Abstract
Free full text

Low-grade inflammation as a key mediator of the pathogenesis of osteoarthritis
Abstract
Osteoarthritis (OA) has long been viewed as a degenerative disease of cartilage, but accumulating evidence indicates that inflammation has a critical role in its pathogenesis. Furthermore, we now appreciate that OA pathogenesis involves not only breakdown of cartilage, but also remodelling of the underlying bone, formation of ectopic bone, hypertrophy of the joint capsule, and inflammation of the synovial lining. That is, OA is a disorder of the joint as a whole, with inflammation driving many pathologic changes. The inflammation in OA is distinct from that in rheumatoid arthritis and other autoimmune diseases: it is chronic, comparatively low-grade, and mediated primarily by the innate immune system. Current treatments for OA only control the symptoms, and none has been FDA-approved for the prevention or slowing of disease progression. However, increasing insight into the inflammatory underpinnings of OA holds promise for the development of new, disease-modifying therapies. Indeed, several anti-inflammatory therapies have shown promise in animal models of OA. Further work is needed to identify effective inhibitors of the low-grade inflammation in OA, and to determine whether therapies that target this inflammation can prevent or slow the development and progression of the disease.
Osteoarthritis (OA) is the most prevalent arthritic disease and a leading cause of disability, with radiographically established OA affecting approximately 37% of the US population over 60 years of age1. OA can affect the knees, hips, spine, and fingers, and is characterized by progressive breakdown of articular cartilage and remodelling of the underlying bone in the synovial joints. Common clinical features include pain, joint dysfunction, and deformity. Women, older individuals, obese individuals, and individuals with prior joint injuries all have an increased risk of developing OA, as do individuals with certain genetic and biomechanical predisposing factors2–4. Current therapies for OA focus on pain control, viscosupplementation (via intra-articular injections of hyaluronic acid), and joint replacement, which all simply target the symptoms of advanced disease. Novel therapeutics are needed to inhibit the processes that drive OA pathology.
The past decade has seen a gradual but fundamental shift in our understanding of the mechanisms underlying OA. We no longer view OA as a prototypical degenerative disease resulting from normal bodily wear and tear, but rather as a multifactorial disorder in which low-grade, chronic inflammation has a central role (BOX 1). This inflammation comes into play early in the course of OA, as a result of interactions between the immune system and factors including local tissue damage and metabolic dysfunction5. Further unravelling of the mechanisms underpinning the inflammatory pathophysiology of OA is likely to yield new therapeutic approaches that can modify the course of OA.
In this Review, we examine the emerging evidence for a critical role of low-grade inflammation in the pathogenesis of OA, and discuss the current understanding of the underlying molecular mechanisms. We also explore the potential of therapeutic strategies that target this low-grade inflammation in the prevention or treatment of OA.
OA — a whole-organ disease of the joint
Traditionally, OA has been viewed as a disorder affecting articular cartilage2. However, we now know that this disease affects the entire joint structure6–10. The pathologic changes that occur in OA joints are fibrillation and degradation of the articular cartilage, thickening of the subchondral bone, formation of osteophytes, inflammation of the synovium (synovitis), degeneration of ligaments and menisci, and hypertrophy of the joint capsule6 (FIG. 1,,2).2). Radiographic evaluation of OA bone reveals the presence of subchondral sclerosis and cysts, and microscopic examination reveals microfractures and microcracks in advanced OA11.
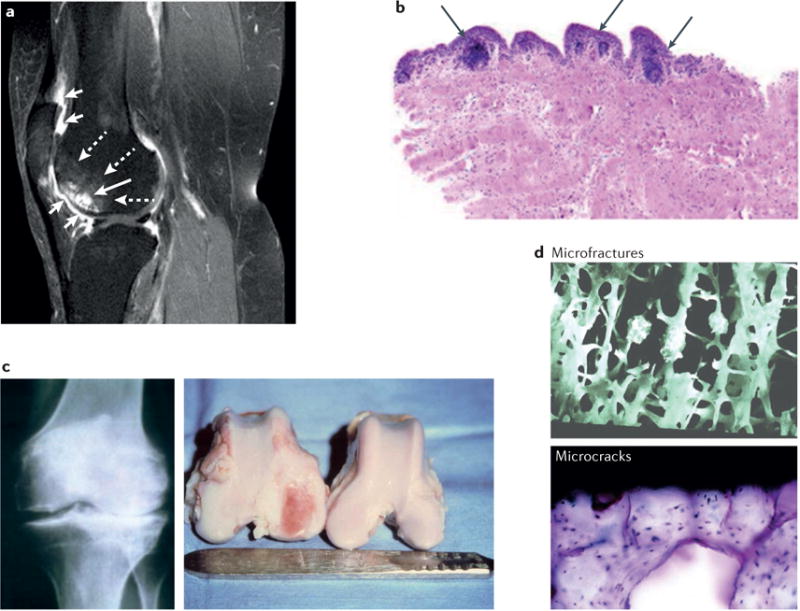
a | Gadolinium-enhanced MRI (sagittal view) scan of a knee with multiple features typical of OA: synovial inflammation, cartilage degradation, and bone remodelling. Short white arrows indicate marked peripatellar synovitis, dashed white arrows indicate bone marrow lesions, and the long white arrow pointing to bright white structures indicates bone cysts. b | A synovial biopsy specimen obtained during meniscectomy from a patient with knee OA, showing histological evidence of inflammation. Arrows indicate the presence of perivascular mononuclear cell accumulation. Original magnification × 5, haematoxylin and eosin stain. c | Remodelling of the subchondral bone in OA, as detected by radiography of the knee of an individual with OA (left), and by gross examination of distal femurs of a dog (right) that had undergone unilateral anterior cruciate ligament transection. In the destabilized dog knee, full-thickness ulceration of the articular cartilage has developed on the medial femoral condyle, and striking remodelling of the subchondral bone has occurred, with enlargement of the medial femoral condyle. The articular cartilage and bone on the contralateral dog knee appear grossly normal. d | Microfractures and microcracks in subchondral bone of an individual with OA. Part a adapted from Felson, D. T. Developments in the clinical understanding of osteoarthritis. Arthritis Res. Ther. 11, 203 (2009)168. The original article is an open access article distributed under the terms of the Creative Commons Attribution License (http://creativecommons.org/licenses/by/2.0), which permits unrestricted use, distribution, and reproduction in any medium, provided the original work is properly cited. Part b reproduced from Scanzello, C. R. et al. Synovial inflammation in patients undergoing arthroscopic meniscectomy: molecular characterization and relationship to symptoms. Arthritis Rheum. 63, 391–400 (2011)26. Parts c, d reproduced from Brandt, K. D., Dieppe, P. & Radin, E. L. Commentary: is it useful to subset “primary” osteoarthritis? A critique based on evidence regarding the etiopathogenesis of osteoarthritis. Semin. Arthritis Rheum. 39, 81–95 (2009)11.
