Abstract
Objective
Noninvasive brain stimulation (NIBS) techniques, such as transcranial direct current stimulation (tDCS) and repetitive transcranial magnetic stimulation (rTMS), are increasingly being used to treat mental disorders, particularly major depression. The aim of this comprehensive review is to summarize the main advances, limitations, and perspectives of the field.Methods
We searched PubMed and other databases from inception to July 2017 for articles, particularly systematic reviews and meta-analyses, evaluating the use of NIBS in psychiatric disorders.Results
We reviewed the mechanisms of action, safety, tolerability, efficacy, and relevant clinical parameters of NIBS. Repetitive TMS is already an established technique for the treatment of depression, and there is theoretically room for further methodological development towards a high-end therapeutic intervention. In contrast, tDCS is a technically easier method and therefore potentially suitable for wider clinical use. However the evidence of its antidepressant efficacy is less sound, and a recent study found tDCS to be inferior to antidepressant pharmacotherapy. Clinical trials using rTMS for other mental disorders produced mixed findings, whereas tDCS use has not been sufficiently appraised.Conclusion
The most promising results of NIBS have been obtained for depression. These techniques excel in safety and tolerability, although their efficacy still warrants improvement.Free full text

Noninvasive brain stimulation in psychiatric disorders: a primer
Abstract
Objective:
Noninvasive brain stimulation (NIBS) techniques, such as transcranial direct current stimulation (tDCS) and repetitive transcranial magnetic stimulation (rTMS), are increasingly being used to treat mental disorders, particularly major depression. The aim of this comprehensive review is to summarize the main advances, limitations, and perspectives of the field.
Methods:
We searched PubMed and other databases from inception to July 2017 for articles, particularly systematic reviews and meta-analyses, evaluating the use of NIBS in psychiatric disorders.
Results:
We reviewed the mechanisms of action, safety, tolerability, efficacy, and relevant clinical parameters of NIBS. Repetitive TMS is already an established technique for the treatment of depression, and there is theoretically room for further methodological development towards a high-end therapeutic intervention. In contrast, tDCS is a technically easier method and therefore potentially suitable for wider clinical use. However the evidence of its antidepressant efficacy is less sound, and a recent study found tDCS to be inferior to antidepressant pharmacotherapy. Clinical trials using rTMS for other mental disorders produced mixed findings, whereas tDCS use has not been sufficiently appraised.
Conclusion:
The most promising results of NIBS have been obtained for depression. These techniques excel in safety and tolerability, although their efficacy still warrants improvement.
Introduction
Noninvasive brain stimulation (NIBS) techniques use electric currents (injected or induced by magnetic fields) as a method to treat mental and neurological disorders.1 Unlike invasive techniques such as deep brain stimulation (DBS) and vagus nerve stimulation (VNS), or noninvasive but convulsive techniques such as electroconvulsive therapy (ECT) and magnetic seizure therapy (MST), NIBS techniques use non-implantable devices and do not require sedation or anesthesia (Table 1).
Table 1
Technique | Mechanism of action | Sedation/anesthesia | Advantages | Disadvantages | Current situation |
---|---|---|---|---|---|
DBS | Electrodes implanted into the area of interest inside the brain | Necessary | Pilot studies are promising; no cognitive impairment reported | Invasive; long-term adverse events include tremor and transient hypomania | Experimental use (FDA-approved for OCD under a Humanitarian Device Exemption) |
VNS | Electrodes connected to the vagus nerve generate impulses transmitted to subcortical areas | Necessary | No cognitive impairment reported | Invasive; transient hypomania reported | FDA-approved for patients who have failed + four antidepressant treatments |
ECT | Electric current injection | Necessary | Highly effective for severe MDD, psychotic MDD, TRD, and MDD with suicidal ideation | Cognitive impairment, which may be persistent depending on ECT type; not available in many contexts | Approved for clinical use |
rTMS | Electric current induced by an electromagnetic field | Not necessary | As effective as antidepressant drugs; no cognitive impairment reported | Seizures are rare but possible; expensive | Approved for clinical use |
tDCS | Electric current injection | Not necessary | No serious adverse events; increased portability; excellent tolerability | Mixed results so far | In the process of regulation |
tACS | Electric current injection | Not necessary | No serious adverse events; increased portability; excellent tolerability | Not consistently evaluated for clinical disorders | Experimental use |
Other tES methods | Electric current injection | Not necessary | No serious adverse events; increased portability; excellent tolerability | Not consistently evaluated for clinical disorders | Experimental use |
DBS = deep brain stimulation; ECT = electroconvulsive therapy; FDA = Food and Drug Administration; MDD = major depressive disorder; OCD = obsessive-compulsive disorder; rTMS = repetitive transcranial magnetic stimulation; tACS = transcranial alternating current stimulation; tDCS = transcranial direct current stimulation; tES = transcranial electric stimulation; TRD = treatment-resistant depression; VNS = vagus nerve stimulation.
