Abstract
Free full text

Exosomes generated from iPSC-derivatives: new direction for stem cell therapy in human heart diseases
Abstract
Cardiovascular disease (CVD) is the leading cause of death in modern society. The adult heart innately lacks the capacity to repair and regenerate the damaged myocardium from ischemic injury. Limited understanding of cardiac tissue repair process hampers the development of effective therapeutic solutions to treat CVD such as ischemic cardiomyopathy. In recent years, rapid emergence of induced pluripotent stem cells (iPSC) and iPSC-derived cardiomyocytes (iCM) presents a valuable opportunity to replenish the functional cells to the heart. The therapeutic effects of iPSC-derived cells have been investigated in many preclinical studies. However, the underlying mechanisms of iPSC-derived cell therapy are still unclear and limited engraftment of iCMs are well known. One facet of their mechanism is the paracrine effect of the transplanted cells. Microvesicles such as exosomes secreted from the iCMs exert protective effects by transfering the endogenous molecules to salvage the injured neighboring cells by regulating apoptosis, inflammation, fibrosis and angiogenesis. In this review, we will focus on the current advances in the exosomes from iPSC-derivatives and discuss their therapeutic potential in the treatment of CVD.
Since the discovery of induced pluripotent stem cells (iPSCs) by Yamanaka in 20061, the stem cell field has made remarkable progress. The challenges of immune rejection and the ethical consideration of human embryonic stem cells (hESCs) were obviated by the advent of somatic cell reprogramming. Over the years, scientific advances in the iPSC technology have addressed the critical biological issues, including the viral delivery system, transgene integration, and random mutagenesis2. However, significant challenges still remain in clinical translation of the revolutionary iPSC biology.
Cardiovascular diseases (CVDs) are still the leading cause of death worldwide despite significant global research effort. This reality necessitates the introduction of novel intervention for effective detection, prevention, and treatment. Stem cells have been broadly employed to address some of these complex issues during the past two decades. Although the derivation of iPSCs provide autologous pluripotent stem cells, salient difficulties related to cell retention, engraftment, tumorigenesis3, and ventricular arrhythmia4,5 in the ischemic myocardium remain as one of the major challenges for their clinical translation. Extensive studies using various stem cells, including hESCs6 and iPSCs7,8, mesenchymal stem cells (MSCs)9,10, adipose stem cells (ASCs)11–13, and cardiac progenitor cells (CPCs)14–16 have been attempted. All these approaches have their own unique benefits and limitations. Studies with beneficial effects are mostly recognized by their paracrine effects or actions of the cytokines generated from the transplanted cells. Therefore, many research has shifted the focus to the secretomes from the transplanted stem cells17–19.
It is well established that the cellular secretomes transfer various bioactive information among the cells20,21. These cell-secreted extracellular vesicles (EVs) comprise an essential component of intercellular exchange and has pleiotropic functions. Specifically, the exosomes, a subset of these vesicles, are considered critical vehicles for intercellular communication in the human body. Exosomes were classified to be originating from the endosomal compartment or the microvesicles of the cellular membrane, resulting in the budding multivesicular bodies (MVBs)22. In recent years, numerous scientific investigations identified the application of exosomes in the development of molecular diagnostics, drug delivery systems and therapeutic agents. The transferred information has regulatory effects on the genetic and epigenetic processes in the recipient cells23. Exosomes have been proposed to present beneficial effects in stimulating or signaling pathways for endogenous repair in human cardiovascular disease (CVD)24, particularly in mediating the pro-angiogenic, proliferative, anti-apoptotic, and anti-inflammatory actions25–31 through miRNA, small molecule, and protein cargo, facilitating intercellular communication32. Exosomes appear to mediate many therapeutic properties of the stem cells when administered into the heart33,34. In CVDs, exosomal transfer of miRNAs provides a molecular gauge of the microenvironment of the pathology of interest. They have been considered as the promising targets or effectors for CVD-associated therapeutics for many years35. In myocardial ischemia or fibrosis, paracrine or endocrine transfer of miRNA-containing exosomes into cardiomyocytes or active fibroblasts have been demonstrated36. These studies provide interesting insights into the future of precision medicine where the application of exosomes generated from the autologous cells provide a novel agent for personalized therapy by simulating endogenous repair. In this review, we will discuss the role of exosomes generated from autologous iPSC-derivatives in treating the human heart diseases. Their potential diagnostic and therapeutic promises are examined.