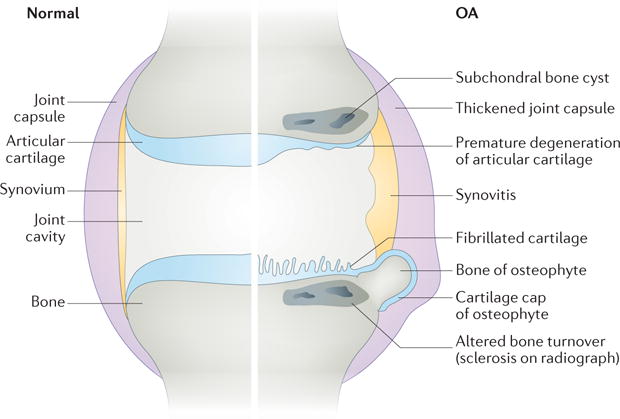
Comparison of the normal joint (left side) and the OA joint (right side), demonstrating that OA is a disease that affects the entire joint structure, including the articular cartilage, synovium, subchondral bone, joint capsule, and other components of the joint.
Several cellular and molecular processes are involved in these pathological changes: an increase in cartilage catabolism and a concomitant decrease in cartilage anabolism and repair; hypertrophy and death of chondrocytes; impairment or dysregulation of autophagy; osteoclast-mediated remodelling of bone; and infiltration and activation of immune cells6. However, many questions remain about how these processes combine to mediate the pathogenesis of OA. For instance, how does the mechanical and molecular interplay between different joint tissues drive OA pathology?6 What is the precise nature of the crosstalk between the affected tissues — that is, which factors produced by one joint tissue cause pathological changes in another? In what sequence do the pathological events occur? What feedback mechanisms are involved? Answering these questions will be important for designing next-generation therapeutic interventions that can prevent, slow, or reverse the development of OA.
The importance of inflammation in OA
Clinically, many individuals with OA have symptoms of joint inflammation, such as morning stiffness, warmth, pain, and joint effusions that arise, in part, from synovial thickening or synovial fluid effusion7. Indeed, synovitis, which is detectable by imaging, arthroscopy, or histology, is now recognized as a common finding in OA7,12–15. Histologically, this synovitis is characterized by infiltration of inflammatory cells into the synovium16,17. Furthermore, molecular evidence of low-grade inflammation in OA is accumulating. As early as 1959 came the discovery that inflammatory plasma proteins are present at abnormally high levels in both the blood and the synovial fluid of patients with OA18. More recent studies have shown that OA tissue and synovial fluid have abnormally high levels not only of plasma proteins19, but also of complement components20 and cytokines19, and that chondrocytes and synovial cells in OA produce or overproduce many of the inflammatory mediators (for example, IL-1β, TNF, and nitric oxide (NO))21 that are characteristic of inflammatory arthritides.
Accumulating evidence supports the association between OA pathology and different markers of inflammation. Imaging studies involving serial MRI scans coupled with histopathologic analysis of synovial tissues have shown that the presence of synovitis in OA is associated with an increased severity of joint symptoms, increased cartilage loss, decreased mobility, and elevated radiographic grades12,22–28. Likewise, the presence of synovitis at the time of arthroscopy is associated with subsequent accelerated destruction of cartilage29.
Whether these associations reflect a causal role for inflammation in OA pathogenesis, or indicate that joint inflammation is merely an epiphenomenon in a process that is primarily degradative or mechanical, remains unclear. The timing of the inflammation, as well as mechanistic plausibility and findings from animal models genetically deficient in specific inflammatory molecules, suggests that inflammation could indeed be pivotal in the pathogenesis of OA. The presence of synovitis often predates the development of radiographic damage in OA30, and mononuclear cell infiltration and overexpression of inflammatory mediators in the synovium are prominent in early OA31. Likewise, elevated serum levels of C-reactive protein, a marker of inflammation, are predictive of the development and progression of OA21. The inflammatory mediators detected in OA joints are synthesized, in part, in response to tissue injury and breakdown in the joint. They can alter chondrocyte differentiation and function, as well as induce the expression and activation of matrix metalloproteinases (MMPs) and aggrecanases, enzymes that degrade cartilage and are thought to be the downstream effectors of OA pathogenesis10,16,32,33. Finally, evidence from animal models suggests that inflammation contributes to joint degeneration in OA34, and that synovial macrophages are critical for the formation of osteophytes35. Thus, current evidence suggests that targeting inflammation therapeutically has the potential to prevent or reduce multiple pathologic features of OA, and thereby slow the progression of the disease.
In addition to local inflammation in the joint, systemic inflammation might also have an important role in OA pathogenesis. For instance, obesity is known to predispose individuals to OA36 — possibly not only by increasing the mechanical load on joints, but also by causing chronic, systemic inflammation through inflammatory mediators (such as adipokines and other proinflammatory cytokines) that are produced by adipose tissue and released into the bloodstream37,38. Weight loss is associated with a substantial reduction in systemic levels of C-reactive protein and IL-6 in individuals with OA, and can prevent OA onset or alleviate existing OA symptoms39,40. It is possible that the systemic inflammation associated with chronic inflammatory states, such as obesity or certain chronic diseases, promotes local inflammation in joints that ultimately results in OA.