NIBS is represented by two techniques: repetitive transcranial magnetic stimulation (rTMS), introduced in 1985,1 and transcranial electric stimulation (tES), with its most widespread variant being transcranial direct current stimulation (tDCS), reintroduced in its modern form in 2000.2 The effects of rTMS are known to vary according to coil shape (figure-of-eight, H-coil, double-cone coil), stimulation pattern (high-frequency, low-frequency, theta-burst), and stimulation site.3 The effects of tES also vary according to current (direct, alternating, pulsed, random noise), polarity (anodal or cathodal for tDCS), current intensity, and stimulation site.4
Repetitive TMS has been used in clinical settings since the past decade, and is already regulated for clinical use in many countries, such as the United States, Israel, Australia, and across the European Union.5 In Brazil, it has been approved for clinical use by the Agência Nacional de Vigilância Sanitária (ANVISA), Conselho Federal de Medicina (CFM), and Conselho Federal de Fisioterapia e Terapia Ocupacional (COFITO). tDCS has been regulated by the ANVISA and bears a CE mark, although it has not yet been approved by the U.S. Food and Drug Administration.6,7 Nevertheless, according to recent clinical guidelines,8,9 tDCS is possibly/probably effective for depression.
As NIBS is being steadily incorporated into clinical practice, the aim of this review is to provide an overview of the main NIBS techniques used in the treatment of mental disorders. Since major depressive disorder (MDD) is the main condition in which rTMS and tDCS are used, most examples will refer to NIBS protocols for MDD.
Methods
We carried out a comprehensive review of articles describing the use of tDCS and rTMS in psychiatry. The PubMed, Google Scholar and PsycINFO databases were searched from inception until July 2017. The following terms were used: “transcranial magnetic stimulation”; “transcranial direct current stimulation”; “transcranial electric stimulation”; “non-invasive brain stimulation”; “major depressive disorder”; and “mental disorders.” Preference was given to recent systematic reviews and meta-analyses describing the clinical use of NIBS in mental disorders. The reference lists of the retrieved articles were also hand-searched for additional articles. We excluded articles evaluating NIBS use solely in animals, non-psychiatric disorders, or children and adolescents.
Mechanisms of action
Transcranial magnetic stimulation (TMS)
TMS is based on the principle of electromagnetic induction by an electric current passing through a coil. In contact with conductive tissues (such as the brain), the generated magnetic field induces a secondary electric field.10 Unlike that of a static magnet, the magnetic field generated by the TMS device is variable within milliseconds, which leads to the induction of an electric field in the region under the coil. The magnetic field passes through the skin, bone, and fat (tissues with low electrical conductivity) essentially without resistance and with little deflection, which ensures that the induced current is relatively focal. However, there is a decline in intensity with increasing distance from the center of the coil, which causes the magnetic field (and induced current) to have relatively small penetration.10
The magnetic fields are produced with flux lines perpendicular to the plane of the coil. Within 0.1-0.6 ms, the field reaches a magnetic flux density comparable to that of the static field of a magnetic resonance imaging (MRI) coil, i.e., 1.5-3 Tesla. In a circular coil, the magnetic field is more concentrated on the internal periphery of the coil, with virtually no induced current at the center. The figure-of-eight coil is, in turn, more focal, with the sum of the field lines induced by each loop producing a more intense magnetic field at the center of the figure-eight. The secondary electric current induced in neurons is produced perpendicularly to the magnetic field, i.e., parallel to the plane of a flat-shaped coil.10
The effects of a single pulse of TMS on the motor cortex are usually measured using electromyography (EMG)-based motor evoked potentials (MEP). Each TMS pulse indiscriminately stimulates all neurons in the region where the stimulus is produced. However, depending on the depolarization threshold, orientation, and proximity of the coil, they may or may not fire. Stimulation of cortical-spinal pyramidal neurons when firing may produce direct waves (D waves), which are the first to be generated and of greater amplitude. Stimulation of interneurons generates indirect waves (I wave), which appear at regular intervals and with a smaller amplitude after the D wave. Different pulse intensities and coil positions may evoke different D-wave and I-wave patterns.10
The excitatory and inhibitory effects of rTMS are hypothesized to be long-term potentiation (LTP)- and long-term depression (LTD)-like. LTP and LTD are two mechanisms of synaptic plasticity that involve several biological phenomena and ultimately lead to synaptic strengthening (LTP) or weakening (LTD) (i.e., an increase or decrease in synaptic efficiency). The similarities between the effects of rTMS and LTP/LTD include: 1) rTMS induces effects beyond the period of stimulation; 2) the temporal pattern of stimulation is important for the direction of the effect; 3) the effects of rTMS depend on the prior activity of the neural network; 4) rTMS is involved in the expression of brain-derived neurotrophic factor (BDNF) and c-fos, molecules associated with LTP; 5) the effects of rTMS are inhibited by blockade of N-methyl-D-aspartate (NMDA) receptors; and 6) the effects of rTMS are associated with BDNF polymorphisms.11,12