1. The biogenesis of exosomes
The unique role of exosomes was originally recognized in the cancer microenvironment where the cancer cell–released exosomes regulated their invasive, metastatic, and angiogenic potential37. These exosomes determine the delivery of DNA and RNA fragments, enzymes, and growth factors, which all impact the surrounding cellular niche38. Various physiologic factors and cellular conditions influence the release and payload of these exosomes such as intracellular levels of calcium, cellular energetics, membrane phospholipid component, membrane-acting enzymes, cytoskeleton–membrane interactions, and other effectors of exocytosis, hypoxia, and oxidative stresses39,40. The size of exosomes is between 30-150nm, and their membranes usually contain high levels of sphingomyelin, cholesterol, phosphatidylserine and tetraspanins. They are associated with a variety of transmembrane and cytosolic proteins, including integrins CD9, CD63, and CD81 to form specific complexes41. Exosomes expressing these complexes are readily internalized by specific target cell ligands42. Furthermore, these unique bi-lipid membranes of exosomes not only have the ability to protect the contents from degradation enzymes but also enable targeted delivery of large cargo43,44 (Figure 1).
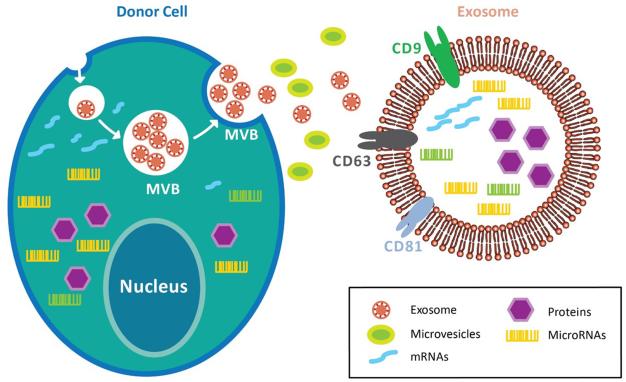
Exosomes are compartmentalized into the multivesicular bodies (MVBs), which fuse with the cell membrane and released to the extracellular space. This process prevents exosomes from degradation by the lysosomes. Exosomes comprise of various transmembrane and cytosolic proteins, such as integrins, CD9, CD63, and CD81. Furthermore, exosomes retain donor cells’ proteins, DNA fragments, miRNAs, and non-coding RNAs within the bi-lipid membrane. As such, exosomes preserve the genetic information of donor cells from enzymatic degradation while enabling targeted delivery of specific cargo.
One of the main theories of the exosome uptake mechnisms is that exosomes can be endocytosed or internalized into an endocytic compartment from which they may undergo reverse-fusion to release their cargo into the cytoplasm of the recipient cell. After endocytosis, exosomes may subsequently fuse with the endosomal membrane or be targeted to lysosomes for degradation45. Another mechanism is through receptor–ligand-mediated interaction, which leads to exosome internalization46,47. The third likely mode is by fusion of the exosome membrane with the plasma membrane, releasing the cargo directly into the cytoplasm of the recipient cell48. Interestingly, several studies suggest that exosome release is strictly controlled by specific signaling pathways, which consequently regulates cellular miRNA release49,50. In addition, neutral sphingomyelinase 2, the rate-limiting enzyme in the conversion of sphingomyelin to ceramide, is a critical regulator of exosome biogenesis and secretion51. Another study demonstrated that Rab27 (GTPases) isoforms play essential roles in the exosome secretion pathway52. According to a recent study, molecular packaging of miRNA-loaded exosomes is controlled by the recognition of sequence motifs present in the miRNAs by the sumoylated protein hnRNPA2B153. Koppers-Lalic and colleagues showed that 3′-end-uridylated miRNA isoforms are selectively packaged into exosomes while 3′-end-adenylated miRNA isoforms remain relatively enriched in the original cells54. Exosomes are known to be capable of shuttling RNA between cells and greatly increase the complexity by which these cells communicate. Thus, the potential use of exosomes in a clinical setting includes their role as antigen-presenting vesicles to stimulate anti-inflammatory, anti-fibrotic, anti-immunogenic proliferative, or angiogenic effects55,56.
