Abstract
Free full text

Microglia in the spinal cord and neuropathic pain
Abstract
In contrast to physiological pain, pathological pain is not dependent on the presence of tissue‐damaging stimuli. One type of pathological pain – neuropathic pain – is often a consequence of nerve injury or of diseases such as diabetes. Neuropathic pain can be agonizing, can persist over long periods and is often resistant to known painkillers. A growing body of evidence shows that many pathological processes within the central nervous system are mediated by complex interactions between neurons and glial cells. In the case of painful peripheral neuropathy, spinal microglia react and undergo a series of changes that directly influence the establishment of neuropathic pain states. After nerve damage, purinergic P2X4 receptors (non‐selective cation channels activated by extracellular adenosine triphosphate) are upregulated in spinal microglia in a manner that depends on the transcription factors interferon regulatory factor 8 and 5, both of which are expressed in microglia after peripheral nerve injury. P2X4 receptor expression on the cell surface of microglia is also regulated at the post‐translational level by signaling from CC chemokine receptor chemotactic cytokine receptor 2. Furthermore, spinal microglia in response to extracellular stimuli results in signal transduction through intracellular signaling cascades, such as mitogen‐activated protein kinases, p38 and extracellular signal‐regulated protein kinase. Importantly, inhibiting the function or expression of these microglial molecules suppresses the aberrant excitability of dorsal horn neurons and neuropathic pain. These findings show that spinal microglia are a central player in mechanisms for neuropathic pain, and might be a potential target for treating the chronic pain state.
Introduction
Neuropathic pain is a chronic pain condition that occurs after nerve damage, such as that induced by bone compression in cancer, infection, autoimmune disease, trauma and diabetes1. In addition to spontaneous pain and hyperalgesia (the increased pain perception of noxious stimuli), a troublesome symptom of neuropathic pain is pain hypersensitivity to normally innocuous stimuli, called tactile allodynia. A considerable proportion of diabetic patients describe abnormal hypersensitivity to normally innocuous stimuli2, 3. Allodynia is often resistant to the currently available drugs when administered at doses that do not produce significant side‐effects4, 5, 6. We are now beginning to understand that neuropathic pain is not just a symptom of disease, but is a consequence of disordered functioning of the nervous system7, 8. Unraveling the mechanisms of pain hypersensitivity caused by nerve damage is therefore essential for the development of new therapeutic drugs for neuropathic pain.
The spinal dorsal horn receives sensory information from primary afferent Aδ and C fibers after nociceptive stimuli (Figure 1)9, 10, 11. Aδ fibers have medium diameter myelinated afferents and mediate acute, localized sharp pain sensation. C fibers have small diameter unmyelinated afferents and convey the poorly localized delayed pain. The largest‐diameter, myelinated primary afferent Aβ fibers transmit innocuous mechanical information (i.e., light touch). The terminals of C and Aδ fibers are concentrated in the superficial dorsal horn, and these fibers activate projection neurons and excitatory interneurons (Figure 1). In contrast, the terminals of Aβ fibers are concentrated in the deeper dorsal horn, and mainly target excitatory and inhibitory interneurons (Figure 1) and projection neurons located in the deeper dorsal horn (not shown). Although Aβ fibers polysynaptically link to projection neurons in the superficial dorsal horn, the link is considered to be normally strongly repressed by inhibitory interneurons. Therefore, under normal conditions, Aβ fibers do not activate nociceptive projection neurons and do not cause pain. However, the neuronal networks in the dorsal horn are modulated and modified under pathological conditions, such as peripheral tissue inflammation and peripheral nerve injury (PNI)9, 11. Accumulating evidence from diverse animal models of neuropathic pain suggests that neuropathic pain might involve aberrant excitability in the dorsal horn, resulting from multiple functional alterations after PNI including loss of function of inhibitory interneurons (e.g., diminished activity of these cells or reduced effectiveness of the inhibitory neurotransmitters γ‐aminobutyric acid and glycine)6, 12. Furthermore, a growing body of evidence has shown that PNI‐induced hyperexcitability might not be a consequence merely of changes in neurons, but rather of multiple alterations in glial cells, such as microglia, the immune cells of the CNS13, 14, 15, 16, 17. In the present article, we highlight recent advances that further increase our understanding of the mechanisms underlying neuropathic pain caused by PNI and diabetes, with a specific focus on microglia in the spinal cord.
