Abstract
Free full text

Paediatric pulmonary arterial hypertension: updates on definition, classification, diagnostics and management
Abstract
Paediatric pulmonary arterial hypertension (PAH) shares common features of adult disease, but is associated with several additional disorders and challenges that require unique approaches. This article discusses recent advances, ongoing challenges and distinct approaches for the care of children with PAH, as presented by the Paediatric Task Force of the 6th World Symposium on Pulmonary Hypertension. We provide updates of the current definition, epidemiology, classification, diagnostics and treatment of paediatric PAH, and identify critical knowledge gaps. Several features of paediatric PAH including the prominence of neonatal PAH, especially in pre-term infants with developmental lung diseases, and novel genetic causes of paediatric PAH are highlighted. The use of cardiac catheterisation as a diagnostic modality and haemodynamic definitions of PAH, including acute vasoreactivity, are addressed. Updates are provided on issues related to utility of the previous classification system to reflect paediatric-specific aetiologies and approaches to medical and interventional management of PAH, including the Potts shunt. Although a lack of clinical trial data for the use of PAH-targeted therapy persists, emerging data are improving the identification of appropriate targets for goal-oriented therapy in children. Such data will likely improve future clinical trial design to enhance outcomes in paediatric PAH.
Short abstract
State of the art and future perspectives in paediatric pulmonary hypertension with special emphasis on classification, diagnosis and treatment http://ow.ly/uVPo30mksOj
Introduction
Pulmonary hypertension (PH) in children is associated with diverse diseases with onset at any age. The distribution of aetiologies in paediatric PH is quite different to that of adults, with children having a greater predominance of idiopathic pulmonary arterial hypertension (IPAH), pulmonary arterial hypertension (PAH) associated with congenital heart disease (PAH-CHD) and developmental lung diseases. Differences in aetiology, presentation and outcomes require a unique approach in children. The management of children remains challenging because treatments have long depended on evidence-based adult studies and the clinical experience of paediatric experts. Although there is still a lack of data on effectiveness, formulation, pharmacokinetics, optimal dosing and treatment strategies, data are emerging that allow for the definition of appropriate treatment targets and goal-oriented therapy in children. Nevertheless, children with PAH are currently treated with targeted PAH drugs with benefit. We provide an overview of recent updates in the current definition, epidemiology, classification, diagnostics and treatment of PAH in children, and identify current needs based on discussions and recommendations from the Paediatric Task Force of the 6th World Symposium on Pulmonary Hypertension (WSPH) in Nice, France (2018).
Definitions
Historically, the definition of PH in children has been the same as in adults, i.e. mean pulmonary arterial pressure (mPAP) ≥25 mmHg. In the normal fetal circulation, PAP is similar to systemic pressure and rapidly falls after birth, achieving levels that are similar to the adult by 2–3 months of age. Due to variability in pulmonary haemodynamics during post-natal transition, paediatric PH has been defined as mPAP ≥25 mmHg after 3 months of age. In paediatric PH, especially in association with CHD, it is recommended to use pulmonary vascular resistance (PVR) as indexed to body surface area (PVRI) in order to assess the presence of pulmonary vascular disease (PVD), as defined by PVRI ≥3 WU·m2.
The 6th WSPH proposed to modify the definition for PH in adults as mPAP >20 mmHg and to include PVR ≥3 WU to identify pre-capillary PH. Whether or not the same findings of high normal or “borderline PH” with mPAP of 21–24 mmHg in subgroups of adult PH such as scleroderma, chronic obstructive pulmonary disease and interstitial lung disease is a risk factor for developing PAH and related morbidities in children as in adults will require further study [1]. However, in order to speak a universal language and facilitate transition from paediatric to adult PH care, the Paediatric Task Force chose to follow the newly proposed adult definition of PH and encourages further study of these patients. The proposed use of PVR to assess PVD at the 6th WSPH had already been included previously in the haemodynamic assessment of PH in children. The Paediatric Task Force further reinforced the need for indexing of PVR in children.
Vasoreactivity
In IPAH/heritable PAH (HPAH), acute vasodilator testing (AVT) is recommended to identify patients who are likely to have a good long-term prognosis when treated with long-term calcium channel blocker (CCB) therapy. In the recent past, positive vasoreactivity was believed to be more common in children with IPAH when compared with adults and that specific response criteria were indicated for children. However, using the same criteria for adult and paediatric subjects with IPAH/HPAH showed that the proportion of AVT responders was similar in both age groups [2]. A recent large study including 382 patients showed a substantial discrepancy in how AVT is performed and interpreted in children, and that standardisation is required [2]. As in adults, inhaled nitric oxide at 10–80 ppm is the preferred agent, but i.v. epoprostenol, i.v. adenosine or inhaled iloprost may be used as alternatives. However, optimal dosing in small children is not well defined for the latter drugs. As recently reported, the Sitbon criteria for positive AVT, as defined by a decrease in mPAP by at least 10 mmHg to a value of <40 mmHg with sustained cardiac output that is commonly used in adult IPAH/HPAH, have been shown to identify children who will show sustained benefit from CCB therapy [2, 3]. Positive AVT in subjects with mPAP <40 mmHg at baseline is defined by a drop of at least 10 mmHg without a fall in cardiac output. Based on these data it is advised to use the Sitbon criteria for AVT in children. Since it has been shown that only half of the adult responders have a long-term haemodynamic and clinical improvement on CCB therapy, close long-term follow-up is required.
Can AVT predict operability if resting PAP and PVR are elevated in children with CHD and open systemic-to-pulmonary shunts?
In CHD-associated PH, AVT is often performed for other reasons than determining the potential use of CCB therapy and predictor of outcome, as shown in IPAH/HPAH. AVT is also used to distinguish between reversible and progressive PAH in patients with PAH-CHD, and thus potential operability [4]. However, specific criteria for defining a positive AVT response or specific haemodynamic targets that predict reversal of PAH and good long-term prognosis following surgical correction remain lacking. In fact, other factors beyond the haemodynamic response to AVT have been shown to be associated with PAH reversal after surgical repair, including age, type of cardiac lesion, comorbidities, resting and exercise saturation, and clinical history. In the absence of robust data on haemodynamic predictors, current guidelines suggest criteria for operability of CHD in the presence of PAH that are based on expert opinion. The Paediatric Task Force agreed on general guidance for assessing operability in CHD-PAH, but emphasises that the long-term impact of defect closure in the presence of PAH with increased PVR is unknown (table 1).
TABLE 1
Guidance for assessing operability in pulmonary arterial hypertension associated with congenital heart disease
Pulmonary vascular resistance index WU·m2 | Pulmonary vascular resistance WU | Correctability/favourable long-term outcome |
<4 | <2.3 | Yes |
4–8 | 2.3–4.6 | Individual patient evaluation in tertiary centres |
>8 | >4.6 | No |
WU: Wood Units. Special considerations include: age of patient, type of defect, comorbidities, resting or exercise-induced desaturation is a concern and PAH therapy (treat with intent-to-repair approach has not been proven).
