasites must then develop into motile ookinetes, penetrate and traverse the protein- and chitin-containing peritrophic matrix, and then cross the midgut epithelium to form oocysts (4). Developmentally regulated antigens of these stages are potential targets of antibodies induced by vaccination of the vertebrate host that are co-ingested with parasites as a mosquito takes a blood meal (5). Such antibodies are called transmission-blocking antibodies, which act by interfering with parasite development within the mosquito midgut, thus preventing parasite transmission to the mosquito vector. Importantly, proteins expressed in the mosquito stages are less likely to be mutated in response to human immunological responses (6). Hence, interfering in this part of the life cycle has the potential to reduce both transmission as well as the spread of drug-resistant parasites.
Ookinete-expressed proteases have been proposed to play vital roles in ookinete invasion of peritrophic matrix and mosquito midgut (7,–9). The peritrophic matrix is the first physical barrier faced by the ookinete as it escapes the blood meal. The peritrophic matrix is composed of proteins, glycoproteins, proteoglycans, and chitin (10, 11). Proteins, including chitin cross-linking proteins (peritrophins), have been reported to account for 22–55% of the total mass of the peritrophic matrix (10, 11). Specific protease inhibitors added to infectious blood meals have been observed to reduce ookinete infectivity for the mosquito (12). These observations suggest that ookinetes could use proteases to cross the midgut peritrophic matrix.
The genome of P. falciparum encodes a large variety of proteases, including a diverse family of 10 aspartic proteases (designated plasmepsins) (13). Four plasmepsins are known to degrade hemoglobin in the digestive vacuole of asexual stage malaria parasites (14, 15). Functions of the remaining six plasmepsins have not been determined, although gene expression profiling and comprehensive proteomic analysis have demonstrated the presence of several plasmepsins in the sexual stage forms of P. falciparum, Plasmodium berghei, and Plasmodium yoelii (16, 17). No role for any plasmepsin has been demonstrated in Plasmodium-mosquito interactions.
In this study, we demonstrate that the P. gallinaceum plasmepsin 4 (PgPM4)2 synergizes with the P. gallinaceum chitinase PgCHT2 (the ortholog of the P. falciparum chitinase (18)) to facilitate malaria parasite invasion of the mosquito midgut and/or may be involved in the development of ookinete to oocyst. These data are the first to indicate a specific mechanistic function for a plasmepsin in any stage of the malaria parasite other than the asexual blood stage. This aspartic protease plays an important role in ookinete invasion of the mosquito midgut and/or parasite development, and thus may be a novel target of blocking malaria transmission.
MATERIALS AND METHODS
Parasite, Mosquitoes, and Membrane Feeding Assay
P. gallinaceum strain 8a was maintained by chicken and mosquito passage. Preparations of zygotes and ookinetes were performed as described previously (19). Aedes aegypti was maintained at 26 °C and 80% relative humidity and fed on 10% sugar solution. P. gallinaceum-infected blood, with or without inhibitors or antibodies, was fed to A. aegypti mosquitoes between 5 and 7 days post-emergence. Midguts were dissected in a drop of mercurochrome in phosphate-buffered saline 8 days after the blood feed, and oocysts were counted under light microscopy. Differences in infection rates of mosquitoes and geometric mean number of oocysts were analyzed by Mann-Whitney test.
Identification of P. gallinaceum Ookinete-secreted Plasmepsin 4
Plasmepsin 4 was isolated from P. gallinaceum ookinete-conditioned medium using affinity chromatography. Briefly, the conditioned medium was concentrated using a Centricon-10 apparatus (Amicon) and passed over a benzamidine-Sepharose column (GE Biosciences), and the column was washed extensively with 0.1% Triton X-100 in PBS. Bound proteins were eluted from the column with soluble benzamidine in PBS, yielding a single symmetrical peak. The eluate was subjected to SDS-PAGE; the most intense Coomassie-stained band was excised from the gel and analyzed by de novo mass spectrometry sequencing (Bill Lane, Microchemistry Laboratory, Harvard University).
Two-dimensional gel electrophoresis was carried out on acetone-precipitated ookinete-conditioned medium. Approximately 70 μg of the protein was loaded onto an 11-cm immobilized pH gradient IPG strip (nonlinear pH 3–10, Bio-Rad) and applied to isoelectric focusing strips that were rehydrated overnight in a buffer consisting of 9.8 m urea, 4% CHAPS, 0.2% ampholyte, 10 mm dithiothreitol, and bromphenol blue. The sample was passively rehydrated for 4 h and then actively rehydrated for 10 h at 50 V. Focusing was accomplished with 500 V for 15 min, 8,000 V for 4 h, and a third step of 35,000 V for 12 h at 500 V-h. Resolved proteins were equilibrated for 15 min in buffer I consisting of 6 m urea, 0.376 m Tris-HCl, pH 9.4, 2% SDS, 2% dithiothreitol and buffer II consisting of 6 m urea, 0.376 m Tris-HCl, pH 9.4, 2% SDS, 3% iodoacetamide and then washed with SDS running buffer and separated with SDS-PAGE using 12.5% Tris-HCl gels in a Protean II apparatus (Bio-Rad) operated at 200 V for 57 min. Mass spectrometry-compatible silver staining (Silverquest silver staining kit, Invitrogen) was used to detect proteins. Gel spots were manually excised, digested with trypsin, and submitted for mass spectrometry analysis.
Monoclonal Antibody Production against PgPM4
Murine monoclonal antibodies (mAbs) were generated using synthetic peptides CEDKFYEGEITYEK, CKFIEVTDTDDLEP, and CEPKFLYDTLEEVDE derived from the predicted Plasmodium gallinaceum PgPM4 amino acid sequence. The N-terminal cysteine was added to each peptide to facilitate coupling to the antigenic carrier protein keyhole limpet hemocyanin. Peptide composition was confirmed by mass spectrometry and purified by HPLC prior to immunization. Hybridoma supernatants were screened by analyzing their ability to recognize native and recombinant protein on immunoblots and by immunofluorescence assay of P. gallinaceum ookinetes.