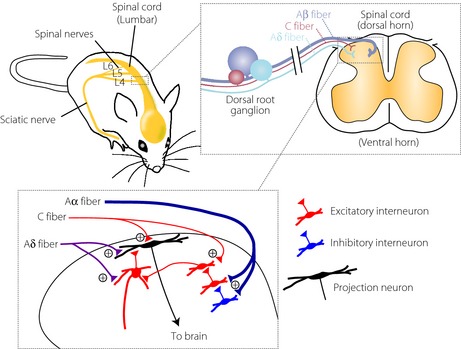
Schematic illustration of primary afferent sensory fibers and neuronal circuits in the dorsal horn. The dorsal root ganglion contains cell bodies of primary afferent neurons that transmit sensory information from the periphery to the spinal dorsal horn. Nociceptive information is mainly mediated by Aδ and C fibers, and innocuous mechanical information is mediated by Aβ fibers. C and Aδ fibers terminate in the superficial dorsal horn, and activate projection neurons and excitatory interneurons. The terminals of Aβ fibers are concentrated in the deeper dorsal horn, and connect to excitatory and inhibitory interneurons.
Microglia in the Spinal Cord in PNI and Diabetic Animals
Glial cells make up over 70% of the total cell population in the central nervous system (CNS), and are classified into astrocytes, oligodendrocytes and microglia. Microglial cells are known as resident macrophages in the CNS, which derive from primitive macrophages in the yolk sac18. In the adult, microglia are ubiquitously distributed throughout CNS and have small cell bodies bearing branched and motile processes, which might monitor the local environment in the CNS19, 20. Microglia rapidly respond to a wide range of stimuli that threaten physiological homeostasis, including PNI. In a growing body of literature, it is evident that PNI leads to a dramatic activation of microglia in the spinal dorsal horn (Figure 2). This response is commonly observed among various models of neuropathic pain including diabetic neuropathy. The morphological features of microglial activation are a cell body hypertrophy with thickened and retracted processes, an increase in cell number and an increase in the staining level of microglial markers, such as CD11b and ionized calcium‐binding adapter molecule‐1 (Figure 2). Using a model of painful diabetic neuropathy caused by streptozotocin (STZ), we found that microglia in the dorsal horn showed hypertrophic morphology, an increase in cell number and in expression of microglial markers. These changes were also consistently observed in other studies21, 22, 23, 24, 25, 26, and also found in a model of type 2 diabetes, db/db mice27. An interesting observation in STZ‐induced diabetic rats was that microglia activation was more pronounced in the fourth lumbar (L4) segment of the dorsal horn than in the L5 or L6 segments. Similar segmental difference has been observed in the dorsal horn in response to injury to tibial and common peroneal nerves28, 29. Furthermore, activated microglia were observed mainly in the medial side of the dorsal horn, an area that has been shown to receive inputs from these nerves28, and also to be innervated by myelinated fibers29, 30. These lines of evidence lead to the possibility that microglia activation might be related to diabetes‐induced damage and/or aberrant activity of a subpopulation of primary afferent sensory neurons projecting to the L4 dorsal horn rather than to a direct action of glucose on microglia. L4 dorsal root ganglion neurons, whose central fibers project to the L4 dorsal horn, are the principal sensory neurons projecting to the hindpaw31. Because tactile allodynia is consistently observed in the hindpaw of diabetic models, activated spinal microglia could be a mechanistic link to diabetes‐induced tactile allodynia. Clinical evidence also indicates that tactile allodynia in patients with diabetes is observed mainly in distal regions of the body, such as feet and ankles32. Mechanisms underlying the spatial‐specific activation of microglia in the dorsal horn remain to be determined. However, the area in the spinal dorsal horn receiving damaged primary afferent sensory neurons matches that having markedly activated microglia, therefore, microglial activation requires a signal(s) related to ‘nerve injury.’ Indeed, blocking peripheral input from primary afferent fibers by bupivacaine inhibits microglial activation in the dorsal horn after PNI33. In STZ‐treated diabetic mice, Suzuki et al.23 showed that continuous systemic administration of lidocaine inhibited microglial activation in the dorsal horn (although they have proposed a direct action of lidocaine on microglia).