2. Exosomes in cardiovascular diseases
CVDs are leading cause of death worldwide, necessitating investigations into novel, safe, and effective therapeutic approaches. Various strategies for the transplantation of human stem cells or stem cell–based therapies to treat cardiac disease have been attempted57. In vivo mechanistic studies to date have not been forthcoming clinically because of the difficulty of the performing appropriate assays. Nevertheless, most of the researchers recognize the importance of the paracrine factors from the cells rather than the direct effects of the transplanted cells to repair or regenerate the injured tissue. Recently, many studies have provided evidence regarding the importance of exosomes and their miRNAs in cell–cell communication within the cardiovascular system58, specifically, from stem cells to cardiovascular cells59–61, and from the heart to bone marrow stem cells62. Exosomes and their miRNAs are also recognized as important regulators in cardiomyocytes, endothelial cells, vascular smooth muscle cells, platelets, and inflammatory cells, which contribute to the initiation and progression of atherosclerosis63. miRNAs retain strong stability, express tissue-specific pattern, and represent the corresponding body fluids. Particularly, several miRNAs, already identified in exosomes, play important roles in CVDs and stem cell trans-differentiation (Figure 2). Ekstrom and co-workers observed that the exosomes from mast cells carry selective miRNAs to bone marrow CD34+ progenitor cells64. Bang et al. reported that miRNAs are involved in the crosstalk between cardiac fibroblasts and cardiomyocytes. They demonstrated that the exosome-derived miRNA-21 is transported to cardiomyocytes, leading to cellular hypertrophy by affecting target genes, SORBS2 and PDLIM558. miRNA-150 delivered to endothelial cells enhances migration by the downregulation of c-Myb65. Apoptotic bodies are shown to transfer functional miRNA-126 to endothelial cells inducing CXCL12 expression, which are involved in the mobilization of progenitor cells and, therefore, play an anti-apoptotic role66. miRNA-126, miRNA-223, and miRNA-197 expression have been found to risk stratifty the predilection to myocardial infarction (MI)67. miRNA-133 is specifically expressed in cardiomyocytes68 and those undergoing controlled cardiac hypertrophy69. miRNA-133a is considered a strong diagnostic marker for acute MI and coronary artery stenosis70. Notably, several studies have shown that miR-133 is involved in direct cardiac reprogramming of adult cardiac fibroblasts71,72. These pleiotropic properties of the miRNAs contained in the exosomes could be leveraged to treat various forms of CVD.
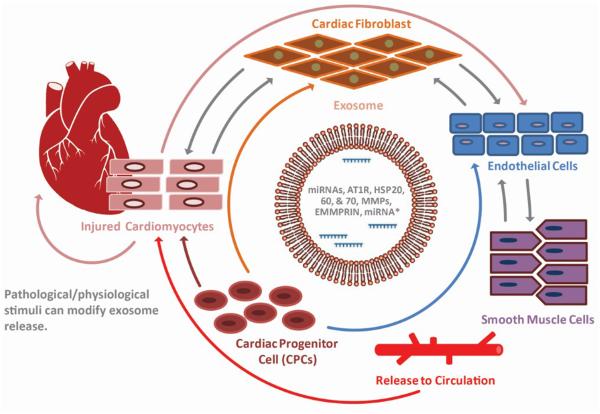
Exosomes facilitate communication amongst cardiomyocytes, endothelial cells, and vascular smooth muscle cells in the infarcted area of the heart. Exosomes transfer signaling molecules, such as miRNAs, mRNAs, and proteins to confer paracrine effects on the neighboring cells. In addition, pathological and physiological influence on the heart stimulates exosome secretion. Therefore, cardiac exosomes under pathological conditions could be utilized as ideal markers for diagnostic tools in the clinic.
3. Diagnostic capability of exosomes in heart injury
Biomarkers serve as indicators of normal biological functions, pathologic processes, or pharmacological responses to therapeutic intervention. As the direct assessment of biological states is often too invasive or costly, biomarkers have considerable clinical utility in identifying disease status and evaluating disease risk. Moreover, biomarkers allow for the early detection of pathology and subsequent therapy. As a diagnostic tool, for example, exosomes from prostate cancer cells can be obtained from a simple urine sample, making an exosome-based test essentially noninvasive. Exosome tests may detect several RNA-encoding key biomarkers in prostate cancer, such as PCA-3 and TMPRSS2:ERG. Other tumor markers can be added as they are identified and matched to a patient’s exosomal RNA profile. Initial clinical studies have shown that exosome tests for prostate cancer have a 70% accuracy rate, which is almost comparable to the accuracy of a biopsy73. For the implementation of these tests, the standard disease-specific antigen test could be hugely improved by incorporating exosome tests, which would be useful in the diagnosis and prognosis of diseases and the disease states that are difficult or inherently impossible to diagnose.
As exosomes are formed under specific conditions of stress or injury, circulating exosomes are being considered increasingly as candidates for CVD biomarkers. In particular, patients with atherosclerosis associated with vascular injury, inflammation, and prothrombotic state exhibit elevated plasma exosome levels. Several studies have shown an association between the Framingham risk score, used to predict cardiovascular disease risk, and circulating exosomes74,75. Their formation and clearance reflect a subtle balance between cell activation and damage; cell survival and apoptosis; and vascular remodeling and angiogenesis. Several investigations have demonstrated the selective packaging of miRNAs in the exosomes and their functional transfer by specific signaling molecules76,77. In addition, the exosomes facilitate the detection of endogenous processes for myocardial restoration, regeneration or protection78. These disease-specific expression patterns of exosomes from body fluids suggest the potential of exosomes as molecular markers and therapeutic targets for the treatment of CVDs.