The inflammation in OA is low-grade
The inflammation observed in OA is generally chronic and low-grade, and is believed to involve an immune response, primarily innate and to a lesser degree adaptive41. However, this inflammation is fundamentally different from that in rheumatoid arthritis (RA), the prototypical inflammatory arthritis. For example, the increase in levels of inflammatory plasma proteins in the blood and synovial fluid from individuals with OA (relative to healthy controls) is modest compared to that in patients with RA18,19. Histological comparisons reveal that inflammation is also less pronounced in typical OA synovium than in the RA synovium14,42–44. These two types of inflammation differ not only in degree, but also in the cellular and molecular players involved45. Whereas the numbers of most immune cells (for example, macrophages and T cells) in synovial tissues are lower in OA than in RA, the number of mast cells in OA is as high as, or sometimes higher than, in RA45. The expression of cytokines related to macrophage or T-cell function in OA synovial tissues is also lower than in RA synovial tissues (although higher than in normal tissue)45. Finally, systemically blocking the activity of conventional inflammatory cytokines with biologic therapies approved for the treatment of RA (such as anti-TNF or anti-IL-1β therapies) provides no benefit in generalized OA, and no or only minimal benefit in erosive OA (see below for more discussion on why these trials might have failed)32,46–49. Collectively, these lines of evidence suggest that the inflammatory mechanisms operating in OA differ from those in RA, and that targeting them will require novel approaches to anti-inflammatory therapy.
Molecular inflammatory mechanisms in OA
In the OA joint, acute, subacute, or chronic injuries and joint tissue breakdown, often in the context of other risk factors (such as obesity, advanced age, metabolic disorders, dysregulation of bioenergy sensors, and certain genetic factors), can trigger a progressive cycle of local tissue damage, failed tissue repair, and inflammation, resulting in further cartilage loss and progressive joint degeneration over time10,30,42. In this paradigm (BOX 2, FIG. 3), a number of molecular components and mechanisms could transduce joint trauma, chronic injury, or overuse into inflammatory processes. These factors are discussed in the following sections.
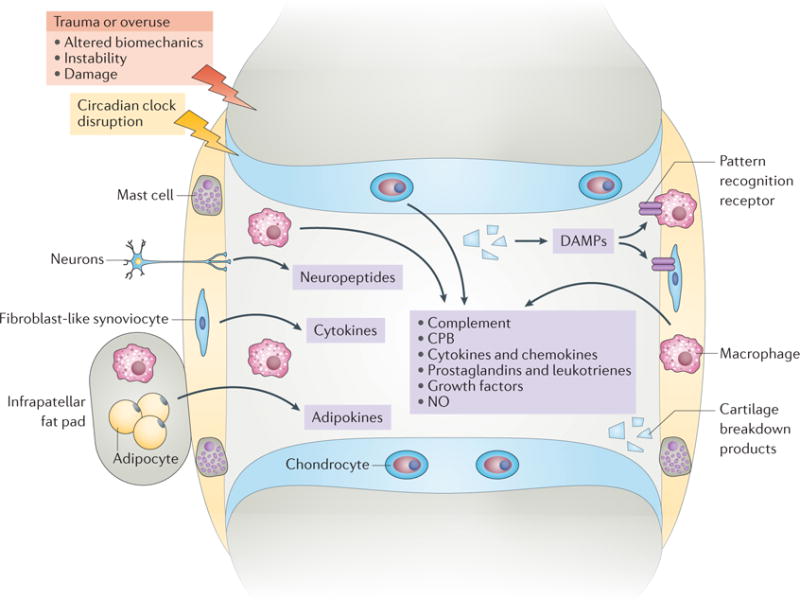
Several inflammatory pathways and mechanisms are likely to contribute to the pathogenesis of OA. In this paradigm, injury or overuse, often in the context of other risk factors, triggers a vicious cycle of local tissue damage, failed tissue repair, and low-grade inflammation involving a number of molecular components and mechanisms in the joint. This low-grade inflammation contributes to or mediates progressive cartilage loss, pain, and joint dysfunction. CPB, carboxypeptidase B; DAMPs, disease-associated molecular patterns; NO, nitric oxide.
Innate immune mechanisms
The innate immune system recognizes conserved features of pathogens via invariable pattern-recognition receptors (PRRs), which comprise multiple families of cell-surface, endosomal, and cytosolic receptors. PRRs provide a first-line immune response to microbial invaders. However, they recognize not only pathogen-associated molecular patterns, but also damage-associated molecular patterns (DAMPs). Produced during tissue damage, DAMPs are endogenous molecules that signal to innate immune cells (for example, macrophages and mast cells) to trigger a protective response. Once activated by DAMP-PRR signalling, the innate immune system produces an array of inflammatory mediators that normally initiate immune responses, and that ultimately lead to repair. However, prolonged or dysregulated activation of PRR-DAMP-induced inflammation can be destructive, and has been implicated in the chronic inflammation observed in OA30,50.
Like PRRs, the complement system is an innate immune mechanism by which the body recognizes pathogens51,52, and has also been implicated in inflammation and damage in OA joints53. The complement system enhances the ability of antibodies and phagocytic cells to clear pathogens from an organism54,55. Complement activation leads to chemotaxis, exudation of plasma proteins at inflammatory sites, and opsonization of infectious agents and damaged cells. Interestingly, several products of tissue breakdown in the joint are capable of activating both PRRs and complement56–61.
DAMPs
At least four classes of DAMPS are associated with OA (reviewed elsewhere30): the products of extracellular matrix (ECM) breakdown, which occurs at sites of inflammation (such as biglycan, fibronectin, low-molecular-weight hyaluronic acid, and tenascin C); plasma proteins that exude from blood vessels at sites of inflammation-induced or damage-induced vascular leakage (for example, a1 microglobulin, α2 microglobulin, fibrinogen, and vitamin D-binding protein); intracellular alarmins released from stressed, damaged, or necrotic cells (for example, HMGB1 and the S100 family of proteins); and microscopic crystals released from cartilage into the synovial space by injury or wear and tear (for example, basic calcium phosphate, calcium pyrophosphate dihydrate, and uric acid).
When inducing inflammation, one of the main classes of PRRs bound by DAMPs are the Toll-like receptors (TLRs). Stimulation of TLRs ultimately leads to activation of inflammatory transcriptional programs via transcription factors such as interferon-regulatory factors, nuclear factor-κB (NF-κB), and activator protein 1 (AP-1)42. Among the ten functional TLRs in humans, TLR1-TLR7 and TLR9 have been detected in the synovium of individuals with OA or RA (reviewed elsewhere15). TLR activation has been implicated in the development of synovitis, degeneration of cartilage, and susceptibility to disease in OA15, although MyD88-dependent TLRs were shown to be dispensable in a mouse model of OA62. Indeed, in vivo studies of deficiency in DAMPs or TLRs and their agonists have generated mixed results63, and the effects of targeting these molecules in human OA remains to be determined (TABLE 1).