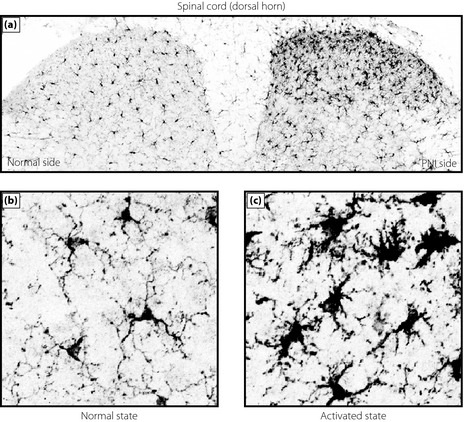
Activation of microglia in the dorsal horn of the spinal cord after peripheral nerve injury. (a) Immunofluorescence of the microglia marker ionized calcium‐binding adapter molecule‐1 in the spinal dorsal horn 7 days after nerve injury. High‐magnified images of (b) normal and (c) activated states of microglia in the contralateral and ipsilateral side, respectively, of the spinal dorsal horn.
A neuronally derived signaling molecule that might be important for microglial activation remains to be determined, but several candidates have been reported. Those include monocyte chemoattractant protein‐1 (MCP‐1 or CCL2) and metalloproteinase‐9 (MMP‐9), whose expressions are markedly increased in dorsal root ganglion neurons after PNI34, 35, 36, 37, 38. Mice lacking chemotactic cytokine receptor 2, which is a receptor for MCP‐1, or MMP‐9‐deficient mice showed a reduction of microglia activation caused by PNI38, 39. Conversely, intrathecal administration of MCP‐1 or MMP‐9 into normal rats produced microglial activation, as well as allodynia37, 38. Substrates of MMP‐9 for microglial activation are unclear, but fractalkine, interleukin‐1β (IL‐1β) and tumor necrosis factor‐α could be potential candidates17.
The role of CCL2 in neuropathic pain might also be associated with its ability to attract and activate monocytes. Zhang et al.39 have shown that bone marrow‐derived cells injected intravenously into lethally irradiated recipient mice were observed in the spinal cord parenchyma ipsilateral to the PNI in a manner that depended on the level of spinal CCL2 expression. Interestingly, bone marrow cells that had migrated into the dorsal horn underwent proliferation, expressed ionized calcium‐binding adapter molecule‐1 and showed microglia‐like morphology. However, the ability of bone marrow‐derived cells to migrate into the parenchyma of the CNS, including the spinal cord remains controversial, as a result of experimental manipulations, such as irradiation (which could influence the blood–spinal cord barrier) and exogenously injected donor cells40. Thus, the extent to which bone marrow‐derived cells contribute to spinal microglia activation after PNI remains unresolved.
It was found that spinal microglia express a receptor for the cytokine interferon‐γ (IFN‐γR), and that stimulating IFN‐γRs in naïve animals by means of intrathecal administration of IFN‐γ produces a hypertrophic morphology and an increase in the microglial number41. Furthermore, IFN‐γR‐knockout mice showed the reduction of morphological and numerical changes of microglia after PNI. Although the source of IFN‐γ remains to be identified, the IFN‐γ/IFN‐γR system is critical in transforming resident spinal microglia into the activated state. Whether IFN‐γ signaling also contributes to microglial activation in diabetic animals remains unknown.
The role of toll‐like receptors, a family of type I transmembrane signaling proteins that recognize pathogen‐associated molecular patterns, has also been reported in PNI models of neuropathic pain. Mice lacking either toll‐like receptor 2, 3 or 4 show impaired microglial activation in the dorsal horn after PNI42, 43, 44.
Spinal Microglia are Crucial for Neuropathic Pain
Activated microglia show dramatic changes in the expression of various genes, including cell‐surface receptors for neurotransmission (e.g., purinergic receptors) and intracellular signaling molecules (e.g., mitogen‐activated protein kinases [MAPKs]) and bioactive diffusible factors (e.g., pro‐inflammatory cytokines and neurotrophic factors).