Intercellular signals between endothelial and vascular smooth muscle cells are critical for the maintenance of blood vessel structure, vascular function, and blood pressure79 and for functional vessel formation after myocardial injury80. When exposed to high atheroprotective shear stress, endothelial cells secrete exosomes that are enriched in miRNA-143/145. Once transferred to smooth muscle cells, exosomes reduce the expression of miRNA-143/145 targets such as ELK1 and CAMK2D, the two central regulators of the smooth muscle cell phenotype81. In this respect, exosomes might serve as excellent diagnostic candidates in the clinical setting, given that they reflect the real-time microenvironment conditions of the injured lesions. Genetic information of cells in a target region can be achieved without invasive biopsy, requiring only blood or urine sample collection. This is a remarkable advantage, especially when the sample tissue is difficult to access, such as the human heart. Since exosomes can be collected in a straightforward way and with regularity, real-time tracking of a patient’s disease progression is possible.
4. Therapeutic effects of exosomes derived from the different sources of human stem cells
Due to the natural role in intercellular secretion and transport, exosomes have generated great interest as therapeutic tools. They are natural biologics with autologous origin while they maintain cargo integrity and stability. Furthermore, exosomal membranes contain certain proteins that have binding affinities to specific receptors on the surface of the recipient cells. Thus, they can selectively target cell types of interest and manipulate the molecular components through the miRNA cargo to provide promising therapeutic tools for personalized medicine and gene therapy35,47. More importantly, the exosomes shuttle the RNAs or exosomal shuttle RNAs (esRNAs) between cells, which serves as a novel and effective means to control cell–cell communication82,83.
Mesenchymal stem cell (MSC)-derived exosomes have been investigated in the field of cardiac regenerative medicine, especially in myocardial ischemia/reperfusion (I/R) injuries34,84. Exosomes bearing endothelial differentiation signals were found to effect neovascularization, indicating their usefulness in treating angiogenic defects. Exosomes from human umbilical cord mesenchymal stem cells (hucMSCs) have been reported to promote heart repair following ischemic injury by protecting myocardial cells from apoptosis and promoting cell proliferation and angiogenesis. hucMSCs without exosomes have nearly no effect on cardiac function recovery, suggesting hucMSC-exosome–mediated myocardial repair85,86. hucMSC-exosomes promoted wound healing and angiogenesis in vivo, and this pro-angiogenic effect was mediated by Wnt4/β-catenin activation in endothelial cells87. MSC-exosomes also increased ATP levels, which led to decreased oxidative stress via the PI3K/Akt pathway to enhance myocardial viability and prevent adverse remodeling after I/R injury88. Similarly, exosomal heat shock protein-70 (HSP70) stimulated toll-like receptor-4 (TLR4) signaling, leading to the activation of ERK1/2 and p38MAPK and subsequent HSP27 phosphorylation in cardiomyocytes89,90. Cardiac progenitor cells (CPCs) derived from the adult hearts have also emerged as potential stem cell population for cardioprotection and repair. CPC-derived exosomes conferred a protective preconditioning effect when injected into mouse hearts immediately after the induction of ischemia. They appeared to protect cardiomyocytes from oxidative stress in vitro and IR injury in vivo91 and exerted anti-fibrotic effects on the fibroblasts and promote angiogenesis60.
4.1. Therapeutic effects of iPSC-derived exosomes
Previous report indicated that the embryonic stem cell-derived exosomes promote endogenous repair and enhance cardiac function following MI26. Although the contents in the secretome of iPSCs are not fully elucidated, iPSC-culture medium show improved alveolar epithelial wound repair properties in lung epithelial wound healing model and reduce lung fibrosis in vivo92. Isolated exosomes/EVs secreted from iPSCs culture medium seemed to provide the protective effects on ischemic myocardium. iPSC-derived exosomes protect H9C2 cells against H2O2-induced oxidative stress by inhibiting caspase3/7 activation93. Recent studies have characterized the bioactive molecules in the iPSC derived exosomes. Bobis-Wozowicz and colleagues showed that microvesicles derived from hiPSCs are capable of transfering bioactive molecules including mRNA, miRNA, and proteins. These bioactive molecules from iPSC-derived microvesicles are delivered to human cardiac mesenchymal stromal cells (cMSCs) and are found to exert protective effects by affecting the transcriptomes and proteomic profiles of the recipient cells. In addition, the microvesicles from the hiPSCs exhibit enhanced cardiac and endothelial differentiation potential of cMSCs94. Microvesicles derived from mouse iPSC were shown to contain a group of iPSC-specific pluripotent transcription factors critical in maintaining iPSC pluripotency95. The promising results encourage more investigation on iPSC-derived exosomes although information inside the iPSC-exosomes are not completely identified yet.