Updates in paediatric PH epidemiology and classification
Current epidemiological data on paediatric PH are mainly derived from registry cohorts; as a result they are affected by study design, logistics and the scope of clinical practice underlying patient selection for these registries. Geographic coverage, referral patterns, inclusion criteria and disease definitions may differ between registries, leading to potential selection bias that can affect reported incidence and prevalence rates. The estimated incidence of sustained PH in all categories was reported at 4–10 cases per million children per year with a prevalence of 20–40 cases per million in Europe (Spain, the Netherlands) and 5–8 cases per million children per year and 26–33 per million children in the USA [5–7]. A Dutch nationwide epidemiological study, that minimised potential bias by including all hospitals in the country by combining hospital registries with local paediatric cardiology databases over a 15-year period, reported an annual incident rate of PH in children of 63.7 per million children [6]. The majority of children (2845 out of 3262) had “transient” PAH and were infants with either persistent PH of the newborn (PPHN) or repairable cardiac shunt defects. Of the remaining children, 27% had other forms of PAH (IPAH, PAH-CHD, PAH associated with connective tissue disease (CTD) and pulmonary veno-occlusive disease (PVOD)), while a significant proportion (34%) had PH associated with developmental lung disease, including bronchopulmonary dysplasia (BPD), congenital diaphragmatic hernia (CDH) and congenital pulmonary vascular abnormalities [6].
Group 1: PAH
Group 1.1: IPAH
Estimated incidence rates for IPAH vary from 0.47 to 1–2 cases per million children, with estimated prevalence rates from 2.1 to 4.4 cases per million children [5–9].
Group 1.2: HPAH
As in adult PAH, gene mutations that have been implicated in the pathogenesis of HPAH have been identified in 20–30% of paediatric sporadic PAH cases and 70–80% of familial PAH cases. These include known mutations such as those in BMPR2 (bone morphogenetic protein receptor type 2) and ACVRL1 (activin receptor-like kinase 1). However, compared with adult PAH, the genetic architecture of paediatric PAH differs and seems enriched in TBX4 and ACVRL1 mutations [10–13]. Whether mutation carriers in paediatric PAH have a different phenotype or clinical course than non-carriers remains to be demonstrated [11]. Furthermore, paediatric PAH is frequently associated with chromosome and syndromic anomalies, in which the mechanistic basis for PAH is generally uncertain. A recent exome sequencing study in paediatric PAH suggests that de novo variants in novel genes may explain approximately 19% of paediatric-onset IPAH cases. The prevalence of known PAH gene mutations in PAH-CHD is controversial as several studies have not detected PAH mutations in these patients, whereas other groups have identified BMPR2 mutations in patients presenting with PAH after correction of a defect [11, 14], and a recent study shows variants in SOX17 associated with PAH-CHD [15]. Genetic testing is still not performed routinely in all paediatric PH as it may lead to significant psychological impacts, particularly in asymptomatic individuals, especially in the setting of incomplete penetrance. Genetic testing should be combined with genetic counselling by experts in this field so that families have all information before and after testing.
The genetics of PH and genetic testing specifically in paediatrics requires further work and should be performed in expert centres with a genetic counselling group.
Group 1.3: Drug- and toxin-induced PAH
Several cases of transient PAH that resolved after discontinuation of diazoxide have been described in the literature, suggesting that hyperinsulinaemic and hypoglycaemic neonates treated with diazoxide require echocardiographic surveillance [16]. The US Food and Drug Administration (FDA) issued a warning about diazoxide and PAH in neonates in 2015 [17].
Group 1.4: Associated PAH
Group 1.4.1: PAH associated with CTD
PAH-CTD in children is uncommon, but deterioration is usually rapid when associated with PAH. PAH-CTD occurs in 0–4% of patients in PH clinics [5–7, 18–21]. In a multinational cohort of 389 children with systemic juvenile arthritis from the CARRA registry, 16 (4%) were diagnosed with PAH [18]. A recent study suggests that PAH was diagnosed by echocardiography in 2% of a cohort of 850 children with systemic lupus erythematosus within the first 2 years of diagnosis, These patients with PH were mostly asymptomatic and, in some cases, the PAH resolved or improved [19].
Group 1.4.2: PAH associated with HIV infection
PAH-HIV in children appears to be rare outside of endemic areas, with one case in each of the Spanish, Dutch and UK registries [5, 6, 22].
Group 1.4.3: PAH associated with portal hypertension
Patients with liver disease suffer from two distinct pulmonary vascular complications: hepatopulmonary syndrome (HPS) and portopulmonary hypertension (POPH). Whereas HPS is characterised by increased pulmonary blood flow, low PVR and hypoxaemia, POPH has striking pulmonary vascular remodelling that adversely affects the outcome of orthotopic liver transplantation [23]. POPH appears to be rare in children, with 0–2% of cases reported in PH registries [5, 6, 22, 24].
Group 1.4.4: Congenital heart disease
Group 1.4.4 PAH includes patients with simple operable and inoperable CHD, subgrouped as those with Eisenmenger physiology, those with PAH and left-to-right shunts, those with PAH thought to be incidental to their CHD and those with postoperative/closed defects. This classification for PAH associated with cardiac or arterial shunt has not changed since the previous WSPH 2013 classification [25]. Transient PH following repair of congenital heart disease occurs in 21.9 cases per million and is one of the commonest forms of PAH in children, second only to persistent pulmonary hypertension of the newborn [6]. Complex heart diseases have been assigned to group 5.4.
Group 1.4.5: Schistosomiasis
Schistosomiasis is uncommon in developed countries and lacks studies of targeted PH therapy in children.
Group 1.5: PAH long-term responders to CCBs
As in adults, a subgroup of children with IPAH can be identified who are positive AVT responders and would now be classified as “PAH long-term responders to CCBs” [25]. Based on the Sitbon criteria, this subgroup is estimated to include roughly 8–15% of the children with IPAH.
Group 1.6: PAH with overt features of venous/capillaries (PVOD/PCH) involvement
PAH with overt features of venous/capillaries (PVOD/pulmonary capillary haemangiomatosis (PCH)) involvement in children appears to be rare. PVOD and/or PCH was diagnosed in 0.7–2% cases of PAH in the Spanish, Dutch and TOPP registries [5, 6, 26]. The EIF2AK4 (eukaryotic translation initiation factor 2α kinase 4) mutation was present in two-thirds of children diagnosed with PVOD in France [11].