Usually, rTMS is considered excitatory when using high-frequency protocols (HF) (≥ 10 Hz) or intermittent theta-burst stimulation (iTBS), and inhibitory when using low-frequency (LF) (≤1 Hz) protocols or continuous theta-burst stimulation (cTBS). However, these assumptions are rules of thumb and need to be experimentally verified for single applications. The clinical effects of rTMS are also influenced by the number of sessions, session length, intensity of the pulses (indexed according to the subject's motor threshold), the intervals between pulses and trains, number of sessions, session length, method used to position the coil, coil shape, and other factors.13
Transcranial direct current stimulation (tDCS)
In tDCS, an electric current of low intensity (usually 1-2 mA) is injected into the brain through electrodes placed over the scalp. The current passes through the skin, subcutaneous tissue, skull, and cerebrospinal fluid (CSF) to the gray matter. As the first three layers exhibit high impedance, only approximately 10% of the injected current reaches the brain. In addition, as the sponge-electrode set is large (25 to 35 cm2), the stimulation is nonfocal.14
Electrons flow from the cathode to the anode, in the radial direction. In the first protocols used by Nitsche et al., electric currents of 1 mA, 7 to 13 minutes long, induced excitatory (anodal) or inhibitory (cathodal) effects on motor cortex excitability, i.e., MEP amplitudes. The electric current injected does not generate action potentials per se, but facilitates or inhibits synaptic transmission, which is expressed by an increase or decrease in the frequency of action potentials in endogenous neuronal firing.15
Previous studies have shown that the effects of tDCS on motor cortical excitability are nonlinear. For instance, cathodal and anodal stimulations of 2 mA for 13 minutes were reported to generate excitatory effects,16 whereas cathodal and anodal stimulations of 1 mA for 26 minutes were observed to exert inhibitory effects.17
The nonlinear effects of tDCS have been attributed to the influence of the electric current on intracellular Ca+2 concentrations, which could reverse the direction of neuroplasticity from LTD to LTP (for example, cathodal electric current with greater intensity, increasing Ca+2 concentration) or LTP to LTD (e.g., a prolonged anodal low-intensity current, leading to a progressive decrease in Ca+2 concentration).18 However, these effects were observed in the motor cortex of healthy individuals, and it is unknown to what extent they can be translated to tDCS of non-motor regions or pathological situations, e.g., in MDD and other mental disorders.
As in rTMS, several mechanisms of tDCS appear to be LTP or LTD-like, such as: 1) effects dependent on prior network activity; 2) loss of effects with NMDA or Ca+2 channel blockade; 3) potentiation of the effect by NMDA agonists; and 4) increased BDNF expression after stimulation.18
TMS parameters and their relevance for clinical practice
An rTMS protocol involves the selection of several parameters, which should take into consideration the desired physiological effect, as well as existing evidence for TMS treatment of the patient’s condition.19 An rTMS session consists of delivering several repetitive pulses, usually between 600 and 3,000 per session (Table 2).
Table 2
rTMS method | Pattern | Pulse mode | Pulses per burst | Frequency (Hz) | Total trains | Pulses per train | Inter-train intervals (seconds) | Pulses per session | Total time per session (minutes) |
---|---|---|---|---|---|---|---|---|---|
HF |
![]() | Single pulse | NA | ≥ 10 | 60 | 50 | 25 | 3,000 | 30 |
LF |
![]() | Single pulse | NA | ≤ 1 | 1 | 1,200 | 0 | 1,200 | 20 |
iTBS |
![]() | Burst | 3 (at 50 Hz) | 5 | 20-30 | 30 | 8 | 600-900 | 4-7 |
cTBS |
![]() | Burst | 3 (at 50 Hz) | 5 | 1 | 600-900 | 0 | 600-900 | 2-3 |
cTBS = continuous theta-burst stimulation; HF = high frequency; iTBS = intermittent theta-burst stimulation; LF = low frequency; NA = not applicable; rTMS = repetitive transcranial magnetic stimulation.
Stimulation frequency
HF-rTMS and LF-rTMS protocols are commonly described as either excitatory or inhibitory. Typical parameters for an HF-rTMS session involve a sequence of pulses at 10 Hz delivered in 5 seconds (a “train”), with a 20-to-40-second pause between trains, for a total of 1,200-3,000 pulses delivered over about 15 to 45 minutes. An interval between trains in HF stimulations is recommended, and the precise timing should follow current safety guidelines.20 LF-rTMS does not require interval between “trains,” so sessions can contain a continuous sequence of pulses at 1 Hz (60 pulses per minute), with 600-1,500 pulses delivered in a relatively shorter period.
Another rTMS modality is “theta-burst stimulation” (TBS). This modality consists of a series of pulses, usually between 3 to 5, at 50 Hz (called a “burst”), delivered at a frequency of 5 Hz. This frequency coincides with the theta frequency band of the electroencephalography (EEG), hence its name. TBS protocols are also either inhibitory or excitatory. iTBS has been reported to exert excitatory effects and consists of a train of “bursts” with a pause in between (usually, a 2-second train with an 8-second pause). Therefore, a session containing 1,200 pulses can be performed in less than 8 minutes. cTBS shows inhibitory effects and, just as conventional LF-rTMS, does not require intervals between trains. Therefore, a session of cTBS with 1,200 pulses can be delivered in approximately 3 minutes.21 The short duration of sessions compared to standard rTMS makes TBS an appealing therapeutic tool, as discussed below.