Besides exosomes derived from iPSCs, many studies indicate plasticity and immunomodulatory capabilities of the iPSC-derived MSCs (iMSCs), which may contribute towards unique therapeutic potentials of the MSCs96. The iMSCs seem to provide reliable source for better survival, proliferation and differentiation potentials, compared to adult MSCs97. The exosomes derived from iMSCs partly carry on the protective features from MSCs. In preclinical studies, exosomes secreted by iMSCs can improve local angiogenesis in limb ischemia mice model and rat skin wound model. From in vitro assays, iMSC-exosomes promote endothelial cell migration, proliferation, and tube formation98,99. In osteopenic animal models, iMSC-exosomes have proven to enhanced angiogenesis and osteogenesis100. Furthermore, iMSCs-exosomes alleviate hepatic I/R injury possibly by suppression of inflammatory responses, attenuation of the oxidative stress response, and inhibition of apoptosis101. In this respect, iPSCs and iPSC-derivatives may serve as a source of autologous bioactive exosomes that could be utilized for future clinical translation to treat heart diseases. It provides a great platform for safe, effective, and reliable delivery of bioactive molecules to the recipient cells. However, further analysis of the bioactive molecules inside of exosomes should be investigated for the future application.
4.2. Therapeutic expectation of exosomes from iPSC-derived cardiomyocytes (iCM)
Given the limited regenerative capacity of the human heart, stem cell-derived cardiomyocytes are a promising source for replacement cell therapy. iPSC-derived cardiomyocytes (iCMs) have shown potential to attenuate ischemic damage and restore cardiac function in pre-clinical MI models3,17,102. The patient-specific iCMs provide a great platform for developing personalized treatments, employing autologous cells. The paracrine role of these iCM-derived exosomes remains to be determined. Previous studies have elucidated the exosomes released from native cardiomyocytes. In isolated exosomes from cultured adult mouse cardiomyocytes (HL-1), 423 biologically relevant mRNAs and 343 different chromosomal DNA sequences were identified103. These exosomal signaling molecules were deemed to exert influence on the surrounding cells, either protective or detrimental. In the fibroblasts transfected by the same exosomes, 175 up- and 158 down-regulated genes were identified although the protective mechanisms have not been described. Recent studies have indicated that heat shock protein 20 (HSP20), HSP27, HSP60, HSP70, and HSP90 were detected in cardiomyocyte-derived exosomes with multiple functions90,104,105. For instance, in vitro assays showed that extracellular HSP20 promotes proliferation, migration and tube formation of HUVECs. In vivo study of HSP20 increased capillary density, suggesting a pro-angiogenic role of the iCM-derived exosomes105. Our group has focused on the iCMs derived from human placenta amniotic mesenchymal stem cell (MiCMs), which retained the immunomodulatory properties of the original cells and engrafted successfully in the immunocompetent SVJ mice106. Our MiCMs have shown to improve cardiac function of injured mouse hearts after MI. We observed that the MiCM-derived exosomes exhibited similar functions, such as cardiac-protective role, improving LVEF (left ventricular ejection fraction) and myocardial viability106,107. The exosomal cargo contained several up-regulated miRNAs, including miR-92a believed to stimulate endogenous anti-apoptotic, pro-angiogenic, and anti-fibrotic pathways.
Differential exosome production and cargo content were observed when cardiomyocytes were stimulated under various stress conditions. For instance, membrane stretch and cardiac pressure overload are shown to enhance exosome generation. Interestingly, functional angiotensin 1 receptor (AT1R) is identified in exosomes derived from stretched cardiomyocytes, exposed to pressure overload. Functional AT1Rs are transferred via exosomes in an in vitro model of cardiomyocyte stretch. Using mice with global and conditional cardiomyocyte deletion of β-arrestin2, researchers showed that under conditions of in vivo pressure overload, the cellular source of the exosomes containing AT1R is the cardiomyocytes108. In Garcia’s study, glucose deprivation increased H9C2 cardiomyocyte secretion of exosomes. Gene Ontology (GO) analysis of exosome cargo identified the molecules, which alters the angiogenic activity of the endothelial cells. In glucose deprived medium, the exosomes were identified to be loaded with functional glucose transporters and glycolytic enzymes, which are internalized by ECs to increase glucose uptake, glycolytic activity, and pyruvate production in recipient cells27,109. A paracrine miRNA crosstalk between cardiac fibroblasts (CFs) and cardiomyocytes (CMs) was found to be mediated by the CF secreted miRNA-enriched exosomes. Lyu and collegues studied the angiotensin II (Ang II) stimulation, which enhanced the exosome production in cultured CFs via the activation of AT1R and AT2R whereas lipopolysaccharide, insulin, endothelin (ET)-1, transforming growth factor beta (TGFβ)1 or hydrogen peroxide did not. The CF-derived exosomes up-regulated the expression of renin, angiotensinogen, AT1R, and AT2R; downregulated angiotensin-converting enzyme 2; and enhanced Ang II production in cultured cardiomyocytes. These findings demonstrate that Ang II stimulates CFs to release exosomes, which in turn increase Ang II production and its receptor expression in cardiomyocytes, thereby intensifying Ang II-induced pathological cardiac hypertrophy. Consequently, specific targeting of Ang II-induced exosome release from CFs may serve as a novel therapeutic approach to induce cardiac pathological hypertrophy in the injured cardiomyocytes110. Together, the iCM-derived exosomes bear a mixed signaling content from their parental cells. By using iCM-derived exosomes as therapeutical tools, we can apply different stimulus to enhance/adjust exosomal content. For instance, we identified differentially expressed genes in the iCM-derived exosomes in normoxic and hypoxic conditions (unpublished data), which suggest a functional difference in exosomes in oxygen-rich and oxygen-poor iCMs. Finally, gene editing techniques can be used to precisely knock in or delete exosomal contents.