Group 1.7: Persistent PH of the newborn syndrome
PPHN is the most common cause of transient PAH (30.1 cases per million children per year) and may be increasing in frequency [7]. In the era before inhaled nitric oxide therapy, PPHN occurred in approximately 2 per 1000 live births [27]. In contrast, between 2003 and 2012, the prevalence of PPHN among 12954 extremely pre-term infants enrolled was 8.1% (95% CI 7.7–8.6%), with the trend increasing annually, in part due to increased survival of extremely low-birthweight infants and growing awareness of PPHN in pre-terms. The proportion of newborns with PPHN is inversely related to gestational age, with an incidence of 18.5% (range 15.2–22.4%) for infants born at 22–24 weeks compared with 4.4% (range 3.8–5.2%) for those born at 27 weeks [28]. The current WSPH Paediatric Task Force emphasised that PPHN is a syndrome with multiple associated conditions (table 2). Although multifactorial in origin, recent epidemiological studies show that PPHN is associated with ante-natal events, including pre-eclampsia, chorioamnionitis and other peri-natal events, leading to abnormal pulmonary vascular growth and function, and perhaps increasing the risk for PAH later in life [26].
TABLE 2
Persistent pulmonary hypertension of the newborn (PPHN) and associated disorders
Idiopathic PPHN | Myocardial dysfunction (asphyxia, infection) |
Down syndrome | Structural cardiac diseases |
Meconium aspiration syndrome | Hepatic and cerebral arteriovenous malformations |
Respiratory distress syndrome | |
Transient tachypnoea of the newborn | Associations with other diseases: |
Pneumonia/sepsis | ![]() |
Developmental lung disease | ![]() |
Peri-natal stress | ![]() |
Group 2: PH due to left heart disease
Very little epidemiological data are available on this condition in children; however, left ventricular diastolic dysfunction and impaired myocardial performance can contribute to PH severity in diverse settings, including PPHN, BPD and CDH. Congenital left heart inflow/outflow obstructions are common in children with CHD, and outcome is dependent on aetiology and the stage in pulmonary vascular development that the obstruction occurs. Pulmonary vein stenosis, which has a very poor prognosis, can complicate the course and is emerging as an important cause of sustained PH, especially in the setting of ex-premature infants with BPD [29–32]. Table 3 shows congenital post-capillary obstructive lesions most frequent in childhood now classified as group 2.4 PH
TABLE 3
Congenital post-capillary obstructive lesions (group 2.4)
Pulmonary vein stenosis |
![]() |
![]() |
Cor triatriatum |
Obstructed total anomalous pulmonary venous return |
Mitral/aortic stenosis (including supra/subvalvular) |
Coarctation of the aorta |
Group 3: PH due to lung diseases and/or hypoxia
Group 3.5: Developmental lung disorders
This category comprises an important and increasingly recognised proportion of children with PH. BPD is a common developmental disorder of prematurity that is characterised by impaired alveolar and vascular growth and maturation. Past registry data report that 10–12% of children with PH have associated lung disease, with BPD being the most common disorder [26, 33]. This might likely be an underrepresentation of its frequency due to bias in registry enrolment, as previously mentioned. The Netherlands epidemiological study revealed that 34% of patients with sustained PH have developmental lung disease. The incidence and prevalence of severe BPD and PH increase with increasing survival of 23–26-week pre-terms. In a prospective study, PH at 7 days of age was present in 42% of premature babies (birthweight 500–1250 g) and was associated with late PH (at 36 weeks corrected age) in 14%, worse severity of BPD, longer need for mechanical ventilation and neonatal intensive care unit hospitalisation, and higher mortality [34, 35]. In children with BPD, PH can resolve with respiratory and PH-targeted drug therapy over time; however, even in the surfactant era the morbidity and mortality of PH in BPD infants remains high. Recent meta-analyses found that the presence of PH in premature born infants was strongly associated with mortality (risk ratio 4.7) with an accumulative estimated mortality rate of 16% prior to discharge and of 40% during the first 2 years of life. However, the same meta-analyses identified that most reports have studied selected patient populations, and accurate estimates of incidence and prevalence rates later in life are lacking [36]. No trials have yet been conducted to formally assess the effect of PH-specific therapies on children with BPD.
Table 4 provides a summary of developmental lung disorders that share the common feature of developmental vascular disturbances.
TABLE 4
Developmental lung disorders associated with pulmonary hypertension
Bronchopulmonary dysplasia |
Congenital diaphragmatic hernia |
Down syndrome |
Alveolar capillary dysplasia with “misalignment of veins” (FOXF1) |
Lung hypoplasia, acinar dysplasia |
Surfactant protein abnormalities |
![]() |
![]() |
![]() |
TTF1/NKX2-1 |
TBX4 |
Pulmonary interstitial glycogenesis |
Pulmonary alveolar proteinosis |
Pulmonary lymphangiectasia |
Group 4: PH due to pulmonary artery obstructions
Chronic thromboembolic PH remains uncommon as a cause of PAH in children, at around 0–1.4% of cases [22, 26]. In contrast, pulmonary artery obstructions occur in a number of CHDs, either congenitally or acquired after corrective surgery [25].
Group 5: PH with unclear and/or multifactorial mechanisms
Group 5.4: Complex CHD
This group includes haematological disorders, systemic and metabolic disorders, others, and complex CHD. Of specific interest for the paediatric age group are complex heart diseases that are associated with congenital anomalies of the pulmonary vasculature such as segmental disorders, single ventricle physiology and the scimitar syndrome (table 5). PH in these settings is extremely difficult to define or classify [37]. After extensive discussion at the 5th WSPH in 2013, the Paediatric Task Force agreed to classify several anomalies with differential pulmonary blood flow under the category of “segmental PH”, indicating the distinct nature of these entities when compared with other forms of PH [38, 39].
TABLE 5
Complex congenital heart disease (group 5.4)
Segmental pulmonary hypertension |
![]() |
![]() |
![]() |
![]() |
![]() |
Single ventricle |
![]() |
![]() |
Scimitar syndrome |
During the current 6th WSPH, the Paediatric Task Force also considered including patients with single ventricle physiology as representing yet another difficult group to define; this group continues to increase and represents a significant proportion of subjects with PVD at major medical centres. At various stages these patients may have increased or decreased pulmonary blood flow, and as they reach an age suitable for the Fontan procedure or total cavo-pulmonary connection these subjects have variable degrees of PVD and bronchopulmonary collaterals. Subsequently, the chronic non-pulsatile pulmonary circulation associated with a Fontan circulation likely induces a very specific form of PVD that is dissimilar to PVD in other diseases associated with PH [40]. Patients with Fontan circulation do not usually fulfil the definition of PH with mPAP >20–25 mmHg and, accordingly, these patients were previously excluded from the official WSPH classification. Nevertheless, these patients develop PVD that markedly impacts survival and, according to the Paediatric Task Force, PVD in the setting of single ventricle physiology deserves inclusion and has been classified with other forms of group 5 PH. The nature and mechanisms underlying the pathobiology of PVD in this setting urgently require further investigation.
In these complex CHD categories (group 5.4), the general definition of PH does not suffice and should be customised. At present, there is insufficient data showing that targeted therapies are safe and efficient in this population, and further studies are required [41, 42].