Purinergic Receptors
In 1972, Burnstock45 proposed new roles of nucleotides as neurotransmitters, even though it was primarily recognized that intracellular adenosine triphosphate (ATP) is the source of free energy to maintain life, and nucleotides are key molecules within cells. In 1993, the first receptors for nucleotides, called P2 purinoceptors, were cloned46, 47. Afterwards, numerous subtypes of these receptors were also cloned, and subsequently scientists began to gradually accept the ‘purinergic system.’ Now purinergic P2 receptors are divided into two families, ionotropic receptors (P2X) and metabotropic receptors (P2Y). P2X receptors (of which there are seven types, P2X1–P2X7) contain intrinsic pores that open on binding of extracellular ATP48. P2Y receptors (of which there are eight types, P2Y1, 2, 4, 6, 11, 12, 13 and 14) are coupled to heteromeric G‐proteins49. Nucleotides are considered to be released or leaked from glial cells as well as neurons, and thus purinergic signaling plays an important role in cell‐to‐cell communications under physiological and pathophysiological conditions50.
It was found that expression of P2X4Rs was upregulated exclusively in microglia after PNI, and that PNI‐induced tactile allodynia was reversed by pharmacological blockade of P2X4Rs in the spinal cord51. It was shown that PNI‐induced pain hypersensitivity depends on ongoing purinergic signaling through microglial P2X4Rs. A marked reduction in neuropathic pain in both mice knocked down and knocked out of P2X4R further demonstrated the necessity of P2X4Rs51, 52, 53. Intrathecal delivery of P2X4R‐stimulated microglia caused normal rats to produce allodynia, indicating the sufficiency of P2X4R14, 51. These findings on the necessity and sufficiency of microglial P2X4Rs provided the first definitive evidence for a causal role of spinal microglia in neuropathic pain. This was supported by the findings in studies using minocycline, a tetracycline antibiotic that has an inhibitory effect on microglial activation. These studies consistently showed that minocycline attenuates both microglial activation and mechanical allodynia in models of neuropathic pain caused by PNI and diabetes24, 54, 55, 56.
The mechanisms by which microglia are crucial for producing neuropathic pain must involve signaling from activated microglia to dorsal horn neurons. It was shown that activation of microglial P2X4Rs stimulates the synthesis and release of brain‐derived neurotrophic factor (BDNF)52, 57, and that BDNF then causes an alteration of transmembrane anion gradient in a subpopulation of dorsal horn lamina I neurons presumably through the downregulation of the neuronal chloride transporter, KCC2, which in turn renders γ‐aminobutyric acid and glycine effects depolarizing, rather than hyperpolarizing, in these neurons (Figure 3). Furthermore, Keller et al.58 have shown that local spinal administration of ATP‐stimulated microglia changes the phenotype of in vivo spinal lamina I output neurons, such that they relay innocuous mechanical input, which could account for allodynia. Thus, P2X4R‐stimulated microglia release BDNF as a crucial factor to signal to lamina I neurons, causing aberrant nociceptive output that contributes to neuropathic pain (Figure 3)8. Interestingly, a decrease in KCC2 expression in the spinal cord has also been reported in STZ‐induced diabetic rats24. The decrease was suppressed by treatment with minocycline. Furthermore, blocking the BDNF action in STZ‐injected rats by tropomyosin‐related kinase B/fragment, crystallizable domain was found to induce moderate effects on mechanical hyperalgesia, although BDNF levels were not increased in STZ‐diabetic rats24. Although P2X4R expression in spinal microglia under diabetic conditions remains unknown, microglial regulation of KCC2 could be a mechanism of diabetes‐induced neuropathic pain.