5. Possible limitations to the use of exosome-based therapeutics
Exosomes would provide a more feasible approach for bench-to-bedside translation compared to the cell-based therapy. However, their stability, off-target effects, and inefficient endocytosis by target cells must be addressed111. A parameter affecting the stability of exosomes is the zeta potential, which is a physical property of exosomes that measures the magnitude of electrostatic repulsion or attraction between particles112. Nanoparticles are usually stable at absolute zeta potential values ranging from 31 to 40 mV113. Recent experiments showed that the zeta potential detected in mouse cardiac fibroblast exosomes and cardiac fibroblast-derived iPSC-exosomes were very low (−23.85 mV vs. −23.93 mV at 37°C). Hence, these exosomes are very unstable and aggregate easily93.
Exosome-based miRNA delivery technique has been considered as attractive tools in several diseases to provide reliable evidence to overcome the inefficient, non-specific delivery of miRNAs. However, this technique needs further optimization to investigate the miRNA biology, long-term biochemical and off-target effects, and pathway analysis35. Exosomes may have only short-term benefit owing to their short half-life. Takahashi and colleagues showed that murine melanoma B16-BL6 cells exosomes disappeared very quickly from the blood circulation with a half-life of approximately 2 min114. Exosomes may also have pro-fibroblast effects. For example, iPSC-derived MSCs exosomes can facilitate cutaneous wound healing by promoting collagen synthesis and angiogenesis99. It is established that fibroblasts are important to prevent cardiac rupture after acute MI115. Thus, in the short term, iPSC-exosome treatment might reduce the risk of acute heart rupture; however, in the long-term, increased proliferation of fibroblasts could increase scarring, leading to left ventricular remodeling in the ischemic myocardium.
Cell manipulations aimed at enhancing beneficial properties of exosomes may include hypoxia and stress preconditioning60, genetic modification116, and epigenetic reprogramming of exosome-producing cells117. For instance, as shown by Gray et al., the exosomal content depends on the state of the donor cell and disease conditions60. The standardization of the conditions under which exosomes are collected is therefore of utmost significance. Although the cargo of these stem cell–derived exosomes can pose beneficial effects on injured heart, the underlying mechanisms are to be uncovered. In addition, many of the proteins in the exosomes are enzymes. Since enzyme activities are catalytic rather than stoichiometric and are influenced by their microenvironment, enzyme-based therapeutic activities of exosomes could be activated or attenuated according to the release of injury-associated substances. The resolution of the disease-precipitating microenvironment would reduce the release of injury-associated substrates, as well as the activity of the exosomal enzymes. Consequently, the efficacy of exosome-based therapeutics could be highly responsive to, but also limited by, the niche and the microenvironment118. Exosomes may also facilitate the uptake of therapeutic proteins or RNAs into injured cells. Although cellular uptake of exosomes has been demonstrated to occur through endocytosis, phagocytosis, and membrane fusion, the mechanism by which these processes are regulated remains to be determined. The efficiency of exosome uptake has been found to correlate directly with intracellular and microenvironmental acidity119,120.
Many studies demonstrate that the translation of exosomes has great potential in clinical applications. However, this might be complicated by the relative novelty of exosomes with few precedents in the regulation and safety of the biopharmaceuticals. Despite its advantages, exosome delivery may need to address some of the potential complications. In the case of the factors operating at the intracellular level, delivery into the correct cellular compartments while maintaining the stability, integrity, and biological potency of these factors remains challenging and expensive. Certainly, the coming few years will provide many more insights into the biological significance of cell–cell transport via exosomes. Systematic approach to the structural and functional biology of exosomes will create an opportunity to increase our understanding of the role of exosomes in repairing injured myocardium.