Table 1
Examples of genetic and pharmacologic manipulations targeting inflammation in OA
Target | Effects on OA in animal models | Effects on OA in human trials |
---|---|---|
Tenascin C | Tnc−/− mice showed resolution of acute joint inflammation and were protected from sustained and erosive joint inflammation; intra-articular injection of tenascin C promoted inflammation169 | Not tested |
S100A8, S100A9 | S100A9−/− mice (also lacking S100A8 at protein level) showed reduced synovial activation, osteophyte formation, and OA cartilage destruction170,171 | Not tested |
C5 | C5− mice showed less cartilage loss, osteophyte formation, and synovitis; treatment with an anti-C5 monoclonal antibody or CR2-fH (a fusion protein that inhibits C3 and C5) attenuated OA53 | Not tested |
C6 | C6−mice were protected from OA development and synovitis53 | Not tested |
CD59a | Cd59a−/− mice developed more severe OA and synovitis53 | Not tested |
IL-1β | Anti-IL-1β treatment reduced infiltration of inflammatory cells and cartilage damage in collagen-induced arthritis172; pralnacasan (inhibitor of IL-1β converting enzyme) reduced OA173 | IL-1β blockade associated with liver toxicity or lack of DMOAD efficacy46,49 |
TNF | Intra-articular injection of infliximab (an anti-TNF monoclonal antibody) attenuated OA pathological changes174; ESBA105 (an anti-TNF scFv antibody) inhibited inflammation and prevented TNF-induced cartilage damage175 | Lack of DMOAD efficacy in generalized OA; no or minimal benefit in erosive OA47,48 |
NGF | An anti-NGF monoclonal antibody improved pain in a fracture model176 | Treatment with tanezumab (an anti-NGF monoclonal antibody) improved symptoms of OA107 |
FGF18 | Intra-articular injection of FGF18 stimulated chondrogenesis and cartilage repair177 | Trial of intra-articular injection of FGF18 ongoing178 |
COX2 | Celecoxib (a COX2 inhibitor) reduced OA-like histological changes and suppressed chondrocyte apoptosis179 | Celecoxib and rofecoxib (COX2 inhibitors) were associated with cardiovascular toxicity and other adverse effects180–183 |
Etoricoxib (a COX2 inhibitor) inhibited acute inflammation and adjuvant-induced arthritis184 | Etoricoxib improved symptoms of OA185,186 | |
COX1, COX2 | Naproxen (a non-selective COX inhibitor) suppressed MMP activities and the decrease in proteoglycan content187 | Naproxen improved pain associated with knee OA more than hip OA188, but was associated with upper gastrointestinal complications189 |
5LO | PF-4191834 (a 5LO inhibitor) reduced OA inflammation and pain190 | Phase II study of PF-4191834 was terminated owing to serious adverse events138 |
COX1, COX2, 5LO | Licofelone (a COX and 5LO inhibitor) attenuated the progression of OA191 | Licofelone improved OA symptoms and reduced cartilage volume loss192 |
Flavocoxid (a COX and 5LO inhibitor) improved inflammatory conditions193 | Flavocoxid improved OA symptoms194, but was associated with acute liver injuries195 | |
Bradykinin | Icatibant (a bradykinin B2 receptor antagonist) reduced inflammation149 | Icatibant showed an analgesic effect on knee OA149, but no detectable anti-inflammatory effects150 |
CPB | Cpb2−/− mice showed more severe cartilage damage, osteophyte formation, and synovitis71 | Not tested |
NO, iNOS | L-NMMA (a broad NOS inhibitor) reduced synovial inflammation and tissue damage in animal models of arthritis196–198; S-methylisothiourea attenuated MIA-induced pain and histopathological changes in the knee joint199 | Cindunistat (an iNOS inhibitor) failed to slow progression of OA147 |
MMPs | MMP inhibitors prevented the destruction of articular cartilage and bone200–204 | PG-116800 (an MMP inhibitor) failed to show benefit and was associated with the development of musculoskeletal toxicity94 |
IL-1β, MMPs | Strontium ranelate reduced the progression of OA structural changes165 | Strontium ranelate showed disease-modifying effects163 |
5LO, arachidonate 5-lipoxygenase; COX, cyclooxygenase; CPB, carboxypeptidase B; DMOAD, disease-modifying OA drug; FGF18, fibroblast growth factor 18; LTB4, leukotriene B4; iNOS, inducible NO synthase; L-NMMA, NG-monomethyl-L-arginine; MIA, monosodium iodoacetate; MMPs, matrix metalloproteinase; NGF, nerve growth factor; NO, nitric oxide; NOS, NO synthase; OA, osteoarthritis; scFv, single-chain variable fragment.
Complement system
Complement can be activated by any of three independent initiation pathways (the classical, alternative, and lectin pathways), which all subsequently converge on complement components C3 and C5, resulting in the formation of C3 and C5 convertases, respectively. Activation of these convertases induces the generation of the effector components of the complement cascade: the C3a and C5a anaphylatoxins promote inflammation by chemoattracting proinflammatory leukocytes, and the membrane attack complex (MAC) promotes tissue damage and inflammation. The MAC binds to the outer surface of cell membranes, and can form a transmembrane pore that results in cell lysis64,65. When bound to a cell surface at sublytic levels, however, the MAC instead induces proinflammatory cell signalling, such as that mediated by mitogen-activated protein kinases, the Janus kinase-signal transducer and activator of transcription (JAK-STAT) pathway, and NF-κB66.
Evidence from both human and mouse studies suggests the complement system has a central role in the pathogenesis of OA53. Decades ago, several reports described complement and immunoglobulin deposits in OA cartilage67 and synovium68, and complement components have since been shown to be produced, in part, by chondrocytes69. In 2011, our group reported that OA synovium expresses higher levels of complement effectors and lower levels of complement inhibitors than healthy synovium53. DAMPs present in OA joints, such as cartilage ECM proteins, calcium crystals, and components of apoptotic debris, also bind and activate complement56–61. Findings from mouse studies suggest that this overproduction and hyperactivation of complement in the joint has an important role in OA pathology: deficiency in the complement components C5 or C6 attenuated experimental OA, whereas deficiency in CD59a (a protein that inhibits MAC formation, and hence also MAC activity70) worsened it53. Still unknown, however, is whether complement deposition in OA joints is itself a triggering event or whether it is secondary to low-grade inflammation or to the damage of cartilage or joint tissues. These findings suggest that strategies that block complement activation have therapeutic potential for OA, but whether chronic inhibition of complement would be required and what adverse effects this inhibition might have are unclear.