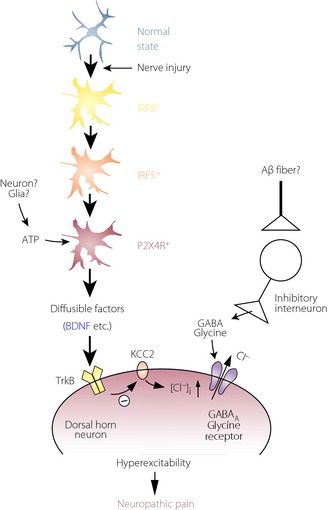
Schematic illustration for shifting spinal microglia toward a P2X4 receptor (P2XR4)‐expressing reactive state through an interferon regulatory factor 8 (IRF8)–IRF5 transcriptional axis after nerve injury, and a potential mechanism by which P2X4+ microglia cause hyperexcitability in dorsal horn neurons and neuropathic pain. After nerve injury, activated microglia show increased expression of IRF8, which in turn leads to induction of IRF5 expression. IRF5 then induces P2X4R expression by directly binding to the promoter region of the P2rx4 gene. P2X4R is activated by extracellular adenosine triphosphate (ATP; which could be presumably released from neurons or glial cells) and, in turn, release bioactive diffusible factors, such as brain‐derived neurotrophic factor (BDNF). BDNF downregulates the potassium‐chloride transporter, KCC2, through tropomyosin‐related kinase B, causes an increase in intracellular [Cl−], and leads to the collapse of the transmembrane anion gradient in dorsal horn neurons, which in turn induces depolarization of these neurons after stimulation by γ‐aminobutyric acid and glycine (which might be released in response to stimulation of Aβ fiber). The resultant hyperexcitability in the dorsal horn pain network induced by microglial factors could be responsible for neuropathic pain. GABA, γ‐aminobutyric acid.
The upregulation of P2X4R expression in microglia is therefore a key process in neuropathic pain. Several studies have identified molecules that upregulate P2X4R expression in microglia59, 60, 61. We have recently identified interferon regulatory factor‐8 (IRF8) as a crucial transcription factor for P2X4R upregulation62. IRF8 is a member of the IRF family (IRF1–9), and is expressed in immune cells, such as lymphocytes and dendritic cells63. The role of IRFs in the CNS was entirely unknown, but Masuda et al.62 recently showed that within the spinal cord, IRF8 expression is selectively upregulated in microglia after PNI. It was also found that IRF8‐deficient mice show a reduction of PNI‐induced tactile allodynia. Furthermore, suppressing upregulated expression of spinal IRF8 caused a significant recovery of the PNI‐induced allodynia. These results show that microglial IRF8 is necessary for the development and maintenance of tactile allodynia after PNI. In in vitro studies, it was shown that IRF8 promotes the expression of genes associated with reactive states of microglia including P2X4R. Furthermore, the PNI‐induced P2X4R upregulation was prevented in IRF8‐deficient mice. More recently, we identified IRF5 as a target of IRF864. IRF5 expression was also induced selectively in spinal microglia after PNI. IRF5‐deficient mice showed substantial resistance to pain hypersensitivity. Interestingly, IRF5 expression in activated microglia led to an induction of P2X4R expression by directly binding to the promoter region of the P2rx4 gene. Consistent with this, IRF5‐deficient mice did not upregulate spinal P2X4R after PNI. Thus, an IRF8–IRF5 transcriptional axis could contribute to shifting spinal microglia toward a P2X4R‐expressing reactive state after PNI (Figure 3)65, 66.
Other transcription factors regulating the states of microglia have also been reported. For example, the E26‐transformation‐specific family transcription factor and master regulator of myeloid development, PU.1, is expressed in the microglia of adult CNS tissue67. PU.1 has been shown to control function and phenotype of human brain microglia68. Furthermore, expression of spinal cord PU.1 is markedly increased after PNI69. Likewise, Runt‐related transcription factor 1 has been reported to regulate the activity state of microglia70, and its expression in the spinal microglia is also upregulated after PNI70. These microglial transcription factors might play a part in altering microglial phenotype and modulating pain signaling. However, their roles in the pathogenesis of neuropathic pain remain unknown and thus require further investigation, which could provide new insights into the molecular mechanisms underlying microglial activation and neuropathic pain.