Recombinant Plasmepsin IV Expression
To express PgPM4, the post-signal peptide coding sequence (including the last 48 amino acids of the predicted proenzyme domain) was cloned into the expression vector pET3a and expressed in Escherichia coli BL21 cells as described previously for plasmepsin IV of other Plasmodium spp. (20). Refolding was carried out by rapid dilution of inclusion bodies into buffer of varying pH values. Autoactivation was assessed by SDS-PAGE after addition of 10 μm of pepstatin A and Laemmli sample buffer (50 mm Tris, pH 6.8, 6.3% SDS, 46% glycerol,; 7.8% 2-mercaptoethanol, 0.1% bromphenol blue).
Western Immunoblot Analysis
Purified P. gallinaceum zygotes and ookinetes, ookinete-condition medium, uninfected chicken blood, P. gallinaceum-infected chicken blood, mosquito midguts from mosquitoes fed with either an uninfected blood meal or P. gallinaceum-infected blood meal at 0, 24, and 48 h after blood meals, and rPgPM4 were subjected to Western immunoblot analysis.
Immunofluorescence Localization of PgPM4
Fixed, permeabilized P. gallinaceum zygotes, ookinetes, and asexual blood stage parasites were analyzed by IFA. Parasites were fixed on glass slides with 100% acetone at −20 °C for 20 min and then rehydrated by two changes of PBS for 5 min each at room temperature. For membrane permeabilization and blocking of nonspecific binding, fixed cells were incubated in PBS, 3% bovine serum albumin, 3% Triton X-100 for 1 h at room temperature. The preparations were then incubated with mAbs (1:1000 dilution) for 1 h at room temperature followed by Alexa Fluor 488 rabbit anti-mouse IgG (1:200 dilution) (Invitrogen).
For live IFA, staining was performed on ice. Live P. gallinaceum ookinetes were washed with PBS and incubated with mAbs (1:1000) for 1 h, washed with PBS, and incubated with Alexa Fluor 488 rabbit anti-mouse IgG (1:200). DNA was counterstained using 300 nm 4′,6-diamidino-2-phenylindole (Invitrogen), mounted onto glass slides, and covered with a drop of Gelvatol. Qdot quantum dot 655 was conjugated to mAb 1H10 against PgPM4 following the manufacturer's instructions (QDOT 655 antibody conjugation kit, Quantum Dot Corp., Hayward, CA). For dual staining, ookinetes were incubated with 10 nm Qdot 655-labeled anti-PgPM4 (mAb 1H10) for 1 h, followed by staining with mAb 1C3 against PgCHT2 for 1 h, followed by 10 nm secondary antibody Qdot 565 goat anti-mouse IgG conjugate. After slides were mounted in Gelvatol, fluorescence signals were observed and documented with a DeltaVision deconvolution fluorescence microscope system (Applied Precision, Inc., Issaquah, WA).
Inhibitor and mAb Effects on P. gallinaceum Ookinete Formation and PgPM4 Expression
Inhibitor experiments were performed with P. gallinaceum ookinetes obtained from in vitro culture. Zygotes were washed three times with M199 and then resuspended in ookinete medium with different amounts of aspartic protease-specific inhibitor pepstatin A, calpain inhibitors ALLN and ALLM, and mAbs raised against PgPM4, incubated at 26 °C. After 24 h of incubation, ookinete formation was checked by light microscopy as well as the ookinete-conditioned medium, and ookinete lysates were subjected to Western immunoblot.
Immunoelectron Microscopy Localization of PgPM4 in the P. gallinaceum Ookinete
Cells were fixed with 2.5% glutaraldehyde in 0.1 m cacodylate buffer for 2 h at room temperature, post-fixed in 1% OsO4 in 0.1 m cacodylate buffer (1 h) at room temperature, and embedded in LX-112 (Ladd Research, Williston, VT), as described previously (21). Cryosections were made, applied to grids, blocked in 1% bovine serum albumin in PBS for 1 h, incubated in 1:500 dilution of mAb against PgPM4, chitinase (1C3, against PgCHT2 (22)), or mAb isotype control, washed, and incubated with anti-mouse IgG conjugated to 5 nm colloidal gold particles. Stained sections were observed using a Philips CM-10 electron microscope.
RESULTS
Identification of P. gallinaceum Ookinete-secreted Plasmepsin 4 (PgPm4)
Conditioned medium from P. gallinaceum ookinetes cultured in chemically defined axenic culture medium (M199) was subjected to benzamidine-Sepharose affinity chromatography. The darkest band in SDS-PAGE analysis of elute was excised from the gel and subjected to de novo mass spectrometry sequencing (Fig. 1). Four peptide sequences were obtained and identified as a plasmepsin. BLAST searching showed that all four peptides were derived from a single plasmepsin gene. Independently, two-dimensional gel analysis of P. gallinaceum zygotes, ookinetes, and ookinete-secreted proteins identified PgPM4, which was also found in high throughput proteomic analysis of P. gallinaceum ookinetes (23). By searching the recently completed 3-fold coverage of P. gallinaceum genome, the four peptides were found to be encoded by a single gene, designated PgPM4 based on the highest degree of similarity to plasmepsin 4 of P. falciparum (PfPM4), P. vivax (PvPM4), Plasmodium ovale (PoPM4), and Plasmodium malariae (PmPM4) (supplemental Fig. 1B).