Carboxypeptidase B
Evidence from both human and mouse studies suggests that the enzyme carboxypeptidase B (CPB) protects against the inflammatory destruction of OA joints by inhibiting proinflammatory anaphylatoxins and effectors of the complement system71. Produced primarily by the liver as a circulating plasma zymogen, CPB is activated by the thrombin-thrombomodulin complex during thrombotic events. In the joint, thrombomodulin expressed by synovial lining cells72,73 and neutrophils74 forms a 1:1 complex with thrombin, and activates procarboxypeptidase B (also called thrombin-activatable fibrinolysis inhibitor), resulting in the generation of CPB. By cleaving its substrates’ C-terminal arginine or lysine, CPB can in turn inactivate several inflammatory proteins, such as fibrin, C5a, C3a, bradykinin, and thrombin-cleaved osteopontin75,76, and thereby protect the joints from inflammatory destruction71. Whether treatments that target CPB have protective effects in OA clinical trials remains to be determined.
Macrophages and mast cells
Innate immune cells that can be activated by DAMPs and complement include macrophages and mast cells77,78, both of which are implicated in OA pathogenesis. Macrophages in the synovium of OA joints are activated and contribute to cartilage breakdown and osteophytosis by producing cytokines, such as IL-1β and lymphotoxin-a, as well as pro-MMPs17,35,79,80. Activated mast cells are also present in OA synovial tissues, and higher numbers of mast cells in OA synovial tissues are associated with a greater degree of both synovitis and structural damage in the synovial joints45. The mechanisms by which macrophages are activated in the OA joint and contribute to the pathogenesis of OA are currently under investigation.
Mast cells are a heterogeneous group of cells that can be subdivided into those containing only tryptase and those containing both tryptase and chymase81. The increase in number of mast cells in OA synovial tissue (compared with their numbers in normal tissue) is attributable to the selective expansion of the tryptase-only population of mast cells, in contrast to the expansion of both mast cell populations that occurs in RA82,83. Furthermore, tryptase activity is higher in mast cells in OA synovial tissues than in normal synovial tissues84; mast cell tryptase released into the synovial fluid stimulates the proliferation of fibroblast-like cells and the production of proinflammatory cytokines by these cells84, and could in this way contribute to OA pathology. Another classic mast cell mediator is histamine. Compared with those in RA, mast cells in OA synovial tissues have similar or higher histamine content85, but lower histamine release86,87. The exact roles of mast cells and their mediators in OA remain poorly understood.
Adaptive immune mechanisms
Levels of antibodies and immune complexes are abnormally high in OA synovial fluid and joint tissues, although the specificities of the antibodies involved and the relevance of these increases in antibody levels are unclear20-67-88. It is possible that the cartilage and cellular debris released during cartilage degeneration exposes neoepitopes that are then targeted by antibodies. Natural antibodies, which are typically not affinity-matured and therefore are of low affinity, can bind cartilage or other cellular debris to form immune complexes. These immune complexes might activate innate immune responses and stimulate inflammatory responses; alternatively, they might facilitate the clearance of cartilage debris and thus promote tissue repair, as has been observed in other diseases89. Further investigation is needed to define the role of antibodies and of the adaptive immune response in the pathogenesis of OA.
Inflammatory mediators
As mentioned earlier, various soluble inflammatory mediators have been identified in OA joint tissues and fluids, including cytokines, chemokines, growth factors, adipokines, prostaglandins and leukotrienes, and regulators such as CPB (reviewed elsewhere30). These mediators can be produced by different cell types within the joint, including fibroblast-like synoviocytes, chondrocytes, and resident or infiltrating immune cells15,90.
Cytokines
Cytokines are among the most extensively studied mediators of inflammation. Several cytokines, such as TNF, IL-1β, IL-6, IL-15, IL-17, IL-18, IL-21, and leukaemia inhibitory factor, have been implicated in the pathogenesis of OA30,90. The precise contributions of different cytokines to OA remains to be elucidated, but inflammatory cytokines have been proposed to induce cartilage catabolism as well as inhibit anabolic processes that are critical to cartilage homeostasis30,91,92. For example, IL-1β and TNF signalling mediated by the transcription factors NF-κB and AP-1 results in autocrine production of these cytokines, as well as expression of other inflammatory and chrondrolytic mediators (including NO, prostaglandin E2 (PGE2), IL-6, MMP1, MMP9, and MMP13), in OA cartilage ex vivo93. Despite encouraging results from animal studies, anti-IL-1β and anti-TNF therapies have not yielded positive results in OA clinical trials46–49. The disappointing results from human trials suggest that targeting a single cytokine (such as IL-1β or TNF) at the systemic level might not be sufficient to have a beneficial effect on OA; unlike RA, in which TNF drives IL-1β production, IL-1β and TNF could act in a parallel, redundant manner in OA32. Failure of these anti-cytokine therapies might also be explained by the inability of systemically administered anti-cytokine therapeutics to penetrate the joints, or by the evaluation of their efficacy in late-stage OA when the disease process might be less-responsive to such therapies, or by other factors32. Likewise, trials of MMP-blocking therapies in humans have shown a discouraging lack of efficacy and/or unacceptable toxicity94–96. Other cytokines could also be targeted therapeutically, but selecting which cytokine to target in OA will require a thorough understanding of the underlying mechanisms90.
Chemokines
Many chemokines (a subset of cytokines that induce the recruitment and trafficking of inflammatory cells and mesenchymal progenitors) are produced within the OA joint15,97,98. Certain chemokines and their receptors (IL-8, CCL5, CCL19, CCR1, CCR2, CCR3, and CCR5, among others) might facilitate the onset and progression of OA15, not only by inducing the release of MMP3 by chondrocytes and hence the breakdown of cartilage matrix components99, but also by promoting osteoclast-mediated remodelling of periarticular bone. Other chemokines might be protective in OA: for instance, stromal cell-derived factor-1 (also called CXCL12) recruits mesenchymal progenitors and thereby promotes tissue repair100. Yet other chemokines could have a dual role in inflammation, depending on the context in which they are produced101.