Additional special considerations for paediatric clinical classification
The WSPH classification for PH was originally designed for adults with PH. The rationale for a clinical classification includes the ability to strengthen clinical practice, including enhanced diagnostic and management strategies, and to help provide guidance for prioritising laboratory, translational and epidemiological research questions. In addition, goals for improving classification systems include the need for clarification of disease phenotype, encouraging new thinking on causation and disease pathobiology, enhancement of diagnostic evaluations, improvements in correlations of phenotype and therapeutic responsiveness, and enhancement of clinical trial design.
While the major categories of PH as classified by the WSPH have been shown to be helpful also in neonates and children, there are persistent and important gaps that should be considered to improve their utility in these specific age groups.
In 2013, the first WSPH Paediatric Task Force reasoned that a common classification for both adults and children is preferred, since children with PH who were diagnosed in the neonatal through adolescent age ranges are now surviving into adulthood and such classification will facilitate transition from paediatric to adult services. They then proposed several modifications in order to highlight aspects of paediatric disorders and better address specific features of paediatric PH within the core of the existing classification. These modifications included the designation of PPHN as a subclass within group 1 disorders, more detailed categorisation of PAH in CHD, the addition of congenital left heart inflow and outflow tract to group 2, and the introduction of the category of “developmental lung disease” to group 3 and of “segmental PH” to group 5.
In 2018, the WSPH Paediatric Task Force aimed to further capture specific paediatric features in the WSPH clinical classification, while preserving the main core of the classification as given in table 2 of the Task Force article by Simonneau et al. [25] in this issue of the European Respiratory Journal. They proposed additional further refinements of these groups as discussed in the previous subsections, including a separate designation for congenital/acquired cardiovascular conditions leading to post-capillary PH (group 2.4), developmental lung disorders (group 3.5), other pulmonary artery obstructions (group 4.2) and complex CHD (group 5.4)
Recent observational paediatric data reveal that PAH in “older” children, despite specific differences, shares many common features with adult PAH. However, PH presenting in neonates is often associated with developmental vascular abnormalities and responses, and in the current classification is assigned to group 3 PH associated with lung disease and/or hypoxia. These PVDs are much less comparable to adult PH, since the impact of PH on the immature, developing lung is recognised as a major factor integral to the presentation, diagnosis, response to therapy and outcome, both immediate and long term. It is clear that although paediatric and adult PH share common features, the aetiology, epidemiology and presentation of neonatal and paediatric PVD differ significantly from those in adults. One of the distinguishing features of PH in children is the injury of the developing fetal, neonatal and paediatric lung circulation [43]. Another distinguishing feature is the frequent association with chromosome, genetic and syndromic anomalies (11–52%), and the phenotypic associations that may result in multifactorial causes of PH in up to 33% of cases [5, 44].
The current WSPH Paediatric Task Force therefore proposed to designate the developmental (vascular) lung disorders as a special subcategory within group 3 PH (group 3.5). In addition to the recognised frequency and importance of PVD and PH in disorders such as BPD and CDH, this category includes a rapidly expanding list of newly recognised genetic developmental lung disorders, including surfactant abnormalities, pulmonary interstitial glycogenesis, alveolar capillary dysplasia, TBX4 mutations and others (table 4).
Paediatric PVDs are often associated with multiple comorbidities that may contribute to PH severity and dictate overall outcomes. As discussed, the genetic background of paediatric PH appears to differ from that of adult PH, and accompanying genetic disorders, syndromes and growth abnormalities are frequent in children with PH. Whether these latter should be regarded as causally related, disease modifiers or innocent bystanders is often not clear. Therefore, accurate phenotyping of children with PH and assessment of the effect on outcome of comorbidities remains of crucial importance in any classification.
Down syndrome
An illustrative example of the complex role of comorbidities in paediatric PH warranting further attention is Down syndrome. This Paediatric Task Force discussed the unique clinical phenotype of neonates, infants and children with Down syndrome, and the potential role for PH screening in this group.
Down syndrome, or trisomy 21, is associated with significant cardiovascular and pulmonary morbidity and mortality in children, including PH, chronic hypoxaemia and recurrent respiratory illnesses. Newborn infants with Down syndrome are at high risk of developing severe PPHN at birth and often have more aggressive PVD secondary to CHD or airways obstruction than do subjects without Down syndrome. Mechanisms that increase the susceptibility of infants and children with Down syndrome to develop worse PH and cardiorespiratory disease are incompletely understood. Past studies have shown that infants dying with Down syndrome can have evidence of lung hypoplasia as demonstrated by decreased alveolarisation, peripheral lung cysts and persistence of the double-capillary network [45]. These early abnormalities of arrested lung development may contribute to increased susceptibility for more aggressive cardiovascular and respiratory diseases in Down syndrome. Although mechanisms for abnormal lung and lung vascular development are uncertain, recent work has shown that three antiangiogenic (anti-vascular endothelial growth factor) genes are present on chromosome 21, and are each overexpressed in human fetal and infant lung tissue, including endostatin, RCAN-1 (regulator of calcineurin-1) and β-amyloid peptide. Experimentally, early disruption of angiogenic signalling decreases vascular growth and increases the risk for PH, and also impairs distal airspace (alveolar) growth [46, 47]. Overall, these laboratory and clinical findings suggest that subjects with Down syndrome are highly susceptible to decreased lung vascular and alveolar growth, which may increase the risk for PH and lung hypoplasia. Impaired lung vascular growth may increase the risk for environmental stimuli, such as haemodynamic stress, especially with associated CHD, intermittent or sustained hypoxia with obstructive apnoea or lung disease, viral infection, aspiration and other factors, to induce more accelerated PH in subjects with Down syndrome than others. Thus, the genetic consequences of Down syndrome may be linked with disruption of lung vascular and alveolar growth, suggesting that Down syndrome represents a “developmental lung disease”. The current WSPH Paediatric Task Force agreed that the phenotype of Down syndrome-related PH is variable and does not universally fit into a single classification group, but that children with Down syndrome will be classified as group 3 in the absence of CHD (group 1 or 2).
Diagnosis of paediatric PH
Since the aetiology of PH is very diverse, a methodical and comprehensive diagnostic approach is crucial to reach an accurate diagnosis and treatment plan. Moreover, IPAH is a diagnosis “per exclusion” and can be made only by excluding known causes of PH. Despite this, recent registries have shown that most children do not undergo a complete evaluation [48]. An updated comprehensive paediatric diagnostic algorithm is shown in figure 1. Special situations may predispose to the development of PAH and should be considered.