Other TMS interventions used in clinical practice involve the combination of HF and LF protocols in the same session. One method is described as “bilateral” rTMS, when HF and LF are applied simultaneously or, more commonly, sequentially, to contralateral brain regions. The other method is “priming” rTMS, when a specific rTMS protocol is applied before another protocol, to the same brain region, to enhance the effects of the second protocol (e.g., HF before LF to enhance LF effects).22
Resting motor threshold (rMT)
The rMT is classically defined as the minimal percentage of the total stimulator output necessary to generate a MEP of 50 µV amplitude or more in at least five out of 10 trials. The rMT is usually examined by targeting the motor cortex in the region of the hand representation and performing EMG on a contralateral small muscle of the hand (e.g., abductor pollicis brevis, first dorsal interosseous, or abductor digiti minimi muscle); the resulting potential is expected to have an amplitude above 50 μV.23 It is important to measure the rMT at is provides evidence that a given intensity of TMS is generating a magnetic field strong enough to reach and depolarize the motor cortex, which can be extrapolated to non-motor cortical areas. The “hotspot” is the area where the minimum rMT is identified.
After measuring the rMT, the desired intensity for the protocol, reported as a percentage of the rMT, is selected. Conventional rTMS protocols apply intensities from 100 to 120% of the rMT, whereas for TBS intensity usually ranges from 80 to 100%. The relevance of intensity for clinical outcomes is uncertain, although use of intensities equal to or higher than 100% of rMT is advised.9
TMS coil
The shape and design of the TMS coil correlate with the intensity output, precision, and depth of the magnetic field induced. Due to the physics of magnetic fields, precision and depth are inversely related: focal fields have very limited depth, whereas deep stimulations necessarily involve the induction of a large magnetic field.3
The most commonly used coil design is the figure-of-eight, which allows induction of a highly focal magnetic field below its center, capable of producing physiological effects up to a depth of 2 cm. “H-coils” and “double-cone” coils can reach higher depths, up to 5 cm.3 Despite this characteristic, the depth of the magnetic field has not yet been correlated with clinical outcomes.
Coil position
The cortical target is a crucial parameter for the therapeutic use of TMS, as the desired physiological effects depend on stimulating a precise cortical region. For instance, protocols for MDD usually target the dorsolateral prefrontal cortex (DLPFC).
Once the cortical target is selected, it is important to determine how to position the coil to hit the target accurately. The gold-standard approach is neuronavigation. This method consists of first scanning the patient’s brain and identifying the cortical target for stimulation. Integration of the image with the spatial position of the patient’s head and the TMS coil is done using a sensor that identifies the position of trackers (attached to the patient’s head and to the TMS coil) and specific software.24
In the absence of neuronavigation, anatomical methods can be used. These methods rely on anatomical landmarks to estimate the target. Perhaps the most used system is the international 10-20 system for EEG. In this system, the DLPFC is estimated to be under the F3 electrode. For improved targeting, a freely available software was developed to estimate the position of the DLPFC based on only three measurements known from the EEG 10-20 system (head circumference, tragus-tragus distance, and nasion-inion distance): the “Beam F3” method (clinicalresearcher.org/software.htm).25 This method has shown a level of precision comparable to neuronavigation.26 Another method to estimate the localization of the DLPFC is the “rule of 5 cm” and its variations, in which the target is estimated to be located 5 cm anterior to the hotspot. This method is now considered outdated and seldom used in clinical practice, as it was shown to be unreliable in locating the prefrontal cortex in varying head sizes.
As neuronavigation is an expensive procedure which requires specialized personnel, its use for TMS is usually limited to research settings. In clinical practice, the level of accuracy provided by neuronavigation has not been ubiquitously associated with greater clinical effects or different treatment responses. Possibly, this occurs because neuronavigation methods are mostly based on anatomical and not functional parameters. In this regard, recent studies suggested that the latter may provide superior responses.27,28 Nonetheless, in usual psychiatric clinical practice, neuronavigation is still reserved for patients with significantly altered cranial or brain anatomy.
tDCS parameters and their relevance for clinical practice
The following parameters determine the clinical effects of tDCS: sponge size and electrode positioning, current intensity and polarity, duration of stimulation, number of sessions, and interval between sessions.2,29,30 The size of the electrodes ranges from 3.5 to 100 cm2. The most common sets are 25 and 35 cm2 electrodes.30,31 Proper positioning of the electrodes is essential to ensure that the electric field is directed to the area of interest. The international 10/20 EEG system is the most common reference for electrode assembly. Current intensity ranges from 1 to 2 mA; the session duration is usually between 9 and 30 minutes.29 The behavioral effects of a single tDCS session are short, lasting only a few minutes. Multiple sessions can prolong the effects of stimulation for weeks32,33 and are thus used in clinical practice (Table 3).
Table 3
tES method | Pattern | Polarity | Variable parameter | Current (mA) | Duration of each session (minutes) |
---|---|---|---|---|---|
tDCS |
![]() | Polar | Anodic stimulation: excitatory effect | 0.5-02 | 5-30 |
![]() | Polar | Cathodic stimulation: inhibitory effect | 0.5-02 | 5-30 | |
tACS |
![]() | Alternating | Frequency (0.1-640 Hz) | 0.5-02 | 5-30 |
tPCS |
![]() | Alternating | Frequency | 0.5-02 | 5-30 |
tACS = transcranial alternating current stimulation; tDCS = transcranial direct current stimulation; tPCS = transcranial pulsed current stimulation.
Contraindications, adverse events, and safety
The contraindications for rTMS and tDCS are broadly similar, and include presence of ferromagnetic hardware or electronic devices near the stimulation area that might be heated or damaged by the electric currents or electromagnetic pulses.