To detect extracellular ATP, P2X4Rs are necessary to be expressed on the cell surface of microglia. Surprisingly, a large amount of P2X4R protein within microglia (and macrophages) localizes predominantly to intracellular lysosomal compartments71, and P2X4R protein remains stable within the proteolytic environment of lysosomes. How P2X4R protein is recruited to the cell surface of microglia remains elusive, but recent studies have shown that trafficking of P2X4R protein to the cell surface occurs when microglia are stimulated by a toll‐like receptor 4 agonist, lipopolysaccharide72, 73, or the Ca2+ ionophore, ionomycin71. Furthermore, the chemokine CCL2 increased P2X4R protein levels on the cell surface (without changing total cellular expression) through chemotactic cytokine receptor 274. Notably, CCL2 changed the distribution of lysosomes with P2X4R protein within microglial cells and induced the release of a lysosomal enzyme74. Thus, CCL2 might promote the expression of P2X4R protein on the cell surface of microglia through exocytosis of P2X4R‐containing lysosomes. A recent study using single‐molecule imaging to track P2X4Rs in the processes of microglia showed that lateral mobility of P2X4Rs is enhanced in activated microglia by the p38, a member of the MAPKs, pathway that selectively regulates slowly mobile P2X4Rs75. These results showed that microglial P2X4Rs are dynamically regulated on the cell surface. Thus, post‐translational regulation to enhance P2X4R expression and mobility on the cell surfaces of microglia might render cells hyperresponsive to extracellular ATP, which might be important in neuropathic pain.
MAPKs
In response to activation of cell‐surface receptors on spinal microglia by extracellular ligands, a variety of cellular responses occur through activation of intracellular signaling cascades. p38 was activated in spinal microglia after PNI, and contributes to neuropathic pain76, 77. p38 activation in spinal microglia has been shown in different animal models of neuropathic pain78, 79, 80, 81, including diabetic models23, 26. Furthermore, pharmacological inhibition of p38 activity by repeated injection or by infusion of p38 inhibitors attenuated tactile allodynia caused by PNI76, 77, 82, 83 and by diabetes21, 84. Thus, activation of this kinase is necessary for the pathogenesis of neuropathic pain. It remains unclear how nerve damage activates p38 in spinal microglia. A blockade of the sciatic nerve using bupivacaine before PNI prevented p38 activation in spinal microglia85, raising the possibility that activity in the peripheral nerve is required for microglial p38 activation. It was found that cathepsin S rapidly induces p38 phosphorylation in spinal microglia after intrathecal injection, an effect that is dependent on fractalkine and its receptor signaling86, 87. In addition, the purinergic P2Y12R might also be involved in p38 activation in spinal microglia88.
In contrast, the other member of MAPKs extracellular signal‐regulated protein kinase (ERK) in the spinal cord was found in dorsal horn neurons soon after nerve damage, then predominantly in microglia for the next several days and in astrocytes after 3 weeks89. In contrast, the sequential activation of spinal ERK was not observed in the spinal cord of a model of diabetic neuropathic pain90, in which ERK activation consistently occurred in microglia at least for 4 weeks. Furthermore, inhibition of ERK phosphorylation in the dorsal horn produced a striking alleviation of existing, long‐term tactile allodynia of diabetic rats. Thus, activated microglia could be a crucial component of PNI‐ and diabetes‐induced tactile allodynia, mediated, in part, by the ERK signaling pathway. However, Xu et al.27 have shown in db/db mice that ERK activation occurs in neurons and astrocytes in the spinal cord, and that inhibition of ERK also attenuated mechanical allodynia. Thus, the type of cells that ERK is activated in might be different among models of diabetes.
Potential candidates for intermediary molecules involved in MAPK‐dependent pain modulation are thought to be pro‐inflammatory cytokines, such as IL‐1β, IL‐6 and tumor necrosis factor‐α13, 14, 15, 91, 92. Inhibiting spinal p38 suppresses IL‐1β upregulation in the spinal cord of PNI animals93. C‐Fiber‐evoked responses, N‐methyl‐D‐aspartate receptor‐mediated responses, and wind‐up in wide‐dynamic‐range dorsal horn neurons are enhanced by IL‐1β94, 95. IL‐1β has also been reported to decrease γ‐aminobutyric acid A receptor‐mediated currents96. A powerful role of these cytokines in excitatory or inhibitory synaptic transmission and on neuronal activity in the superficial dorsal horn neurons has been shown97, 98. Also, the inhibition of microglial ERK decreases the hyperresponsiveness of dorsal horn neurons in spinal cord‐injured rats through a reduction of prostaglandin E2 production99.