Growth factors
Growth factors such as transforming growth factor-β (TGFβ), fibroblast growth factors (FGFs), vascular endothelial growth factor (VEGF), and nerve growth factor (NGF) have been implicated in OA. The TGFβ family of growth factors is broadly expressed in cartilage, bone, and synovial tissues102. TGFβ growth factors are normally crucial for maintaining cartilage homeostasis but have been linked to the promotion of osteophytosis and synovial fibrosis in OA35,42,103. Three members of the FGF family expressed in articular cartilage, namely FGF2, FGF8, and FGF18, are involved in cartilage homeostasis and might be associated with OA104. VEGF, generated by the inflamed synovium, can promote angiogenesis and thereby facilitate infiltration of the joint by immune cells that contribute to inflammation in OA105. NGF regulates monocyte inflammatory responses and the production of IL-1β, TNF, IL-6, and IL-8 via the high-affinity NGF receptor (also known as tyrosine kinase receptor-A (TrkA))106. Inhibiting NGF with monoclonal antibodies, including tanezumab and fulranumab, significantly reduced pain in individuals with OA107.
Therapeutic approaches that target growth factors hold promise for preserving cartilage or stimulating its repair108. Several therapies that inhibit VEGF have proven beneficial in animal models of arthritis, and their anti-inflammatory and anti-angiogenic effects in OA are now being evaluated in clinical trials109. Intraarticular administration of recombinant FGF18 is also being investigated in individuals with OA (TABLE 1). However, local growth factor therapy risks promoting osteochondral bone formation, especially osteophyte outgrowth, whereas systemic diffusion of the growth factor might induce growth in normal tissues or, even worse, in premalignant lesions32.
Adipokines
High systemic and synovial fluid levels of adipokines, a family of cytokines secreted primarily by adipose tissue, are associated with cartilage degeneration and synovial inflammation in OA110–112. Thus, an increase in adipokine levels might explain why obesity (in which altered biomechanics could also have a role) and other features of the metabolic syndrome (hypertension, dyslipidaemia, insulin resistance, and others) increase the risk of developing OA36,111,113,114. Several adipokines, such as leptin, adiponectin, resistin, visfatin, and nefastin-1, have context-dependent immunomodulatory properties115,116 and can induce the production of inflammatory mediators and cartilage-degrading factors, leading to chondrocyte degradation and the development of OA112,115,117–124. Visfatin, for instance, the expression or enzyme activity level of which is regulated by IL-1β, hypoxia-inducible factor 2α (HIF-2α), and other interleukins, not only inhibits the phosphorylation of insulin receptor factors such as IRS1 and AKT, thus reducing proteoglycan production, but also increases the expression of MMPs, NGF, and PGE2, thereby aggravating OA120. Adipokines are also produced in the OA joint by infrapatellar fat pads, synovium, chondrocytes, osteoblasts, and osteoclasts125,126, which could additionally serve as local sources of other inflammatory mediators in the joint, such as neuropeptides and the classic inflammatory cytokines IL-1β, IL-6, and TNF30. Prevention of metabolic syndrome by lifestyle changes might relieve OA symptoms through reductions in adipokine levels and, in the case of weight loss, reduction in joint biomechanical stress.
Prostaglandins and leukotrienes
Lipid mediators, including prostaglandins and leukotrienes, have been detected in the OA joint and might be involved in the pathogenesis of OA19,127,128. In particular, PGE2 is likely to contribute to OA pathology by promoting inflammation, apoptosis, and angiogenesis127, whereas leukotriene B4 (LTB4) might act as a powerful leukocyte chemoattractant129 and could stimulate the production of IL-1β and TNF by synovial tissues130.
Substantial efforts have been made to elucidate the mechanisms governing prostaglandin and leukotriene production in OA, with a view to their therapeutic targeting. Prostaglandins and leukotrienes are generated from arachidonic acid via distinct enzymatic cascades that can be induced by inflammation or trauma127,128,131, such as that occurring in OA. Prostaglandins are produced by the cyclooxygenase pathway, in which cyclooxygenase-2 (COX2, also known as prostaglandin G/H synthase-2) is the rate-limiting enzyme mediating inducible prostaglandin production. COX2 is overexpressed in inflamed articular tissues127, and its expression in human chondrocytes can be induced by proinflammatory cytokines (IL-1β, TNF, IL-6, and others), as well as by TLR4 stimulation132. Likewise, prostaglandin E synthase, the key terminal enzyme in the production of PGE2, is overexpressed in OA cartilage133, and its expression in human OA chondrocytes can be induced by IL-1β and TNF134,135. Moreover, prostaglandin E synthase localizes to the superficial layers of human OA cartilage, which is where OA damage first occurs133. Upregulation of leukotriene production is also evident in OA joints. Arachidonic acid released from cell membranes in the OA synovial tissues, synovial fluid, cartilage, and subchondral bone128,136 can also be converted to the unstable precursor leukotriene A4 (LTA4) via arachidonate 5-lipoxygenase (5LO) and 5LO-activating proteins, with LTA4 in turn being converted to LTB4 by LTA4 hydrolase131.
NSAIDs and other COX2 inhibitors reduce prostaglandin production and inflammation in OA, but serve only to attenuate pain and are associated with a broad spectrum of adverse effects on the liver, kidney, cardiovascular system, gastrointestinal tract, and skin137. Several agents that inhibit both COX2 and COX1 (cyclooxygenase-1, also known as prostaglandin G/H synthase-1) enzymes as well as 5LO are currently in development as inhibitors of bioactive leukotriene generation, and their safety and efficacy in OA are being evaluated in clinical trials138 (TABLE 1).
Nitric oxide
NO might also be involved in inflammatory processes in OA139,140. Cytokines such as those prevalent in OA joints induce the expression of NO via the inducible NO synthase (iNOS) pathway141, and iNOS mRNA and protein have been found in the synovium of individuals with OA142. Indeed, high levels of nitrite have been detected in arthritic synovial fluid and serum143, consistent with the observation that NO production is abnormally high in OA cartilage144. NO has been proposed to contribute to the cartilage destruction in OA by enhancing expression of MMPs, inhibiting collagen and proteoglycan synthesis, and inducing apoptosis (in a process involving COX2-mediated production of PGE2)145,146. However, NO has also been proposed to have protective effects in OA by inhibiting the activation of proinflammatory pathways140. Although in vivo experiments in animal models suggest that inhibiting NO or iNOS could be an attractive avenue for treating OA32, the selective iNOS inhibitor cindunistat failed to slow the progression of OA in clinical trials147 (TABLE 1).