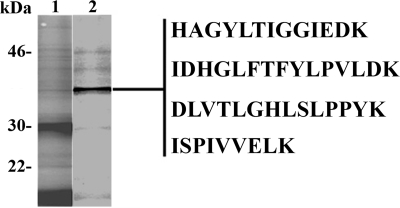
Identification of P. gallinaceum ookinete-secreted plasmepsin 4. SDS-polyacrylamide gel of unfractionated ookinete conditioned medium (lane 1) and the eluate (lane 2) from benzamidine affinity chromatography are shown. The band indicated was excised from the gel and submitted for de novo mass spectrometry sequencing. Four peptide sequences of P. gallinaceum plasmepsin 4 (as indicated by underlining/shading in supplemental Fig. 1B) were obtained. Molecular mass indicated at left in kDa.
Generation of Monoclonal Antibodies Specific for PgPM4
To generate mAbs against PgPM4, three peptides predicted to be immunogenic from the PgPM4 predicted open reading frame (see under “Materials and Methods”) were synthesized, purified by HPLC, confirmed by mass spectrometry, conjugated to keyhole limpet hemocyanin, and used to immunize mice and prepare hybridoma. Hybridoma supernatants were initially screened by enzyme-linked immunosorbent assay using bovine serum albumin-conjugated synthetic peptides, with secondary screens using Western immunoblot against P. gallinaceum ookinete-conditioned medium and ookinete lysate and by IFA on fixed P. gallinaceum ookinetes. Final screening yielded two anti-PgPM4 mAbs, designated 1H10 and 2G4, that reacted against in vitro and in vivo produced P. gallinaceum ookinetes on Western blot and IFA (Figs. 2 and and3).3). Western immunoblot of P. gallinaceum ookinete lysate and conditioned medium showed a band of ~37 kDa, consistent with the predicted size of active enzyme PgPM4 and PM4 of the other Plasmodium species (15), and a band of ~51 kDa, the expected size of the proenzyme. 1H10 showed a stronger affinity for pro-plasmepsin 4 than 2G4, although 2G4 showed a stronger affinity for active enzyme (data not shown). The specificity of the mAbs was also determined by the recognition of the most intense band found in the benzamidine column eluate of ookinete-conditioned medium (data not shown).
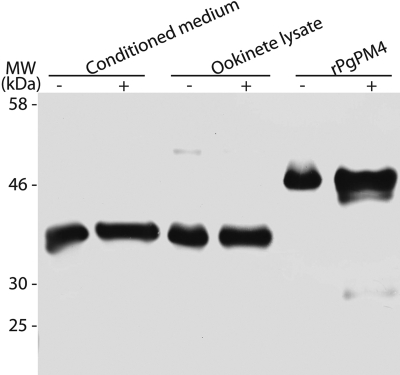
Western immunoblot demonstrating the recognition of recombinant PgPM4 and plasmepsin 4 (PgPM4) in P. gallinaceum ookinete conditioned medium and lysate by mAb 1H10. −, unreduced condition; +, reducing conditions with dithiothreitol.
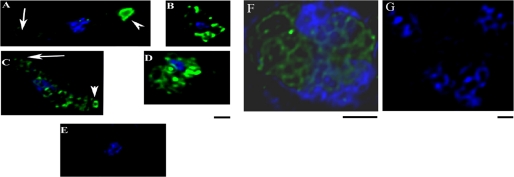
Localization of PgPM4 in P. gallinaceum zygote, ookinete, and asexual blood stages as demonstrated by deconvolution immunofluorescence microscopy. A, C, and E, ookinete. B and D, zygote. F and G, asexual stage parasite (trophozoite). A and B, fixed, permeabilized ookinetes and zygotes stained with mAb 2G4. C and D, surface staining of live ookinetes and zygotes using mAb 2G4. E, negative control using isotype IgG2b as primary antibody. F and G, fixed, permeabilized trophozoite. F, stained by mAb 2G4. G, negative control using isotype IgG2b as primary antibody. Blue, 4′,6-diamidino-2-phenylindole staining of nucleus. Scale bar, 1 μm in all panels.
Recombinant PgPM4 Protein Expression in E. coli and Confirmation of mAb Specificity
To confirm the reactivity of mAbs 1H10 and 2G4 against PgPM4, rPgPM4 was produced as a nonfusion pro-plasmepsin protein. The complete coding sequence starting at 48 amino acids of the post-signal proenzyme domain was cloned into the pET3a expression vector, and protein expression was carried out in E. coli BL21 (DE3) cells (15). The recombinant protein was purified as inclusion bodies and refolded by rapid dilution. Change of pH confirmed autocatalytic activation of rPgPM4 at pH 4.5 (data not shown). As determined by Western immunoblot, both mAbs 1H10 (Fig. 2) and 2G4 reacted with rPgPM4, confirming their specificity. Compared with native proteins, both mAbs recognized a smaller recombinant active enzyme, which suggested that the E. coli cleaved the active enzyme at some unknown sites.
Immunolocalization of PgPM4 and Co-localization of PgPM4 with Chitinase (PgCHT2)
Western immunoblot analysis was carried out to determine the presence of PgPM4 in the different life cycle stages of P. gallinaceum. mAbs 1H10 and 2G4 (both isotype IgG2b) recognized the predicted 37-kDa protein in asexual blood stages, zygotes and ookinetes, and detected a fainter band of 51 kDa in zygote and ookinete (Fig. 4). As determined by indirect immunofluorescence analysis of P. gallinaceum ookinetes, both mAbs 1H10 and 2G4 delineated the presence of PgPM4 on the apical surface of ookinete (Fig. 3A), consistent with a putative role of this protein in mosquito midgut invasion. Zygotes had no obvious polarization of PgPM4 protein (Fig. 3B). As a negative control, an irrelevant (anti-dengue virus), isotype control mAb did not yield fluorescent signals in any parasite stage (Fig. 3E). To determine whether PgPM4 was on the surface of the ookinete, live staining without permeabilization was carried out. 1H10 and 2G4 mAbs recognized a surface-localized, bilaterally symmetric secretory structure on the apical surface of the ookinete (Fig. 3C), similar to the configuration for the second P. gallinaceum chitinase, PgCHT2. To determine whether PgPM4 and the chitinase PgCHT2 were similarly localized on the ookinete apical surface, dual staining with mAbs 1H10/2G4 and the anti-chitinase PgCHT2 (mAb 1C3) was carried out on live ookinetes. Deconvolution immunofluorescence microscopy showed that PgPM4 and P. gallinaceum chitinase PgCHT2 are both localized on the ookinete apical surface (Fig. 5). This finding, reminiscent of a drill bit, suggests a role for PgPM4 in ookinete invasion of the midgut, particularly at the level of the chitin- and protein-containing peritrophic matrix. Immunoelectron microscopy demonstrated the presence of PgPM4 within ookinete micronemes (Fig. 6), similar to the subcellular location of chitinase and other known ookinete proteins such as CTRP (24, 25) and WARP involved in invasion of ookinete (8, 26, 27).