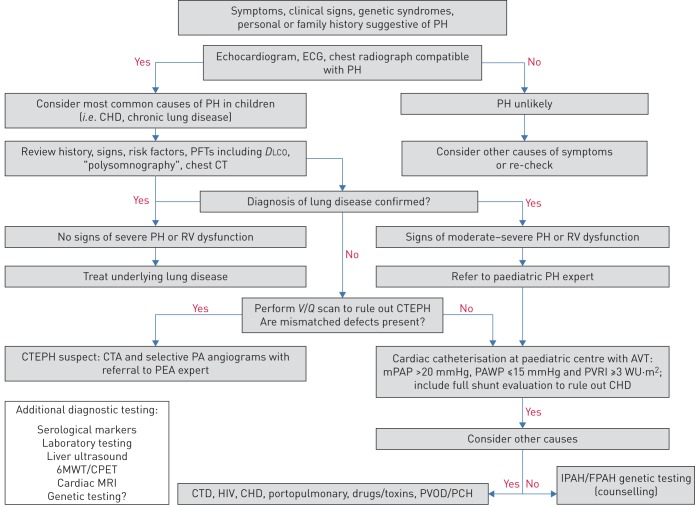
Diagnostic algorithm for pulmonary hypertension (PH) in children. CHD: congenital heart disease; PFT: pulmonary function test; DLCO: diffusing capacity of the lung for carbon monoxide; CT: computed tomography; RV: right ventricular; V/Q: ventilation/perfusion; CTEPH: chronic thromboembolic PH; CTA: CT angiography; PA: pulmonary artery; PEA: pulmonary endarterectomy; AVT: acute vasodilator testing; mPAP: mean pulmonary arterial pressure; PAWP: pulmonary arterial wedge pressure; PVRI: pulmonary vascular resistance index; WU: Wood Units; 6MWT: 6-min walk test; CPET: cardiopulmonary exercise test; MRI: magnetic resonance imaging; CTD: connective tissue disease; PVOD: pulmonary veno-occlusive disease; PCH: pulmonary capillary haemangiomatosis; IPAH/FPAH: idiopathic/familial pulmonary arterial hypertension.
Right heart catheterisation
The Paediatric Task Force addressed several questions regarding the risks and benefits of right heart catheterisation (RHC) in confirming the diagnosis of PH or PAH in children, and which children may be at highest risk for adverse events during RHC.
RHC remains the gold standard for the definitive diagnosis and nature of PAH, performing AVT, and providing useful data for risk stratification. This necessity should be balanced with associated risks. Major complications associated with RHC in children with PH have been reported to be 1–3%, and are generally associated with clinical condition and young age (newborns and young infants) [49–52]. As a result, cardiac catheterisation in paediatric PAH is strongly recommended to be performed in experienced paediatric PH centres using strategies to prevent these potential complications and having the ability to manage complications including PH crisis with aggressive interventions such as extracorporeal life support (ECLS). In rare instances, a child may be too sick to undergo cardiac catheterisation safely (e.g. World Health Organization Functional Class (WHO FC) IV). In these cases, when the suspicion of PAH is high and the non-invasive imaging is highly supportive, one should stabilise first and cautiously initiate appropriate PH therapy under careful observation, most often in the intensive care unit setting. RHC can then be performed more safely when the patient is sufficiently stabilised. Every attempt should be made for children with IPAH/HPAH to undergo RHC and AVT safely so one can determine whether they are acutely responsive to vasoreactivity testing and could benefit from CCB treatment. For those robustly responsive, but with poor cardiac function, a CCB would not be utilised unless the function improved.
Indications for repeat cardiac catheterisation in children with PH are not well defined, but include assessment of treatment effect, clinical deterioration, detection of early disease progression, listing for lung transplantation and prediction of prognosis. It has, however, not been shown whether changes in haemodynamic parameters are associated with change in clinical outcome and therefore these parameters do not meet the requirements to serve as established treatment goals.
Treatment strategies and clinical end-points
Currently, a goal-oriented treatment strategy is suggested for the treatment of paediatric PAH. Despite the absence of validated treatment goals in paediatric PAH, various treatment guidelines were proposed for children with PAH, predominantly based on expert opinion [53–56]. An apparent lack of consensus in these opinions resulted in important differences in reported paediatric treatment recommendations, stressing the need for evidence.
In 2013, the Paediatric Task Force of the 5th WSPH summarised determinants of higher risk in children, which included clinical evidence of right ventricular failure, progression of symptoms, syncope, failure to thrive, WHO FC III or IV, significantly elevated or rising brain natriuretic peptide (BNP) levels, echocardiographic signs of severe right ventricular enlargement or dysfunction, pericardial effusion, and haemodynamic parameters such as mPAP/mean systemic arterial pressure (mSAP) ratio >0.75, mean right atrial pressure (mRAP) >10 mmHg and PVRI >20 WU·m2 [54]. A recent systematic review and meta-analyses concluded that WHO FC, N-terminal pro-BNP (NT-proBNP)/BNP, mRAP, PVRI, cardiac index and acute vasodilator response are consistently reported as useful prognostic factors for assessing long-term outcomes in paediatric PAH, and thus could be used for initial risk stratification and as such incorporated in recommendations and guidelines [57, 58].
However, parameters with prognostic capabilities are not automatically suitable to serve as a treatment goal. Treatment goals are either clinically meaningful parameters that reflect how a patient feels or functions and can thus be a target for treatment, or should be surrogates for survival. Surrogates for survival by definition are parameters with a strong correlation with survival, which can be changed by treatment, while such change should indicate disease worsening or improvement and should be predictive of long-term outcome.
WHO FC, used in children with PAH and indicating how the child feels and function, has been demonstrated to be also a strong predictor of transplant-free survival. Moreover, WHO FC has been shown recently in paediatric PAH to be also a surrogate for survival and as such WHO FC qualifies as a treatment goal, despite its disadvantage of being a potentially subjective assessment [53]. A functional class designed specifically for children was proposed in 2011, but is still being considered because of its complexity and influence by comorbidities, and therefore has not yet reached broad use [59, 60].
In adults with PAH the 6-min walk test (6MWT) has been used to demonstrate drug efficacy. Although its ability to serve as a surrogate end-point for late outcomes is debatable, the 6MWT may be useful as a treatment goal in paediatric patients developmentally able to perform the test (children >6 years of age) [61, 62]. Unfortunately, younger children cannot reliably perform the test, making this a suboptimal primary end-point in a study of the full age range of children. Cardiopulmonary exercise testing (CPET) is even more demanding with respect to developmental skills and paediatric reference values for CPET in association with outcome are lacking [63].
Echocardiography seems an obvious tool to monitor treatment effect in children with PH. It provides functional and structural assessment of the heart and estimates of pulmonary haemodynamics, and is widely available, non-invasive and well tolerated by children. Unfortunately, echocardiography is also subject to significant operator and interpretation variability [64]. Where several echocardiographic variables have been suggested as predictors of outcome in paediatric PAH, today only tricuspid annular plane systolic excursion (TAPSE) has been shown as strongly associated with improved survival during treatment, indicating its potential utility as a treatment goal [53]. New echocardiographic modalities for evaluating right ventricular function (three-dimensional echocardiography, strain and strain rate, and right ventricular stroke work) as well as magnetic resonance imaging (MRI) assessment of right ventricular volume and function both hold promise here, but more data in the paediatric population are needed to determine the value of these techniques regarding establishment of predictive value or surrogacy for clinical outcomes [65, 66].