Repetitive TMS can, in certain conditions, induce seizures. Nonetheless, the number of episodes reported is very small (< 0.1%), and there are no recorded cases of irreversible sequelae or death.20,34 Furthermore, experience gained over the years has allowed the development of safer protocols, which consider frequency of stimulation, train duration, interval between trains, and motor threshold. Seizure risk is associated with higher rMTs, higher stimulation frequencies, higher train duration, and shorter interval between trains.20 For patients who have a history of seizures, LF stimulation is recommended, as it is not associated with seizure induction and might in fact have protective effects.35
Common adverse events of rTMS include pain, headache, and discomfort at the application site,20,34 whereas adverse events of tDCS include tingling, paresthesia, skin redness, and discomfort at the application site. These effects occur in approximately 30% of patients.36 The most common adverse event of tDCS is erythema, which usually occurs in > 80% of cases.37 Patients often do not perceive this effect, although it can be an issue in double-blinded trials. There are no reports of serious adverse events with tDCS.
Finally, meta-analyses have suggested that there is no increased risk of treatment-induced mania/hypomania following rTMS or tDCS38,39 when treating patients with unipolar or bipolar depressive disorder.
Clinical evidence of NIBS efficacy in psychiatric disorders
The evidence of NIBS efficacy in major psychiatric disorders is summarized in Table 4.
Table 4
Disorder/method | Summary |
---|---|
MDD | |
rTMS | Large RCTs and M-As showing antidepressant effects of HF-rTMS and LF-rTMS; M-As showing antidepressant effects of TBS and bilateral rTMS; large RCT showing antidepressant effects for dTMS |
tDCS | Large RCTs and M-As showing antidepressant effects of bifrontal tDCS, although the overall number of studies is low |
Bipolar depression | |
rTMS | M-As showing effects of LF-rTMS and HF-rTMS; small RCT showing effects of dTMS |
Schizophrenia – Auditory hallucinations | |
rTMS | M-As but no large RCT showing clinical effects of LF-rTMS over the left temporoparietal cortex |
tDCS | Small RCT showing clinical effects of cathodal tDCS over the left temporoparietal cortex |
Schizophrenia – Negative symptoms | |
rTMS | One M-A suggesting efficacy of HF-rTMS over the DLPFC, although a large RCT showed no significant results |
Obsessive-compulsive disorder | |
rTMS | M-As, but no large RCTs, showing efficacy of different rTMS modalities for treating OCD; most effective approach seems to be LF-rTMS over the supplementary motor area |
Nicotine addiction | |
rTMS | One large RCT showing efficacy of dTMS over the PFC |
Posttraumatic stress disorder | |
rTMS | Small RCTs showing clinical effects of LF- and HF-rTMS over the DLPFC, regardless of laterality |
Addiction | |
tDCS | Small RCTs suggesting clinical effects for alcohol use, crack/cocaine use, and smoking |
DLPFC = dorsolateral prefrontal cortex; dTMS = deep transcranial magnetic stimulation; HF = high-frequency; LF = low-frequency; M-As = meta-analyses; MDD = major depressive disorder; OCD = obsessive-compulsive disorder; PFC = prefrontal cortex; RCTs = randomized clinical trials.
Major depressive disorder (MDD)
Repetitive transcranial magnetic stimulation (rTMS)
According to the Canadian Network for Mood and Anxiety Treatments (CANMAT),9 rTMS is a first-line treatment for patients in whom at least one antidepressant trial has failed. The recommended stimulation parameters are 110-120% of rMT (70-80% for TBS), five times per week, and 20-30 sessions or fewer if clinical response is obtained.9 HF-, LF-, and bilateral rTMS have high evidence level of efficacy.40
A recent network meta-analysis evaluated the efficacy and tolerability of different rTMS modalities.40 The review included not only the more widespread techniques such as HF-, LF-, and bilateral rTMS, but also TBS, H1-coil (“deep”) TMS, accelerating rTMS (i.e., TMS performed four times a day), priming rTMS, and synchronized TMS.
Figure 1 shows the main results of this network meta-analysis. Each of the eight active treatment modalities was individually compared against sham rTMS. Effect sizes are represented by the odds ratios (ORs) of clinical response (i.e., patients who exhibited > 50% improvement in depression from baseline). Treatments that were more effective than sham presented the lower bound of the confidence interval (CI) of the OR above 1 and included HF-, LF-, bilateral, priming rTMS and TBS. The ORs for priming and bilateral rTMS showed a nonsignificant trend toward superiority compared to the other techniques. Conversely, H1-coil rTMS did not appear to be more effective than sham treatment, despite a large randomized clinical trial (RCT) showing superiority.41 This discrepancy can be explained because the meta-analysis adopted different endpoint criteria, more comparable to the other included studies, than the endpoint used in the RCT. In addition, the meta-analysis was insufficiently powered for comparisons other than with HF-, LF-, and bilateral rTMS, owing to the low number of studies of the different modalities (Figure 1).