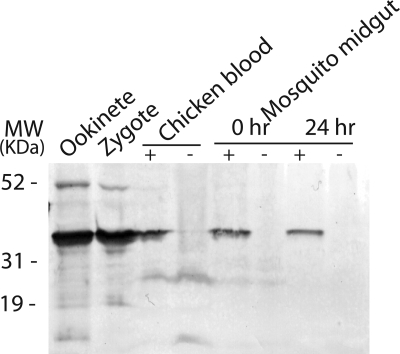
Western immunoblot demonstrating the presence of plasmepsin 4 (PgPM4) in P. gallinaceum zygotes, ookinetes, and asexual blood stage probed with mAb 1H10 that recognizes PgPM4. Ookinete lysate (1st lane, 2.5 × 103 parasites loaded), zygote lysate (2nd lane, 2.5 × 103 parasites loaded), P. gallinaceum-infected chicken blood lysate (3rd lane, 10 μl of blood loaded), uninfected chicken blood lysate (4th lane, 10 μl of blood loaded), A. aegypti midgut lysate at 0 h after blood meal (5th lane, infected; 6th lane, uninfected; 1 midgut/lane) and at 24 h (7th lane, infected; 8th lane, uninfected; 1 midgut/lane).
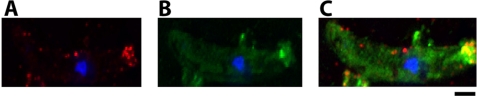
Surface localization of plasmepsin 4 in relation to the chitinase PgCHT2 on the apical surface of the P. gallinaceum ookinete. Live, nonpermeabilized ookinetes were subjected to live IFA with dual staining of PgPM4 and PgCHT2. A, PgPM4 detected by mAb 1H10; B, PgCHT2 detected by mAb 1C3; C, PgPM4 stained with QDot 655 streptavidin-conjugated mAb 1H10 (red) and PgCHT2 recognized by mAb 1C3 stained with QDot 565 goat anti-mouse IgG (green). Blue, 4′,6-diamidino-2-phenylindole staining of nucleus. This pattern of staining was reproducibly observed in the vast majority of mature, elongated P. gallinaceum ookinetes. Scale bar, 1 μm in all panels.
Effect of Inhibitors and mAbs on P. gallinaceum Ookinete Formation and PgPM4 Expression
Different concentrations of pepstatin A, calpain inhibitors ALLN and ALLM, and mAbs 1H10 and 2G4 were added to the P. gallinaceum ookinete in vitro culture for 24 h. Compared with negative control, a lower concentration (1 μm) of ALLM reduced ookinete formation significantly, and a higher concentration (3–20 μm) blocked ookinete formation completely (Fig. 7A). For ALLN, only a higher concentration (10 and 20 μm) reduced ookinete formation significantly (Fig. 7A). In contrast, pepstatin A and mAbs had no effect on ookinete formation (Fig. 7). As determined by immunoblot analysis, the processing of pro-plasmepsin 4 at both the ookinete-conditioned medium and ookinete lysate was not impaired by enzyme inhibitors or mAbs (data not shown).
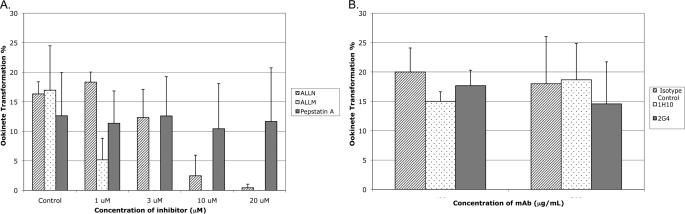
Effect of inhibitors and monoclonal antibodies on in vitro P. gallinaceum ookinete development. A, effect of calpain-like protease inhibitors ALLN and ALLM and pepstatin A on P. gallinaceum ookinete formation in vitro. B, effect of anti-P. gallinaceum plasmepsin IV monoclonal antibodies 1H10 and 2G4 on P. gallinaceum ookinete formation in vitro.