6. Conclusion and future perspectives
Until the early 2010s, stem cell transplantation was regarded as a potentially therapeutic alternative in treating ischemic heart disease. Despite the fact that functional cardiomyocytes derived from human pluripotent stem cells improved cardiac morphology and function in animal models of heart failure, they also triggered an arrhythmogenic response3,48. Teratoma formation has also been observed after the transplantation of an unpurified pluripotent-derived cardiomyocytes17,102. The derivation of iPSCs appears to have partially addressed the issues related to immune rejection. Nonetheless, pluripotent stem cell-derived cells still pose the same difficulties to engraft in the ischemic myocardium as is noted for adult stem cells. Therefore, there is a critical need for exploiting the powerful regenerative biology of the pluripotent stem cells while avoiding the problems associated with cell transplantation121. More importantly, the exact mechanism of action of stem cells has not been elucidated. Future studies on paracrine factors such as the exosomes will shed more mechanistic understanding of stem cell therapy.
Among the stem cell secretomes, exosomes appear to be the most suitable vehicle for delivery because of their ability to target the specific lineage related and physiologically relevant cells due to their endosomal origin. Exosomes of different cellular origin bear distinct antigenic proteins of their native cell type122. This enables specific binding property to the recipient cell receptors, providing a targeted delivery to the desired cell type. This approach may enable the reality of personalized medicine in the near future, utilizing the exosomes generated from autologous iPSC-derivatives such as the iCMs.
The biotechnological approach to create exosome-based delivery systems used by Alvarez-Erviti et al. was the first demonstration of an exosome-based drug delivery system, which showed efficient in vivo delivery of siRNA123. Another study demonstrated that the successful introduction of exogenous siRNAs into various human exosomes and delivered to human mononuclear blood cells. Plasma exosomes effectively delivered the siRNA into the target cells, causing selective gene silencing of MAPK-1124.
6.1. Application of exosomes to personalized medicine
Exosomes could become a game-changer for both diagnostic and therapeutic purposes for chronic diseases with advanced clinical manifestations. Exosomes are natural vehicle to deliver molecules from one cell to another. Researchers are trying to harness this natural ability of the exosomes to deliver therapeutic payloads to the desired cells. Furthermore, gene editing of the native stem cells will produce exosomes with specific cell surface proteins and cargo for targeted delivery of the desired exosomes. Numerous attempts to enhance stem cell biology in the area of reprogramming, transdifferentiation/differentiation, and engraftment of the cells have been made. The results have been quite encouraging. However, significant obstacles remain for clinical translation of pluripotent stem cell-derivatives. Particularly, mutagenic complication, long-term engraftment, mechanism of action, and local physiologic effects must be understood. A more exact and effective approach needs to be developed to translate the pluripotent stem cell biology to enable specific modification of the injury at targeted location. Exosomes from iPSC-derivatives can be applied to enable an autologous therapy by simulating endogenous repair. Thus, the exosomes generated from patient-specific iPSC-derivatives are expected to provide higher therapeutic specificity, quality control, ease of production, and safety than stem cell transplantation. Exosomes from gene-edited patient-specific stem cells can be directed to the specific lesions of each individual patient to promote the salvage of the existing injured cells. (Figure 3). In conclusion, exosomes hold potential for a wide spectrum of beneficial effects on cell function recovery to restore the myocardial injury by simulating and activating the endogenous repair, consisting of the native transfer of proteins, mRNAs, and miRNAs. They will serve as an excellent therapeutic tool for cardioprotection or regeneration of the injured mycordium. Exosomes represent the most feasible approach to translate the enormous potential of pluripotent stem cell biology. Exosome-based treatment approach present an exciting new concept in translational medicine with huge potential to impact the thereapeutic effect of CVDs.
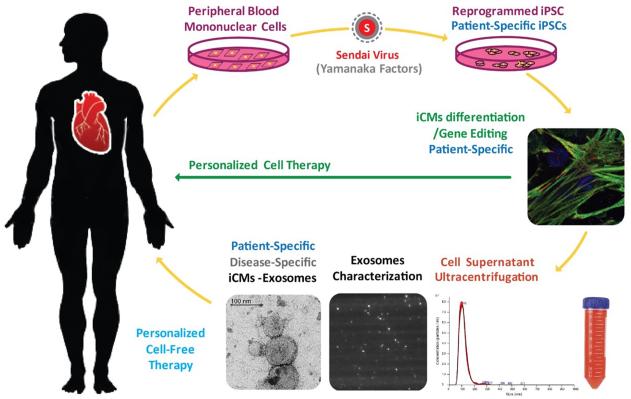
Exosomes from patient-specific iPSC-derivatives provide a platform for personalized therapy by simulating endogenous repair. Exosomes can be directed to specific injured site of each individual patient to promote salvage of the existing injured cardiac cells. Exosomes isolated from the supernatant of iCM culture undergo ultracentrifugation methods, as well as advanced characterization and quantification by transmission electronical microscopy (TEM), Nanosight, and Dynamic Light Scattering (DLS).