The efficacy of rTMS and antidepressants seems to be similar in patients with moderate and high degrees of refractoriness. Two RCTs compared rTMS against a full dose of venlafaxine and found that both therapies had similar efficacy.42,43 Moreover, the combination of rTMS and antidepressant drugs is more effective than rTMS alone.44
Conversely, rTMS is inferior to ECT for treating MDD, especially in psychotic patients.45 Also, rTMS responsiveness is low in ECT-resistant patients, indicating that it should not be used if ECT treatment was not successful.45
Specific subtypes of MDD may respond preferentially to stimulation of left or right DLPFC. For instance, bipolar depression and depression with anxious symptoms might respond better to LF-rTMS over the right DLPFC than HF-rTMS over the left DLPFC.46,47 Deep rTMS using the H1-coil over the left DLPFC was also found to be effective for bipolar depression.48
Finally, rTMS might improve not only depressive symptoms but also performance on cognitive tasks involved in the pathophysiology of depression. A meta-analysis found that these effects on cognitive enhancement are modest and specific to psychomotor speed, visual scanning, and set-shifting ability.49
Transcranial direct current stimulation (tDCS)
There is less evidence for the efficacy of tDCS than for rTMS. Nevertheless, the latest edition of CANMAT9 and a European consensus of leading experts8 considered the technique to be probably effective for treating MDD.
A meta-analysis50 that collected individual data from five centers (in Brazil, Canada, Australia, France, and Germany) found that active tDCS is superior to sham tDCS in terms of response, remission, and depression improvement. Despite this positive finding, the results were influenced by the findings of one large RCT,51 as some of the other studies, possibly due to different sample characteristics and low sample size, did not find superiority of tDCS. The meta-analysis and also identified that treatment-resistant depression was a predictor of poor tDCS response.
The role of tDCS as an augmentative and substitutive treatment for antidepressants was investigated in two large RCTs. The Sertraline vs. Electric Current Therapy for Treating Depression Clinical Study (SELECT-TDCS)51 recruited 120 antidepressant-free patients with moderate to severe depression who were randomized into four groups (2 × 2 design): sham tDCS and placebo, sham tDCS and sertraline, active tDCS and placebo, and active tDCS and sertraline. The parameters were: 2 mA, 30 minutes/day for 2 weeks, and two extra tDCS sessions every 2 weeks until week 6 (end of study). The dose of sertraline was 50 mg/day. The main conclusions were that: 1) combined treatment was significantly more effective than each treatment alone; 2) active tDCS as monotherapy was more effective than placebo; 3) tDCS was well tolerated, with mild adverse effects, although five cases of hypomania/mania were reported in the combined group (Figure 2).
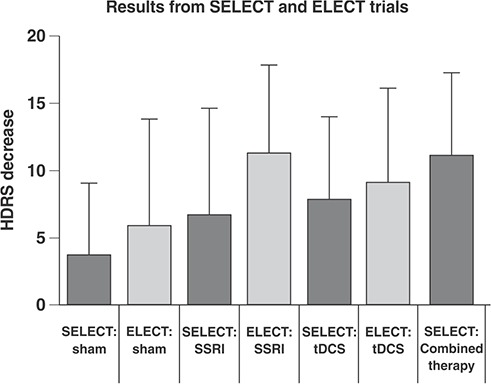
One proposed mechanism of action to justify the synergistic effect of the two interventions is based on neuroimaging findings which showed that depressed patients exhibit relative hypoactivity of some cortical areas (such as the left DLPFC, where tDCS acts) and a relative hyperactivity of some subcortical areas (such as Brodmann area 25), where some antidepressant drugs act.53 Thus, the effect of the two techniques would be potentiated because they would act on different regions of the brain. This hypothesis, however, has not yet been validated by neuroimaging studies.
The finding that tDCS and sertraline were not statistically different is limited, as the sertraline dose was low and the study was not specifically designed to assess noninferiority. This issue was addressed in the Escitalopram vs. Electric Current Therapy to Treat Depression Clinical Study (ELECT-TDCS), which sought was to demonstrate noninferiority of tDCS in relation to a maximum dose of escitalopram (20 mg/day). The noninferiority margin was established as 50% of the efficacy of escitalopram over placebo. In other words, tDCS, compared to escitalopram, would have to retain at least 50% of the efficacy of escitalopram over placebo. ELECT-TDCS had a longer duration than SELECT-TDCS (10 instead of 6 weeks), and more tDCS sessions were applied (22 instead of 12). At the end of the study, 245 patients were randomly assigned to receive escitalopram, tDCS, or placebo.52
ELECT-TDCS failed to demonstrate the noninferiority of tDCS compared to escitalopram. Superiority analyses revealed superiority of escitalopram over tDCS and placebo, and superiority of tDCS over placebo alone. The adverse event profiles also differed between tDCS (more tingling and redness at the stimulation site, two cases of hypomania) and escitalopram (more sedation and constipation)52 (Figure 2). The tDCS protocol in this study only administered sessions five times a week for 3 weeks, followed by tapered sessions over 7 weeks; a more intense stimulation protocol might have produced higher efficacy. Early rTMS studies involving 2 weeks of acute stimulation showed modest results compared to later studies which involved 4 to 6 weeks of stimulation.
The efficacy of tDCS has also been studied in other clinical conditions, such as post-stroke depression54 and bipolar depression (study under review).
Schizophrenia
NIBS techniques have been used for the treatment of auditory hallucinations (AH) and negative symptoms55 -57 in schizophrenia.