Transmission Blocking Activity of Plasmepsin Inhibitors and Antibodies against PgPM4
Complementary approaches were taken to assess the role of plasmepsins in P. gallinaceum infectivity for A. aegypti mosquitoes. Mosquitoes were fed on plasmepsin inhibitors and antibodies against PgPM4 to address the role of this protease to block or interfere with oocyst development in the midgut. Mosquitoes were allowed to take a blood meal from P. gallinaceum-infected chicken blood mixed with plasmepsin inhibitors and antibodies using a membrane feeder. The midguts were dissected, and oocysts were enumerated 8 days after feeding. Infection rates of mosquitoes, geometric mean number of oocysts on the midgut, and percentage transmission blockade are shown in Tables 1 and and2.2. Compared with negative control mosquitoes, aspartic protease-specific inhibitors (pepstatin A, SQ 3000, and Po/vPM) as well as PgPM4 mAbs dramatically blocked oocyst development in a dose-dependent manner. The mosquitoes fed on inhibitors had lower infection rates and significantly reduced (Mann-Whitney's U test; p < 0.01) oocyst number compared with mosquitoes in the control groups. Plasmepsin convertase inhibitors ALLN and ALLM (28) reduce oocyst intensities and infection rate compared with negative control mosquitoes; doses of 1–20 μm ALLM and 20 μm ALLN significantly reduced the infectivity of P. gallinaceum to A. aegypti mosquitoes (p < 0.01). Compared with isotype control, PgPM4 mAbs (Both 1H10 and 2G4) significantly prevented the formation of oocysts in mosquitoes in a dose-dependent manner. A dose of 100 μg/ml 1H10 and 50–200 μg/ml 2G4 resulted in a significant reduction in the percentage of mosquitoes with oocysts and the number of oocysts per infected mosquito. The transmission blocking activities of combining mAb against chitinase, PgCHT2 (1C3) and PgPM4, are shown in Table 3. Compared with the mosquitoes fed on individual mAb, the mosquitoes fed on the combination of 1C3 and 1H10/2G4 had lower oocyst number and infection rate.
TABLE 1
Transmission blocking activity of aspartic protease inhibitors and calpain-like plasmepsin convertase inhibitors on P. gallinaceum infection of A. aegypti mosquitoes
Control is 0.5–1% DMSO; inhibitors are as follows: pepstatin A (Pep A), SQ 3000, Po/vPM, ALLN (calpain inhibitor I), and ALLM (calpain inhibitor II). Statistical significance was determined by Mann-Whitney U test. Results were considered statistically significant with a p value ≤0.01. 200 μl of P. gallinaceum-infected chicken blood was placed into each membrane feeder, with final concentration of inhibitors as noted. Mosquitoes were dissected 8 days after infection. Each protease inhibitor was tested in an independent experiment and is compared against solvent (DMSO) alone as a negative control. Each protease inhibitor experiment was performed of five independent experiments with comparable results.
Treatment | Geometric mean no. of oocysts | Range (oocysts) | No. of infected/no. of dissected | p value |
---|---|---|---|---|
% | ||||
1% DMSO | 6.86 | 0–143 | 38/40 (95.0) | |
0.1 μm Pep A | 3.48 | 0–125 | 19/40 (47.5) | 0.01 |
1.0 μm Pep A | 2.12 | 0–114 | 19/40 (47.5) | <0.01 |
3.0 μm Pep A | 1.30 | 0–32 | 16/40 (40.0) | <0.01 |
10 μm Pep A | 1.22 | 0–92 | 14/40 (35.0) | <0.01 |
20 μm Pep A | 0.35 | 0–20 | 9/40 (22.5) | <0.01 |
0.5% DMSO | 21.19 | 0–209 | 37/39 (94.9) | |
0.1 μm SQ 3000 | 8.16 | 0–136 | 32/37 (86.5) | 0.01 |
1.0 μm SQ 3000 | 3.04 | 0–85 | 22/40 (55.0) | <0.01 |
10 μm SQ 3000 | 0.69 | 0–24 | 11/40 (27.5) | <0.01 |
20 μm SQ 3000 | 0.03 | 0–2 | 1/40 (2.5) | <0.01 |
0.5% DMSO | 12.84 | 0–148 | 34/40 (85.0) | |
0.1 μm Po/vPM | 4.97 | 0–69 | 25/32 (78.1) | 0.01 |
1.0 μm Po/vPM | 5.85 | 0–115 | 28/36 (77.8) | 0.04 |
10 μm Po/vPM | 2.22 | 0–68 | 16/33 (48.5) | <0.01 |
20 μm Po/vPM | 2.04 | 0–74 | 19/33 (57.6) | <0.01 |
0.5% DMSO | 16.05 | 1–87 | 40/40 (100.0) | |
0.1 μm ALLN | 10.28 | 0–49 | 36/40 (90.0) | 0.09 |
1.0 μm ALLN | 10.47 | 0–107 | 39/40 (97.5) | 0.05 |
10 μm ALLN | 9.78 | 0–75 | 36/40 (90.0) | 0.06 |
20 μm ALLN | 2.14 | 0–34 | 25/40 (62.5) | <0.01 |
0.5% DMSO | 13.22 | 1–115 | 37/40 (92.5) | |
0.1 μm ALLM | 9.85 | 1–154 | 27/40 (67.5) | 0.13 |
1.0 μm ALLM | 3.75 | 0–47 | 27/40 (67.5) | <0.01 |
10 μm ALLM | 5.75 | 0–56 | 26/40 (65.0) | <0.01 |
20 μm ALLM | 3.32 | 0–111 | 35/40 (87.5) | <0.01 |
TABLE 2
Effect of mAbs raised to PgPM4 on P. gallinaceum infectivity to A. aegypti mosquitoes
Control is isotype IgG2b; mAbs are 1H10 and 2G4 raised against PgPM4. Statistical significance was determined by Mann-Whitney U test. Results were considered statistically significant with a p value ≤0.01. 200 μl of P. gallinaceum-infected chicken blood was placed into each membrane feeder, with final concentration of mAbs as noted. Mosquitoes were dissected 8 days after infection. Each monoclonal antibody was tested in an independent experiment and within each experiment was compared against an isotype control monoclonal antibody with specificity against a non-Plasmodium protein alone as a negative control. Each monoclonal antibody was tested in five independent membrane feeding experiments with comparable results.