Acknowledgment
No other persons besides the authors have made substantial contributions to this manuscript.
Non-standard Abbreviations and Acronyms
ASCs | Adipose stem cells |
AT1R | Angiotensin 1 receptor |
Ang II | Angiotensin II |
CPCs | Cardiac progenitor cells |
CFs | Cardiac fibroblasts |
CMs | Cardiomyocytes |
CVD | Cardiovascular disease |
EVs | Extracellular vesicles |
esRNAs | Exosomal shuttle RNAs |
GO | Gene Ontology |
HSP70 | Heat shock protein-70 |
hESCs | Human embryonic stem cells |
hMSCs | Human mesenchymal stem cells |
hucMSCs | Human umbilical cord mesenchymal stem cells |
MiCMs | Human placenta amniotic mesenchymal stem cell |
iPSC | induced pluripotent stem cells |
iCM | iPSC-derived cardiomyocytes |
LVEF | Left Ventricular Ejection Fraction |
MSCs | Mesenchymal stem cells |
mESC | Mouse embryonic stem cell |
MVBs | Multivesicular bodies |
MI | Myocardial infarction |
I/R | Myocardial ischemia/reperfusion |
TLR4 | Toll-like receptor-4 |
TGFβ1 | Transforming growth factor beta 1 |
Footnotes
Sources of funding
This work is supported by NIH/NHLBI 5UM1 HL113456-02, NIH/NHLBI 1 K24 HL130553, and CIRM DISC1-08650
Disclosures
None
References
Full text links
Read article at publisher's site: https://doi.org/10.1161/circresaha.116.309307
Read article for free, from open access legal sources, via Unpaywall:
https://www.ahajournals.org/doi/pdf/10.1161/CIRCRESAHA.116.309307
Citations & impact
Impact metrics
Article citations
Landscape of exosomes to modified exosomes: a state of the art in cancer therapy.
RSC Adv, 14(42):30807-30829, 26 Sep 2024
Cited by: 0 articles | PMID: 39328877 | PMCID: PMC11426072
Review Free full text in Europe PMC
Cardiomyopathy: pathogenesis and therapeutic interventions.
MedComm (2020), 5(11):e772, 25 Oct 2024
Cited by: 0 articles | PMID: 39465141 | PMCID: PMC11502724
Review Free full text in Europe PMC
Vascularized platforms for investigating cell communication via extracellular vesicles.
Biomicrofluidics, 18(5):051504, 23 Sep 2024
Cited by: 0 articles | PMID: 39323481
Review
Unlocking the therapeutic potential: odyssey of induced pluripotent stem cells (iPSC) in precision cell therapies.
Int J Surg, 04 Jul 2024
Cited by: 0 articles | PMID: 38963728 | PMCID: PMC11487032
Exosomes in the pathogenesis and treatment of cancer-related cachexia.
J Transl Med, 22(1):408, 30 Apr 2024
Cited by: 1 article | PMID: 38689293 | PMCID: PMC11062016
Review Free full text in Europe PMC
Go to all (102) article citations
Similar Articles
To arrive at the top five similar articles we use a word-weighted algorithm to compare words from the Title and Abstract of each citation.
Induced Pluripotent Stem Cells 10 Years Later: For Cardiac Applications.
Circ Res, 120(12):1958-1968, 01 Jun 2017
Cited by: 143 articles | PMID: 28596174
Review
Microfluidic Single-Cell Analysis of Transplanted Human Induced Pluripotent Stem Cell-Derived Cardiomyocytes After Acute Myocardial Infarction.
Circulation, 132(8):762-771, 01 Aug 2015
Cited by: 61 articles | PMID: 26304668 | PMCID: PMC4557214
Paracrine Effects of the Pluripotent Stem Cell-Derived Cardiac Myocytes Salvage the Injured Myocardium.
Circ Res, 121(6):e22-e36, 25 Jul 2017
Cited by: 87 articles | PMID: 28743804 | PMCID: PMC5783162
Exosomes From Induced Pluripotent Stem Cell-Derived Cardiomyocytes Promote Autophagy for Myocardial Repair.
J Am Heart Assoc, 9(6):e014345, 05 Mar 2020
Cited by: 56 articles | PMID: 32131688 | PMCID: PMC7335524
Funding
Funders who supported this work.
NHLBI NIH HHS (2)
Grant ID: UM1 HL113456
Grant ID: K24 HL130553