In addition to right ventricular function, right ventricular pulmonary vascular coupling reflects right ventricular afterload, and is regarded to be an important measure for the cardiovascular state and thus prognosis in patients with PH. MRI and echocardiography are potential candidates for non-invasive monitoring of this coupling state.
At the pulmonary arterial side of ventricular–arterial coupling, pulmonary arterial stiffness parameters are gaining interest as prognostic indicators in PAH. Recently, pulmonary vascular stiffness indices have been shown to predict the development of advanced PAH and mortality in paediatric PVD [67–71].
Serum biomarkers have the advantage of being relatively easy to obtain in peripheral blood and several biomarkers have been studied in paediatric PAH. Two serum biomarkers have been repeatedly shown to have prognostic capabilities in paediatric PAH: NT-proBNP and uric acid. A recent meta-analysis confirmed that NT-proBNP correlated strongly and consistently with survival in children with PAH, and thus can be used for risk stratification in this population. However, to be used as a treatment target or clinical end-point, biomarkers should be representative of the disease process and its evolution, which is often difficult to demonstrate. Nevertheless, changes in NT-proBNP levels following treatment initiation were recently shown to be predictive for survival in paediatric PAH, indicating that NT-proBNP qualifies as a treatment goal [72, 73]. Baseline uric acid levels had previously been shown to correlate with survival in paediatric PAH [74]. More recently, it was shown that the development of uric acid levels over time correlates with outcome in paediatric PAH [75]. These findings show that uric acid is capable of predicting outcome not only at baseline, but also during the disease course of PAH, and therefore may also qualify to be a treatment goal.
Recently, clinical worsening has been introduced as a composite end-point for large randomised controlled trials (RCTs) in adults with PAH. Components of clinical worsening included unambiguous events such as death or lung transplantation, which are combined with softer events, including hospitalisations, need for additional therapy and worsening of function. The use of clinical worsening as an end-point has been validated in adults with PAH and the soft clinical worsening end-point components were shown to be highly predictive for subsequent mortality. These results have now been reproduced in the paediatric PAH population using clinical worsening components: death, lung transplantation, non-elective PAH-related hospitalisations, including hospitalisations for atrial septostomies, initiation of i.v. prostanoids and functional deterioration (worsening of WHO FC, ≥15% decrease in 6MWD or both) [76]. Moreover, this study showed that clinical worsening occurred with a high event rate in paediatric PAH, indicating that clinical worsening may serve as a suitable end-point in future paediatric trials.
Monitoring daily physical activity has also been suggested as an alternative tool to assess functional capacity in children. A recent pilot study using three-axis accelerometry in 29 children with PAH and 60 controls showed that physical activity was markedly decreased in children with PAH, and that accelerometer output correlated with clinical disease severity markers and predicted outcome [77]. Larger studies are in progress to validate the use of accelerometry output as a clinically meaningful end-point for clinical trials in paediatric PAH.
In summary, the emerging paediatric data provide increasing evidence and support for the risk stratification model proposed by the WSPH Paediatric Task Force in 2013, with some minor modifications in 2018. For example, the prognostic significance of syncope could not be demonstrated and is therefore questioned as a high-risk factor for poor outcomes. Importantly, upcoming evidence suggests that in paediatric PAH striving for a low-risk profile using this WSPH paediatric risk assessment tool might also be used as treatment target, as has recently been suggested in adults [78–80].
Updates to the paediatric treatment algorithm
The prognosis of children with PAH has improved in the past decade owing to new therapeutic agents and aggressive treatment strategies. However, the use of targeted pulmonary PAH therapies in children is almost exclusively based on experience and data from adult studies, rather than evidence from clinical trials in paediatric patients. Due to the complex aetiology and relative lack of data in children with PAH, selection of appropriate therapies remains difficult. We propose a pragmatic treatment algorithm based on the strength of expert opinion that is most applicable to children with IPAH (figure 2). The ultimate goal of treatment should be improved survival and to facilitate normal activities of childhood without self-limitation.
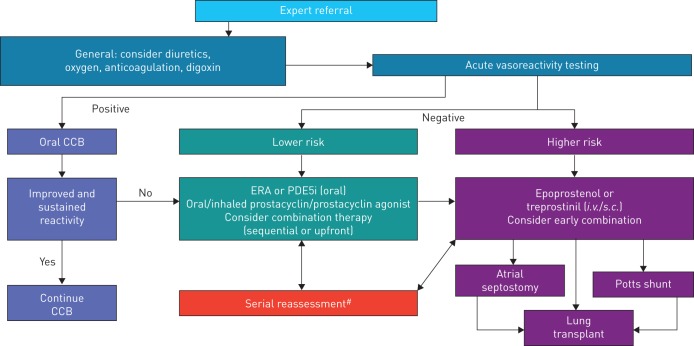
Paediatric idiopathic/familial pulmonary arterial hypertension treatment algorithm. CCB: calcium channel blocker; ERA: endothelin receptor agonist; PDE5i: phosphodiesterase type 5 inhibitor. #: deterioration or not meeting treatment goals.
Background therapy with diuretics, oxygen, anticoagulation and digoxin should be considered on an individual basis. Care should be taken to not overly decrease intravascular volume due to the pre-load dependence of the right ventricle. Following the complete evaluation for all causes of PH, AVT is recommended to help determine therapy.
In children with a positive AVT response, oral CCBs may be initiated [2–4]. In the child with a sustained and improved response, CCBs may be continued, but patients may deteriorate, requiring repeat evaluation and additional therapy [81]. Clinical experience suggests that these children remain on CCBs in addition to targeted PAH therapy. For children with a negative AVT response or in the child with a failed or non-sustained response to CCBs, risk stratification should determine additional therapy (table 6). Although the specific number of lower- or higher-risk criteria to drive therapeutic choices is not yet known, a greater proportion of either should be considered as justification for therapy. As in adult patients, determinants of higher risk in children include clinical evidence of right ventricular failure, progression of symptoms, WHO FC III or IV, significantly elevated or rising BNP/NT-proBNP levels, severe right ventricular enlargement or dysfunction and pericardial effusion. Additional haemodynamic parameters that predict higher risk include mPAP/mSAP ratio >0.75 [82], mRAP >10 mmHg and PVRI >20 WU·m2 [83]. Additional high-risk parameters include failure to thrive. In the child with a negative acute vasoreactivity response and lower risk, initiation of oral monotherapy is recommended. Treatment of choice is an endothelin receptor antagonist (bosentan [83–90], ambrisentan [91, 92]) or phosphodiesterase type 5 (PDE5) inhibitor (sildenafil [93–100], tadalafil [101, 102]).