Treatment | Geometric mean no. of oocysts | Range (oocysts) | No. of infected/no. of dissected | p value |
---|---|---|---|---|
% | ||||
50 μg/ml isotype control | 17.95 | 0–105 | 36/40 (90.0) | |
100 μg/ml isotype control | 16.43 | 0–102 | 36/40 (90.0) | 0.40 |
200 μg/ml isotype control | 15.00 | 0–129 | 37/40 (92.5) | 0.32 |
50 μg/ml mAb 1H10 | 17.22 | 0–143 | 36/40 (90.0) | 0.37 |
100 μg/ml mAb 1H10 | 7.14 | 0–96 | 28/40 (70.0) | 0.01 |
200 μg/ml mAb 1H10 | 3.83 | 0–57 | 25/40 (62.5) | <0.01 |
50 μg/ml isotype control | 4.41 | 0–147 | 29/40 (72.5) | |
100 μg/ml isotype control | 2.69 | 0–75 | 23/40 (57.5) | 0.10 |
200 μg/ml isotype control | 2.44 | 0–75 | 23/40 (57.5) | 0.07 |
50 μg/ml mAb 2G4 | 1.84 | 0–31 | 18/40 (45.0) | 0.01 |
100 μg/ml mAb 2G4 | 0.72 | 0–22 | 11/40 (27.5) | <0.01 |
200 μg/ml mAb 2G4 | 0.02 | 0–1 | 1/40 (2.5) | <0.01 |
TABLE 3
Synergistic effect of combining mAbs against PgCHT2 and PgPM4 on P. gallinaceum infectivity to Ae. Aegypti mosquitoes
Control: Isotype IgG2b, mAb: 1C3 raised against P. gallinaceum chitinase PgCHT2, 1H10 and 2G4 raised against PgPM4. Statistical significance determined by Mann-Whitney U test. Results considered statistically significant with a p value ≤0.01. 200 μl of P. gallinaceum-infected chicken blood was placed into each membrane feeder, with final concentration of mAbs as noted. Mosquitoes were dissected 8 days after infection. The data are representative of five independent experiments.
Treatment | Geometric mean no. of oocysts | Range (oocysts) | No. of infected/no. of dissected | p value |
---|---|---|---|---|
% | ||||
100 μg/ml isotype control | 11.38 | 0–192 | 33/40 (82.5) | |
100 μg/ml 1C3 | 4.33 | 0–110 | 24/40 (60.0) | 0.01 |
100 μg/ml mAb 1H10 | 7.79 | 0–165 | 36/40 (90.0) | 0.1 |
100 μg/ml mAb 2G4 | 3.67 | 0–56 | 26/40 (65.0) | 0.005 |
50 μg/ml 1C3 + 50 μg/ml 1H10 | 3.83 | 0–76 | 27/40 (67.5) | 0.005 |
50 μg/ml 1C3 + 50 μg/ml 2G4 | 0.84 | 0–45 | 12/40 (30.0) | 0.0000005 |
DISCUSSION
Here, we demonstrate that the Plasmodium ookinete-expressed plasmepsin, PgPM4, plays a role in parasite invasion of the mosquito midgut and is involved somewhere along the pathway along which ookinetes develop into oocysts. These observations are the first to demonstrate, at the protein level, the role of a plasmepsin expressed by the mosquito midgut stages of the malaria parasite, although gene expression and proteomic profiling have shown the presence of plasmepsin gene products in the sexual stages (16, 17). In the avian-infecting malaria parasite P. gallinaceum, both PgPM4 and the chitinase PgCHT2 are present in micronemes, consistent with their roles as secreted proteins, and both proteins are present on the apical surface of the ookinete. Collectively, these observations suggest the possibility that both chitinase and PgPM4 are involved in penetrating the chitin- and peritrophin-containing peritrophic matrix. Alternatively, PgPM4 might have additional protease functions involved in parasite-mosquito midgut interactions other than at the level of the peritrophic matrix, such as crossing the epithelial surface or cleaving other parasite or mosquito protein targets. Further experiments are needed to distinguish among these possibilities.
The biological importance of plasmepsin 4 was demonstrated in experimental mosquito infections using membrane feeding experiments that incorporated plasmepsin inhibitors and anti-plasmepsin 4 monoclonal antibodies into the infectious blood meal. The data from these experiments provided further evidence of the importance of plasmepsin 4 in parasite-mosquito interactions and strongly suggested its role as a potential target of new transmission blocking strategies.
In the process of midgut invasion, the ookinete must cross a series of physical barriers, the first of which is the chitin- and peritrophin-containing peritrophic matrix, followed by the epithelial surface. A series of ookinete-expressed proteins have been reported to be critical for host cell recognition, binding, and motility and have been involved in passage of the ookinete through the mosquito midgut (8, 26, 29, 30, 33, 34), none of which have been demonstrated to be proteases. Plasmepsins (PM1, PM2, PM4, and HAP in P. falciparum but only PM4 in other Plasmodium spp. (15)) have previously only been reported to be present in blood stage parasites. These proteases, localized to the digestive vacuole of the asexual blood stage parasites, participate in the metabolism of hemoglobin and perform other lysosomal functions (14, 35, 36). Our data, based upon experiments of an avian model of malaria transmission, P. gallinaceum, suggest a novel role of plasmepsin 4 in the malaria parasite life cycle.
Remarkably, little is known about transport and trafficking of secretory proteins in Plasmodium ookinete stages. Apicomplexan parasites, although morphologically defined based on the apical structure, characteristically have three types of secretory organelles found in Plasmodium in merozoites and sporozoites but not ookinetes (which only have micronemes) as follows: rhoptries (involved in invasion and formation of parasitophorous vacuole); dense granules (thought to be involved in maintenance of the parasitophorous vacuole); and micronemes (involved in the secretion of both soluble and transmembrane proteins). Transmission-blocking targets such as chitinase, CTRP, and WARP are found in micronemes and are discharged onto the ookinete surface to facilitate invasion of the mosquito midgut (8, 25, 37). The micronemal localization and prominent apical staining pattern for PgPM4 and co-localization with chitinase, as demonstrated by deconvolution immunofluorescence microscopy, imply that these enzymes synergize in mosquito invasion at the level of the peritrophic matrix. This interpretation is further supported by P. gallinaceum membrane feeding experiments in which the combination of mAbs raised against PgCHT2 and PgPM4 reduces the oocyst number significantly compared with using either one of them individually. Again, the mechanism by which plasmepsin 4 is involved in ookinete infection of the mosquito has not been definitely established but must be experimentally distinguished from other potential protein and structural targets with which the ookinete comes into contact in the mosquito midgut.