Patients with AH have increased regional brain flow in the left hemisphere, particularly in the upper temporal gyrus58; therefore, LF-rTMS or cathodal tDCS over this area has been particularly investigated. A recent meta-analysis56 demonstrated a significant clinical effect of LF TMS over the left temporoparietal region, but no large RCTs have confirmed this finding. Regarding tDCS, preliminary results indicate that the technique may be useful in the treatment of schizophrenia. In a small RCT enrolling 30 patients, anodal stimulation over T3P3 and cathodal stimulation over F3 decreased AH, an effect that was maintained for 3 months.55
HF-rTMS has been used to treat negative symptoms of schizophrenia. For this indication, rTMS is applied over the DLPFC, a brain area that exhibits decreased blood flow and metabolism in those with negative symptoms.59 Although initial results were promising, including one meta-analysis that suggested efficacy,57 a recent RCT recruiting 156 patients with schizophrenia and major negative symptoms did not show superiority of HF-rTMS to the left DLPFC through 105 days of follow-up.60 Studies with small samples of patients receiving tDCS suggested efficacy of the technique (anodal stimulation over the left DLPFC) and association of clinical improvement with increased connectivity of the left DLPFC and the left temporal gyrus.8,55,61
Obsessive-compulsive disorder (OCD)
The role of rTMS in treating OCD is uncertain. A meta-analysis showed that rTMS is more effective than placebo stimulation62; however, it included studies using different protocols and different stimulation sites. Still, the results suggested that LF-rTMS over the supplementary motor area, seeking to reduce excess activity of the fronto-striato-pallido-thalamic circuits, would be the most effective protocol.63
There have been no controlled studies evaluating the efficacy of tDCS in OCD. An ongoing RCT64 is investigating the efficacy of cathodal stimulation over the supplementary motor area at 2 mA. Open studies have shown promising results using this montage.65,66
Anxiety disorders, trauma, and stress disorders
To date, only one RCT has used rTMS in generalized anxiety disorder, finding significant results with LF-rTMS over the right DLPFC.67 Another RCT used rTMS in patients with panic disorder and also reported positive results with the same protocol.68 Three RCTs used rTMS for posttraumatic stress disorder, all with significant results,69-71 regardless of laterality (right or left DLPFC) or intensity (HF or LF).
There have been no RCTs of tDCS in the described disorders.
Addiction
RCTs of rTMS and tDCS for addiction disorders have been few and heterogeneous.72 -78 There is no evidence of rTMS efficacy for any specific addiction, except possible efficacy for nicotine addiction.79 Studies with rTMS have used HF on the left DLPFC. tDCS, in turn, has proved to be more promising for addiction. Four small RCTs have been performed, with nicotine (two studies), crack/cocaine, and alcohol dependence,80 -83 all reporting positive results.
Future perspectives
New therapeutic targets
The DLPFC has been a common target of NIBS for treating depression.84,85 Although it is involved in the pathophysiology of depression,86 it was chosen as a target in early rTMS studies mainly because it was easily accessed, as devices could not reach deeper regions of the brain. In addition, knowledge of other circuits involved in depression was limited.87
Paradoxically, the strongest evidence of prefrontal asymmetry and hypoactivity of the DLPFC in depression comes from rTMS studies themselves.87 Research has been more consistent in showing global prefrontal hypoactivity.53,88 The most consistent evidence is that the DLPFC is involved in “cold” cognitive processes, which, despite being part of the depressive spectrum, are not among the core symptoms of depression.87 Therefore, the antidepressant effects of rTMS on the DLPFC might be indirect, via changes in the connectivity of neural networks.
A possible novel target for future studies of rTMS in depression is the dorsomedial prefrontal cortex (DMPFC). Lesions in this area cause mood disorders in more than 80% of cases.89 A meta-analysis of cerebral morphometry showed consistent changes in the DMPFC – such as gray matter reduction in volumetric studies of depression – and the adjacent anterior cingulate cortex (ACC), while reporting only minimal changes in DLPFC.90 DMPFC is also the converging hub of neural networks involved in cognitive control, affective regulation and self-reflexive thoughts. An open-label clinical trial suggested that rTMS over the DMPFC is at least as effective as over the DLPFC.91
Other areas involved in depression pathophysiology are the frontopolar cortex (FPC), ventromedial prefrontal cortex (VMPFC), and ventrolateral prefrontal cortex (VLPFC), which are hyperactive in depression and are associated with symptomatic improvement when deactivated.87,89 However, the VM and VLPFC are not easily accessible, being 7 cm from the scalp, while the FPC (Brodmann area 10), despite being a superficial area, is under a region where stimulation may be uncomfortable, generating muscular contraction of the face and visual perceptions due to retinal stimulation.87
Biomarkers
The use of biomarkers for NIBS may be useful to identify groups of patients more likely to respond to rTMS compared to another intervention or placebo. To date, most studies investigating biomarkers have been of low methodological quality.92 However, two recent studies indicated possible predictors of response.
The first study93 showed that patients did not respond uniformly, but in a bimodal fashion, to rTMS treatment: at the beginning of treatment, non-responders presented with higher anhedonia and lower connectivity in a brain network classically associated with reward, consisting of the ventral tegmental area, striatum, and part of the VMPFC. This study indicated that a subtype of depressive patients, identified on the basis of syndromic and neuroimaging characteristics, may respond better to rTMS.