Neuropeptides
In the OA joint, neuropeptides have an active role not only in pain modulation, but also in the initiation and perpetuation of inflammation (by activating infiltrating inflammatory cells and by inducing production of proinflammatory cytokines)7,148. Examples of such proinflammatory neuropeptides include substance P, bradykinin, corticotropinreleasing factor, urocortin, and vasoactive intestinal peptide7,148. In OA, substance P is present in the subintimal portion of the synovium and in areas containing osteophytes or cartilage erosions, and can contribute to the inflammatory response by activating immune cells, stimulating the release of cytokines (such as IL-1β and TNF), inducing synoviocytes to proliferate and produce PGE2 and collagenase, and increasing osteoclast formation and synovial hypertrophy148. Bradykinin in the OA synovium can contribute to the initiation and maintenance of inflammation by activating synoviocytes and chondrocytes and possibly by synergistically potentiating the proinflammatory effects of cytokines149. However, intra-articular administration of the specific bradykinin B2 receptor antagonist icatibant in individuals with knee OA had a long-lasting analgesic effect149 but no detectable anti-inflammatory effect, as assessed by ultrasonography or MRI150 (TABLE 1).
Circadian clock dysrégulation
Disruption of the circadian rhythm could be another mechanism by which chronic inflammation contributes to OA pathogenesis. Findings published in 2016 indicated a role for the circadian clock component aryl hydrocarbon receptor nuclear translocator-like protein-1 (also known as brain and muscle Arnt-like protein-1 (BMAL1)), which is downregulated in OA cartilage, in maintaining homeostasis and integrity of cartilage tissue151. Indeed, environmental disruption of their circadian rhythms predisposes mice to OA-like damage of their knee cartilage152, and autonomous circadian rhythms in cartilage become dysregulated with age and with chronic inflammation, as well as in mouse models of injury-induced OA153156. Interestingly, a local circadian clock in pulmonary epithelial cells controls neutrophil recruitment to the lungs in response to local bacterial stimuli157, which could explain the diurnal variation in symptom severity of inflammatory lung diseases. Weakening of the local circadian clock in cartilage (and possibly in other joint tissues as well) might likewise contribute to the circadian variation seen in OA symptoms. Disruption of the cartilage circadian clock could conceivably both result from and contribute to inflammation in OA. In such a scenario, a vicious cycle operates in which chronic inflammation weakens the cartilage circadian clock, leading not only to a decrease in expression of proteins involved in cartilage anabolism151, and hence to cartilage loss, but also to an increase in expression of inflammatory cytokines151, and hence to the propagation of inflammation in the joints. Furthermore, circadian rhythms intrinsic to immune cells affect cytokine responses and immune cell trafticking158,159 and, therefore, dysregulation of the immune cell clock could potentially contribute to inflammation in OA.
Anti-inflammatory therapeutics for OA
Given the critical role of low-grade inflammation in OA, novel anti-inflammatory therapeutics could offer new opportunities for treating this disease. Although lifestyle changes involving exercise and/or diet-induced weight loss are simple and (in some individuals) effective139 ways of slowing the progression of OA, such regimens are difficult to implement in individuals with OA who are elderly or have limited mobility. Currently, only symptom-modifying agents, such as analgesics, NSAIDs, steroids, and hyaluronic acid, have been approved as therapeutic interventions for OA; that is, until joint replacement is needed139,160–162. None of the approved treatments can restore the original structure and function of damaged joints, nor can they slow the progression of OA. Moreover, these medications frequently have deleterious adverse effects. A substantial need remains for safe and effective disease-modifying OA drugs (DMOADs).
Inhibition of the low-grade inflammation in OA could form the basis of such much-needed DMOADs (FIG. 4). Because inflammation is present early in the course of OA, before structural changes have occurred30, therapeutic strategies targeting the low-grade inflammatory processes might be able to not only halt the progression of OA, but also prevent the onset of radiographic OA. Several anti-inflammatory therapeutics have been tested in human OA, but the results have thus far been disappointing139,160–162. Findings from the SEKOIA study suggest that strontium ranelate has disease-modifying effects in individuals with knee OA163,164, possibly through anti-inflammatory mechanisms involving inhibition of IL-1β165 and MMP166 production. Nonetheless, whether the benefits provided by strontium ranelate can actually prevent or delay joint replacement, and whether individuals with OA will be compliant in taking this drug (which has diverse adverse effects, including gastrointestinal disorders, thromboembolism, cardiovascular disorders, and allergy) for a period of time sufficient to prevent or slow the progression of OA (≥3 years164,167) remains unclear. Several promising findings showing disease-modifying effects of inhibitors of low-grade inflammation in animal models of OA warrant follow-up in human clinical trials (TABLE 1).
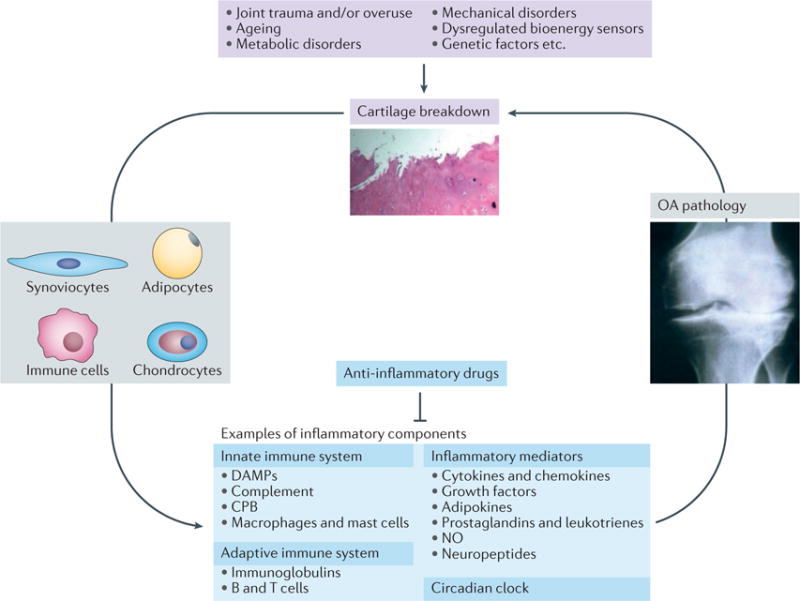
Can abrogating low-grade inflammatory responses break the feed-forward cycle of joint damage and breakdown leading to inflammation that promotes the pathogenesis of OA? Examples of risk factors for OA, cell types involved in its pathogenesis, and molecular components in the inflammatory pathways that are potential therapeutic targets for preventing or treating OA are shown. CPB, carboxypeptidase B; DAMPs, disease-associated molecular patterns; NO, nitric oxide; OA, osteoarthritis.