One potential concern about the presence of plasmepsin 4 in the ookinete culture supernatant is that perhaps it arises either from contaminating asexual stage parasites or spontaneous lysis of ookinetes during in vitro culture. In the P. gallinaceum cultures we performed, significant numbers of asexual forms are not observed because the gradient centrifugation step eliminates this stage of parasite. It is formally possible that ookinetes lysed under suboptimal culture conditions to a degree sufficient to yield detectable plasmepsin 4 in the culture supernatant. The results presented indicate that plasmepsin 4 is present within micronemes and on the parasite surface, and therefore its presence in ookinete culture supernatant is not parsimoniously explained by parasite lysis.
Further evidence of the role of plasmepsin activity in parasite invasion of the mosquito midgut was suggested by the transmission blocking activity of aspartic protease-specific inhibitor pepstatin A, other peptidomimetic aspartic protease inhibitors, and calpain inhibitors.
A number of studies have revealed an effect of pepstatin and other aspartic protease inhibitors on asexual blood stage parasite, suggesting one or more of the plasmepsins are essential for the survival of the parasites (38,–41). However, these studies did not address whether plasmepsins or aspartic proteases may also be required for the parasite development outside the asexual stage. To determine the role of plasmepsins in midgut invasion and oocyst development, studies with pepstatin A and a variety of peptidomimetic and natural product aspartic protease inhibitors were performed using membrane feeding assays. As shown in Table 1, nontoxic concentrations of pepstatin A, SQ 3000, and Po/vPM led to inhibited oocyst formation and reduced Plasmodium infectivity to the mosquito in a dose-dependent manner. The effective concentration started as low as 0.1 μm. Consistent with the results of pepstatin A on asexual stages parasites (28), immunoblot experiments on in vitro culture of ookinetes incubated with pepstatin A demonstrated that pepstatin A did not interfere with the processing of pro-plasmepsin 4, suggesting blocking of pro-plasmepsin 4 processing requires not only aspartic protease inhibitors but also other inhibitors, such as cysteine protease inhibitors, like what happened at asexual blood stages (28). Furthermore, ookinete formation assay revealed that pepstatin A had no effect on the development of zygote to ookinetes in ookinete culture in vitro. These results suggest that PgPM4 was involved in penetration of peritrophic matrix/epithelial membrane and/or development of ookinete to oocyst.
ALLN (calpain inhibitor I) and ALLM (calpain inhibitor II), inhibitors of the Ca2+-dependent neutral cysteine protease calpain I and calpain II, are also thought to be plasmepsin convertase inhibitors (28). Previous studies have shown that at asexual stages calpain inhibitors I and II prevent activation of Plasmodium aspartic proteases (28) through inhibition of falcipain (31). However, our data indicate that although activation of pro-plasmepsin is unaffected by the addition of ALLN or ALLM to in vitro P. gallinaceum zygote cultures, membrane feeding experiments demonstrated that ALLM and high dose (20 μm) ALLN reduced oocyst numbers significantly. Calpain inhibitors did not interfere with the activation of the pro-plasmepsin 4 during the development of zygote to ookinete (data not shown), but calpain II and high concentrations of calpain I remarkably reduced ookinete formation and even block the ookinete development. It was obvious that the processing of pro-plasmepsin 4 occurred previous to the zygote stage, and some calpain-like proteins (other than PgPM4), which were critical for the development of zygote to ookinete, were inhibited by calpain inhibitors. In vitro, the activation of the rPgPM4 was pH-dependent and autocatalytically activated at pH 4.5. It is possible that in the ookinete plasmepsins traffic through the secretory organelle, microneme, to the plasma membrane of the parasite. However, it is not clear where the processing of pro-plasmepsin 4 takes place and whether an acidic pH is required inside the mosquito midgut. Western immunoblot experiments showed that PgPM4 found in the ookinete-conditioned medium is a catalytically active enzyme identical to that in the lysate of ookinete and zygote. There was no difference of PgPM4 expression patterns between conditioned medium/lysate under reduced and unreduced conditions (Fig. 2). The immunoelectron microscopy results demonstrated that PgPM4 was present in the microneme of ookinete, indicating it may be secreted via the microneme. The pH of the microneme remains unknown; however, it is possible that the pro-plasmepsin 4 is activated within the microneme prior to secretion. Alternatively, the activation/processing of pro-plasmepsin 4 may occur in acidic vacuoles in the zygote before secretion; EM micrographs have revealed many previously unreported cellular organelles in P. falciparum3 or mediated by other proteases at neutral pH after secretion. This interpretation is supported by evidence that P. falciparum plasmepsin PfPM2 not only digests hemoglobin in the food vacuole during its asexual proliferation in the erythrocytes but is also involved in remodeling the erythrocyte cytoskeleton by cleaving spectrin at neutral pH (32).