TABLE 6
Determinants of paediatric idiopathic/heritable pulmonary arterial hypertension risk
Lower risk | Determinants of risk | Higher risk |
No | Clinical evidence of RV failure | Yes |
No | Progression of symptoms | Yes |
>350 | 6MWT (>6 years old) m | <350 |
Normal | Growth | Failure to thrive |
I, II | WHO FC | III, IV |
Minimally elevated | Serum BNP/NT-proBNP | Significantly elevated |
Rising level | ||
Echocardiography | RA/RV enlargement | |
Reduced LV size | ||
Increased RV/LV ratio | ||
Reduced TAPSE | ||
Low RV FAC | ||
Pericardial effusion | ||
Systemic CI >3.0 L·min−1·m−2 | Haemodynamics | Systemic CI <2.5 L·min−1·m−2 |
Systemic venous saturation >65% | mRAP >10 mmHg | |
Acute vasoreactivity | PVRI >20 WU·m2 | |
Systemic venous saturation <60% | ||
PACI <0.85 mL·mmHg−1·m−2 |
RV: right ventricle; 6MWT: 6-min walk test; WHO: World Health Organization; FC: Functional Class; BNP: brain natriuretic peptide; NT-proBNP: N-terminal pro-BNP; RA: right atrium; LV: left ventricle; FAC: fractional area change; TAPSE: tricuspid annular plane systolic excursion; CI: cardiac index; mRAP: mean right atrial pressure; PVRI: pulmonary vascular resistance index; WU: Wood Units; PACI: pulmonary arterial compliance index.
Children who deteriorate on either endothelin receptor antagonists or PDE5 inhibitors may benefit from consideration of early combination therapy (add-on or up-front). If the child remains in a low-risk category, addition of inhaled prostacyclin (iloprost [103–106], treprostinil [107]) to background therapy may be beneficial. It is crucial to emphasise the importance of continuous repeat evaluation for progression of disease in children on any of these therapies. In children who are at higher risk, initiation of i.v. epoprostenol [108, 109] or treprostinil [110] should be strongly considered. Experience using s.c. treprostinil in children is increasing as well [111–113]. In the child deteriorating with high-risk features, early consideration of lung transplantation and interventional palliative bridges are important.
Interventional palliative bridges
Atrial septostomy
Atrial septostomy in children with IPAH has been performed to treat syncope, and improve cardiac output and systemic oxygen carrying capacity, especially in countries without easy access to targeted PH drugs or in IPAH refractory to medical therapy, or as a bridge to lung transplantation [114]. Atrial septostomy improves symptoms and quality of life in paediatric PAH, and may serve as a bridge to lung transplantation [56]. It appears to be safe in centres with experience, and one study reported lung transplantation-free and repeat-balloon atrial septostomy (BAS)-free survival at 30 days, 1 year and 5 years was 87%, 61% and 32%, respectively [115].
However, in most cases BNP levels do not change after BAS and it seems likely that the creation of the Potts shunt (see following subsection) may ultimately be the preferred procedure as in contrast to BAS it unloads the pulmonary vascular bed, as well as the right ventricle with preserved oxygenation to the upper body including coronary and cerebral vessels. Relative contraindications for atrial septostomy include 1) mRAP >20 mmHg, 2) resting arterial oxygen saturation <90%, 3) severe right ventricular failure and 4) patients with impending death.
Atrial septostomy may be considered in the child with worsening PAH despite optimal medical therapy, but should be considered before the later stages with increased risk. Atrial septostomy can be considered in patients with WHO FC III and IV symptoms and recurrent syncope on combined medical therapy, as palliative bridge to transplant, increasing the chance for survival while waiting for a donor organ.
Reversed Potts shunt
The Paediatric Task Force also discussed whether the reversed Potts shunt should be offered to children with severe IPAH/HPAH who are refractory to medical therapy.
Surgical creation of a palliative reversed Potts shunt (left pulmonary artery to descending aorta) has been described as a new option for severely ill children with suprasystemic IPAH [56, 116]. This surgical procedure implies the creation of a connection between the left pulmonary artery and the descending aorta, which allows right-to-left shunting, similar to a patient with patent ductus arteriosus-related Eisenmenger syndrome. The use of a reversed Potts shunt in suprasystemic PH is considered advantageous compared with atrial septostomy as it provides high oxygen saturated blood to the coronary arteries and the central nervous system, and only causes desaturation of the lower body. Another benefit arises from its effect on haemodynamics by the relief of right ventricular pressure overload in systole and, in part, also in diastole, with a subsequent reduction in shifting of the interventricular septum towards the left ventricle with an improvement in systolic and diastolic left ventricular performance. A run-off through the Potts shunt, if too big, with decreased pulmonary perfusion and extreme desaturation of the lower body, with subsequent undersupply of the myocardium and the brain, should be avoided. The procedure may be considered in patients with suprasystemic PH refractory to any medical treatment, including combined therapy presenting with WHO FC IV symptoms.
The largest series published consisted of 24 children with drug-refractory PAH in which a permanent Potts shunt was created (19 surgical left pulmonary artery–descending aorta, six via stenting of a persistent ductus arteriosus) [117]. Six patients experienced severe post-operative complications and there were three early deaths related to low cardiac output. After a median follow-up of 2.1 years, the 21 survivors showed persistent improvement in functional capacities and none of the patients had syncope or overt right ventricular failure [76]. These favourable long-term results suggest that creation of a Potts shunt can be a valuable alternative, or bridge to bilateral lung transplantation, at least in selected cases.
Recently, several case series demonstrated the feasibility of the pure catheter-based interventional implementation of the connection between the left pulmonary artery and the descending aorta [118]. The most elegant method is obviously the implantation of a stent in a still patent persistent ductus arteriosus, which is not infrequently present in infants and young children. This procedure is an established method in CHD with duct-dependent circulation and can be established with considerable low peri-procedural risk in experienced centres. The interventional de novo creation of a left pulmonary artery–descending aorta connection with a covered stent from the left pulmonary artery or descending aorta side [119] has been shown to be feasible, but currently must be considered a high-risk procedure in patients with end-stage PAH who are too sick to undergo surgery and only in a programme with expertise in performing this procedure. Salna et al. [120] described a novel successful approach to the Potts shunt in a young adult with IPAH using a unidirectional valved shunt from the main pulmonary artery to the descending aorta, which has the advantage of preventing any back flow from the aorta when the PAP is subsystemic and during diastole. Whether this will prove to be a preferred approach remains to be seen. The current WSPH Paediatric Task Force decided to include the Potts shunt in the treatment algorithm, but cautions that it should only be done in selected patients in a centre with the expertise to perform the procedure, including ECLS back-up (figure 2). Whether this is preferred over an atrial septostomy will require further experience and study.
Clinical trial design
The Paediatric Task Force further discussed whether we can develop new paediatric-specific clinical end-points in clinical trial design.