Because of the heterogeneity of OA, the large number of diverse molecules involved in its pathogenesis, and the multifaceted roles of these molecules under normal or pathologic conditions, effective treatment of OA might require a combination of approaches. Furthermore, teasing apart the precise roles of certain inflammatory components in different contexts will also be important, as targeting these components might not be feasible if they also have protective functions in tissue repair. That is, as we have learned from failed attempts at targeting IL-1β46–48 and MMPs94, anti-inflammatory therapies for OA will likely have to be broad enough to be effective, but selective enough to avoid deleterious off-target effects. Ongoing advances in imaging technologies and biomarker development should facilitate the testing of candidate therapies by enabling antiinflammatory interventions early in the course of OA — when they could be most effective — through earlier diagnosis and better stratification of patients, as well as earlier and more accurate assessment of clinical response to novel therapeutics.
Conclusions
Although the evidence supporting a role of chronic, low-grade inflammation in OA is well-established, only recently have we begun to appreciate that this inflammation has a pivotal role in the pathogenesis of OA. A further shift in our understanding has come with the realization that OA is a disease of the whole joint, affecting not only the articular cartilage, but also the synovium, tendons, muscles, ligaments, subchondral bone, and adipose tissue; perhaps it is even a systemic disease, with inflammation having a critical role in the interplay between the joint tissues. The complexity of the inflammatory mechanisms in OA pathophysiology is becoming apparent. Future work is needed to fully define the molecular pathways mediating the low-grade inflammation in OA, and to use this information either to repurpose existing drugs or candidate therapeutics that target these pathways, or to develop next-generation therapeutics. Ultimately, clinical trials are needed to determine whether targeting low-grade inflammation can indeed prevent or slow the development and progression of human OA.
Acknowledgments
The authors’ research is supported by the following awards (to W.H.R.): US Department of Veterans Affairs Merit Review Awards I01BX002345, I01RX000934 and I01RX000588; NIH National Institute of Arthritis and Musculoskeletal and Skin Diseases (NIAMS), National Institute of Allergy and Infectious Diseases (NIAID) and the Foundation for the NIH Accelerating Medicines Partnership Program UH2 AR067681; and the Northern California Chapter of the Arthritis Foundation (NCCAF) Center of Excellence.
Footnotes
Author contributions
W.H.R. and R.M. wrote the article. All authors researched the data for the article, contributed substantially to discussions of its content, and participated in review and/or editing of the manuscript before submission.Competing interests statement
J.S. is an employee of AbbVie. All other authors declare no competing interests.
References
Full text links
Read article at publisher's site: https://doi.org/10.1038/nrrheum.2016.136
Read article for free, from open access legal sources, via Unpaywall:
https://www.ncbi.nlm.nih.gov/pmc/articles/PMC5500215
Citations & impact
Impact metrics
Article citations
Engineered self-regulating macrophages for targeted anti-inflammatory drug delivery.
Arthritis Res Ther, 26(1):190, 06 Nov 2024
Cited by: 0 articles | PMID: 39501398 | PMCID: PMC11539832
Ononin delays the development of osteoarthritis by down-regulating MAPK and NF-κB pathways in rat models.
PLoS One, 19(10):e0310293, 31 Oct 2024
Cited by: 0 articles | PMID: 39480787 | PMCID: PMC11527302
Targeting FAP-positive chondrocytes in osteoarthritis: a novel lipid nanoparticle siRNA approach to mitigate cartilage degeneration.
J Nanobiotechnology, 22(1):659, 26 Oct 2024
Cited by: 0 articles | PMID: 39456041 | PMCID: PMC11515236
Effects of a feed supplement, containing undenatured type II collagen (UC II®) and Boswellia Serrata, in the management of mild/moderate mobility disorders in dogs: A randomized, double-blind, placebo controlled, cross-over study.
PLoS One, 19(10):e0305697, 30 Oct 2024
Cited by: 0 articles | PMID: 39475935 | PMCID: PMC11524509
Analyzing the Role of Specific Damage-Associated Molecular Patterns-Related Genes in Osteoarthritis and Investigating the Association between β-Amyloid and Apolipoprotein E Isoforms.
J Innate Immun, 16(1):501-512, 29 Oct 2024
Cited by: 0 articles | PMID: 39471788 | PMCID: PMC11521507
Go to all (611) article citations
Similar Articles
To arrive at the top five similar articles we use a word-weighted algorithm to compare words from the Title and Abstract of each citation.
Inflammation (or synovitis)-driven osteoarthritis: an opportunity for personalizing prognosis and treatment?
Scand J Rheumatol, 45(2):87-98, 20 Oct 2015
Cited by: 31 articles | PMID: 26484849
Review
Dysregulated integrin αVβ3 and CD47 signaling promotes joint inflammation, cartilage breakdown, and progression of osteoarthritis.
JCI Insight, 4(18):128616, 19 Sep 2019
Cited by: 34 articles | PMID: 31534047 | PMCID: PMC6795293
The role of cytokines in osteoarthritis pathophysiology.
Biorheology, 39(1-2):237-246, 01 Jan 2002
Cited by: 561 articles | PMID: 12082286
Review
Histomorphology and innate immunity during the progression of osteoarthritis: Does synovitis affect cartilage degradation?
J Cell Physiol, 233(2):1342-1358, 15 Jun 2017
Cited by: 27 articles | PMID: 28513840
Funding
Funders who supported this work.
BLRD VA (1)
Grant ID: I01 BX002345
NIAMS NIH HHS (2)
Grant ID: R01 AR063676
Grant ID: UH2 AR067681
RRD VA (2)
Grant ID: I01 RX000588
Grant ID: I01 RX000934