The other study27 used functional magnetic resonance imaging (fMRI) in a large, multisite sample of 1,188 depressive patients, and identified four distinct neurophysiological subtypes (“biotypes”) based on distinct patterns of dysfunctional connectivity in frontostriatal and limbic networks. Patients in “biotype 1” were approximately three times more likely to benefit from rTMS over the DMPFC than those in “biotypes 2 or 4.” The authors suggested that there is a “neural signature” associated with clinical response to rTMS.
Conclusion
NIBS techniques, with rTMS and tDCS being the most common methods, are being increasingly used in clinical practice, with substantial progress over the past decades. The most promising clinical results have been obtained when treating MDD and, to a lesser extent, schizophrenia. NIBS modalities are especially advantageous because of their mild side effects and the lack of absolute contraindications. However, the therapeutic effects of NIBS are still modest, possibly due to the limited comprehension of their interaction with brain function in health and disease. Further development of NIBS techniques, particularly tDCS, is crucial for elucidating their role in the therapeutic arsenal against psychiatric disorders.
Disclosure
ARB is a consultant of neuroCare Group GmbH (Munich, Germany). FP has received speaker’s honoraria from Mag & More GmbH and neuroCare Group GmbH, as well as equipment support from neuroConn Technologie, Ilmenau, Germany, Mag & More GmbH, and Brainsway Inc., Jerusalem, Israel. The other authors report no conflicts of interest.
Acknowledgements
ARB is the recipient of a Coordenação de Aperfeiçoamento de Pessoal de Nível Superior (CAPES)/Alexander von Humboldt fellowship award for experienced researchers. The authors thank Associação Beneficente Alzira Denise Hertzog da Silva and CAPES/the Institutos Nacionais de Ciência e Tecnologia (INCT) program for financial support provided to Instituto Nacional de Biomarcadores em Neuropsiquiatria (INBioN).
Footnotes
How to cite this article: Brunoni AR, Sampaio-Junior B, Moffa AH, Aparício LV, Gordon P, Klein I, et al. Noninvasive brain stimulation in psychiatric disorders: a primer. Braz J Psychiatry. 2019;41:70-81. 10.1590/1516-4446-2017-0018
References
Articles from Brazilian Journal of Psychiatry are provided here courtesy of Brazilian Psychiatric Association
Full text links
Read article at publisher's site: https://doi.org/10.1590/1516-4446-2017-0018
Read article for free, from open access legal sources, via Unpaywall:
http://www.scielo.br/pdf/rbp/v41n1/1516-4446-rbp-1516444620170018.pdf
Citations & impact
Impact metrics
Article citations
The distinct and potentially conflicting effects of tDCS and tRNS on brain connectivity, cortical inhibition, and visuospatial memory.
Front Hum Neurosci, 18:1415904, 30 May 2024
Cited by: 0 articles | PMID: 38873654
Concurrent self-administered transcranial direct current stimulation and attention bias modification training in binge eating disorder: feasibility randomised sham-controlled trial.
BJPsych Open, 10(4):e118, 06 Jun 2024
Cited by: 2 articles | PMID: 38840537 | PMCID: PMC11363089
Home-Use Transcranial Direct Current Stimulation for the Treatment of a Major Depressive Episode: A Randomized Clinical Trial.
JAMA Psychiatry, 81(4):329-337, 01 Apr 2024
Cited by: 4 articles | PMID: 38170541
Transcranial Magnetic Stimulation and Transcranial Direct Current Stimulation Across Mental Disorders: A Systematic Review and Dose-Response Meta-Analysis.
JAMA Netw Open, 7(5):e2412616, 01 May 2024
Cited by: 2 articles | PMID: 38776083 | PMCID: PMC11112448
Review Free full text in Europe PMC
The Pathophysiological Underpinnings of Gamma-Band Alterations in Psychiatric Disorders.
Life (Basel), 14(5):578, 30 Apr 2024
Cited by: 0 articles | PMID: 38792599 | PMCID: PMC11122172
Review Free full text in Europe PMC
Go to all (62) article citations
Similar Articles
To arrive at the top five similar articles we use a word-weighted algorithm to compare words from the Title and Abstract of each citation.
The intervention, the patient and the illness - Personalizing non-invasive brain stimulation in psychiatry.
Exp Neurol, 341:113713, 31 Mar 2021
Cited by: 10 articles | PMID: 33798562
Review
Transcranial Magnetic Stimulation and Transcranial Direct Current Stimulation Across Mental Disorders: A Systematic Review and Dose-Response Meta-Analysis.
JAMA Netw Open, 7(5):e2412616, 01 May 2024
Cited by: 2 articles | PMID: 38776083 | PMCID: PMC11112448
Review Free full text in Europe PMC
[Non-invasive brain stimulation techniques in psychiatric disorders: influential factors and combination of interventions].
Tijdschr Psychiatr, 59(10):594-599, 01 Jan 2017
Cited by: 0 articles | PMID: 29077133
Noninvasive brain stimulation for behavioural and psychological symptoms of dementia: A systematic review and meta-analysis.
Int J Geriatr Psychiatry, 34(9):1336-1345, 17 Oct 2018
Cited by: 18 articles | PMID: 30246461
Review