Because it is possible that these inhibitors are affecting mosquito-secreted as well as ookinete-secreted proteases, we repeated these studies using monoclonal antibodies that specifically recognize ookinete-secreted plasmepsins. Comparison of both the mAbs and polyclonal antibody raised against PgPM4 with negative controls using isotype control or normal mouse serum, we demonstrate that anti-PM4 antibodies markedly reduced oocyst number and infection rate in a reproducible, dose-dependent manner. The concentration of mAb 2G4 that started to affect the transmission was as low as 50 μg/ml, consistent with the Western immunoblot result that showed that 2G4 had a stronger affinity for the mature enzyme, suggesting the efficiency and specificity of the mAb. Compared with negative control, the mAbs had no effect on ookinete formation and the processing of pro-plasmepsin 4 in the ookinete culture in vitro. These results suggested a possible role of PgPM4 involved in the mosquito midgut invasion and/or development of oocyst from ookinete. The experiments here indicate an important role of plasmepsin 4 in the molecular interaction of the malaria parasite with the mosquito midgut and/or the development of ookinete to oocyst. Further delineation of the mechanisms by which Plasmodium ookinete-secreted PM4 facilitates parasite invasion of the mosquito vector and/or development in mosquito midgut may suggest novel approaches to malaria control.
Acknowledgments
We thank Dr. C. C. King for help in proteomic analysis of two-dimensional gels; Professor James Feramisco and Brendan Brinkmann for assistance in deconvolution immunofluorescence microscopy; and Professor Marilyn Farquhar and Krystyna Kudlicka for their help in the immunoelectron microscopy.
*This work was supported, in whole or in part, by National Institutes of Health Grant AI45999 from the USPHS.
The on-line version of this article (available at http://www.jbc.org) contains supplemental Fig. 1.
3V. Bounkeua and F. Li, unpublished data.
2The abbreviations used are:
- PgPM4
- P. gallinaceum plasmepsin 4
- IFA
- indirect immunofluorescence assay
- mAb
- monoclonal antibody
- ALLN
- calpain inhibitor I (sequence N-acetyl-Leu-Leu-norleucinal (N-acetyl-Leu-Leu-Nle-CHO)
- ALLM
- calpain inhibitor II (sequence N-acetyl-Leu-Leu-Met-CHO)
- PBS
- phosphate-buffered saline
- HPLC
- high pressure liquid chromatography
- CHAPS
- 3-[(3-cholamidopropyl)dimethylammonio]-1-propanesulfonic acid
- rPgPM4
- recombinant PgPM4.
REFERENCES
Articles from The Journal of Biological Chemistry are provided here courtesy of American Society for Biochemistry and Molecular Biology
Full text links
Read article at publisher's site: https://doi.org/10.1074/jbc.m109.063388
Read article for free, from open access legal sources, via Unpaywall:
http://www.jbc.org/article/S0021925819644467/pdf
Free to read at intl.jbc.org
http://intl.jbc.org/cgi/content/abstract/285/11/8076
Free after 12 months at intl.jbc.org
http://intl.jbc.org/cgi/reprint/285/11/8076.pdf
Free after 12 months at intl.jbc.org
http://intl.jbc.org/cgi/content/full/285/11/8076
Citations & impact
Impact metrics
Citations of article over time
Article citations
The complexities of blood-feeding patterns in mosquitoes and sandflies and the burden of disease: A minireview.
Vet Med Sci, 10(5):e1580, 01 Sep 2024
Cited by: 0 articles | PMID: 39171609 | PMCID: PMC11339650
Review Free full text in Europe PMC
Host-parasite interactions during Plasmodium infection: Implications for immunotherapies.
Front Immunol, 13:1091961, 04 Jan 2023
Cited by: 8 articles | PMID: 36685595 | PMCID: PMC9845897
Review Free full text in Europe PMC
Transmission-Blocking Strategies Against Malaria Parasites During Their Mosquito Stages.
Front Cell Infect Microbiol, 12:820650, 16 Feb 2022
Cited by: 14 articles | PMID: 35252033 | PMCID: PMC8889032
Review Free full text in Europe PMC
Evolutionary Insights into the Microneme-Secreted, Chitinase-Containing High-Molecular-Weight Protein Complexes Involved in Plasmodium Invasion of the Mosquito Midgut.
Infect Immun, 90(1):e0031421, 04 Oct 2021
Cited by: 4 articles | PMID: 34606368 | PMCID: PMC8788677
Review Free full text in Europe PMC
A Hetero-Multimeric Chitinase-Containing Plasmodium falciparum and Plasmodium gallinaceum Ookinete-Secreted Protein Complex Involved in Mosquito Midgut Invasion.
Front Cell Infect Microbiol, 10:615343, 08 Jan 2021
Cited by: 4 articles | PMID: 33489941 | PMCID: PMC7821095
Go to all (14) article citations
Data
Data behind the article
This data has been text mined from the article, or deposited into data resources.
BioStudies: supplemental material and supporting data
Similar Articles
To arrive at the top five similar articles we use a word-weighted algorithm to compare words from the Title and Abstract of each citation.
Plasmodium falciparum ookinete expression of plasmepsin VII and plasmepsin X.
Malar J, 15:111, 24 Feb 2016
Cited by: 19 articles | PMID: 26911483 | PMCID: PMC4765185
A Hetero-Multimeric Chitinase-Containing Plasmodium falciparum and Plasmodium gallinaceum Ookinete-Secreted Protein Complex Involved in Mosquito Midgut Invasion.
Front Cell Infect Microbiol, 10:615343, 08 Jan 2021
Cited by: 4 articles | PMID: 33489941 | PMCID: PMC7821095
Identification of novel Plasmodium gallinaceum zygote- and ookinete-expressed proteins as targets for blocking malaria transmission.
Infect Immun, 70(1):102-106, 01 Jan 2002
Cited by: 15 articles | PMID: 11748169 | PMCID: PMC127631
Do malaria ookinete surface proteins P25 and P28 mediate parasite entry into mosquito midgut epithelial cells?
Malar J, 4:15, 25 Feb 2005
Cited by: 29 articles | PMID: 15733320 | PMCID: PMC555762
Review Free full text in Europe PMC
Funding
Funders who supported this work.
NIAID NIH HHS (2)
Grant ID: AI45999
Grant ID: R01 AI045999