Our understanding of the pathobiology and treatment of children with PH has improved considerably during the past 20 years, but treatment is still based on clinical trial evidence of efficacy from clinical trial data in adults, individual clinical experience, registry data, short-term trials and open-label studies. RCTs have not been conducted. Clinical trials in adults are most helpful when conducted on the most homogeneous, “purest” form of the disease, i.e. IPAH, but this is rare in children. Growth and development entail constant hormonal and metabolic change necessitating age-related study, understanding the effect on long-term outcome is crucial and choice of end-points is difficult [121]. The European Medicines Agency (EMA) and US FDA both require RCTs, but the EMA emphasises the need for pharmacokinetic and safety studies, which have been done in some instances, while the US FDA seeks to include a clinical end-point for evidence of efficacy. Relevant clinical end-points include death, transplantation and hospitalisation, and determining how the child feels. The traditional means of evaluation in adults, such as the 6MWT, are obviously not applicable to young children. Potential surrogate end-points in children include weight, echocardiography, biomarkers (primarily NT-proBNP), MRI and exercise testing. None of these possibilities have been fully validated. Haemodynamic evaluation does not seem to be an appropriate end-point since there are ethical considerations and sequential studies are not known to relate to long-term outcome. Recruitment, retention and evaluation of a continually maturing small population of children is a considerable challenge.
However, since the 5th WSPH, paediatric-specific biomarkers, such as functional classification, TAPSE and NT-proBNP, growth and composite clinical end-points have been evaluated. TAPSE, NT-proBNP and WHO FC identified transplant-free survival in 70 children with PAH [53]. Height for weight was also identified as a clinical end-point [122]. The Panama “Paediatric Functional Class” correlated well with outcome [60]. A composite end-point of clinical worsening composed of death, lung transplantation and initiation of parenteral prostanoid therapy was identified [53, 76]. A novel approach to clinical evaluation of treatment efficacy or disease progression may be the use of home accelerometers and a pilot study suggested that there is a difference in spontaneous physical activity in children with PAH compared with controls [77]. Thus, paediatric-specific clinical end-points and trial design are evolving.
Conclusions
Despite many unique characteristics, children with PH are often assessed and managed based on adult PH guidelines. This current WSPH Paediatric Task Force had an opportunity to further highlight some of the inherent differences between children and adults with PH, novel findings in paediatric PH since the 5th WSPH meeting in 2013, and develop additional current paediatric-specific recommendations. The current 2018 6th WSPH classification incorporates the growing population of children with developmental lung diseases, such as BPD and CDH, complex CHD, and novel mutations. The Paediatric Task Force addressed a new definition for PAH and AVT in children, a novel palliative bridge approach with the reversed Potts shunt, and clinical trial design. The field of paediatric PH still requires future paediatric-specific clinical trials in order to develop specific treatment strategies and clinical end-points for children with PH.
Footnotes
Number 12 in the series “Proceedings of the 6th World Symposium on Pulmonary Hypertension” Edited by N. Galiè, V.V. McLaughlin, L.J. Rubin and G. Simonneau
Conflict of interest: E.B. Rosenzweig reports institutional grants from Actelion, GSK, Gilead, Bayer and United Therapeutics/Lung Biotech, outside the submitted work.
Conflict of interest: S.H. Abman reports grants from Shire and United Therapeutics, outside the submitted work.
Conflict of interest: I. Adatia reports personal fees (for data and safety monitoring board work for selexipag trial in children, and for clinical oversight committee work for macitentan trial in children) from Actelion, personal fees (for data and safety monitoring board work for citruline trial in children) from Asklepion, and personal fees (for clinical oversight committee work for tadalafil trial in children) from Eli Lilly, outside the submitted work.
Conflict of interest: M. Beghetti reports grants and personal fees from Actelion, grants, personal fees and non-financial support from Bayer Healthcare, and personal fees from GSK, Eli Lilly, MSD and Pfizer, during the conduct of the study.
Conflict of interest: D. Bonnet reports personal fees from Actelion Pharmaceuticals Novartis, Bayer Healthcare and Eli Lilly, outside the submitted work.
Conflict of interest: S. Haworth has nothing to disclose.
Conflict of interest: D.D. Ivy: the University of Colorado School of medicine contracts with Actelion, Bayer, Lilly and United Therapeutics for D.D. Ivy to be a consultant and perform research studies.
Conflict of interest: R.M.F. Berger: University Medical Center Groningen contracts with Actelion and Lilly for consultancy and advisory board activities of R.M.F. Berger.
References
Full text links
Read article at publisher's site: https://doi.org/10.1183/13993003.01916-2018
Read article for free, from open access legal sources, via Unpaywall:
https://erj.ersjournals.com/content/erj/53/1/1801916.full.pdf
Citations & impact
Impact metrics
Article citations
Embracing the challenges of neonatal and paediatric pulmonary hypertension.
Eur Respir J, 64(4):2401345, 31 Oct 2024
Cited by: 2 articles | PMID: 39209483 | PMCID: PMC11525338
Review Free full text in Europe PMC
Extracorporeal membrane oxygenation (ECMO) support for children with pulmonary hypertension: A single-institutional experience of outcomes.
Pulm Circ, 14(4):e12442, 25 Oct 2024
Cited by: 0 articles | PMID: 39463825 | PMCID: PMC11502935
Survival, morbidity, and quality of life in pulmonary arterial hypertension patients: a systematic review of outcomes reported by population-based observational studies.
Respir Res, 25(1):373, 16 Oct 2024
Cited by: 0 articles | PMID: 39415261 | PMCID: PMC11481430
Review Free full text in Europe PMC
The Pulmonary Artery Pulsatility Index Provides No Additional Prognostic Information in Pediatric Pulmonary Arterial Hypertension.
Children (Basel), 11(10):1152, 24 Sep 2024
Cited by: 0 articles | PMID: 39457117 | PMCID: PMC11506445
Usefulness and importance of echocardiography in the diagnosis of pediatric pulmonary hypertension.
J Cardiovasc Imaging, 32(1):33, 08 Oct 2024
Cited by: 0 articles | PMID: 39375770 | PMCID: PMC11460101
Go to all (216) article citations
Similar Articles
To arrive at the top five similar articles we use a word-weighted algorithm to compare words from the Title and Abstract of each citation.
Embracing the challenges of neonatal and paediatric pulmonary hypertension.
Eur Respir J, 64(4):2401345, 31 Oct 2024
Cited by: 2 articles | PMID: 39209483 | PMCID: PMC11525338
Review Free full text in Europe PMC
Meaningful and feasible composite clinical worsening definitions in paediatric pulmonary arterial hypertension: An analysis of the TOPP registry.
Int J Cardiol, 289:110-115, 25 Apr 2019
Cited by: 3 articles | PMID: 31072635
The challenges in paediatric pulmonary arterial hypertension.
Eur Respir Rev, 23(134):498-504, 01 Dec 2014
Cited by: 18 articles | PMID: 25445948 | PMCID: PMC9487412
Review Free full text in Europe PMC
Advances in targeted therapy for pulmonary arterial hypertension in children.
Eur J Pediatr, 182(5):2067-2076, 17 Dec 2022
Cited by: 3 articles | PMID: 36527480
Review