Conclusion
We have primarily focused on the role of microglia in neuropathic pain caused by PNI and diabetes. A model of mechanisms underlying microglia‐mediated neuropathic pain modulation in the dorsal horn is presented in Figure 3. Importantly, pharmacological, molecular and genetic manipulations of the function or expression of these microglial molecules have substantially influenced pain behaviors and hyperexcitability of the dorsal horn pain pathway. Therefore, spinal microglia critically contribute to pathologically enhanced pain processing in the dorsal horn, and microglial molecules might be promising targets for treating neuropathic pain. In addition to microglia, recent studies have also identified astrocyte‐specific molecules, and have shown a critical role of spinal astrocytes in neuropathic pain27, 38, 100, 101, 102, 103. Interestingly, it was found that oral administration of gabapentin attenuated activation of microglia and astrocytes in STZ‐induced diabetic rats21, raising the possibility that gabapentin could exert its anti‐allodynic actions partially through alterations of activation of glial cells in the spinal cord. It is expected that increased understanding of the functions of microglial molecules will provide us with exciting insights into pain mechanisms and clues to develop new therapeutic agents for the management of neuropathic pain.
Notes
J Diabetes Investig 2016; 7: 17–26 [Europe PMC free article] [Abstract] [Google Scholar]
References
Articles from Journal of Diabetes Investigation are provided here courtesy of Wiley
Full text links
Read article at publisher's site: https://doi.org/10.1111/jdi.12379
Read article for free, from open access legal sources, via Unpaywall:
https://onlinelibrary.wiley.com/doi/pdfdirect/10.1111/jdi.12379
Citations & impact
Impact metrics
Article citations
Mechanisms and Therapeutic Prospects of Microglia-Astrocyte Interactions in Neuropathic Pain Following Spinal Cord Injury.
Mol Neurobiol, 29 Oct 2024
Cited by: 0 articles | PMID: 39470872
Review
Diabetic Neuropathic Pain and Circadian Rhythm: A Future Direction Worthy of Study.
J Pain Res, 17:3005-3020, 16 Sep 2024
Cited by: 1 article | PMID: 39308994 | PMCID: PMC11414757
Review Free full text in Europe PMC
The relationship between changes in inflammation and locomotor function in sensory phenotypes of central neuropathic pain after spinal cord injury.
Pain Rep, 9(6):e1184, 10 Oct 2024
Cited by: 0 articles | PMID: 39399305 | PMCID: PMC11469887
Pregnancy ameliorates neuropathic pain through suppression of microglia and upregulation of the δ-opioid receptor in the anterior cingulate cortex in late-pregnant mice.
J Anesth, 08 Sep 2024
Cited by: 0 articles | PMID: 39244720
Metabolomic and lipidomic profiling of the spinal cord in type 2 diabetes mellitus rats with painful neuropathy.
Metab Brain Dis, 39(6):1117-1130, 09 Jul 2024
Cited by: 0 articles | PMID: 38980579 | PMCID: PMC11349861
Go to all (115) article citations
Data
Data behind the article
This data has been text mined from the article, or deposited into data resources.
BioStudies: supplemental material and supporting data
Similar Articles
To arrive at the top five similar articles we use a word-weighted algorithm to compare words from the Title and Abstract of each citation.
P2X4 receptors and neuropathic pain.
Front Cell Neurosci, 7:191, 28 Oct 2013
Cited by: 76 articles | PMID: 24191146 | PMCID: PMC3808787
Review Free full text in Europe PMC
Catestatin is involved in neuropathic pain mediated by purinergic receptor P2X4 in the spinal microglia of rats.
Brain Res Bull, 142:138-146, 21 Jul 2018
Cited by: 7 articles | PMID: 30016729
Purinergic signaling in microglia in the pathogenesis of neuropathic pain.
Proc Jpn Acad Ser B Phys Biol Sci, 93(4):174-182, 01 Jan 2017
Cited by: 21 articles | PMID: 28413195 | PMCID: PMC5489427
Review Free full text in Europe PMC
Neuron-microglia interaction by purinergic signaling in neuropathic pain following neurodegeneration.
Neuropharmacology, 104:76-81, 29 Aug 2015
Cited by: 50 articles | PMID: 26327676
Review